Introduction
Clays are abundant and inexpensive raw materials and have been used since antiquity to build various types of structures and to produce many kinds of ceramic objects (Reference VeldeVelde, 1992). Over time, clays have become an essential component in other economic sectors where they play a fundamental role, such as in the elaboration of polymeric materials and the manufacture of cosmetics and pharmaceutical products (Reference Aguzzi, Cerezo, Viseras and CaramellaAguzzi et al., 2007; Reference da Silva Favero, dos Santos, Weiss-Angeli, Gomes, Veras, Dani, Mexias and Bergmannda Silva Favero et al., 2019; Reference das Graças Silva-Valenzuela, Chambi-Peralta, Sayeg, de Souza Carvalho, Wang and Valenzuela-Díazdas Graças Silva-Valenzuela et al., 2018; Reference Haddaji, Majdoubi, Mansouri, Alomayri, Allaoui, Manoun, Oumam and HannacheHaddaji et al., 2022; Reference Majdoubi, Haddaji, Mansouri, Alaoui, Tamraoui, Semlal, Oumam, Manoun and HannacheMajdoubi et al., 2021; Reference Moussout, Ahlafi, Aazza, Chfaira and MounirMoussout et al., 2020; Reference Nekhlaoui, Essabir, Bensalah, Fassi-Fehri, Qaiss and BouhfidNekhlaoui et al., 2014; Reference Viseras and Lopez-GalindoViseras & Lopez-Galindo, 1999; Reference Williams and HaydelWilliams & Haydel, 2010). This multipurpose characteristic of clays is mainly due to their physico-chemical and mineralogical attributes, which grant them the ability to adapt to various modes of use, especially in the field of ceramics (Reference CiulloCiullo, 1996).
In Morocco, the artisanal pottery culture is one of the most famous traditional forms of ceramics that has been passed on from one generation to the next and has achieved worldwide fame (Reference FedericoFederico, 2020; Reference GravesGraves, 2007; Reference JumpJump, 2003). In general, distinction is made between the glazed and colorful urban pottery and ceramics, geographically bound to historic cities of the country (Tetouan, Fez, Salé, Marrakech, Meknes, and Safi) and the rural pottery, which is marked by the use of fewer colors and simpler decoration styles and designs. The latter type is geographically located in some villages of southern Morocco (e.g. Amezmiz and Agafay) and others in the north (Fran Ali). The latter are distinguished by the exclusive use of primitive coiling techniques, the use of traditional bread ovens to bake the pottery, and the sex of the potters (mostly women). In total, > 100 female artisans are involved in all stages of pottery production in Fran Ali, from the extraction of the clay material to the firing process (crushing, mixing, shaping, and drying). Men help extract raw materials and sell the final products, whereas women are the actual potters, as is the case also in Turkey (Reference Çolak, Maggetti and GalettiÇolak et al., 2006).
The final product consists of utilitarian clay kitchen objects, such as hollow plates also used for cooking, ‘Tajine’; ‘Guedra’ pots; and ‘Méjmar’ stoves, jars, and gourds. The production rate is ~ 100 to 200 pieces/week for each artisan.
Despite this worldwide fame and the large diversity of pottery styles in the area, the potters themselves are not generally concerned by the physico-chemical and mineralogical characteristics of the materials they use. This limits their capacity to evolve and upscale their business, given the limited amount of raw material available in the region. In addition, the potters are resistant to any changes in the extraction and preparation steps and refuse the introduction of new technology into their pottery-production process. The Fran Ali site is a window to the past that allows us to document very old pottery-production methods in the region and constitutes an essential element of the cultural identity of the Jebala ethnic groups (Reference Bazzana and MontmessinBazzana & Montmessin, 1995).
In fact, Reference Barrios Neira, Martín de la Cruz and Montealegre ContrerasBarrios Neira et al. (2012) carried out a preliminary characterization study of the alterites that serve as raw materials for pottery in the Fran Ali area, as well as their underlying phyllite and greywacke substratum. That study focused on the petrographic analysis of thin sections examined by means of optical microscopy, mineralogy by powder X-ray diffraction (XRD), and semi-quantitative chemical analysis by energy dispersive X-ray fluorescence spectrometry (EDS), all conducted with both the raw material and the pottery paste. Air-dried and fired pottery pieces were also examined by thermal analysis (TGA, DTA). That study concluded that the pottery paste that comes from the alterites is not of good quality because of its composition and texture. It consists of quartz-illite and chlorite-type clays packed with phyllite clasts. Measured in thin sections, the diameter of these clasts ranges from 0.5 to 2.5 mm. Such small debris is the result of crushing and sieving by the potters.
This raw material itself is not sufficient to fuel the pottery activities in the area because of the considerable growth of pottery production in the last two decades, which threatens the sustainability of this traditional industry. Thus, an alternative material with similar mineralogical and geotechnical characteristics is needed to support this tradition, especially given its important socio-cultural value to the Fran Ali area in particular and to the Jebala region as a whole.
Therefore, although the present study resumed the characterization of this raw material with more methods and a larger number of samples, the main objective was the preservation of this rural activity, which may be attained through finding abundant and easily accessible alternative materials which could replace those currently in use. The hope was that, by comparing the material used at present (yellowish to reddish-yellow weathered material) with the potential alternative (phyllite), the mineralogy, geochemistry, and geotechnical characteristics would be similar enough to provide a new source of material.
Geological Setting
Geologically speaking, the Fran Ali area is located in the internal domain of the Rif cordillera. It is part of the Ghomarides morphotectonic units, which consist mainly of epimetamorphic Paleozoic rocks that underwent low-grade metamorphism during the alpine orogenic cycle (Reference Chalouan and MichardChalouan & Michard, 1985; Reference Durand Delga and KornprobstDurand Delga & Kornprobst, 1963; Reference Marrone, Monie, Rossetti, Aldega, Bouybaouene, Charpentier, Lucci, Phillips, Theye and ZaghloulMarrone et al., 2021; Reference Negro, Beyssac, Goffé, Saddiqi and BouybaoueneNegro et al., 2006; Reference Rodríguez-Ruiz, Abad and BentabolRodríguez-Ruiz et al., 2019). Conventionally, the Ghomarides are subdivided into four tectonic units, which are the Akaili, Koudiat-Tizian, Béni Hozmar, and Talembote units (Reference Chalouan and MichardChalouan & Michard, 1990; Reference Durand-Delga, Hottinger, Marçais, Mattauer, Milliard and SuterDurand-Delga et al., 1961).
In the study area, the exposed rocks belong to the Akaili unit, which dominates the Beni Said coastal watersheds of the region (Fig. 1). The stratigraphic sequence of the site reveals low-grade metamorphic shales of Silurian age, which alternate with some greywacke strata that become more abundant toward the top of the stratigraphic log (Reference Chalouan and MichardChalouan & Michard, 1990). Consequently, the intensity of weathering processes tends to vary from one site to another depending on the amount of greywacke strata cropping out in each area. The presence of deep-seated, slow moving landslides tends to mobilize the rocks and promotes the formation of thick saprolite and colluvium layers (Fig. 1), especially in shale-dominated sectors. For pottery purposes, the Silurian shale and its weathering products are what constitute the main focus of the present study.
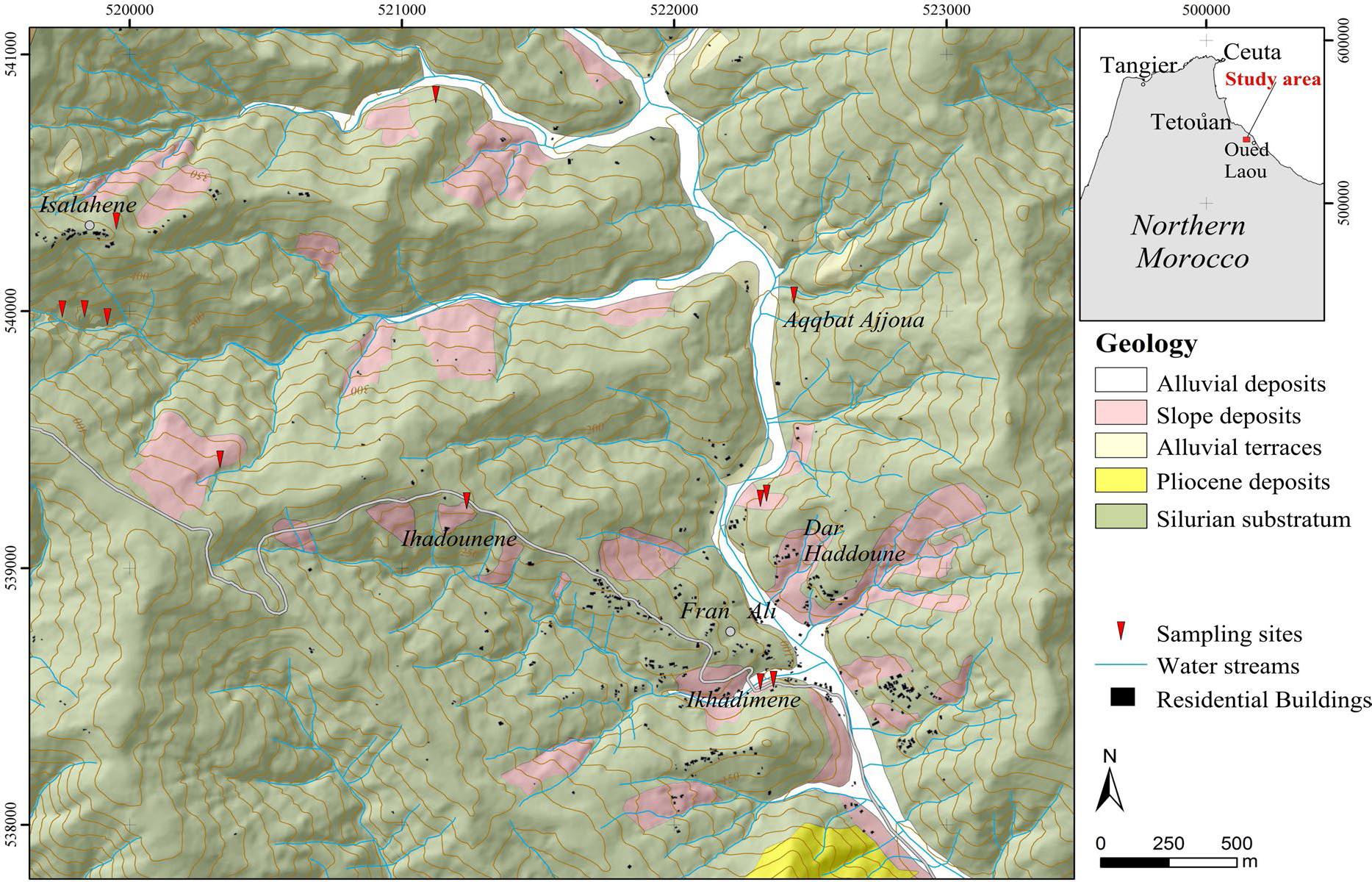
Fig. 1 Geological map of the study area (Fran Ali) showing the locations of the sampling stations
With respect to pottery production, the potters extract two raw materials, one marked by a yellowish color, which is used for the production of the clay paste that forms the skeleton of the sculpted objects, and another used to provide the brick-red color, characteristic of the final product, which is considered a sign of good firing practices. Despite the multitude of extraction sites, the clay resources are being depleted due both to over extraction and the small volume of potentially exploitable materials. As a consequence, the entire tradition may be compromised or may disappear completely from the region if alternative extraction sites are not found in the near future.
Cultural Value of Pottery in Fran Ali
Pottery Production Process in the Fran Ali Area
Each production cycle lasts 6 days, starting with the extraction of raw materials and ending with the marketing of the pottery objects.
In the first day of the production cycle, potters in the Fran Ali area extract the colluvium, the thickness of which varies from 3 to 5 m at each site. In total, seven material-extraction sites (unregulated quarries) were identified by the current authors (Fig. 1), with one being located near Fran Ali village itself and the others found near the Isalahene, Dar El Haddoune, Ihadounene, and Sidi Rahmoun villages. Across all extraction sites, the tool used by potters is the pickaxe, which allows them to dig deep into the ground; this requires persistence, as well as significant effort and quite a lot of time. The process is carried out with no safety protocols in place, despite the considerable dimensions of some quarry tunnels dug by the quarrymen (Fig. 2). Thus, the potters run a daily risk of tunnel collapse, with a risk of death After extraction of the material, it is transported immediately, using donkeys, along the narrow trails of the Fran Ali valleys.

Fig. 2 Typical pottery production steps in the Fran Ali area
On the next (second) day, the raw material is crushed using a wooden stick and then sieved on the spot. Depending on the quantity of clay, the paste-preparation process begins on the same day or is postponed until the following day. The pottery paste is obtained by kneading the raw clays for 40–60 min. To preserve the paste in its wet (and deformable) state, it is enveloped in a plastic film that minimizes any interaction between the paste and the atmosphere.
Then, during the fourth and fifth days, the ceramic objects are shaped from the clay paste using the throwing wheel technique, carried out using traditional, minimalistic tools. Then the objects are air-dried under sunlight for 4–6 h. After the objects are dried, the coloring coat is added using a small quartz pebble that also helps to smoothen the surface of the objects. After a second drying session, the ceramic objects are placed in the kiln at the end of the fifth day and are fired throughout the night. The temperature may reach 600°C or more given the high combustibility of timber from the barbary Thuja trees (Reference PiconPicon, 1995). The sixth day (Saturday) is the market day for the Beni Said tribe where the men of the village attempt to sell the finished products.
Fran Ali Pottery Compared to Old and Historical Pottery Objects
Pottery in northern Morocco dates back to the sixth millennium BC, and is thought to be related to old pottery forms and cultures of the Iberian Peninsula (Reference Martínez Sánchez, Rodríguez, Caro, Pardo-Gordó, Pérez-Jordà and Peña-ChocarroMartínez Sánchez et al., 2021). The oldest forms of pottery discovered in northern Morocco belong to the cultura impressa and cardium ware styles of the Neolithic epoch (Reference Martínez-Sánchez, Vera-Rodríguez, Pérez-Jordà, Pena-Chocarro and BokbotMartínez-Sánchez et al., 2018). Later during the bronze and iron ages, pottery in the region was mainly influenced by Greek, Phoenicean, Carthaginian, and Roman ceramics as indicated by the pottery objects found in the Lixus, Volubillis, Tamuda, and Thamusida archeological sites (Reference Bernal-Casasola, Bustamante-Álvarez, Díaz, López-Sáez, Gutiérrez-Rodríguez, Vargas Girón, Portillo-Sotelo, Pascual Sánchez and MoujoudBernal-Casasola et al., 2021; Reference Gliozzo, D’Aco, Memmi Turbanti, Galli, Martini and SibiliaGliozzo et al., 2009; Reference Maass-LindemannMaass-Lindemann, 1992). In the twelfth and thirteenth centuries, Islamic pottery was introduced to the region with most techniques and decoration styles being the result of the combination of Hispano-Moorish and Amazigh artisanal styles (Reference GravesGraves, 2007). Geographically, this style of pottery and its workshops were limited to big Islamic cities such as Salé, Fes, Safi, Meknes, Marrakech, Al-Basra, Targha, and Tetouan (Reference Bazzana and MontmessinBazzana & Montmessin, 1995; Reference El Ouahabi, Daoudi, Hatert and FagelEl Ouahabi et al., 2015, Reference El Ouahabi, El Idrissi, Daoudi, El Halim and Fagel2019).
In Fran Ali (Oued Laou), the pottery styles (Fig. 3) and techniques (Fig. 2) are primitive and have not yet been dated with confidence. Nevertheless, Reference Bazzana and MontmessinBazzana & Montmessin (1995) suggested that artisanal pottery workshops in the Oued Laou hinterland could date back to the 13th–14th century, based on ethno-archeological investigations, and the fact that their evolution ceased in the 16th century due to a significant demographic and economic decline in the region, exacerbated by Portuguese expansionist wars.
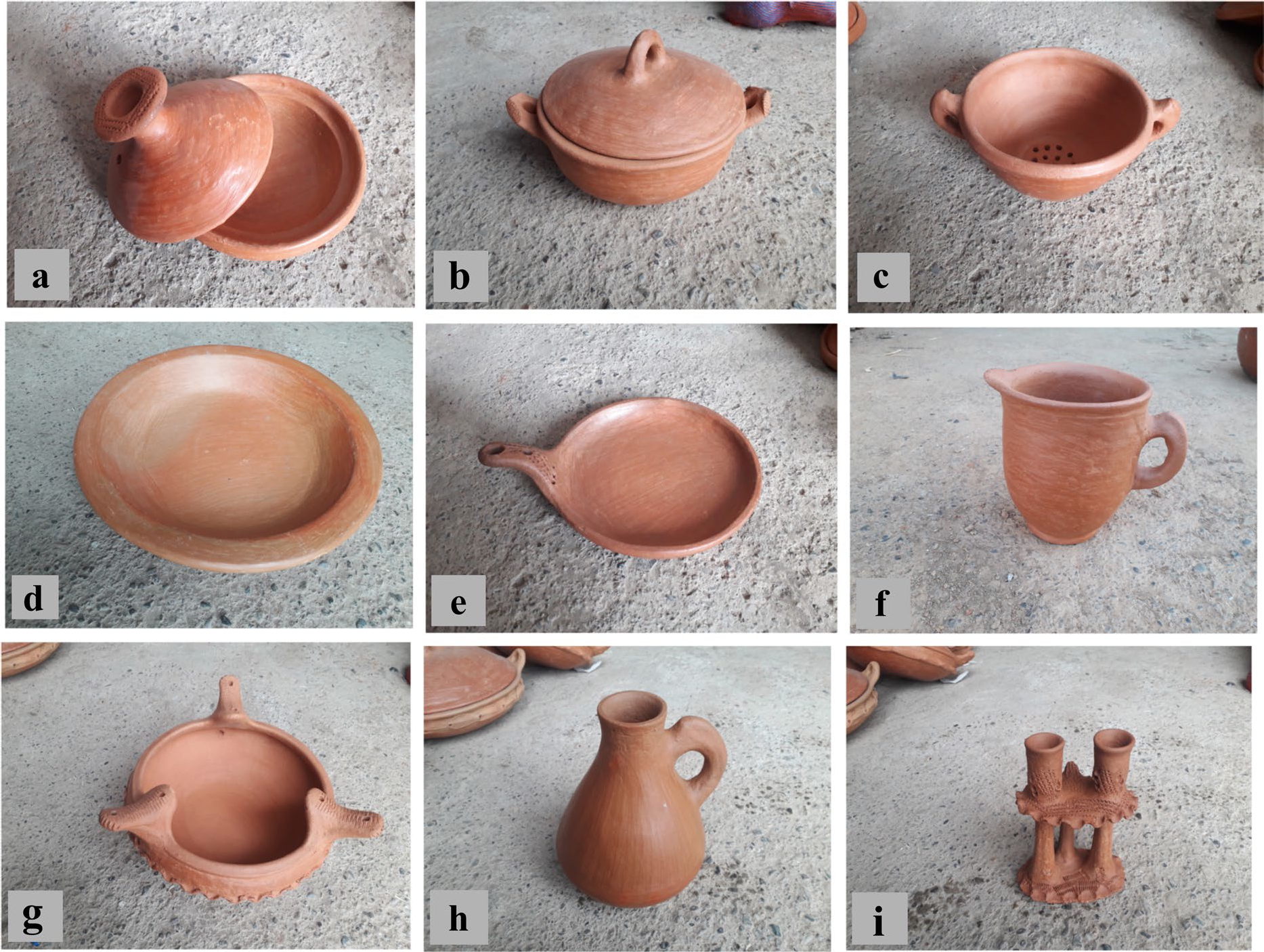
Fig. 3 Pottery objects produced in Fran Ali. a Tajine (plate), b Guedra (cooking pot), c Keskes (steamer), d Kesriya (bread kneading bowl), e Mokla (Bread baker), f Tasse (cup), g Méjmar (charcoal stove), h Aguenbour (jug), and i Haska (Candle holder)
Reference Bazzana and MontmessinBazzana and Montmessin (1995) provided a typological classification of the basic pottery forms produced during medieval times in the Targha archeological site (near Fran Ali). By comparing their basic forms to the morphological inventory in the current study (Fig. 3), the conclusion reached is that the present-day pottery forms are different from those of the past. For example, the cooking pots produced in Fran Ali (Fig. 3b) are different from those produced during the 14th and 15th centuries in terms of their overall morphology. Only objects from Kasriya and Aguenbour (Fig. 3d–h) appear to resemble the medieval equivalents presented by the above-mentioned authors. The other forms are drastically different, which may be due to a loss of knowledge as a consequence of the decline of pottery since the 16th century, or the adaptation of this traditional artisanal activity to the needs of the current markets.
With regards to decoration styles, the techniques used are very primitive and resemble those deployed by primitive cultures of the western Mediterranean. The potters in Fran Ali still use sharp objects to carve drawings and decorative forms into the paste before firing them, which resembles the techniques used in cultura impressa ceramics of the Neolithic epoch (Reference Martínez Sánchez, Rodríguez, Caro, Pardo-Gordó, Pérez-Jordà and Peña-ChocarroMartínez Sánchez et al., 2021). Also worth noting is that these pottery objects are not glazed or colored which makes them minimalistic and practical (Reference El Ouahabi, El Idrissi, Daoudi, El Halim and FagelEl Ouahabi et al., 2019).
Materials and Methods
Sampling Strategy
To achieve a chemical, mineralogical, and geotechnical characterization of all Fran Ali extraction sites, six colluvium and saprolite samples were collected from different extraction sites. Their typical pedological profile (Fig. 4) is formed by the parent rock in the form of grayish shales that are not affected by weathering processes (S1, S2, S3, S4, S5, and S6), followed by a layer of yellow to red soil (FA6) and another thin layer of yellowish clayey soil (FA1 and FA2), a thick horizon of reddish colluvium soil (FA3, FA4 and FA5), and finally a 1–2 m-thick topsoil layer.

Fig. 4 Stratigraphic log of the weathering products exploited by potters in Fran Ali
Given that the bedrock in the vicinity of Fran Ali village consists of both shale and greywacke layers, a potential alternative site has to present thicker layers of shale with thin greywacke intercalations. Such stratigraphy can only be found further from the village. Therefore, six samples were collected (from the sites shown in Fig. 1), which correspond to the above description.
The weathered layers found in the Fran Ali area, which resulted from the chemical weathering and gravitational processes acting on Silurian phyllite and greywacke layers, are characterized by a reddish to yellowish color with a clay-like texture, with small to medium-sized phyllite and greywacke pebbles. Such soils, especially the red layers, can be classified as typical Mediterranean colluvial deposits that require significant drainage and seasonal water variations to form over thousands of years (Reference RuellanRuellan, 1969). Their red color is the result of a slow soil rubification process that releases a significant amount of iron oxides in the soil, i.e. the result of slow weathering processes indicative of ancient climates (Reference El GharbaouiEl Gharbaoui, 1980; Reference RognonRognon, 1987). However, the mineralogy of the above soil layers continued to evolve. Thus, the weathered clay layers found in the study area are not considered to be fossilized or reworked as they continue to change and evolve under current climate conditions (Reference Beaudet, Maurer and RuellanBeaudet et al., 1967).
Mineralogical Characterization Techniques
The mineralogical composition of the clays derived from the above-described sampling sites was characterized by X-ray diffraction using a Bruker D8-Advance diffractometer (Bruker AXS GmbH, Karlsruhe, Germany delivered by Radaco, Casablanca, Morocco) equipped with a CuKα tube (λ = 1.5405 Å) operating at 25 mA and 40 kV and a Bragg–Brentano goniometer in theta-theta geometry with a focusing diameter of 500 mm and a LynxEye-2 fast linear detector (D1 mode). The recording of the patterns and data analysis was carried out using the Diffrac.Suite.Vs.1 software (Bruker).
The whole-rock mineralogy was obtained using the X-ray powder diffraction technique. The samples were crushed manually using an agate mortar and sieved to 250 µm. The preparation of the clay fraction (<2 μm fraction) for the XRD analysis consisted of decalcifying the samples with dilute HCl, washing with demineralized water, and finally separating silt from clay by decantation in a water column for 48 min according to Stokes' Law. Then, oriented aggregate mounts for clay mineral identification were prepared from the residual clayey fraction (Reference Moore and ReynoldsMoore & Reynolds, 1989).
Three analyses were performed on each oriented aggregate mount. First, XRD was performed on clayey <2 μm fractions in their natural state (N). Then, the same analysis was performed on materials saturated for 24 h in a glycol atmosphere (EG). Finally, each oriented aggregate was heated to 500°C for 4 h (C) and analyzed once more using XRD.
Analysis of the diffraction patterns derived from the three tests (N, EG, and C) identified the clay minerals present in the samples. As for the quantitative mineralogical composition analyses, the method of Reference Cook, Johnson, Matti and ZemmelsCook et al. (1975) was used for powder samples and that of Reference BiscayeBiscaye (1965) for the clayey fraction (< 2 μm) in this work.
Chemical Characterization Techniques
The chemical composition of the investigated material was determined by X-ray fluorescence spectrometry using a Epsilon 1 spectrometer (Malvern Panalytical, Palaiseau, France delivered by Technilab-Htds, Casablanca, Morocco). Thermogravimetric analysis (TGA/DTGA) of the samples was also carried out, using a Lab Sys evo equipment (SETARAM Instrumentation, Caluire, France delivered by Radaco, Casablanca, Morocco) consisting of a high-temperature oven capable of reaching 1600°C with a constant nitrogen flow rate and a temperature program of 20°C/min.
The carbonate content was estimated by the Bernard Calcimetry method (French standard NF P 94–048). The amount of CaCO3 was linked to the presence of calcite peaks in the XRD patterns. All chemicals were reagent grade from Sigma-Aldrich and obtained from SMAPROL, Casablanca, Morocco (Sigma-Aldrich Chimie S.a.r.l., Lyon, France).
Geotechnical Characterization
The characterization of clay plasticity in the Fran Ali sector was performed through the Atterberg limit test, which provides accurate estimations of the liquidity (WL) and the plasticity limits (WP) for a given material. The samples were prepared as fine-grained soils, with a particle diameter of 400 µm or less, in accordance with the French standard NF P 94–051. To calculate WL values, the Casagrande apparatus was deployed, while WP was determined by rolling a small ball of clay paste into a boudin shape (Reference CasagrandeCasagrande, 1947). The plasticity index is defined by the interval between the two Atterberg limits (PI = WL–WP).
To characterize particle-size distributions in the clayey material found in the study area, granulometric analyses were carried out through a semi-wet sieving procedure on samples with particle diameters of > 80 µm, in accordance with the French standard NF P 94–057. The proportion of the remaining fine-grained material (i.e. particles < 80 µm) was measured using the Fritsch Analysette 22 NanoTec laser diffraction particle size analyzer.
The specific surface area of the clay fraction was determined using the methylene blue test (MBT) (NF P 94–068). This test consists of mixing the clayey material with water at a constant speed of 400 rpm, and then measuring the quantity of methylene blue adsorbed by the end of the test. The procedure followed in this study was consistent with the French standard NF P 94–068.
Results
Particle-Size Distributions and MBT Results
The granulometric analysis by semi-wet sieving of Fran Ali clayey material showed a variance in particle-size distributions across samples. All of the investigated geological materials consist of fine-grained particles (<80 μm), with a sandy-silty fraction (>20 μm) ranging from 37 to 52%, a clay fraction (<2 μm) averaging 21 to 35%, and a silt fraction (2–20 μm) ranging from 28 to 34%. All samples including those of the parent-rock fall within the boundaries of the 'sand-silt–clay' field of the Reference ShepardShepard (1954) classification (Fig. 5).
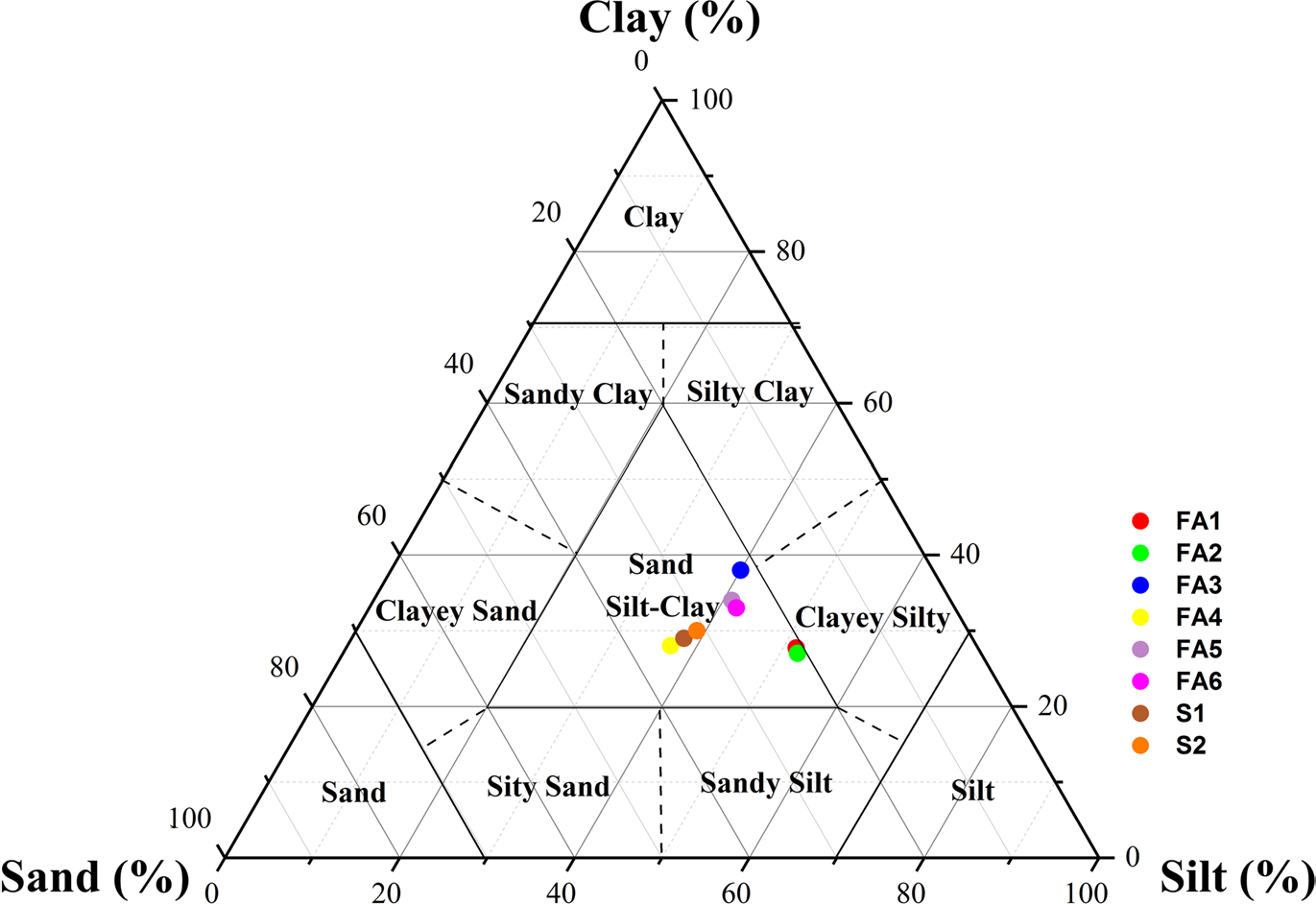
Fig. 5 Projection of Fran Ali soil, subsoil, and parent-rock samples on the Reference ShepardShepard (1954) ternary diagram
Laser granulometric analysis performed on the <80 μm fraction of the Fran Ali samples confirmed the above results (Fig. 6). The dominance of silt and clay materials was clear in all samples, with a smaller sand fraction present in various proportions in FA4 through FA6 as well as bedrock samples (Fig. 6). In fact, particles with a diameter ranging from 0.2 to 2 mm were more abundant in the parent-rock material and completely absent from the surficial reddish colluvium layer. The fact that coarser particles were present in the bedrock and absent from the topsoil layers suggests a physical degradation process that eliminates coarse elements (Fig. 6).
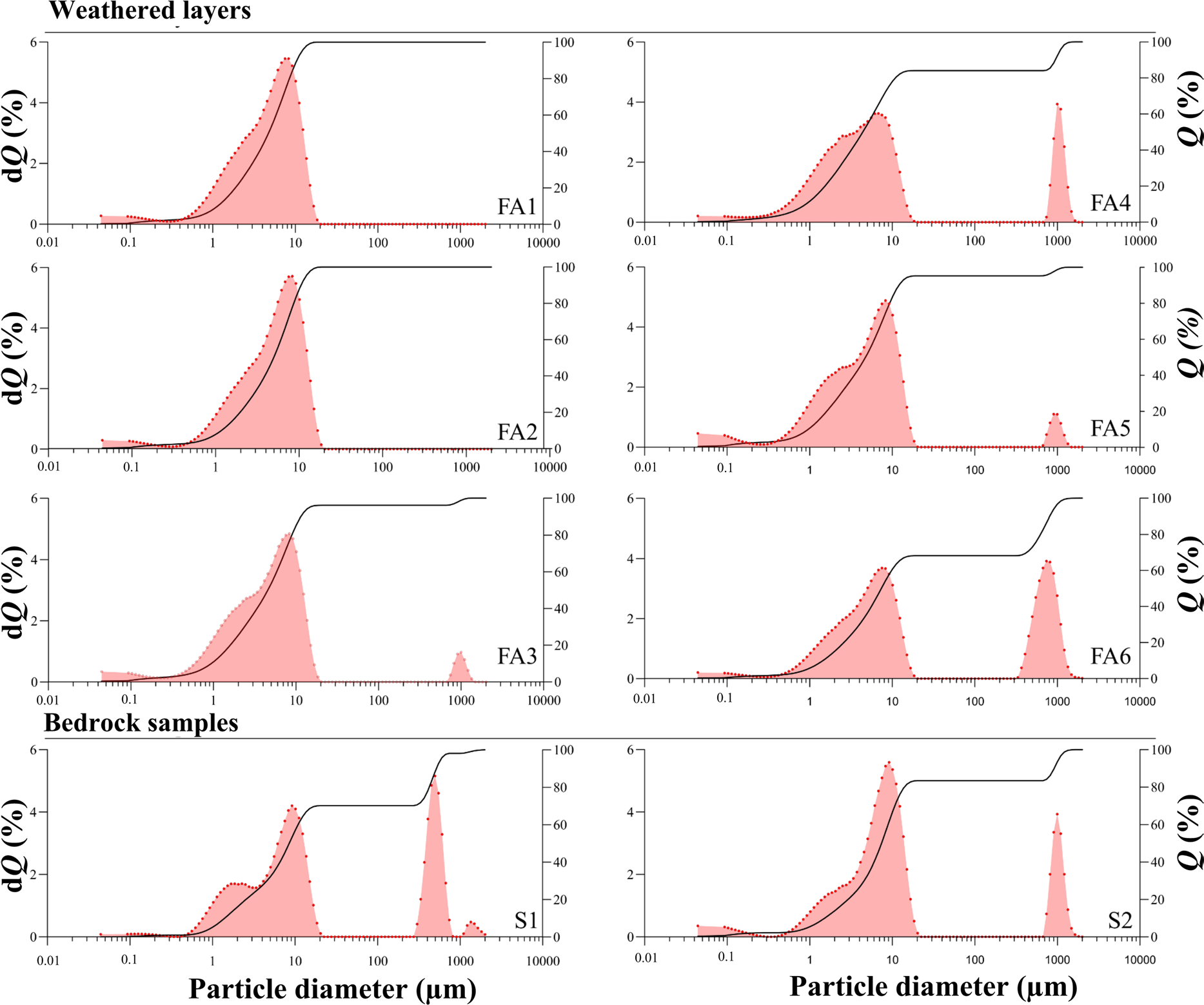
Fig. 6 Laser granulometry analysis results for all Fran Ali samples
The samples adsorbed 3–6.6% of the methylene blue used in the Methylene Blue Test (MTB) (Table 1). Thus, they can be classified as clayey to silty-clayey soils. The carbonate (CaCO3) content was generally small. For the shale samples (S1–S6), CaCO3 content failed to exceed 2.7%, while the chemical composition of samples FA1 through FA6 was characterized by an even smaller calcium carbonate content (2.2–4.8%).
Table 1 MBT and calcimetry results

Plasticity Index Estimations
In pottery, the behavior of the clay in the presence of water is an important variable that potters try to control. Therefore, the consistency limits are essential. The values of the WP, WL, and PI (Table 2) revealed that samples FA1, FA2, and FA4 were characterized by PI values of 21%, and for samples FA3, FA5, and FA6 the value ranged between 18 and 30%. Such values indicate high plasticity based on Reference MurthyMurthy (2002). The parent-rock samples (S1–S6) were characterized by medium plasticity, depending on the classification system (7–9%). This test indicated that the behavior of all samples fell within the range of clayey materials, as all of them were above the A line. The plastic behavior of a material depends on several variables, including the particle-size distribution (Reference Holtz, Kovacs and SheahanHoltz et al., 1981), the mineralogical nature of the clays investigated (Reference Dumbleton and WestDumbleton & West, 1966; Reference Hajjaji, Hachani, Moussi, Jeridi, Medhioub, López-Galindo, Rocha, Labrincha and JamoussiHajjaji et al., 2010), the degree of diagenesis experienced by the said material (Reference DaoudiDaoudi, 1991), the presence of amorphous materials (Reference Wan, Kwong, Brandes and JonesWan et al., 2002), and/or the presence of organic matter (Reference Holtz, Kovacs and SheahanHoltz et al., 1981). Consequently, the relatively abundant sandy fraction in FA4, FA6, and all bedrock samples explains their low plasticity index values.
Table 2 Plasticity index estimations
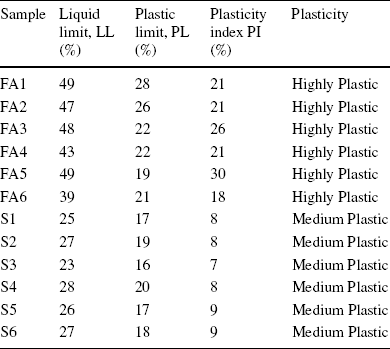
Chemical Characterization
The whole-rock major element composition for each of the 12 samples studied (Table 3) revealed that the most abundant oxides are SiO2, Al2O3, and Fe2O3. Other oxides (MgO, CaO, Na2O, K2O), are present in smaller quantities. The predominant oxides (SiO2 and Al2O3 especially) are mainly associated with kaolinite, chlorite, illite, and plagioclase feldspar minerals. The high SiO2 content (57%) is also indicative of abundant quartz crystals. Fe2O3 (8–10%) is thought to be a product of chemical weathering, which explains the rubified to reddish color of the subsoil strata across the study area. The remaining oxides present a minor proportion of the overall minerals despite the percentage of K2O (2–4%), which indicates the presence of feldspars in the soils. In the XRD patterns, a 3.18 Å peak represents a plagioclase mineral, being relatively high compared to the rest (MgO, CaO, and Na2O). Such minor oxides are expected in clay-dominated material as they tend to occupy the interlayers of the clay crystals. The loss on ignition (LOI) ranges between 5 and 10%.
Table 3 Chemical compositions (%)
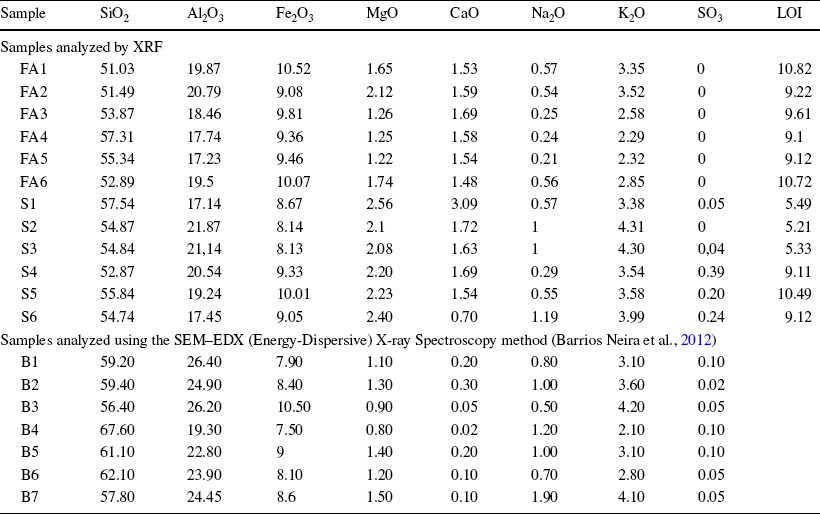
The alterite samples used as a raw material from Fran Ali, analyzed by Reference Barrios Neira, Martín de la Cruz and Montealegre ContrerasBarrios Neira et al. (2012), show the same trend in proportions, although the SiO2 and Al2O3 values are much larger than those analyzed in the present study. This may well be explained by the difference in the techniques used; a surface elemental analysis technique (SEM–EDX technique) used by Reference Barrios Neira, Martín de la Cruz and Montealegre ContrerasBarrios Neira et al. (2012) versus total matrix quantification (XRF) adopted in the present work. The difference may also be attributed to sampling.
Mineralogical Composition
Results from the whole-rock XRD analyses highlight the mineralogical components of the colluvium and the phyllites (Fig. 7). The colluvium soils (Fig. 7a) are dominated by illite (Ilt) and quartz (Qz), with minor proportions of chlorite (Chl), kaolinite (Kln), goethite (Gth), hematite (Hem), orthoclase (Or), plagioclase (Pl), diopside (Di), and muscovite (Ms). The bedrock samples reveal major Chl, Ilt, and Qz peaks (Fig. 7b). Pyrophyllite (Prl), apophyllite (Apo), Pl, Gth, Hem, Or, Di, Ms, and dolomite (Dol) appeared in small amounts (Fig. 7a,b).
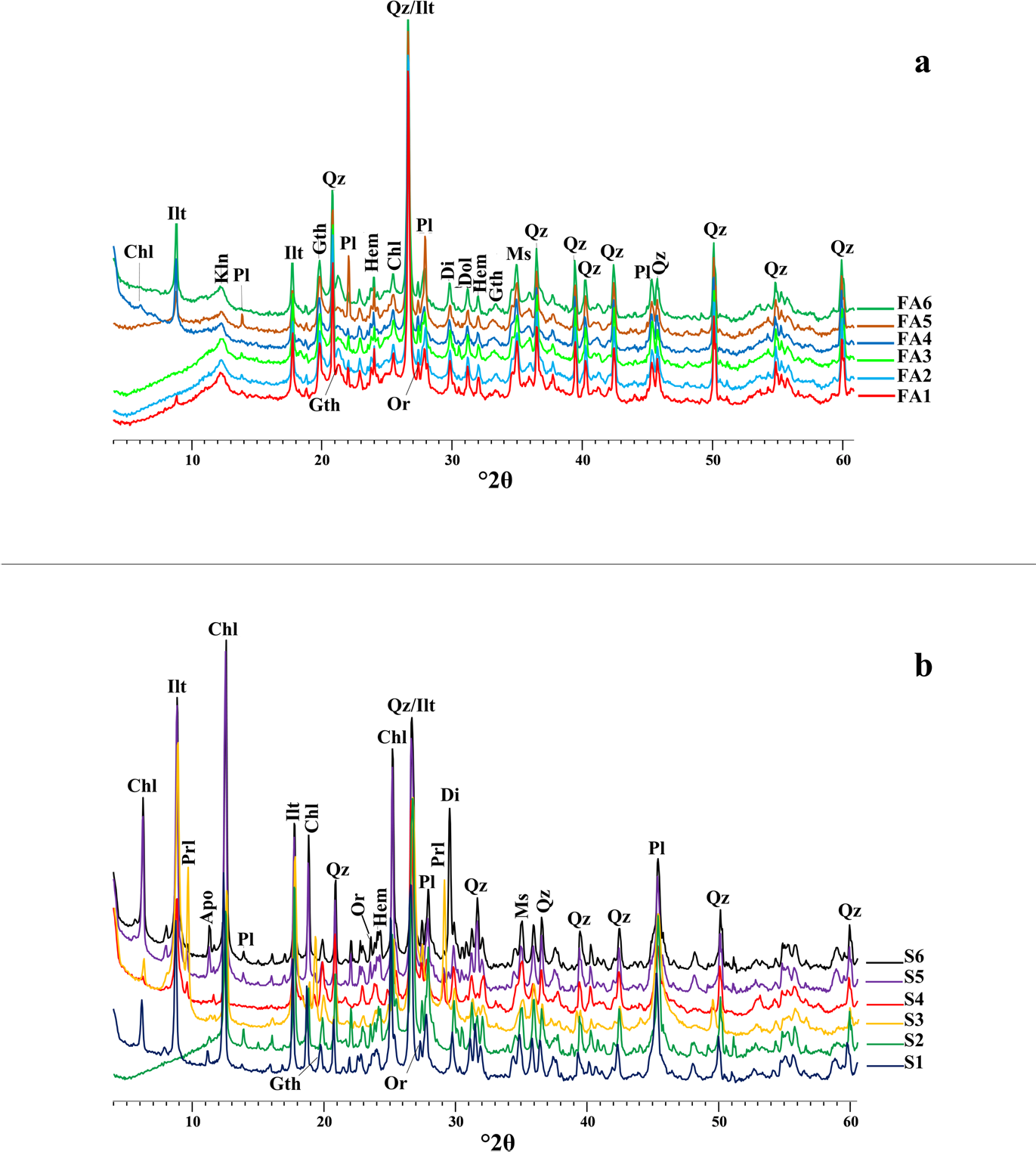
Fig. 7 Whole-rock powder XRD patterns of subsoil layers a and their parent rocks b of Fran Ali samples
The mineral identification and quantification suggest a similarity between the present study and that by Reference Barrios Neira, Martín de la Cruz and Montealegre ContrerasBarrios Neira et al. (2012). The latter estimated large proportions of Qz and mica (Ms/Ilt), which dominated over the other types of minerals (Chl, Kln, Fs, and calcite).
Based on XRD, the clay fractions of the Fran Ali samples (FA1, FA2, FA3, FA4, FA5, and FA6) all have a similar mineralogical compositions. Clay minerals comprise 43–50% and Qz with its intense characteristic peaks, 29–34%. Chlorite (0–2%) is present with a less intense peak at 14.8 Å in the natural sample, which shifts to 16.75 Å in the glycol sample, and in the opposite direction in the 550°C treatment, suggesting that the Chl is mixed with swelling layers (Fig. 8a,b-f).
Illite (19–27%) is characterized by a major peak at 10 Å in the natural test, followed by a secondary peak at 5 Å; Qz is identified by its 3.34 Å peak (Fig. 8a–f). These peaks remain the same for both the glycol and the heated samples. The 7.18 Å and 3.58 Å peaks indicate the presence of Kln (5–9%) in the natural test (Fig. 8a–f). These peaks do not change position after glycol treatment but disappear in the heated samples. Note that the Kln peak is broad, which means that it is poorly crystallized. Goethite and Hem crystals were also observed but with low intensity.
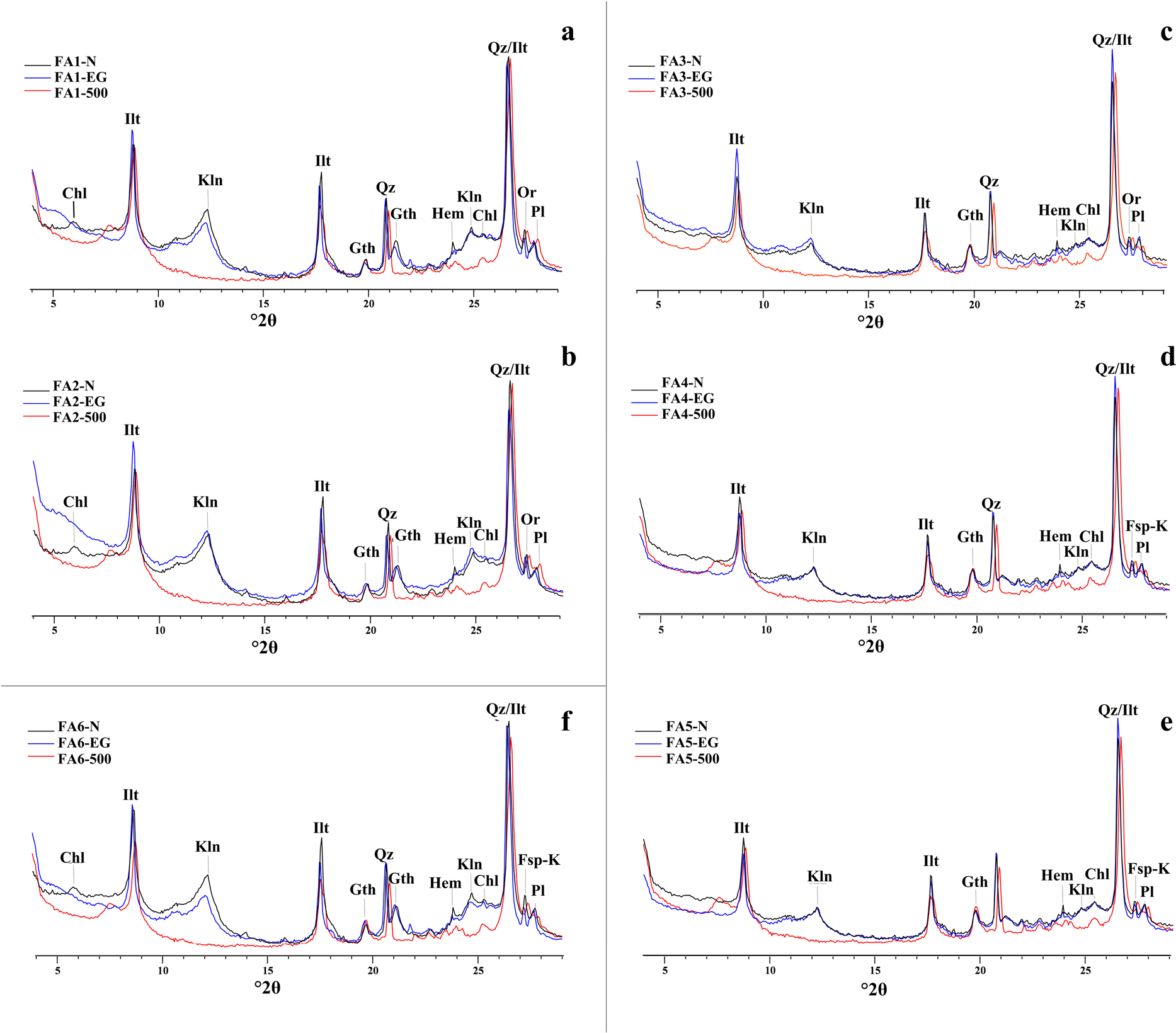
Fig. 8 XRD patterns of oriented subsoil samples taken from the Fran Ali samples
The fine-grained material (clay fraction) extracted from Silurian phyllites (S1–S6) showed the presence of three essential mineral substances: Ilt (27%), Chl (32%), and Qz (31%) (Fig. 9a, f). In fact, Ilt was confirmed by the 10.04 Å (d 001), 5.02 Å (d 002), and 3.34 Å (d 003) peaks that remain unchanged in both glycol- and heat-treated samples. Chlorite was the second mineral phase uncovered by clay-fraction analysis, manifested by its four peaks at 14.14, 7.07, 4.71, and 3.50 Å, which had a lower intensity in the heated sample. In fact, Chl is the more dominant clay mineral in all the phyllite samples. Its even-order reflections (d 002) and (d 004) were more intense than the odd-order reflections (d 001) and (d 003), which suggests that these chlorites are rich in iron (Reference Hénin, Rautureau and CaillèreHénin et al., 1982). In addition to Chl, Qz is present as shown by peaks at 4.26 and 3.34 Å; it is often associated with Pl and Hem. In contrast to soil samples, phyllites show narrow peaks, reflecting the good crystallinity of the minerals there.
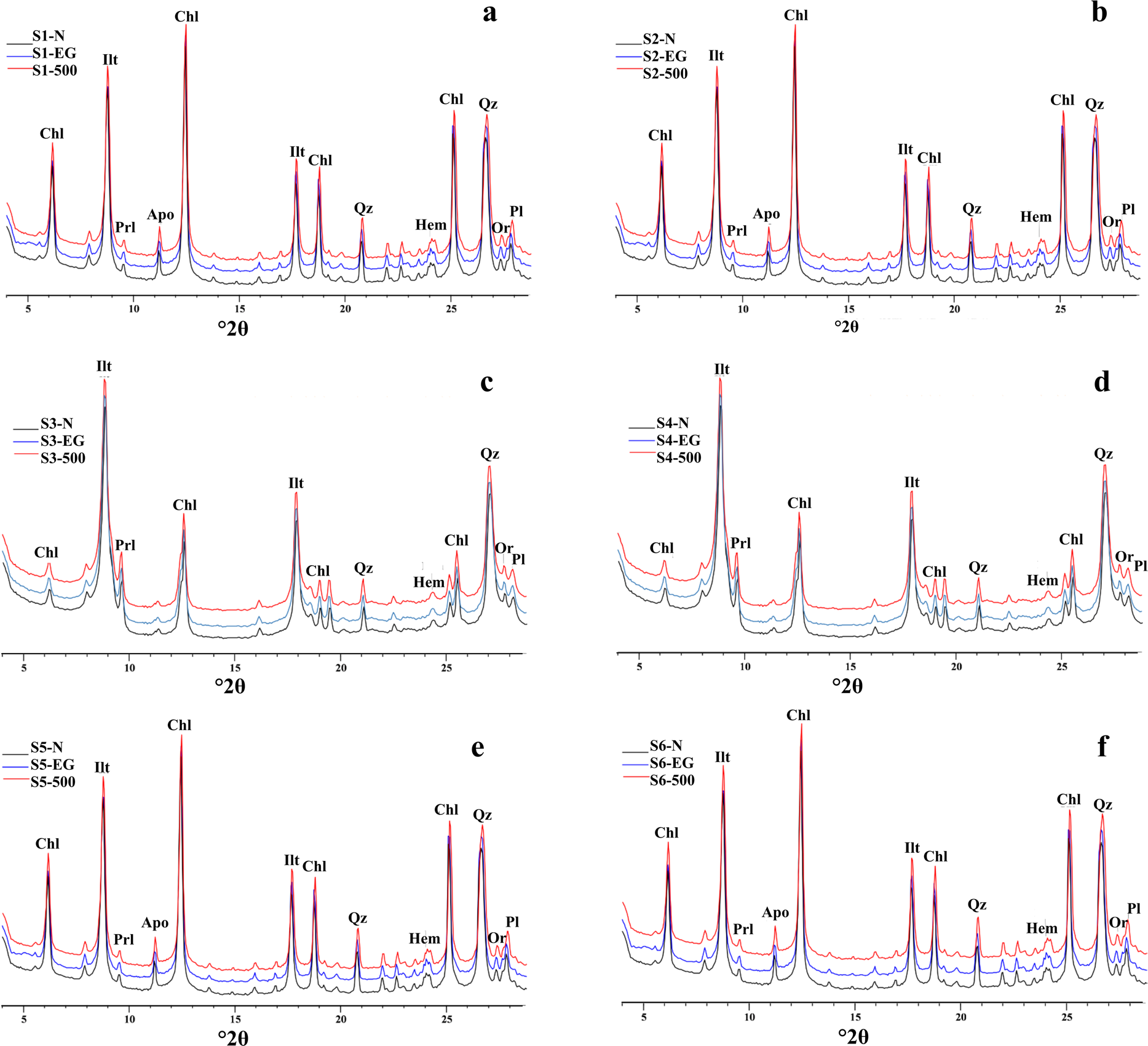
Fig. 9 XRD patterns of oriented samples of the parent rock from the Fran Ali samples
Thermal Behavior Characterization
Thermogravimetric (TGA) and differential thermal (DTA) analyses were carried out on subsoil colluvium and saprolite samples (reddish and yellowish clayey material) and the shaley phyllite material, to quantify the mass lost as a function of temperature.
The thermograms revealed a similar response to heating for both materials (Fig. 10a-b). In fact, a weight loss of 1.8% for the yellowish soils and 1.2% for the reddish soils was observed in the 30–100°C temperature range, which is due to the loss of adsorbed H2O. In the 100–300°C range, a weight loss of ~2.3% was observed in both samples. This weight loss is conventionally linked to hygroscopic water evaporation (Reference AssifaouiAssifaoui, 2002; Reference Hénin, Rautureau and CaillèreHénin et al., 1982). As for the 300–500°C range, a weight loss of ~3% was recorded for the yellowish and reddish subsoil layer. A weight loss in this range is mostly attributed to the dehydroxylation of Kln (Reference Yeskis, Koster van Groos and GuggenheimYeskis et al., 1985). Finally, as the temperature exceeded 500°C, a weight loss of 1.9 to 1.7% was recorded for the yellowish and reddish samples, respectively, which may be explained by Ilt dehydroxylation (Reference Nahdi, Gasmi, Ayedi and Kbir-AriguibNahdi et al., 2001). This mineral is abundant in all colluvium and saprolite samples analyzed using XRD, as shown in previous paragraphs.
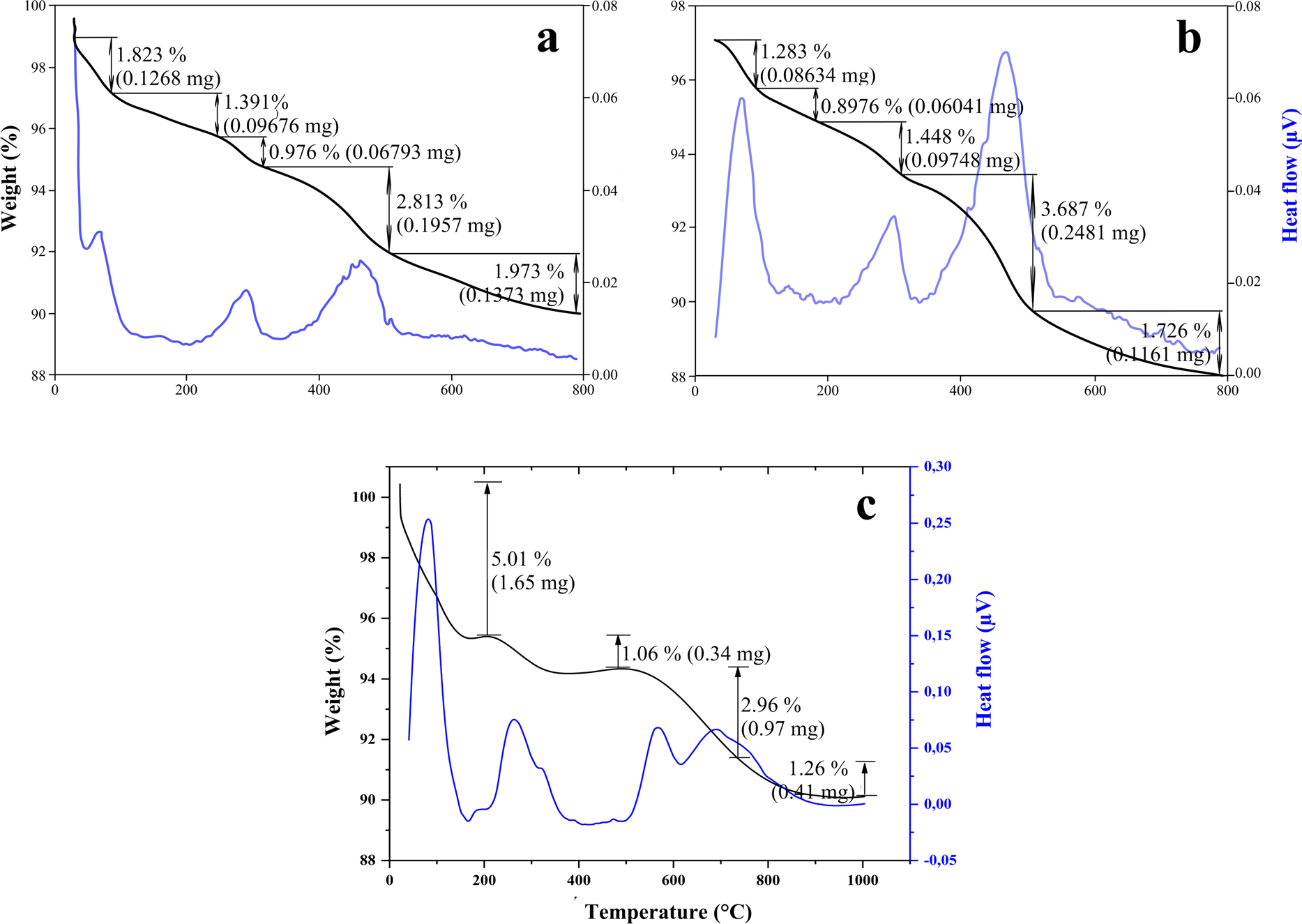
Fig. 10 Thermogravimetric (TGA) and differential thermal (DTA) analysis of a the yellowish soil, b the reddish counterparts of the yellowish soil, and c the shaley phyllite
The thermal behavior of shaley phyllite samples showed a mass loss in steps totaling 10% (Fig. 10c). In the 50–200°C temperature range, a 5% weight loss was observed which can be explained by water evaporation (Reference León, Solano and PeñalozaLeón et al., 2018). The second range (200–500°C) was marked by a 1% weight loss attributed to the liberation of hydroxide molecules present in the Ilt crystals (Reference Abatalib, Gouzouli, Moussa and SaddiqiAbatalib et al., 2014). The third weight loss (4%) was observed at 650–850°C, and is related to Chl dehydroxylation (Reference Ross and KodamaRoss & Kodama, 1976).
Discussion
Silurian Shales as a Substitute for Colluvium and Saprolite
In Fran Ali, the clayey colluvium used by the potters is rich in Ilt, Chl, and Qz and poor in Kln. The shales contain more Ilt and Chl than Qz and little or no Kln. The shape of the XRD peaks revealed that the shale and colluvium samples have similar mineralogical compositions but different crystallinities (higher in the shale).
The colluvium and saprolite layers derived from the weathering of Silurian shales conserve lithological and mineralogical characteristics.
The abundance of clay minerals in the materials investigated can influence significantly the final properties of ceramic objects in general and terracotta products in particular, with Ilt reducing the melting point of the material (Reference Ferrari and GualtieriFerrari & Gualtieri, 2006); and Chl having a large iron content. In the present case study, Qz, Ilt, and Chl are the main constituents of the subsoil samples due to the low metamorphic grade of their parent rock.
Plasticity is also a crucial physical parameter for the preparation, handling, and shaping of pottery paste. It is defined as the property or capacity of a clay to permanently retain its shape without cracking after being subjected to deformation (Reference Maritan, Nodari, Mazzoli, Milano and RussoMaritan et al., 2006; Reference MooreMoore, 1963; Reference ReedReed, 1995). All materials showed medium to high plasticity which makes them appropriate for the production of traditional ceramics, especially in terms of the prevention of cracking during the shaping process (Reference Dondi, Fabbri and GuariniDondi et al., 1998).
Fine-grained materials are most suitable for the manufacture of ceramics (Reference MurrayMurray, 2006; Reference Strazzera, Dondi and MarsigliStrazzera et al., 1997). The abundance of calcium carbonates (CaCO3) in the raw materials used in pottery can affect the quality of the final products, and can even cause technical problems, namely, the formation of white nodules or cracks after the end of firing (Reference Cultrone, Rodriguez-Navarro, Sebastian, Cazalla and De La TorreCultrone et al., 2001). In the present case, the CaCO3 content was < 9% in all samples. The effects of such a small carbonate content on the firing process should be minimal, therefore, and may even serve to increase the porosity of the paste, which helps CO2 to escape during the firing process (Reference El Ouahabi, Daoudi, Hatert and FagelEl Ouahabi et al., 2015).
In addition, Si and Al are among the dominant elements in the colluvium and shale samples and are the major components of the crystal lattice of Ilt, Chl, and Kln. In Fran Ali, the high SiO2 content is associated with Qz (Fig. 11). If the SiO2 concentration is between 35 and 85%, then it acts as a refractory material during ceramic production (Reference KornmannKornmann, 2005; Reference Wetshondo OsombaWetshondo Osomba, 2012). Similarly, when the Al2O3 content is between 9 and 45%, it is considered a refractory material also, which contributes to the whitish color of the finished products (Reference KornmannKornmann, 2005; Reference Wetshondo OsombaWetshondo Osomba, 2012). However, in the present study, and despite the significant amounts of the above-mentioned oxides, the presence of iron oxides influences the color of the paste and dominates over other oxides.
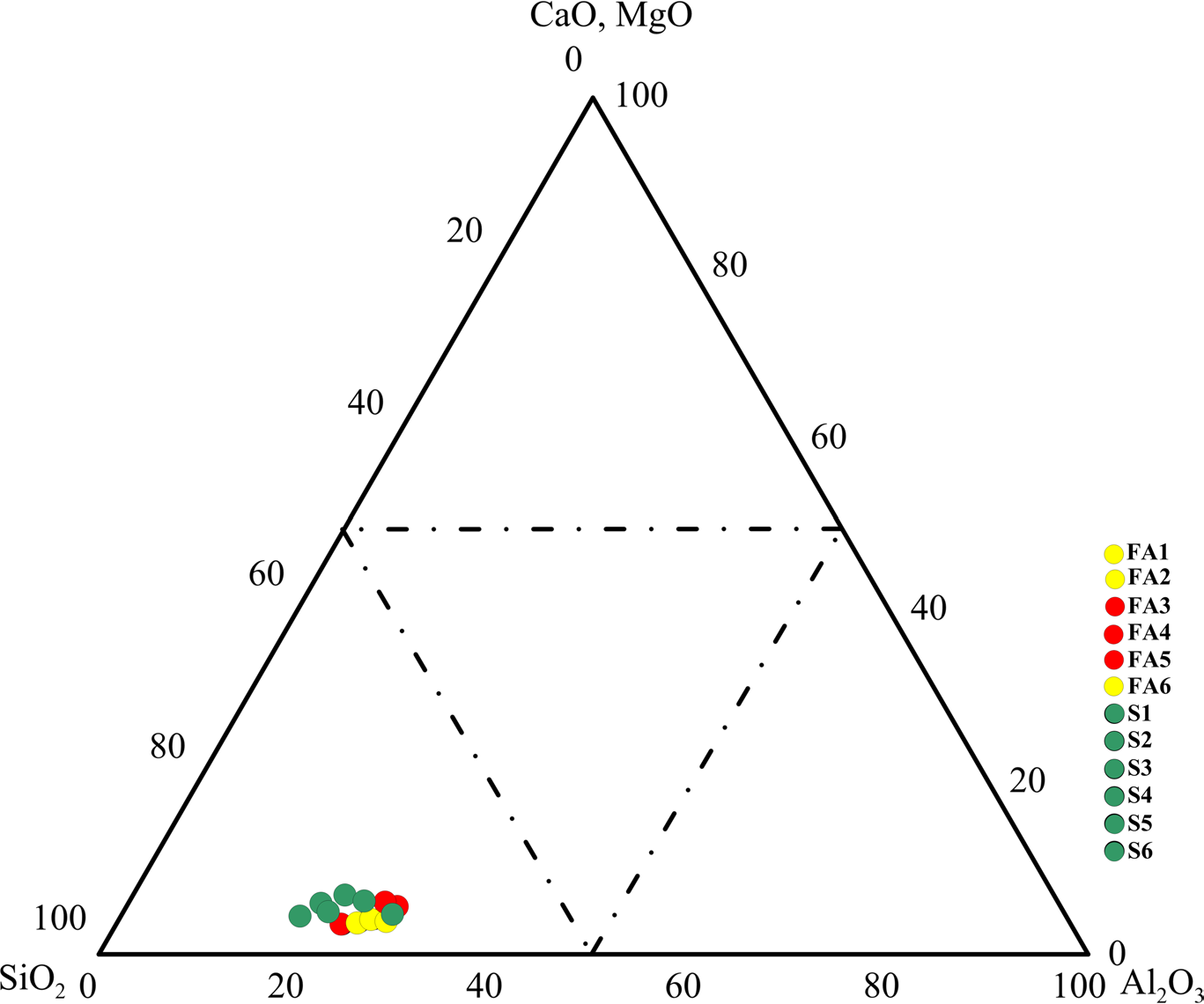
Fig. 11 SiO2/A12O3/CaO, MgO ternary diagram projection of Fran Ali samples
According to the chemical data shown in the ternary diagram (Fig. 12), which led to the classification of raw materials used to produce industrial ceramic objects (Reference Fiori, Fabbri, Donati and VenturiFiori et al., 1989), the Fran Ali samples fall within the range of raw materials suitable for the production of red bricks and red colored artisanal pottery because of their iron oxide concentrations of ~10%. These values confirmed the findings of Reference Baccour, Medhioub, Jamoussi and MhiriBaccour et al. (2009), Fiori et al. Reference Fiori, Fabbri, Donati and Venturi(1989), and Reference MurrayMurray (2006) that clays with Fe2O3 contents of >5% can be used as red firing clays. Such a large iron content in the colluvium and shales of Fran Ali (Table 3), therefore, may be useful in the production of structural ceramics (Reference Daoudi, Hicham, Latifa, Abderrahmane, Jamal, Mohamed, Meriam and NathalieDaoudi et al., 2014) based on the geotechnical, geochemical, and mineralogical characterization of the samples presented here. Furthermore, the parent rock is not very different from the colluvium and saprolite layers exploited by the artisans. Hence, it can be considered as a reasonable alternative for sustainable quarrying in the future.
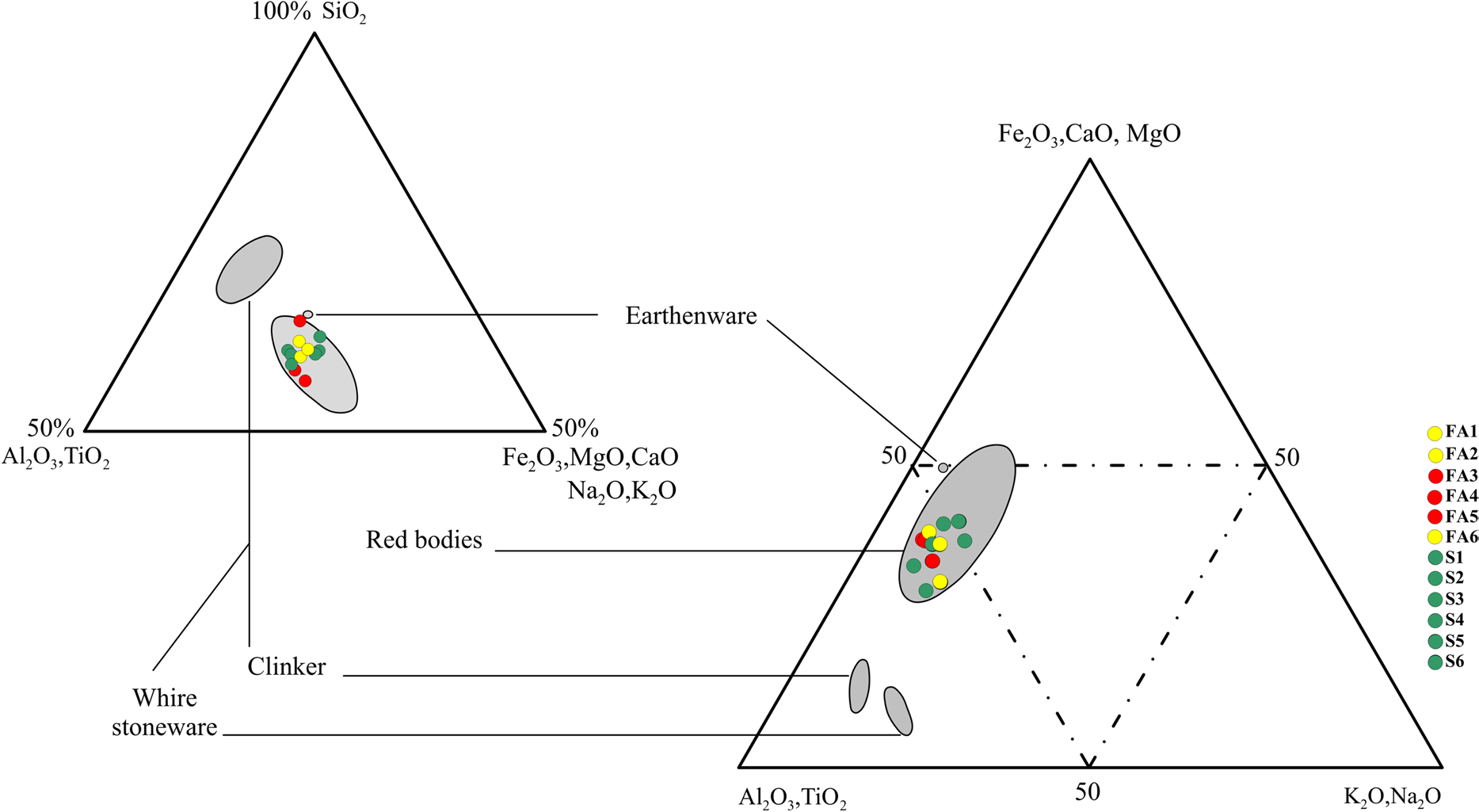
Fig. 12 Projection of Fran Ali samples on the SiO2/A12O3 + TiO2/Fe2O3 + MgO + CaO + Na2O + K2O (upper) and the Fe2O3 + CaO + MgO/A12O3 + TiO2/K2O + Na2O (lower) ternary diagrams according to the Reference Fiori, Fabbri, Donati and VenturiFiori et al. (1989) system
The Feasibility and Practical Aspects of Using the Parent Rock as a Raw Material
Pedogenesis transforms the parent rock and may induce significant mineralogical and/or geotechnical and mechanical changes (Reference Bockheim, Gennadiyev, Hammer and TandarichBockheim et al., 2005; Reference Gaeta, Aldega, Astolfi, Bonechi, Marra, Pacheco, Perinelli and TiberiGaeta et al., 2022; Reference Hole and CampbellHole & Campbell, 1985; Reference Buol, Southard, Graham and McDanielBuol et al., 2011). In Fran Ali, the hydromorphic soils (derived from high-precipitation periods in the Alboran Sea region during Quaternary times (Reference Candy and BlackCandy & Black, 2009; Reference Fletcher and GoñiFletcher & Goñi, 2008; Reference Pérez-Folgado, Sierro, Flores, Grimalt and ZahnPérez-Folgado et al., 2004; Reference RognonRognon, 1987; Reference Sancho, Arenas, Vázquez-Urbez, Pardo, Lozano, Peña-Monné, Hellstrom, Ortiz, Osácar and AuquéSancho et al., 2015) and from slow, deep-seated landslides (Reference Bounab, El Kharim, El Hamdouni and HlilaBounab et al., 2021)) exploited by the potters, were subject to changes in term of color, texture, and mechanical behavior without significant mineralogical changes, according to findings from the present study and to those of (Reference Barrios Neira, Martín de la Cruz and Montealegre ContrerasBarrios Neira et al., 2012). Therefore, the parent rock could be used in the same way as the colluvium and saprolite material to produce pottery paste. However, according to testimonies of local peoples, the extraction of Silurian shale is more difficult due to its greater consistency and hardness. Thus, more time and effort would be required to dig enough material, especially knowing that the quarrying can only by done by pickaxe which makes the extraction of raw materials a physically demanding and time-consuming task. Therefore, modern technological tools, such as jackhammers, would need to be introduced, to facilitate the extraction. On the positive side, the abundance of shale outcrops in the area would reduce the time and effort needed to transport the extracted material from the extraction site to the workshop, which currently may be as much as 2 km apart.
The shaley parent rock is less plastic than its weathered counterpart despite having similar mineralogical assemblages. This may cause some difficulties during the shaping process, which in turn may reduce the quality of the final products. A solution to this problem is the ageing of the phyllitic material, by crushing and depositing stockpiles of raw material in the open air for a certain period of time. This would reduce the cohesion of the material and disturb its primary geological structures. In fact, the early deposition of stockpiles in the open air under the highly variable climate conditions of the temperate southern Mediterranean zone has played an important role in accelerating the weathering cycles of unweathered shales as simulated by the degradability test (action of immersion-drying cycles). This practice is used commonly by brickmakers in the region, who exploit mainly unweathered Cretaceous sedimentary shales of the Tangier unit, and deposited them for 1–2 y before use.
The traditional Pilikwe potters at Moijabana in Botswana accelerate the in situ formation of clays through weathering of granitic rocks by means of a process of crushing, pounding, sifting, and wetting actions (Reference Wilmsen, Griffiths, Thebe, Killick and MolatlhegiWilmsen et al., 2016). Those potters prefer to obtain clay-rich layers in this way, i.e. produced by in situ weathering, in spite of the availability of good quality alluvial clays. This demonstrates the efficacy of the technique for preparation of raw materials.
Conclusions
The raw material extracted from various, random open pits in the Fran Ali has good mineralogical characteristics for firing purposes, especially with regard to the production of pottery objects. This fine material is extracted from inhomogeneous and heterometric colluvium which does not constitute a sustainable resource that can satisfy the growing demand for pottery, a traditional artisanal craft and considered to be an essential component of Moroccan cultural identity. Given the current state of affairs, however, the present authors project the rapid depletion of these resources in Fran Ali, especially due to the increasing demand for the traditional pottery products, and propose the exploitation of the parent rock, the Silurian shales, particularly in areas of fewer greywacke layers. This suggestion would require supplementary preparation steps that may pose a logistical challenge for the potters.
Acknowledgements
The authors thank the people of Jbala in the Fran Ali region for helping them to understand the various production steps in traditional pottery-making.
Data Availability
The data that support the findings of this study are available from the corresponding author, Fatima HILALI, upon request.
Declarations
Conflicts of Interest
The authors declare that there is no conflict of interest.