1. Introduction
Regional hinterland-dipping faults realizing younger-on-older contacts in the hinge/forelimb zone of prominent thrust-related anticlines are commonly found in orogenic belts that have experienced both pre-orogenic and post-orogenic extensional events. Determining the tectonic origin of these faults is frequently problematic because of the absence of adequate constraints to date the extension and contraction (e.g. Tavarnelli, Reference Tavarnelli1999); in particular, if only the geometrical relationships between thrusts and normal faults are considered, the interpretation of their relative age remains controversial. Examples from the Central Apennine thrust belt of Italy have been contrastingly interpreted by different authors as (1) younger-on-older thrust faults within out-of-sequence thrust systems (Ghisetti & Vezzani, Reference Ghisetti and Vezzani1991; Carminati et al. Reference Carminati, Fabbi and Santantonio2013); (2) regional strike-slip faults (Montone & Salvini Reference Montone and Salvini1991; Corrado et al. Reference Corrado, Di Bucci, Naso, Giampaolo and Adatte1998); (3) pre-thrusting normal faults (Tavarnelli, Reference Tavarnelli1996; Calamita et al. Reference Calamita, Pizzi, Ridolfi, Rusciadelli and Scisciani1998, Reference Calamita, Satolli, Scisciani, Esestime and Pace2011, Reference Calamita, Di Domenica and Pace2018; Scisciani et al. Reference Scisciani, Calamita, Tavarnelli, Rusciadelli, Ori and Paltrinieri2001 a; Mazzoli et al. Reference Mazzoli, Deiana, Galdenzi, Cello, Bertotti, Schulmann and Cloetingh2002; Pace et al. Reference Pace, Di Domenica and Calamita2014); (4) normal faults related to syn-thrusting activity (Migliorini, Reference Migliorini1948; Ghisetti & Vezzani, Reference Ghisetti and Vezzani2000; Storti et al. Reference Storti, Balsamo, Mozafari, Koopman, Swennen and Taberner2018); (5) back-thrusts related to Neogene Apennine thrusting (Patacca et al. Reference Patacca, Scandone, Di Luzio, Cavinato and Parotto2008; Fabbi & Smeraglia Reference Fabbi and Smeraglia2020); and (6) normal faults related to late-thrusting extensional collapse (Tavani et al. Reference Tavani, Storti, Bausà and Muñoz2012).
Generally, foreland domains ahead of contractional orogens are characterized by normal faulting related to lithospheric flexure during mountain building (Boccaletti et al. Reference Boccaletti, Calamita, Deiana, Gelati, Massari, Moratti and Ricci Lucchi1990; Bradley & Kidd, Reference Bradley and Kidd1991; Doglioni, Reference Doglioni1995; Tavani et al. Reference Tavani, Storti, Lacombe, Corradetti, Muñoz and Mazzoli2015). These faults can be reactivated with reverse kinematics, displaced and/or rotated until reaching an overturned attitude during the progressive migration of orogenic contraction into the foreland (Butler, Reference Butler2009; Calamita et al. Reference Calamita, Di Domenica and Pace2018). Moreover, foreland domains interposed between converging thrust belts with opposed sense of shear (e.g. Adriatic foreland) can experience repeated pulses of contractional deformation and reworking of pre-existing normal faults (Ziegler et al. Reference Ziegler, Cloetingh and van Wees1995; Lacombe & Mouthereau, Reference Lacombe and Mouthereau2002; Fantoni & Franciosi, Reference Fantoni and Franciosi2010; Scisciani et al. Reference Scisciani, Agostini, Calamita, Cilli, Giori, Pace and Paltrinieri2010, Reference Scisciani, Patruno, Tavarnelli, Calamita, Pace, Iacopini, Wilson, Houseman, McCaffrey, Doré and Buiter2019; Pace et al. Reference Pace, Scisciani and Calamita2011, Reference Pace, Satolli and Calamita2012, Reference Pace, Calamita, Tavarnelli, Mukherjee and Mulchrone2015 a; Toscani et al. Reference Toscani, Bonini, Ahmad, Di Bucci, Di Giulio, Seno and Galuppo2014; Lacombe & Bellahsen, Reference Lacombe and Bellahsen2016; Turrini et al. Reference Turrini, Toscani, Lacombe and Roure2016; Pfiffner, Reference Pfiffner2017; Tavarnelli et al. Reference Tavarnelli, Scisciani, Patruno, Calamita, Pace, Iacopini, Koeberl and Bice2019). Thrust-belt-foreland systems developing along previously rifted continental margins are characterized by interference structures showing a variable degree of complexity in the structural geometries across the hinterland to the foreland (e.g. Butler et al. Reference Butler, Tavarnelli and Grasso2006 a, b). Distinction between extensional and contractional structural signatures can be found in mildly deformed forelands. Indeed, examples of foreland up-thrusts as inversion structures resulting from multiple reverse reactivations of Mesozoic normal faults during the Early Cretaceous – Palaeogene and the Pliocene–Quaternary contraction, coeval with the advancing Dinaric and Apennine orogenic systems respectively, have been identified in the Adriatic foreland along the Mid-Adriatic Ridge (e.g. Scisciani, Reference Scisciani2009; Scisciani & Calamita, Reference Scisciani and Calamita2009; Pace et al. Reference Pace, Scisciani, Calamita, Butler, Iacopini, Esestime and Hodgson2015 b; Figs 1, 2).
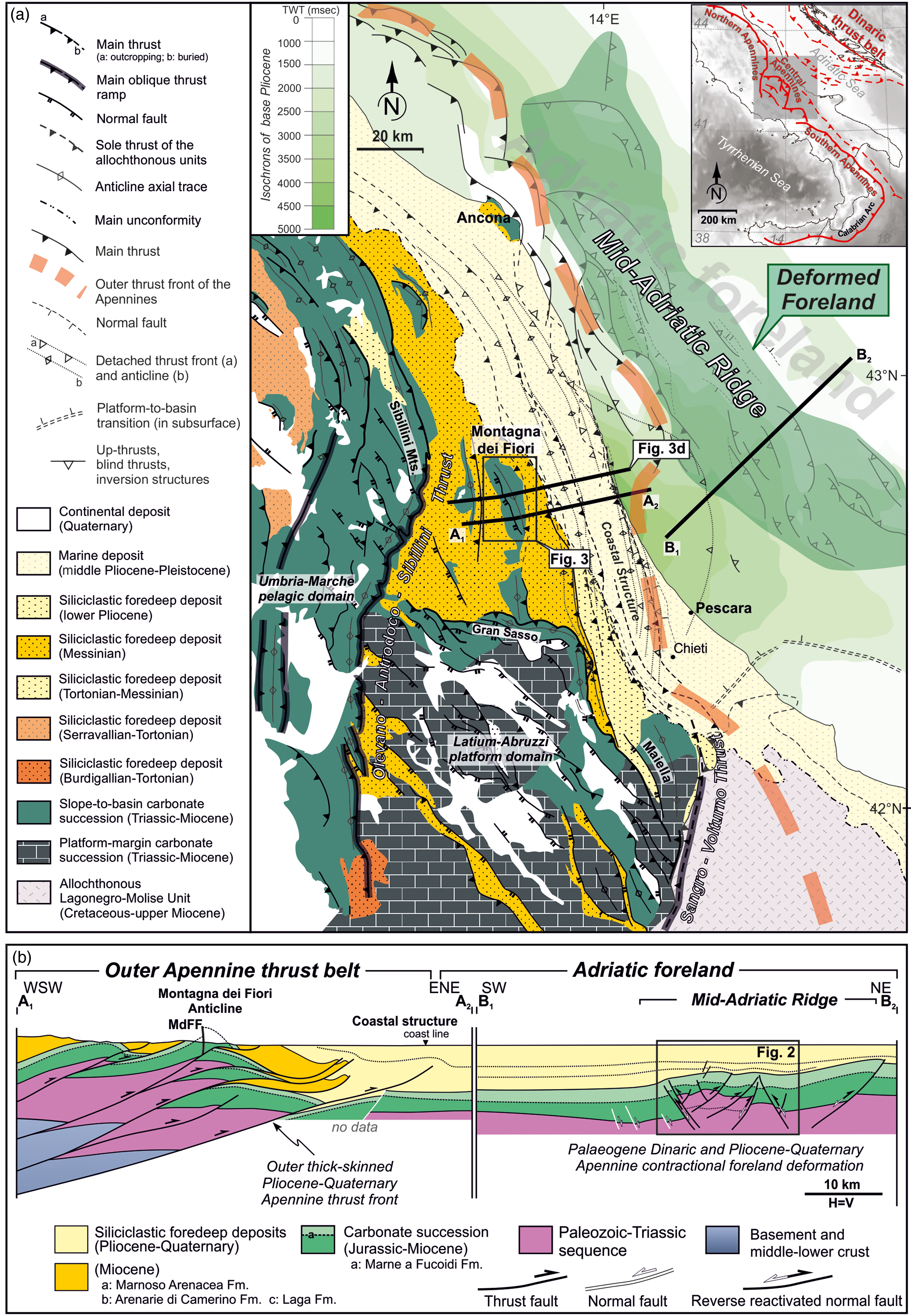
Fig. 1. (a) Structural map of the Neogene–Quaternary Central–Northern Apennine fold-and-thrust belt and the Adriatic foreland. The latter is interposed between the Apennines to the west, and the Dinaric Chain to the east (upper right inset for reference). Foreland deformation occurred by Upper Cretaceous – Miocene (Dinaric phase) contractional structures rejuvenated during Pliocene–Quaternary Apennine thrusting belonging to the Mid-Adriatic Ridge (modified from Scisciani & Calamita, Reference Scisciani and Calamita2009; Pace et al. Reference Pace, Scisciani, Calamita, Butler, Iacopini, Esestime and Hodgson2015 b).
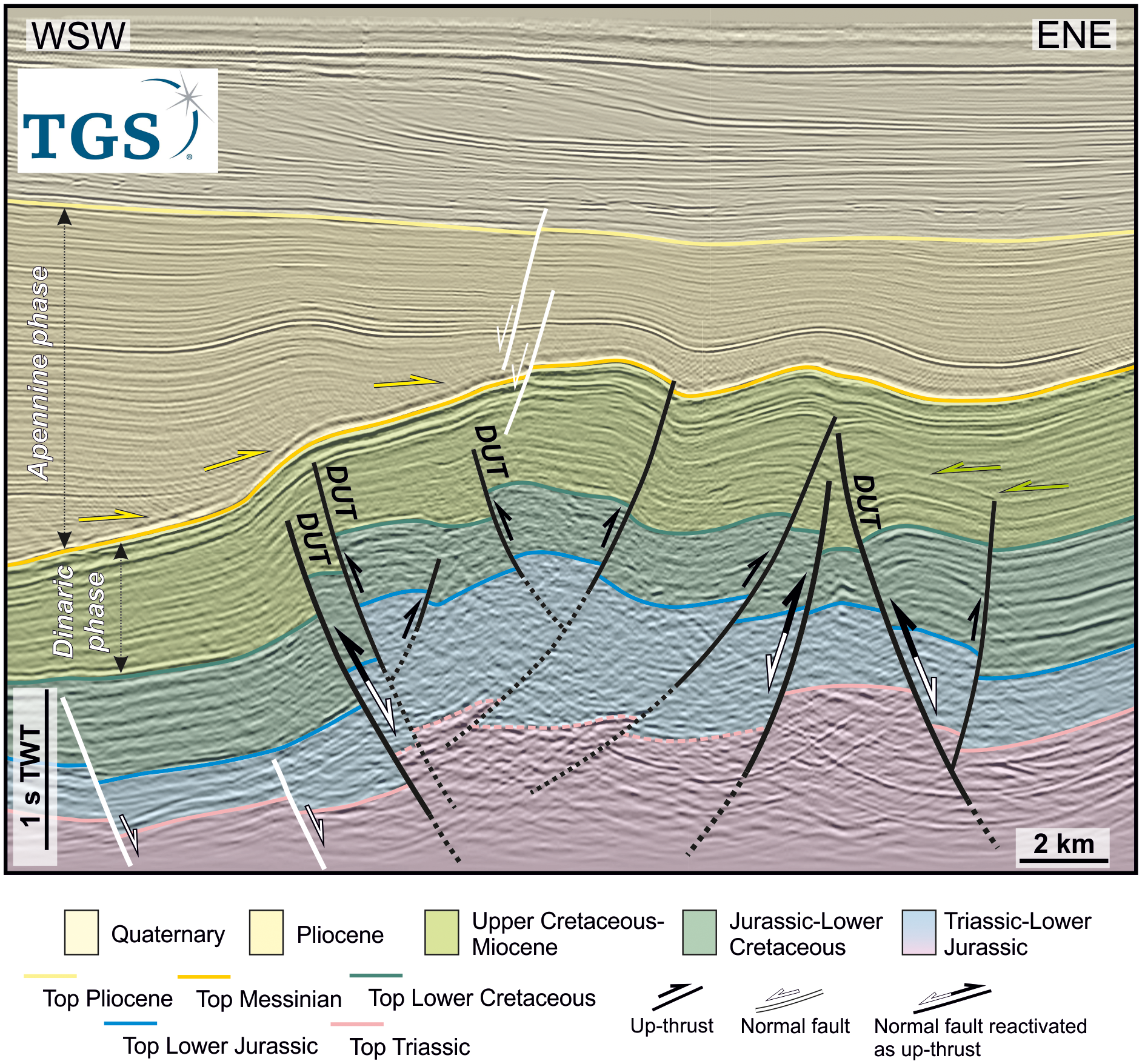
Fig. 2. Seismic section from the Mid-Adriatic Ridge showing an inversion structure composed of several Dinaric up-thrusts (DUT) and related folds having an Upper Cretaceous – Oligocene growth coeval with the onset of the Dinaric foreland contractional deformation and a later reactivation during the Pliocene–Quaternary Apennine phase (modified from Pace et al. Reference Pace, Scisciani, Calamita, Butler, Iacopini, Esestime and Hodgson2015 b).
In the outer zones of orogenic belts, precursor structures developed during the tectonic history of a foreland domain that has experienced repeated events of extension and contraction are usually difficult to decipher since they are strongly overprinted by the progressive incorporation of the foreland into the thrust belt. As a consequence, the unambiguous interpretation of the regional hinterland-dipping faults within the thrust-related anticlines as pre-, syn- or post-thrusting remains challenging, particularly when absolute timing constraints are lacking as a result of the incompleteness of the stratigraphic record.
In the outer sector of the Central Apennine foothills, the Montagna dei Fiori Anticline is a NNW–SSE-trending thrust-related fold characterized by an overturned forelimb, developed during lower Pliocene foreland thrusting (Calamita et al. Reference Calamita, Cello and Deiana1994; Tozer et al. Reference Tozer, Butler, Chiappini, Corrado, Mazzoli and Speranza2006) (Fig. 1). A hinterland- and SW-dipping regional fault (i.e. the Montagna dei Fiori Fault or MdFF), sub-parallel to the anticline axial trend, characterizes the hinge-forelimb zone of the fold. Various interpretations have been proposed for the MdFF, with either a Miocene pre-thrusting normal fault related to foreland flexural processes (Calamita et al. Reference Calamita, Pizzi, Ridolfi, Rusciadelli and Scisciani1998; Scisciani et al. Reference Scisciani, Calamita, Tavarnelli, Rusciadelli, Ori and Paltrinieri2001 a, b, Reference Scisciani, Tavarnelli and Calamita2002; Mazzoli et al. Reference Mazzoli, Deiana, Galdenzi, Cello, Bertotti, Schulmann and Cloetingh2002) or a Pliocene–Quaternary syn-thrusting normal fault (Ghisetti & Vezzani, Reference Ghisetti and Vezzani2000; Storti et al. Reference Storti, Balsamo, Mozafari, Koopman, Swennen and Taberner2018).
This study provides a reappraisal of the structural–geological development of the MdFF by integrating original stratigraphic and structural data and observations with extensive previously published geological and structural information. The newly acquired dataset allows the development of an alternative pre-thrusting structural model for the Montagna dei Fiori structure and the proposal of a new interpretation of the tectonic origin of the MdFF. The newly proposed pre-Apennines model for the Montagna dei Fiori structure, involved in the frontal Pliocene–Quaternary antiformal stack of the Central Apennines, is compared with subsurface analogues that can be found among the Palaeogene–Quaternary structures of the Mid-Adriatic ridge in the Adriatic foreland (Fig. 2).
2. Regional geological framework
The Apennines of Italy are a fold-and-thrust belt developed during the Neogene–Quaternary time interval in response to the convergence between Africa and Europe and following the closure of the Mesozoic Tethys Ocean during the Alpine Orogeny (e.g. Carminati & Doglioni, Reference Carminati and Doglioni2012). The orogeny affected Triassic–Miocene sedimentary successions deposited in different carbonate-dominated domains developed along the Mesozoic Adria continental margin (Ciarapica & Passeri, Reference Ciarapica and Passeri2002). In addition, orogenesis was accompanied by the progressive development of foredeep and piggyback basins migrating towards the foreland from the innermost Oligocene – lower Miocene basin to the present-day Pliocene–Quaternary Adriatic Basin (Boccaletti et al. Reference Boccaletti, Calamita, Deiana, Gelati, Massari, Moratti and Ricci Lucchi1990). The geometry of the Apennine thrust belt is arranged in two NE- to SE-verging arcs – the Northern and Southern Apennines – which are connected through the Central Apennine thrust system via two regional-scale oblique thrust ramps (Calamita et al. Reference Calamita, Satolli, Scisciani, Esestime and Pace2011; Satolli et al. Reference Satolli, Pace, Viandante and Calamita2014).
The Central–Northern Apennines foothills are characterized by fold-and-thrust structures involving a sedimentary cover composed of pre-orogenic Mesozoic–Palaeogene carbonates and Neogene syn-orogenic, siliciclastic, foredeep deposits. The ENE-directed outer Apennine thrust system is stacked onto the undeformed Adriatic plate, representing a common foreland domain shared with the WSW-directed Dinarides thrust system (Fig. 1). In the Apennine–Adriatic thrust-belt–foreland system, faults developed prior to Apennine thrusting that recorded the structural and stratigraphic signature of pre-orogenic extension are widely recognized. These faults record a polyphase tectonic history, with repeated events of basin formation associated with normal faulting (i.e. Jurassic rifting and Cretaceous–Tertiary foreland extension), and were later variously reworked by the contractional deformation during the Upper Cretaceous – Palaeogene Dinaric and Pliocene–Quaternary Apennine thrust belt development (e.g. Tavarnelli & Peacock, Reference Tavarnelli and Peacock1999; Scisciani et al. Reference Scisciani, Calamita, Tavarnelli, Rusciadelli, Ori and Paltrinieri2001 a, b, Reference Scisciani, Tavarnelli and Calamita2002, Reference Scisciani, Agostini, Calamita, Pace, Cilli, Giori and Paltrinieri2014; Di Domenica et al. Reference Di Domenica, Turtù, Satolli and Calamita2012, Reference Di Domenica, Bonini, Calamita, Toscani, Galuppo and Seno2014; Cardello & Doglioni, Reference Cardello and Doglioni2014; Pace et al. Reference Pace, Pasqui, Tavarnelli and Calamita2017, Reference Pace, Di Cuia, Mascolo, Hammerstein, Di Cuia, Cottam, Zamora and Butler2020; Calamita et al. Reference Calamita, Di Domenica and Pace2018).
In the inner zone of the Apennine thrust belt, Quaternary and still-active post-orogenic normal faults dissect the axial zone of the Apennine mountain ridge, whereas in the Apennine foreland, Pliocene–Quaternary thrusts and folds are buried below the syn-orogenic sediments beneath the Po Plain and in the Adriatic Basin (e.g. Doglioni, Reference Doglioni1991; Barchi et al. Reference Barchi, De Feyter, Magnani, Minelli, Pialli and Sotera1998; Finetti et al. Reference Finetti, Boccaletti, Bonini, Del Ben, Geletti, Pipani and Sani2001). The NW–SE-oriented Mid-Adriatic Ridge, developed in the central zone of the Adriatic foreland, represents a polyphase deformed area characterized by up-thrust faults (i.e. basement-rooted, convex-upwards, high-angle reverse faults developing in deformed forelands, sensu Prucha et al. Reference Prucha, Graham and Nickelson1965; Sales, Reference Sales1968) and thrust-related folds resulting from the Palaeogene (i.e. Dinaric phase; Figs 1, 2) and Pliocene–Quaternary (i.e. Apennine phase) reverse/transpressional reactivation of rift-inherited Mesozoic normal faults (Scisciani & Calamita, Reference Scisciani and Calamita2009; Pace et al. Reference Pace, Scisciani, Calamita, Butler, Iacopini, Esestime and Hodgson2015 b).
The Neogene arcuate thrusts of the Olevano–Antrodoco-Mt Sibillini and Gran Sasso represent the Umbria–Marche and Latium–Abruzzi Apennine mountain fronts, respectively. In the footwall of these thrust fronts, the Messinian siliciclastic deposits of the Laga Formation are widely exposed within the former foreland basin (Bigi et al. Reference Bigi, Casero and Ciotoli2011). Contractional structures occur within the Laga Basin and consist of two main double-plunging thrust-related anticlines, namely, the Acquasanta and Montagna dei Fiori anticlines, exposing the pre-orogenic Mesozoic carbonate sequence in the fold core (Fig. 1). These anticlines represent the upper hanging-wall structure of the Pliocene–Quaternary antiformal stack of the Central Apennines foothills (Paltrinieri et al. Reference Paltrinieri, Zanchini, Martini and Roccia1982; Scisciani & Montefalcone, Reference Scisciani, Montefalcone, Mazzoli and Butler2006; Artoni, Reference Artoni2007; Scisciani & Calamita, Reference Scisciani and Calamita2009).
This sector of the Central Apennine thrust belt has received much attention during the last 60 years, with Montagna dei Fiori being a natural laboratory site for investigating foreland thrust tectonics, structural inheritance, basement-involved thrusting, thrust-related folding, fracture patterns and studies of outcrop analogues for hydrocarbon reservoirs (Giannini, Reference Giannini1960; Giannini et al. Reference Giannini, Lazzarotto and Zampi1970; Koopman, Reference Koopman1983; Mattei, Reference Mattei1987; Calamita et al. Reference Calamita, Pizzi, Ridolfi, Rusciadelli and Scisciani1998; Scisciani et al. Reference Scisciani, Tavarnelli and Calamita2002; Ronchi et al. Reference Ronchi, Casaglia and Ceriani2003; Scisciani & Montefalcone, Reference Scisciani, Montefalcone, Mazzoli and Butler2006; Tozer et al. Reference Tozer, Butler, Chiappini, Corrado, Mazzoli and Speranza2006; Di Francesco et al. Reference Di Francesco, Fabbi, Santantonio, Bigi and Poblet2010; Storti et al. Reference Storti, Balsamo and Koopman2017, Reference Storti, Balsamo, Mozafari, Koopman, Swennen and Taberner2018; Francioni et al. Reference Francioni, Pace, Vitulli, Sciarra and Calamita2019; Mozafari et al. Reference Mozafari, Swennen, Balsamo, El Desouky, Storti and Conxita2019).
2.a. Montagna dei Fiori Anticline
The Montagna dei Fiori Anticline is the most prominent structure in the outer Central Apennine fold-and-thrust belt, and consists of a NNW–SSE-trending, thrust-related fold (Figs 1, 3). The anticline is E-verging and is exposed for an along-strike length of c. 25 km with a general N150E trend. The fold profile is asymmetric, with a steep-to-overturned forelimb and a gently dipping (30–50°) backlimb exhibiting a hinge line that plunges NNW- and S-wards (Fig. 3). The fold-and-thrust structure involves the pre-orogenic, Jurassic–Miocene, Umbria–Marche pelagic carbonate sequence overlain by Messinian syn-orogenic, foredeep siliciclastic deposits of the Laga Formation (Giannini, Reference Giannini1960; Giannini et al. Reference Giannini, Lazzarotto and Zampi1970; Koopman, Reference Koopman1983; Mattei, Reference Mattei1987; Calamita et al. Reference Calamita, Pizzi, Ridolfi, Rusciadelli and Scisciani1998; Scisciani et al. Reference Scisciani, Calamita, Tavarnelli, Rusciadelli, Ori and Paltrinieri2001 a, b, Reference Scisciani, Tavarnelli and Calamita2002; Scisciani & Montefalcone, Reference Scisciani, Montefalcone, Mazzoli and Butler2006; Di Francesco et al. Reference Di Francesco, Fabbi, Santantonio, Bigi and Poblet2010; Storti et al. Reference Storti, Balsamo and Koopman2017; Francioni et al. Reference Francioni, Pace, Vitulli, Sciarra and Calamita2019).
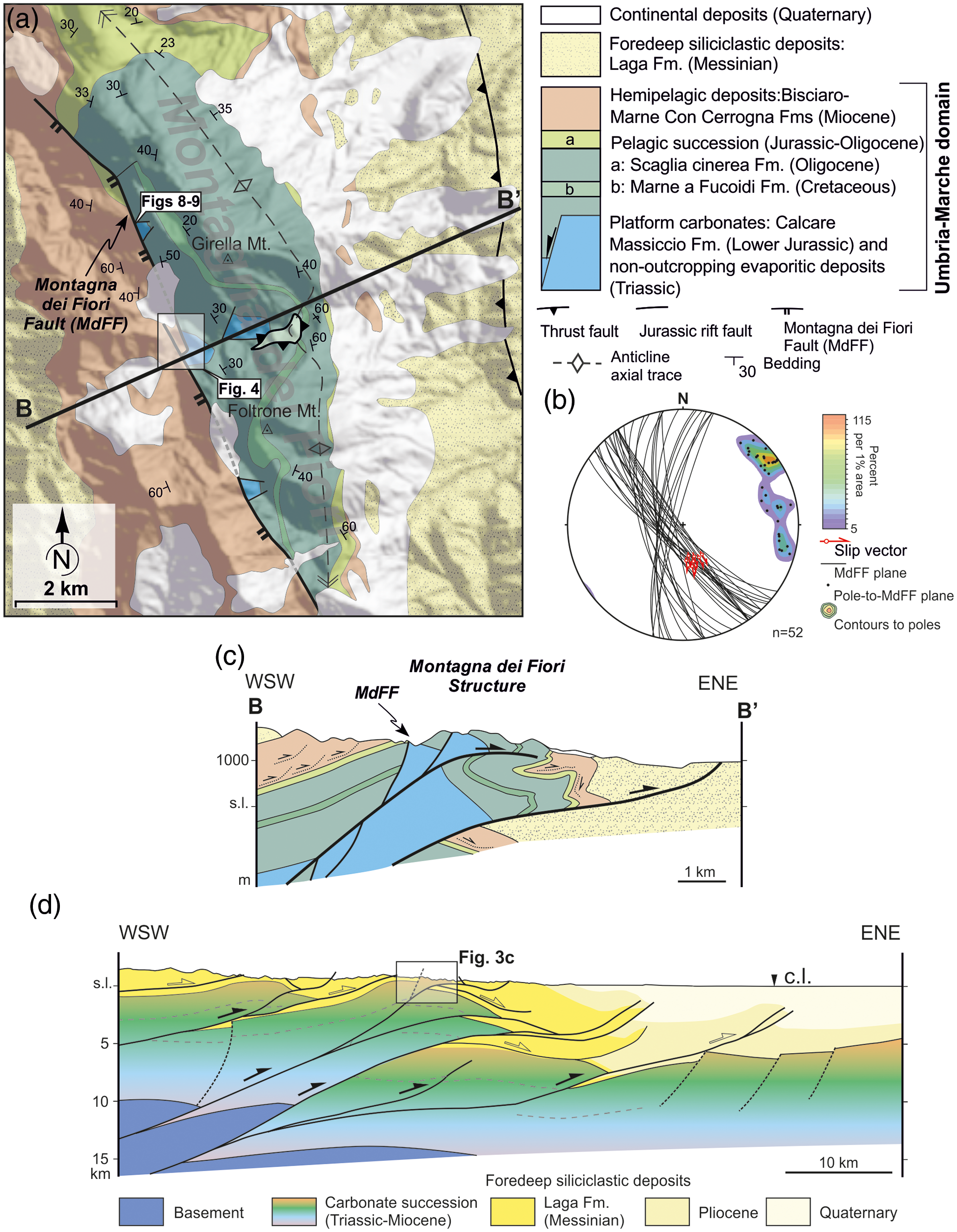
Fig. 3. (a) Geological map of the Montagna dei Fiori structure (modified from Mattei, Reference Mattei1987; Calamita et al. Reference Calamita, Satolli, Scisciani, Esestime and Pace2011). (b) Equal-area lower-hemisphere stereographic projection showing the collected data along the MdFF plane. (c) Cross-section through the Montagna dei Fiori structure showing the Pliocene thrusts and related overturned anticline; the Montagna dei Fiori Fault (MdFF) causes the younger-on-older juxtaposition of the Miocene Marne con Cerrogna Formation on the Jurassic–Tertiary carbonate succession. (d) Crustal-scale cross-section showing the relative position of the Montagna dei Fiori structure within the antiformal stack of the outer Central Apennines, location in Figure 1 (modified from Scisciani & Montefalcone, Reference Scisciani, Montefalcone, Mazzoli and Butler2006).
The main thrust surface, exposed in the fold core, is antiformally folded by the growth of a younger and deeper thrust-related anticline. This is supported by several proposed interpretations of seismic reflection profiles (Bally et al. Reference Bally, Burbi, Cooper and Ghelardoni1986; Ghisetti & Vezzani, Reference Ghisetti and Vezzani2000; Scisciani & Montefalcone, Reference Scisciani, Montefalcone, Mazzoli and Butler2006; Artoni, Reference Artoni2013). Eastwards, a gently inclined, W-dipping thrust cross-cuts the Messinian siliciclastic deposits of the Laga Formation with strata dipping gently towards the east (Calamita, Reference Calamita1990; Scisciani & Montefalcone, Reference Scisciani, Montefalcone, Mazzoli and Butler2006).
The E-dipping and overturned frontlimb of the Montagna dei Fiori Anticline has a NW–SE trend in the northern sector, changing from axial culmination S-wards to a N–S orientation. Contractional shear zones characterized by S-C (schistosité–cisaillement) fabric and parasitic folds occur along the forelimb of the anticline. The kinematic indicators consistently show a top-to-NE (60–70° N) tectonic transport direction (Koopman, Reference Koopman1983). Reverse shear zones and detachment folds are also exposed in the backlimb of the anticline. The shear zones are confined to the upper section of the Miocene marly sequence, whereas the overlying Messinian turbidites (i.e. Laga Formation) appear undeformed (Koopman, Reference Koopman1983; Invernizzi & Ridolfi, Reference Invernizzi and Ridolfi1992; Calamita et al. Reference Calamita, Pizzi, Ridolfi, Rusciadelli and Scisciani1998; Mazzoli et al. Reference Mazzoli, Deiana, Galdenzi, Cello, Bertotti, Schulmann and Cloetingh2002; Scisciani & Montefalcone, Reference Scisciani, Montefalcone, Mazzoli and Butler2006). The similarity between the geometries and kinematics of the shear zones observed along the flanks of the Montagna dei Fiori Anticline indicates that the Palaeogene–Miocene pre-orogenic succession acted as a regional detachment level, along which the thick sedimentary wedge of the Laga Formation was translated foreland-wards with respect to the underlying Mesozoic sequence (Laga Detachment sensu Koopman, Reference Koopman1983).
The deep (> 10 km) structural setting of the Montagna dei Fiori structure has been debated by many authors, and both thin- and thick-skinned tectonic models have been applied to interpret the tectonic style of this sector of the outer Central Apennines (Paltrinieri et al. Reference Paltrinieri, Zanchini, Martini and Roccia1982; Bally et al. Reference Bally, Burbi, Cooper and Ghelardoni1986; Calamita et al. Reference Calamita, Cello and Deiana1994; Tozer et al. Reference Tozer, Butler, Corrado, Bertotti, Schulmann and Cloetingh2002; Albouy et al. Reference Albouy, Casero, Eschard, Rudkiewicz and Barrier2003; Scisciani & Montefalcone, Reference Scisciani, Montefalcone, Mazzoli and Butler2006).
2.b. Montagna dei Fiori Fault
The regional (10–15-km long), SW-dipping MdFF (Fig. 3) affects the crestal zone of the Montagna dei Fiori Anticline and juxtaposes the hanging-wall Miocene Marne con Cerrogna Formation to the pre-orogenic carbonate sequence with a WSW-wards offset of approximately 900 m (Mattei, Reference Mattei1987; Calamita et al. Reference Calamita, Pizzi, Ridolfi, Rusciadelli and Scisciani1998; Scisciani et al. Reference Scisciani, Tavarnelli and Calamita2002). Miocene pre-thrusting, normal fault activity has been proposed by Calamita et al. (Reference Calamita, Pizzi, Ridolfi, Rusciadelli and Scisciani1998), Scisciani et al. (Reference Scisciani, Calamita, Tavarnelli, Rusciadelli, Ori and Paltrinieri2001 a, b), Mazzoli et al. (Reference Mazzoli, Deiana, Galdenzi, Cello, Bertotti, Schulmann and Cloetingh2002) and Scisciani et al. (Reference Scisciani, Tavarnelli and Calamita2002), based on overprinting relationships between extensional and contractional structures and by stratigraphic thickness variations of the Marne con Cerrogna Formation across the fault. However, Storti et al. (Reference Storti, Balsamo, Mozafari, Koopman, Swennen and Taberner2018) suggested that this fault had a normal faulting role during Pliocene syn-thrusting activity, as previously proposed by Ghisetti & Vezzani (Reference Ghisetti and Vezzani2000).
Reconstructions showing low-angle thrust short-cut trajectories through the high-angle MdFF has highlighted a pre-thrusting origin (Scisciani et al. Reference Scisciani, Calamita, Tavarnelli, Rusciadelli, Ori and Paltrinieri2001 a, b, Reference Scisciani, Tavarnelli and Calamita2002; Calamita et al. Reference Calamita, Satolli, Scisciani, Esestime and Pace2011, Reference Calamita, Pace and Satolli2012; Pace & Calamita, Reference Pace and Calamita2014, Reference Pace and Calamita2015). The asymmetric ENE-verging, overturned anticline rides over a blind thrust and tips up-section into folded, thin-bedded, Cretaceous–Tertiary limestones. Based on this geometrical configuration, a simple fault-propagation folding was reconstructed by Di Francesco et al. (Reference Di Francesco, Fabbi, Santantonio, Bigi and Poblet2010) for the Montagna dei Fiori structure.
During compilation of a revisited structural map of the area, we collected new structural–geological data. The documented structures are exposed within the overstep between the two en échelon arranged segments of the MdFF system in the axial culmination of the anticline. The collected field data and observations allow us to suggest a novel interpretation for the MdFF and to propose an alternative pre-thrusting structural setting for the Montagna dei Fiori structure.
3. Geological and structural analysis of the MdFF
3.a. Field data and observations
The crestal zone of the Montagna dei Fiori Anticline is affected by the WSW-dipping (N240-N260) MdFF. The fault extends for an along-strike length of c. 12–14 km, running parallel to the anticlinal axial trend (Fig. 3). The fault system juxtaposes the weak Miocene hemipelagic succession (Marne Con Cerrogna Formation) in the hanging wall onto the mechanically stiff Jurassic–Cretaceous carbonate sequence in the footwall. At Colle Osso Caprino (COC in Fig. 4a, c), the well-exposed fault plane has a dip between 75° and 88°. In the anticline forelimb, c. 50 m from the MdFF footwall damage zone, bedding planes of the Corniola Formation have a constant dip of 30–40° towards the NE (Fig. 4c).
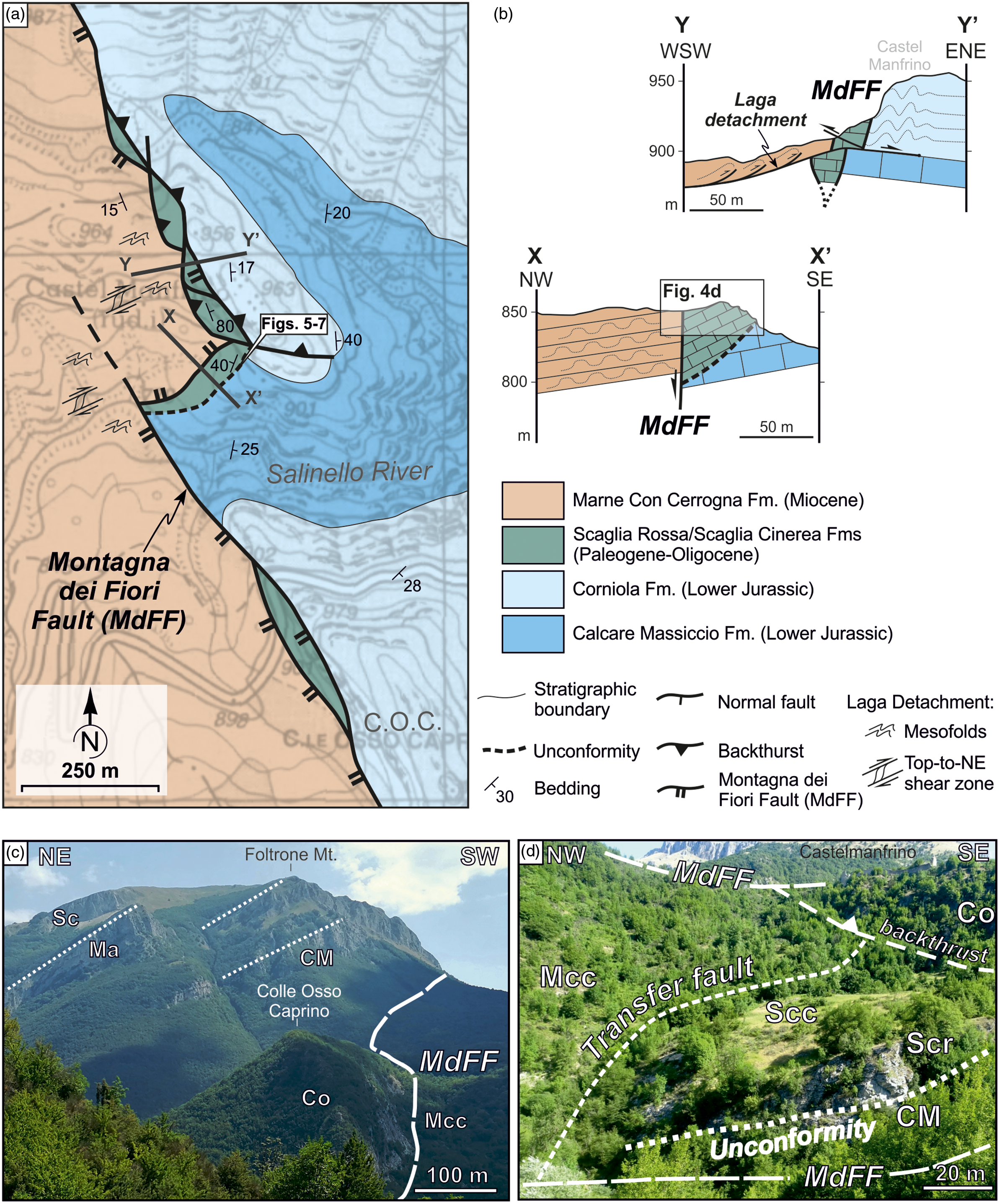
Fig. 4. (a) Geological and structural map of the stepover zone between the two NW–SE-trending en échelon master fault segments of the MdFF linked through the NE–SW-trending transverse fault. (b) Cross-section X–X' shows the transverse fault and the Scaglia Rossa and Scaglia Cinerea formations lying unconformably above the Calcare Massiccio and Corniola formations. Cross-section Y–Y' highlights the occurrence of a tectonic lens of Scaglia Rossa and Scaglia Cinerea formations displaced by a backthrust resulting from the MdFF as buttressing, as well as the mesoscale detachment folds affecting the Corniola Formation, related to the NE-directed Laga detachment. Top-to-NE shear zones and mesoscale folds within the Marne Con Cerrogna Formation represent the deformation related to the Laga Detachment (Koopman, Reference Koopman1983; Calamita et al. Reference Calamita, Pizzi, Ridolfi, Rusciadelli and Scisciani1998; COC – Colle Osso Caprino). (c) Panoramic view of the MdFF along the forelimb/hinge zone of the Montagna dei Fiori Anticline, showing the attitude of the footwall strata characterized by 30–40° dip towards the NE. (d) Panoramic view of the overstep zone with the NE–SW-trending transverse fault realizing the hard linkage between the two regional NW–SE-striking en échelon segments of the MdFF. The unconformity between the Scaglia Rossa and Scaglia Cinerea formations over the Calcare Massiccio Formation is also highlighted. Mcc – Marne con Cerrogna Formation; Scc – Scaglia Cinerea Formation; Sc/Scr – Scaglia Rossa Formation; Co – Corniola Formation; CM – Calcare Massiccio Formation.
In the Castel Manfrino area, the MdFF system is composed of two NW–SE-striking, right-stepping en échelon arranged segments (Fig. 4). Along the Salinello River valley, these fault segments are linked by a transverse fault segment with a NE–SW trend (Fig. 4). Within the MdFF system stepover, the collected stratigraphic and structural data allow us to infer pre-Apennine contractional activity along the MdFF. In this area, a transversal fault palaeo-scarp is exposed on the left cliffside of the Salinello River. Along this outcrop, the Lower–Middle Jurassic Calcare Massiccio – Corniola formations are unconformably overlain by the Scaglia Rossa Formation. Samples collected along this contact and field mapping indicate a reduced succession (8–10 m thick) from the Late Cretaceous (Campanian–Maastrichtian) to late Paleocene (Planorotalites pseudomenardii Biozone) to middle Eocene (transition from the Scaglia Rossa Formation to Scaglia Variegata Formation) periods, as constrained by biostratigraphic dating. The unconformity exhibits an irregular and wavy surface dipping towards the NW with an average angle of 40° (Fig. 5a–d). Below the unconformity, the shallow-water platform carbonates of the Calcare Massiccio Formation are typically massive with metre-thick beds; above it, the pelagic limestones and marly limestones of the Scaglia Rossa Formation with centimetre-thick chert intercalations show strata sub-parallel to the unconformity surface.

Fig. 5. (a–c) Exposures showing the unconformity along the palaeoscarp caused by the NE–SW-trending transverse fault along which the latest Cretaceous – Palaeogene Scaglia Rossa Formation (Scr) is juxtaposed onto the Lower Jurassic Calcare Massiccio Formation (CM). Layer-parallel pressure-solution seams (S) due to lithostatic loading are diffuse within the Scaglia Rossa above the unconformity (see the hammer, 33 cm in height, and the compass, 10 cm in height, for scale). (d) Schematic representation of the fault-controlled stratigraphic variation from latest Cretaceous – Palaeogene through middle Miocene time controlling the accommodation space of the Marne con Cerrogna Formation. (e) Equal-area lower-hemisphere stereographic projection showing the collected data on the exposed unconformity surface.
Pervasive millimetre- to centimetre-spaced, layer-parallel, pressure-solution cleavage affects the Scaglia Rossa Formation, which is very likely the result of lithostatic loading (Fig. 6). This persistent pressure-solution cleavage is involved in asymmetrical, decimetre-scale folds observable within a metre-scale deformation zone above the unconformity (Fig. 6a). Mesoscopic folding is also highlighted by centimetre-thick chert layers (Fig. 6b). Fold axes trend NW–SE on average and plunge gently (c. 20°) towards the NW (Fig. 6).

Fig. 6. (a) Close-up of 2-m-thick deformation beam above the unconformity showing the well-developed layer-parallel pressure-solution seams within the Scaglia Rossa Formation (Scr) affected by asymmetrical mesoscopic folds. (b) The mesoscopic folding is also highlighted by the centimetre-thick chert layers involved in the deformation. The equal-area lower-hemisphere stereographic projection reveal fold axial trends oriented NW–SE on average, plunging about 20° towards the NW.
NW–SE- and NNW–SSE-trending mesoscopic conjugate normal faults, with decimetre- to metre-scale offsets, affect the Scaglia Rossa Formation above the unconformity (Fig. 7a). Sub-horizontal pressure-solution seams are evident within the marly limestones and fault-bounded wedges (Fig. 7). Some of the extensional mesoscopic faults also displace the unconformity surface with metre-scale displacements, and they have caused a local juxtaposition of the Scaglia Rossa Formation onto the Calcare Massiccio Formation (Fig. 7b).
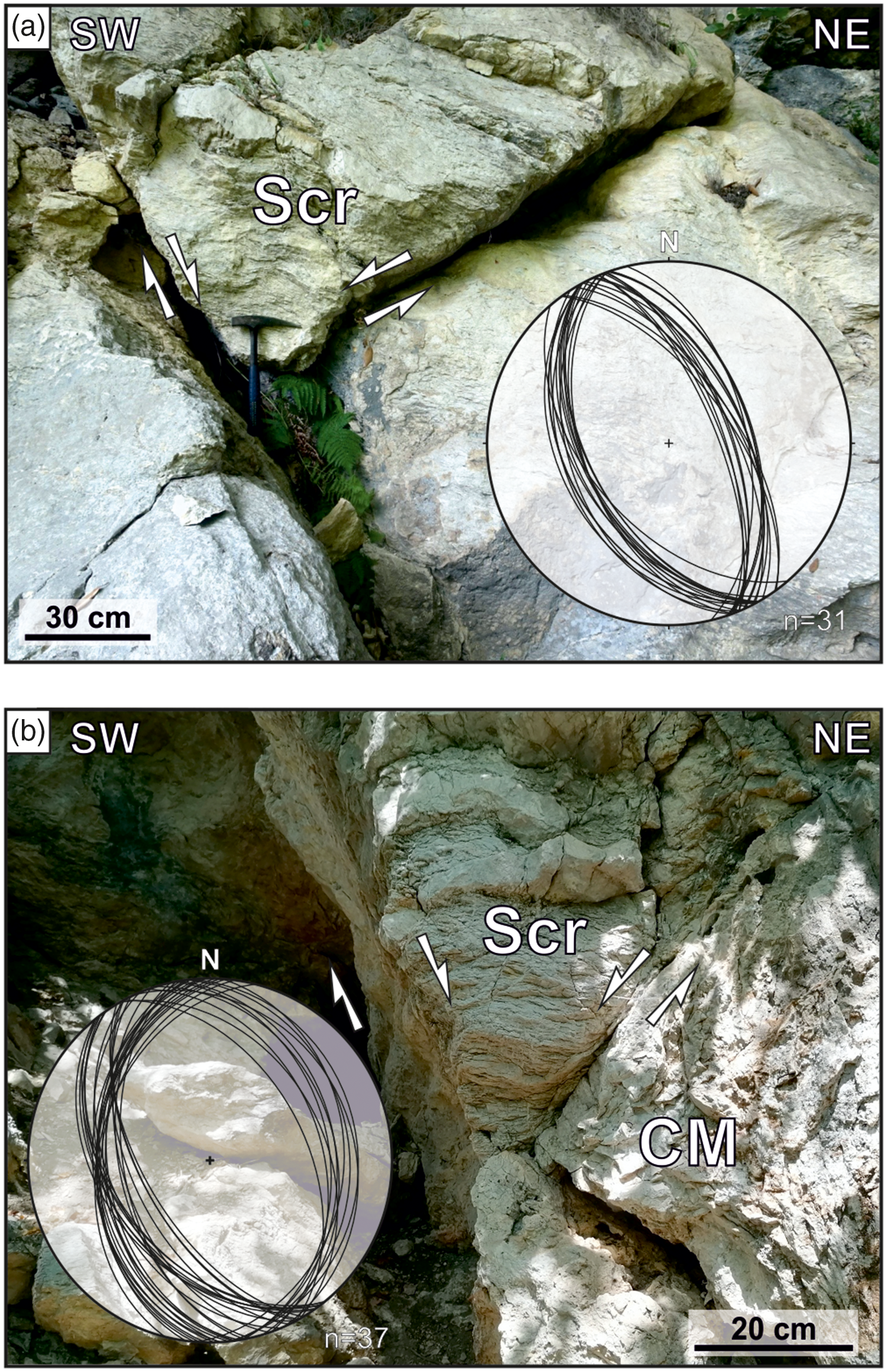
Fig. 7. (a) NW–SE-trending mesoscopic conjugate normal faults and sub-horizontal pressure-solution seams involving the Scaglia Rossa Formation above the unconformity. (b) The outcrop-scale conjugate fault systems also displace the unconformity surface with metre-scale offsets, causing a local juxtaposition of the Scaglia Rossa Formation (Scr) onto the Calcare Massiccio Formation (CM).
Moving laterally along the strike of the two MdFF en échelon segments, decametric tectonic lenses of Scaglia Rossa and Scaglia Cinerea Formations occur both NW-wards and SE-wards of the Salinello River. These lenses, made up of Late Cretaceous – Palaeogene rocks, are interposed between the Jurassic formations in the MdFF footwall and the Marne con Cerrogna Formation, which is widely exposed in the MdFF hanging wall. Penetrative S-C shear fabrics are developed within these tectonic lenses (Fig. 8a, b). The diffuse contractional S-C fabric is characterized by both low- and high-angle, C-type conjugate, reverse shear planes and associated high-angle (60–75°), SW-dipping, pressure-solution, S-cleavage surfaces, which are sub-parallel to the MdFF plane, dipping 60–80° towards the SW (Fig. 8a). The C-shears offset the MdFF plane with a centimetre-to-decimetre scale displacement (Fig. 8b). Another S-C shear fabric with extensional kinematics can be observed within the tectonic lenses of the Scaglia Rossa and Scaglia Cinerea formations along the MdFF damage zone (Fig. 9a), as also documented by Storti et al. (Reference Storti, Balsamo, Mozafari, Koopman, Swennen and Taberner2018). The extensional shear fabric is composed of 60–85°-inclined C-shear planes and 30–40°-inclined S-surfaces, both dipping towards the WSW (Fig. 9a). The contractional and extensional shear fabrics show mutual cross-cutting relationships with high-angle, top-to-WSW extensional, C-shears displacing the low-angle, top-to-NE C-planes and vice versa (Fig. 9a).
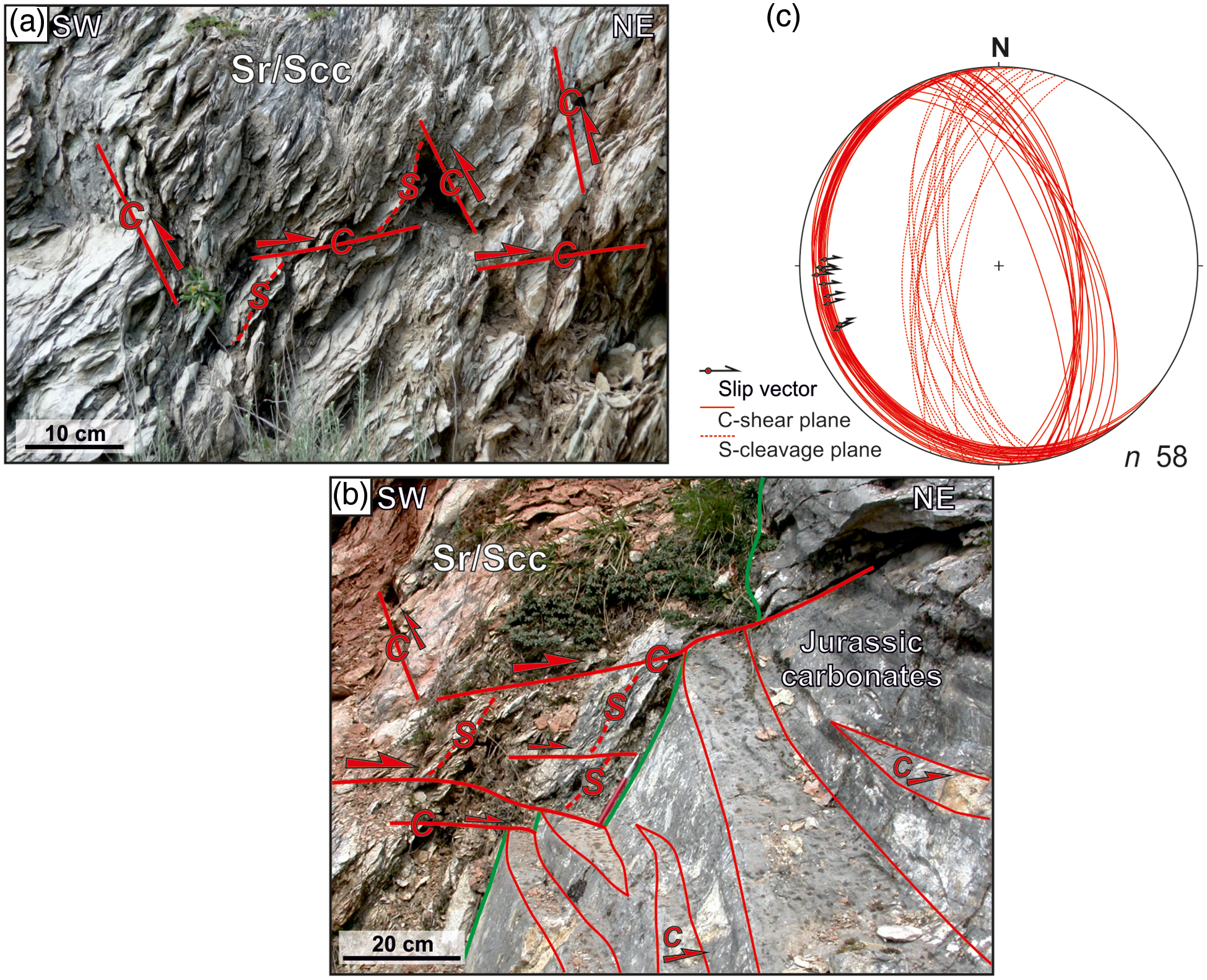
Fig. 8. (a) Contractional S-C fabric within a tectonic slice of Scaglia Rossa and Scaglia Cinerea formations along the MdFF damage zone. (b) High-angle SW-dipping pressure-solution S-cleavage surfaces are sub-parallel to the MdFF plane (green outline), dipping 60–80° towards the SW; low-angle C-type conjugate reverse shear planes offset the MdFF plane accommodating a decimetre-to-metre scale offset. (c) Equal-area lower-hemisphere stereographic projection of the contractional S-C fabric with top-to-NE kinematics.

Fig. 9. (a) Mutual cross-cutting relationships between the contractional and the extensional S-C fabrics within a tectonic slice of Scaglia Rossa and Scaglia Cinerea formations along the MdFF damage zone. (b) Equal-area lower-hemisphere stereographic projection of the extensional S-C fabric with top-to-SW kinematics. (c) By restoring the MdFF footwall bedding to the horizontal, the extensional fabric turns into (d) a contractional fabric with a top-to-SW kinematics.
3.b. Revisited tectonic evolution of the MdFF
The Montagna dei Fiori anticline represents the uppermost structure of the leading antiformal stack of the outer Central–Northern Apennine thrust belt (Fig. 3d).
The occurrence of an unconformable stratigraphic contact between the Lower–Middle Jurassic Calcare Massiccio – Corniola formations and the Scaglia Rossa Formation, preserved within the overstep of the MdFF system, allows us to confirm a pre-thrusting timing for the MdFF, with a main activity during latest Cretaceous – Palaeogene time (Fig. 5).
The attitude (30–40° dip towards the NE) of the footwall strata and the cut-off angle of c. 70° between the MdFF plane and the footwall bedding constrain the restoration to a pre-folding state of the MdFF with important implications for defining the original attitude of the fault plane and associated shear fabrics (Figs 9, 10). Restoring the MdFF footwall bedding to the horizontal by applying a rotation of 40° of magnitude along a NNW–SSE axis, the present-day WSW-dipping attitude of the MdFF plane turns into an ENE dip-direction attaining a high-angle, reverse-faulting character (Fig. 10a–d), and the apparent present-day extensional shear fabric (Fig. 9a) turns into a contractional fabric with top-to-SW kinematics (Fig. 9c, d).
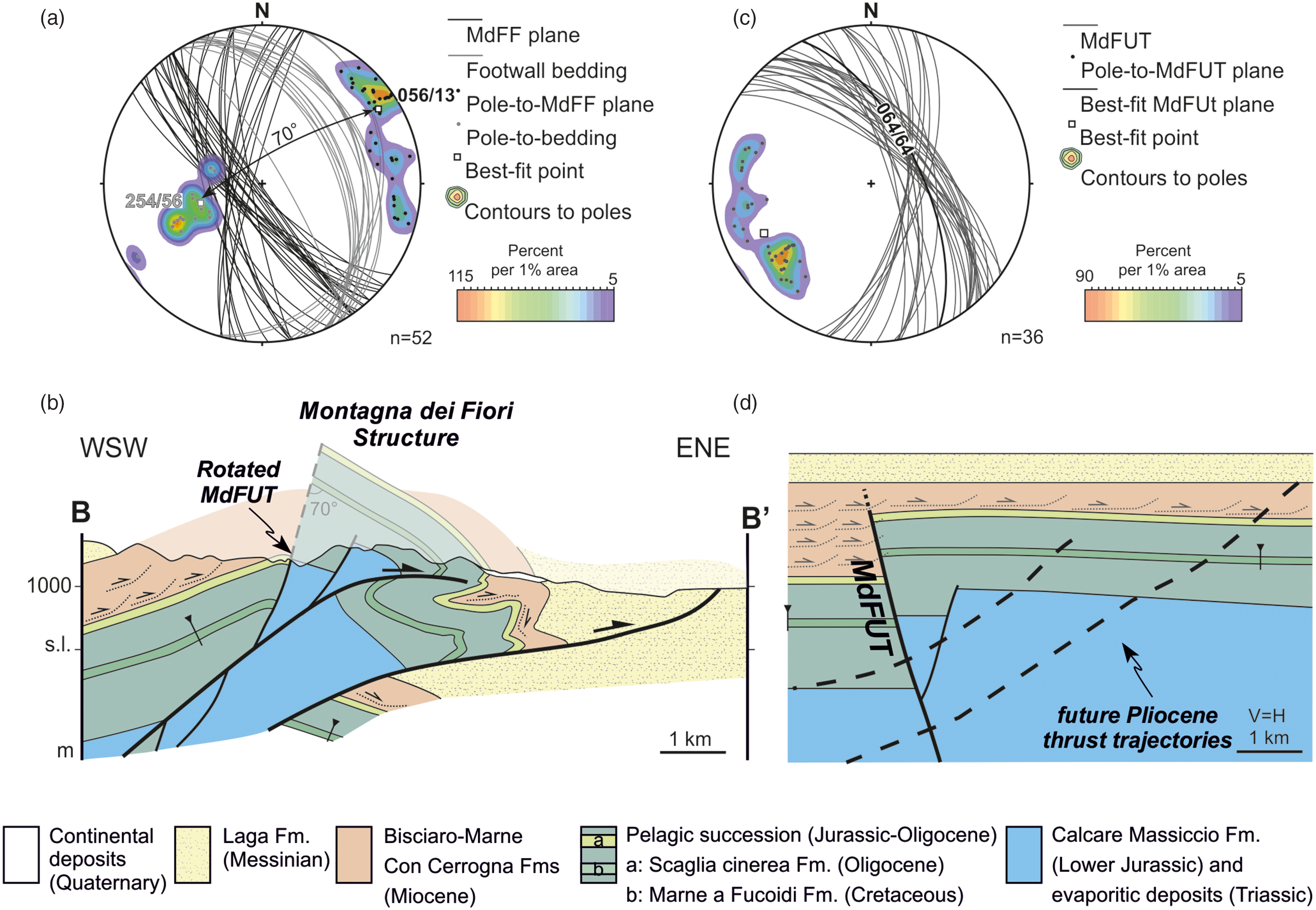
Fig. 10. (a) Equal-area lower-hemisphere stereographic projection showing the attitude of MdFF and the footwall bedding in the carbonate sequence measured 50 m away from the fault damage zone; a cut-off angle value of c. 70° was estimated between the MdFF (056/13) and footwall bedding (254/56) poles best-fit points. (b) Cross-section through the Montagna dei Fiori structure (location in Fig. 3a). (c) Equal-area lower-hemisphere stereographic projection showing the MdFUT by restoring the footwall beddings best-fit to the horizontal. (d) Pre-thrusting restored template with the MdFF considered as a latest Cretaceous – middle Miocene NE-dipping up-thrust; dashed shear planes within the Marne con Cerrogna Formation represent the so-called Laga detachment (Koopman, Reference Koopman1983).
By considering the new time constraint (mainly latest Cretaceous – Palaeogene activity) and the reverse fault geometry in the restored template (Fig. 10d), the MdFF can be interpreted as an up-thrust (Montagna dei Fiori Up-Thrust or MdFUT) with a top-to-the-WSW movement. The newly documented latest Cretaceous – Palaeogene timing is consistent with the onset of Dinaric contractional deformation (e.g. Schmid et al. Reference Schmid, Bernoulli, Fügenschuh, Matenco, Schuster, Schefer, Schuster, Tischler and Ustaszewski2008; Porkoláb et al. Reference Porkoláb, Willingshofer, Sokoutis, Creton, Kostopoulos and Wijbrans2019), allowing us to define this fault as a WSW-directed, up-thrust of Dinaric affinity. This contractional fault system, developed within the Adriatic foreland ahead of the growing Dinaric chain (Fig. 1), would have been composed of two NW–SE regional frontal ramps linked by a NE–SW-trending local lateral ramp, although a different origin for the transverse fault due to stress axes permutations during inversion tectonics (e.g. see Peacock et al. Reference Peacock, Tavarnelli and Anderson2017) cannot be ruled out. The lateral ramp actually accommodates the tectonic contact between the Scaglia Rossa and Scaglia Cinerea formations, cropping out above the unconformity, and the Marne con Cerrogna Formation. In addition, the thickness and facies variations of the Miocene Marne con Cerrogna Formation across the hanging-wall and footwall blocks of the MdFUT document renewed reverse fault activity during middle Miocene time (Fig. 11d). The Miocene syn-tectonic sedimentation in the MdFUT footwall (present-day hanging wall of the MdFF) exceeded 1000 m in thickness in the Marne con Cerrogna Formation with the hemipelagic marly sequence interbedded by abundant thick calcarenite packages. By contrast, the mostly marly and clayey sequence exposed in the hanging wall of the MdFUT (present-day footwall of the MdFF) is more reduced in thickness and reaches a maximum of c. 400 m.

Fig. 11. (a–e) Schematic tectonic evolution of the Montagna dei Fiori structure based on the new geological and structural data presented in this study (not to scale). The MdFF is interpreted to be originated as a primary steep NE-dipping reverse fault (MdFUT) developed in the Adriatic foreland during the Late Cretaceous – middle Miocene time interval, coeval with the Dinaric contractional deformation phase. During the Pliocene Epoch, this sector of the foreland became progressively incorporated into the advancing Apennine thrust belt, causing the reactivation and rotation of the MdFUT within the development of the Montagna dei Fiori Anticline, until assuming an apparent present-day SW-dipping normal attitude.
The NW–SE-trending conjugate mesoscopic normal faults offsetting the Scaglia Rossa, the unconformity surface and the underlying Calcare Massiccio Formation, along the NE–SW-aligned lateral ramp of the MdFUT, can be related to the flexure of the foreland during the Messinian Age (Fig. 7), synchronous with the early onset of the ENE-directed Apennine thrust belt development (e.g. Boccaletti et al. Reference Boccaletti, Calamita, Deiana, Gelati, Massari, Moratti and Ricci Lucchi1990).
Cross-cutting relationships on the well-developed shear fabrics recognized within the lenses of the Scaglia Rossa – Scaglia Cinerea formations along the MdFUT (Fig. 9) allowed us to reconstruct the subsequent evolution of the MdFUT. The reverse S-C fabric, characterized by S-cleavage sub-parallel to the MdFUP surface and mesoscopic shear planes displacing the up-thrust with a top-to-the-ENE kinematic, testifies an early Apennine-affinity contractional overprint of the MdFUT. This structural assemblage can be ascribed to the buttressing effect (terminology after Butler, Reference Butler, Cooper and Williams1989) achieved by the stiff footwall block of the MdFUT during the foreland-directed propagation of the Laga Detachment zone (Messinian – lower Pliocene; Koopman, Reference Koopman1983); a similar reconstruction has also been previously proposed by Calamita et al. (Reference Calamita, Pizzi, Ridolfi, Rusciadelli and Scisciani1998).
By restoring the regional dip of bedding to the horizontal, the S-C fabric, showing present-day normal kinematics, exhibits a reverse character that is consistent with post-Laga Detachment reactivation of the MdFUT during Pliocene Apennine thrusting in the outer zone of the belt (Figs 10, 11f). During this incipient Pliocene development of the Montagna dei Fiori thrust-related anticline, the MdFUT was reactivated as a backthrust defining a pop-up structure with frontal NE-directed thrusting (Fig. 11d). Subsequently, with the progressive evolution of the overturned breakthrough fault-propagation fold, the MdFUT was rotated to acquire an apparent present-day normal attitude (Fig. 11e).
4. Discussion
Regional hinterland-dipping faults in the hinge/forelimb zones of macroscopic anticlines have been widely documented across the Central–Northern Apennine mountain range as well as in the deformed foreland (e.g. Migliorini, Reference Migliorini1948; Ghisetti & Vezzani, Reference Ghisetti and Vezzani1991; Tavarnelli, Reference Tavarnelli1996; Calamita et al. Reference Calamita, Pizzi, Ridolfi, Rusciadelli and Scisciani1998; Scisciani et al. Reference Scisciani, Calamita, Tavarnelli, Rusciadelli, Ori and Paltrinieri2001 a, b, Reference Scisciani, Tavarnelli and Calamita2002; Mazzoli et al. Reference Mazzoli, Deiana, Galdenzi, Cello, Bertotti, Schulmann and Cloetingh2002). The tectonic significance of these faults has largely been debated in the literature with contrasting interpretations considering younger-on-older thrusts within out-of-sequence thrust systems (Ghisetti & Vezzani, Reference Ghisetti and Vezzani1991; Carminati et al. Reference Carminati, Fabbi and Santantonio2014), regional strike-slip faults (Montone & Salvini Reference Montone and Salvini1991; Corrado et al. Reference Corrado, Di Bucci, Naso, Giampaolo and Adatte1998), pre-thrusting normal faults (Tavarnelli, Reference Tavarnelli1996; Calamita et al. Reference Calamita, Pizzi, Ridolfi, Rusciadelli and Scisciani1998, Reference Calamita, Satolli, Scisciani, Esestime and Pace2011, Reference Calamita, Di Domenica and Pace2018; Scisciani et al. Reference Scisciani, Tavarnelli and Calamita2001 b; Mazzoli et al. Reference Mazzoli, Deiana, Galdenzi, Cello, Bertotti, Schulmann and Cloetingh2002; De Paola et al. Reference De Paola, Mirabella, Barchi and Burchielli2006; Pace et al. Reference Pace, Di Domenica and Calamita2014) or normal faults related to syn-thrusting activity (Migliorini, Reference Migliorini1948; Ghisetti & Vezzani, Reference Ghisetti and Vezzani2000; Storti et al. Reference Storti, Balsamo, Mozafari, Koopman, Swennen and Taberner2018) and back-thrusts related to Neogene Apennine thrusting (Patacca et al. Reference Patacca, Scandone, Di Luzio, Cavinato and Parotto2008; Fabbi & Smeraglia Reference Fabbi and Smeraglia2020) or late-thrusting extensional collapse (Tavani et al. Reference Tavani, Storti, Bausà and Muñoz2012).
The NNW–SSE-trending Montagna dei Fiori Anticline is a prominent regional-scale, overturned thrust-related fold within the antiformal stack at the leading edge of the Central Apennine thrust system. This fold represents the outermost E-verging positive structure in the peri-Adriatic foothills facing the deformed foreland basin. A map-scale, SW-dipping fault (MdFF) characterizes the hinge/forelimb zone of this anticline striking almost parallel to the anticlinal axial trend. The steeply dipping (70–80°) fault system produces a younger-on-older juxtaposition of the syn-orogenic Miocene Marne con Cerrogna Formation over the pre-orogenic Jurassic carbonate succession, with a throw of c. 1000 m. Interpretations concerning the tectonic significance and role of this fault within the evolution of this sector of the Apennines are still very controversial. This is due to a strong superimposition of different tectonic events as testified by mutual cross-cutting relationships between extensional and contractional structures recognized in the field at different observational scales and preventing unequivocal determination of the relative timing. Based on stratigraphic thickness variations of the Marne con Cerrogna Formation across hanging-wall and footwall fault blocks, Miocene pre-thrusting normal fault activity has been proposed by Calamita et al. (Reference Calamita, Pizzi, Ridolfi, Rusciadelli and Scisciani1998), Mazzoli et al. (Reference Mazzoli, Deiana, Galdenzi, Cello, Bertotti, Schulmann and Cloetingh2002) and Scisciani et al. (Reference Scisciani, Calamita, Tavarnelli, Rusciadelli, Ori and Paltrinieri2001 a, b, Reference Scisciani, Tavarnelli and Calamita2002). By contrast, Ghisetti & Vezzani (Reference Ghisetti and Vezzani2000) suggested this fault as having a substantial role as a normal fault during Pliocene syn-thrusting activity, which Storti et al. (Reference Storti, Balsamo, Mozafari, Koopman, Swennen and Taberner2018) related to the collapse of the anticline backlimb as a consequence of gravitational re-equilibrium.
The structural–geological analysis presented in this study provides new stratigraphic and structural constraints to unravel the absolute and relative timing of the MdFF. By removing the Apennine contractional deformation from the Montagna dei Fiori Anticline and restoring the footwall strata to a pre-folding horizontal state, the footwall cut-off angle relationship implies an ENE dip direction of the MdFF that reveals an original high-angle reverse-fault kinematics (MdFUT). The unconformable stratigraphic contact between the Lower–Middle Jurassic Calcare Massiccio – Corniola formations and the Scaglia Rossa Formation, preserved within a fault stepover, provides the absolute timing of the MdFUT that was active during Late Cretaceous – Palaeogene time.
The geological and structural setting of the peri-Adriatic foothills is characterized by the leading thrust system of the Apennine chain involving Pliocene–Quaternary foredeep deposits along the coastal area (Fig. 1b). In the Central Adriatic, compressive and transpressive tectonics produced a NW–SE-trending foreland deformation belt, known as the Mid-Adriatic Ridge (Fig. 2). The structural setting of the Mid-Adriatic Ridge is variously interpreted as intraplate inversion-dominated foreland deformation (Argnani & Gamberi, Reference Argnani and Gamberi1995; Scisciani & Calamita, Reference Scisciani and Calamita2009; Pace et al. Reference Pace, Scisciani, Calamita, Butler, Iacopini, Esestime and Hodgson2015 b) or as the thin-skinned thrust front of the NE-verging Apennines (Scrocca, Reference Scrocca2006) or SW-verging Dinarides (Finetti & Del Ben, Reference Finetti, Del Ben and Finetti2005) linked to onshore thrust belts via flat-laying detachments. Independent of the interpretation of the deep structural style of this contractional foreland deformation belt, two main phases of contractional deformation have been widely documented from the interpretation of seismic reflection profiles: a Late Cretaceous – Palaeogene phase, coeval with the onset of the SW-directed Dinaric thrust belt and a later Pliocene–Quaternary phase, synchronous with the late-stage evolution of the outer Apennines (e.g. Argnani & Gamberi, Reference Argnani and Gamberi1995; Finetti & Del Ben, Reference Finetti, Del Ben and Finetti2005; Scisciani & Calamita, Reference Scisciani and Calamita2009). These distinct contractional pulses caused the multiple positive structural inversions of inherited Mesozoic rift basins developing symmetric or asymmetric inversion structures showing Dinaric (SW vergence) or Apennine (NE vergence) polarity, respectively. These inversion structures are frequently associated with up-thrusts (Scisciani, Reference Scisciani2009; Pace et al. Reference Pace, Scisciani and Calamita2011; Mancinelli & Scisciani, Reference Mancinelli and Scisciani2020).
For instance, the Emma inversion structure is composed of an array of up-thrusts, resting mainly blind within the Palaeogene–Miocene succession, whereas the Messinian reflector and the overlying lower section of the Pliocene–Quaternary succession are gently folded. Some of these up-thrusts generated from the polyphase reverse reactivation of pre-existing Mesozoic normal faults, which bounded a Triassic–Jurassic extensional basin (Pace et al. Reference Pace, Scisciani, Calamita, Butler, Iacopini, Esestime and Hodgson2015 b). Unconformities, tilted on-laps and thickness variations with thinning towards the fold crest of the Upper Cretaceous – Oligocene sequence chart a first phase of up-thrusting and related anticline growth that is coeval with the onset of Dinaric foreland contractional deformation. The subsequent Pliocene–Quaternary contraction is mainly accommodated by folding, as extensively documented across the Mid-Adriatic Ridge. Lateral thickness variations of the lower–upper Pliocene sediments and converging on-laps from the fold limbs towards the fold axes of the inverted anticlines clearly constrain the subsequent Apennine foreland contractional phase (Scisciani, Reference Scisciani2009; Pace et al. Reference Pace, Scisciani, Calamita, Butler, Iacopini, Esestime and Hodgson2015 b).
The latest Cretaceous – middle Miocene timing and reverse character of the MdFF, documented for the first time by this study, shows many analogies with the early growth of inversion structures in the Mid-Adriatic Ridge. This allows us to interpret the regional MdFF as a Dinaric-age up-thrust (MdFUT) developed in the Adriatic foreland that was reactivated and rotated during Pliocene Apennine thrusting to acquire an apparent present-day normal attitude in the Apennine foothills. A possible origin for the MdFUT as the result of reverse reactivation of a pre-existing, Permian–Triassic ENE-dipping normal fault cannot be ruled out, by analogy with that of other Dinaric structures identified across the Mid-Adriatic ridge.
5. Conclusions
A geological and structural study was performed in the Montagna dei Fiori Anticline, located within the foothills of the Central–Northern Apennine, Pliocene–Quaternary foreland, fold-and-thrust belt. The tectonic significance of the regional NW–SE-trending MdFF was examined by integrating original stratigraphic and structural data.
The newly acquired dataset enabled us to define the absolute and relative timing of the MdFF and to redefine the pre-thrusting structural setting of the Montagna dei Fiori structure. Based on the new timing and the mesoscopic structural and kinematic constraints, the MdFF is interpreted to have been originally generated as a NE-dipping up-thrust (MdFUT) developed in the Adriatic foreland during Late Cretaceous – middle Miocene time, coeval with the Dinaric contractional deformation phase. During the Pliocene Epoch, this sector of the foreland plate became progressively incorporated into the advancing Apennine thrust belt. This caused the reactivation and rotation of the MdFUT within the developing Montagna dei Fiori Anticline to acquire an apparent present-day SW-dipping normal attitude.
Five main evolutionary deformation steps were reconstructed for the MdFF and related Montagna dei Fiori anticline (Fig. 11):
-
(1) Development of a NE-dipping up-thrust (MdFUT) and related broad fault-bend anticline (Dinaric-age Montagna dei Fiori Anticline) during latest Cretaceous – Palaeogene time, which also recorded middle Miocene reactivation and growth (syn-Marne con Cerrogna Formation) in the Adriatic remote foreland of the SW-directed Dinaric thrust (Fig. 11a).
-
(2) Deposition of the Messinian siliciclastic Laga Formation in the Apennine foredeep (post-dating the Dinaric activity of the MdFUT) related to the foreland flexure and associated normal faulting, as documented at the mesoscopic scale with well developed conjugate systems (Fig. 11b).
-
(3) Development of the upper Messinian Laga detachment related to NE-directed Apennine thrusting, deforming the MdFUT that acted as a buttress (Fig. 11c).
-
(4) Lower Pliocene thrusting with reactivation of the MdFUT as a backthrust within the incipient Apennine-age, Montagna dei Fiori, pop-up anticline (Fig. 11d).
-
(5) Pliocene–Quaternary thrusting in the Apennine foothills with final development of the NE-verging Montagna dei Fiori anticline causing the foreland-wards rotation of the MFdUT to its apparent present-day, SW-dipping normal attitude (Fig. 11e).
This tectonic evolution from the Late Cretaceous – middle Miocene Dinaric event to the Pliocene–Quaternary Apennine stage is further constrained by the overprinting relations among the reverse S-C fabrics within the Scaglia Rossa – Scaglia Cinerea tectonic lenses preserved along the MdFUT (Fig. 11f).
Acknowledgments
The constructive and helpful reviews by S. Seno, E. Tavarnelli and an anonymous reviewer, and the careful editorial handling by O. Lacombe, led to an improved version of the manuscript and are kindly acknowledged. The authors are grateful to Pietropaolo Pierantoni and Petros Didaskalou for their assistance in sample preparation and biostratigraphic analysis. TGS is acknowledged for providing the seismic data and for their permission to publish the seismic profile in Figure 2. Petrel™ seismic interpretation software was kindly provided by Schlumberger to the University of Chieti-Pescara. Stereographic projections of structural data were performed using Stereonet v. 11 software platform developed by Richard W. Allmendinger © 2020 (http://www.geo.cornell.edu/geology/faculty/RWA/programs/stereonet.html). This research was financially supported by the “G. d’Annunzio” University of Chieti-Pescara funds awarded to F. Calamita and V. Scisciani.
Declaration of interest
None.