1. Introduction
Diagenesis refers to a set of processes that begins immediately after the deposition of sediments and continues until the sedimentary rocks are metamorphosed (Tucker, Reference Tucker2001). Various controls affect the diagenesis in marine, meteoric and deep-burial environments (Tucker & Wright, Reference Tucker and Wright1990; Heidari et al. Reference Heidari, Mahboubi, Moussavi-Harami, Gonzalez and Ludvigson2014, Reference Heidari, Shokri, Ghasemi-Nejad, Gonzales and Greg2015). Sedimentary environments, composition, sediment textures and climate primarily control the diagenetic process (Kim & Lee, Reference Kim and Lee2004; Reed et al. Reference Reed, Eriksson and Kowalewski2005). Mineralogy, the presence or absence of fluids, fluid composition, temperature, pressure, sea level fluctuations, pH and Eh are the main controls on diagenesis (Tucker & Bathurst, Reference Tucker and Bathurst1990; Tucker & Wright, Reference Tucker and Wright1990). The diagenetic process in carbonate sediments relates mostly to mineralization (Tucker, Reference Tucker2001). In the diagenetic procedure of siliciclastic successions, some grains such as quartz, feldspars and clay minerals are active (Parcerisa et al. Reference Parcerisa, Gómez-Gras and Travé2005). Furthermore, some parameters such as lithology and composition (Weber & Ricken, Reference Weber and Ricken2005; Oliveria & Truckenbrot, Reference Oliveira and Truckenbrodt2019), palaeoclimate (Reed et al. Reference Reed, Eriksson and Kowalewski2005; Eric et al. Reference Eric, Emile, Romuald, Isaac, Ethel, Paul, Betrant, Gabriel, Shelly and Cedric2019), burial history, subsidence, pore fluid changes (Morad et al. Reference Morad, Ketzer and DeRos2000; Wang et al. Reference Wang, Cao, Costanzo and Feely2018; Oliveria & Truckenbrot Reference Oliveira and Truckenbrodt2019; Poursoltani et al. Reference Poursoltani, Gibling and Pe-Piper2019) and depositional environments (Parcerisa et al. Reference Parcerisa, Gómez-Gras and Travé2005) are considerable in the siliciclastic interval diagenetic process. The diagenetic history of the siliciclastic rocks has received little attention; on the studied succession, in particular there has been no detailed or comprehensive study. Therefore, this work can lay a good foundation for the study of siliciclastic successions in the investigated basin. The results of the current study may represent a firm database of the geochemical properties of many calcite cement types in different diagenetic environments and phases. Many studies have used stable isotopes, trace elements, petrography and cathodoluminescence (CL), but making a connection between them is rarely considered. This study uses all the mentioned methods to provide a data source to help other researchers who access just one or two of the mentioned methods. It provides data on the post-depositional history of the East Tethys basin, which could be utilized in studying the history of diagenesis and reconstruction of the paragenetic sequence of the Danian Era in the East Tethys basin.
In most cases, the CL reflects the redox conditions, not the exact diagenetic environments. To determine the diagenetic environments accurately, it is necessary to have the oxygen and carbon isotope ratios as well as trace element data. Each one of the methods alone can be of some help, but to make the best and most accurate interpretation, it is necessary to use the mentioned methods and data.
2. Geological setting
The Kopet-Dagh Sedimentary Basin was formed after the Early Cimmerian orogeny during the Triassic Period. It formed along with the opening of the Neo-Tethys Ocean in SW Iran. This was an intracontinental basin located in NE Iran, SE Turkmenistan and NW Afghanistan, and formed the northern part of the modern Alpine–Himalayan Super-Mountain Belt (Afshar-Hrab, Reference Afshar-Harb1994; Robert et al. Reference Robert, Letouzey, Kavoosi, Sherkati, Muller, Verǵesb and Aghababaei2014). Compressive forces caused by the opening of the Neo-Tethys Ocean in the SW part of the Iranian plateau led to the closure of the Tethys Ocean. The remnants of the ancient oceans have remained as an intercontinental basin in the northern Iran / southern Eurasian supercontinent (Alavi, Reference Alavi1991; Aghanbati, Reference Aghanabati2003; Robert et al. Reference Robert, Letouzey, Kavoosi, Sherkati, Muller, Verǵesb and Aghababaei2014). Boundaries of most of the sedimentary basins of Iran in the Mesozoic and Cenozoic Eras are coincident with a sea level regression, exposure, lack of sedimentation, and deposition of terrestrial sequences. Such conditions led to the deposition of siliciclastic successions, such as the Kerman conglomerate in Central Iran, the Fajan Formation in the Alborz Basin, and the Amiran Formation in the Zagros Basin (Aghanbati, Reference Aghanabati2003). In the Kopet-Dagh Sedimentary Basin, the K/Pg boundary is accordant with the alluvial and fluvial interval, which is known as the Pestehligh Formation. The lower Palaeocene (Danian) sequence, the Pestehligh Formation, is one of the siliciclastic sedimentary formations of the Kopet-Dagh Sedimentary Basin and composed mainly of conglomerate, sandstone, shale and some evaporitic beds (Heidari, Reference Heidari2008). The studied alluvial and fluvial deposits overlay the Maastrichtian marine carbonates of the Kalat Formation and are overlaid by the Thanetian marine carbonates of the Chehelkaman Formation (Figs 1, 2a, c). The current study considered the lower Palaeocene siliciclastic sedimentary rocks to reconstruct the post-depositional history of the basin. Further attempts were made to connect sea level changes to the diagenetic processes of the studied succession to further comprehend the effects of meteoric, burial and marine diagenetic fluids on fluvial sedimentary rocks.

Fig. 1. Location and geologic map based on 1:250 000 geologic map of the study area, and general geology of the study area (modified from Bolourchi & Moore, Reference Bolourchi and Moore1981; Königshof et al. Reference Königshof, Carmichael, Waters, Jansen, Bahrami, Boncheva and Yazdi2017; Tomić et al. Reference Tomić, Sepehriannasab, Marković, Hao and Lobo2021). The measured stratigraphic sections are marked as Sheikh, Jozak and Garmab with red flags (using Google Earth map). The scale bar is 20 miles (32.2 km).

Fig. 2. (a) New stratigraphic diagram of the Kopet-Dagh Mountains belt and two adjacent basins, i.e. the Amu Darya and the southern Caspian Sea. The stratigraphic position of the Pestehligh Formation is from lower to upper Palaeocene (adapted from Robert et al. Reference Robert, Letouzey, Kavoosi, Sherkati, Muller, Verǵesb and Aghababaei2014). (b) The stratigraphic columns of the studied sections with the specified location of the samples. (c) The outcrop picture from the Danian siliciclastic interval. The studied succession overlays the marine successions of the Maastrichtian and is overlaid by the marine carbonate successions of Thanetian age.
In this study, all the Danian outcrops, including three stratigraphic sections (Jozak, Garmab and Sheikh) in the western Kopet-Dagh Sedimentary Basin, were measured and studied. The Jozak, Garmab and Sheikh stratigraphic thicknesses were 165, 116 and 145 m, respectively (Fig. 2).
3. Methods and material
The post-depositional history of the Danian siliciclastic sequences was considered using petrography, oxygen and carbon stable isotope ratios and trace element contents. A total of 76 thin-sections were prepared from the mentioned sections to evaluate the diagenetic evidence of the lower Palaeocene interval. The thin-sections were investigated by an Olympus polarizing microscope at the Ferdowsi University of Mashhad and Shahid Chamran University petrography laboratories. The petrographic studies helped to identify the diagenetic features. Thin-sections were then examined by CL microscopy at the Department of Geology, Ferdowsi University of Mashhad. Appropriate samples were selected to consider the geochemical content. Using petrographic and CL microphotographs for geochemical analysis, a total of 23 samples were prepared.
Because the purpose of the geochemical data of this study was to identify the diagenetic history, bulk samples were not used. Instead, accurate sampling from different cement types in the pores and veins was performed using a stereo microscope equipped with a dental drill with micron-sized drill bits. A sample preparation map was prepared from the CL images, and the samples were accurately prepared from the different cement types with various luminescence properties for the geochemical study. Each cement generation, according to the time and phase of formation, has been affected by fluids with different elemental and isotopic compositions. Bulk sampling blends the powdered samples and prevents access to correct data. Therefore, in order to accurately understand each generation, the timing of formation, the elemental and isotopic content, it is necessary to do point sampling with the help of CL images.
About 20–50 μg from each sample was powdered by a dental drill with tungsten burrs. This type of drill bit was used to prevent contamination of drill bit erosion. Based on CL images and maps, points were determined for geochemical sampling. Moreover, some thin-sections were stained with alizarin red solution to differentiate dolomite from calcite mineralogy using the Dickson method (Dickson, Reference Dickson1965). Trace element analysis was accomplished by the inductively coupled plasma – mass spectrometry (ICP-MS) equipment at the Elements Laboratory of the University of Kansas, USA. Furthermore, the oxygen and carbon stability was analysed at the Isotope Laboratory of the University of Kansas, USA. Due to the high accuracy of the applied isotope analysis equipment in this study, the samples were selected and powdered by a binocular stereo microscope. By combining the data from the petrographic, CL, trace element and isotope studies, the diagenetic environments of the Danian siliciclastic interval were discovered in the study area. Finally, the diagenetic model of the studied succession was reconstructed in the west of the east Tethys basin.
4. Results
4.a. Petrography
Based on the petrographic study of the sandstone and conglomerate sequences, the diagenetic features of the Danian interval, the Pestehligh Formation, were identified. By combining the petrographic and geochemical data, the processes, history and paragenetic sequence of the lower Palaeocene fluvial succession in the study area were considered. In the studied thin-sections, some diagenetic processes such as physical and chemical compaction, several types of cementation, alteration, dissolution, porosity, fractures and fillings were observed. The diagenetic features are described below.
-
Compaction: Two types of physical and chemical compactions were identified in the studied siliciclastic rocks.
-
Physical compaction: The siliciclastic rocks of the Pestehligh Formation display some evidence of a medium compaction (Fig. 3a, c). In some samples, rock fragment grains show deformation, and some tougher grains such as quartz are immersed in the looser grains such as mudstone and carbonate rock fragments. In some cases, the compaction process destroyed the original arrangement and created concave–convex contacts between quartz grains (Fig. 3b).
-
Chemical compaction: In the studied sandstone samples, chemical compaction was less observed (Fig. 3d).
-
Cementation: Several cement types identified in the studied siliciclastic rocks of the Danian sequence are described here.
-
Iron oxide cement: The iron oxide content has turned the sandstone beds of the Pestehligh Formation red and brown. Iron oxide cement was also seen in the studied thin-sections (Fig. 3e). This type of cement was mainly observed in the contacts between the grains.
-
Calcite cement types: The poikilotopic and blocky cement types were most observed in the studied siliciclastic rocks (Fig. 3f, g). The poikilotopic cement type is inclusive and mostly surrounded by one or more grains (Fig. 3f).
-
Poikilotopic calcite cement: This type of cement refers to the large crystals of sparry calcite which contain several grains (Fig. 3f, h, i and j) and was abundant in the studied rocks. In some cases, they have formed on previous-generation cement and are discriminable by the different extinction angle. This type of cement has a crystal size larger than a few millimetres in the studied samples (Fig. 3f).
-
Blocky calcite cement: This type of cement is identified by the large size of the cement crystals and their sharp boundaries in the studied rocks. They mainly fill the pores in the rocks which have been created by dissolution, porosities between the grains, and fractures. Blocky cement is predominant in the Pestehligh Formation sedimentary rocks (Fig. 3g).
-
Meniscus and pendant calcite cements: The crystal size of these two cement types is c. 10 to 20 µm. They precipitated between and below the grains and are discriminable by different extinctions than the next-generation cements (Fig. 3f).
-
Rim: The size of the crystals of this cement type is c. 10 to 20 µm. They formed around some of the grains. Unlike the meniscus and pendant types of cement that precipitate in some specific parts, this type surrounded grains more uniformly (Fig. 4f). Similar to the meniscus and pendant cements, rim cement also has a different extinction than the next-generation cements.
-
Alteration: This phenomenon is the most common diagenetic process in sandstone sequences. During this process, the unstable minerals alter under certain conditions. In the sandstone sequences of the studied formation, some of the feldspar grains were seen to have been altered along their cleavage surfaces, but some others have not been altered (Fig. 3h).
-
Dissolution: This is the process by which a part of the rock dissolves and its porosity expands. The studied samples were affected by the dissolution process. In some cases, the quartz and feldspar grains dissolved, and in other cases the voids left by dissolution filled up or were substituted by calcite minerals (Fig. 3h–j).
-
Secondary porosity: In the Pestehligh Formation, the dissolution of some feldspar and quartz grains in lower abundance led to the development of porosity. Conversely, the cement formation in the inter-grains spaces reduced the porosity of the studied siliciclastic succession.
-
Fractures and vein fillings: The scarce fractures in the Pestehligh Formation either filled with calcite or remained empty (Fig. 3e). Some of the fractures which were filled with calcite cement were not detectable by polarizing microscopy.

Fig. 3. The diagenetic features of the Pestehligh Formation siliciclastic rocks. (a) Yellow arrows point out the physical compaction. (b) Bending of the mudstone rock fragment; the dashed line indicates the curvature of the grain. (c) Physical compaction; yellow arrows indicate the depression of the quartz grains in a mudstone rock fragment. (d) Chemical dissolution because of the overburden pressure; yellow arrows show the dissolution of quartz at the grain contact. (e) Iron oxide cement in natural light (plane polarized light) is marked by red arrows. (f) Poikilotopic cement –red arrow shows a grain floating in cement which is uniform in extinction; yellow arrows show the grain rim cement surrounding grains; blue arrows show the meniscus cement between two grains. (g) The process of calcite cement formation; red arrows show blocky cement in sandstones. (h) The alteration process; yellow arrows show the feldspar alteration and weathering. (i) The dissolution process; the yellow arrow indicates the dissolution of part of the background limestone cement. (j) The substitution process; yellow arrows show that, on the edge of the quartz grain, calcite substitutes the silica (to make a contrast, the quartz is in extinct situation in crossed polarized light). (k) The processes of dissolving, fracturing and filling of quartz grain – yellow arrow shows the dissolution of the quartz grain; red arrow points out a fracture in quartz grain filled by calcite. (l) Fracture and filling; yellow arrow shows a fracture which has been filled by calcite. The scale bar is 1 mm.
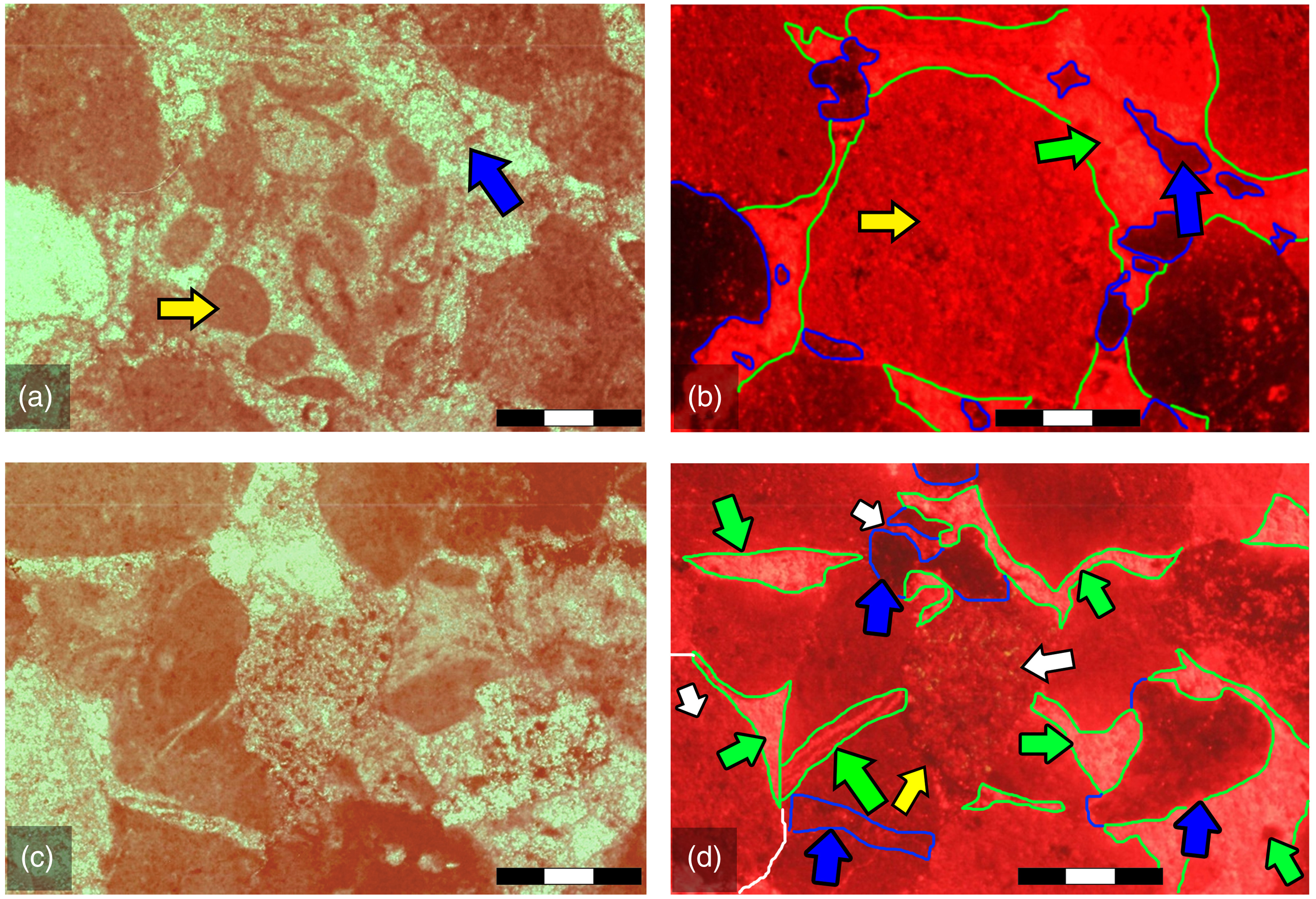
Fig. 4. Photomicrographs in natural light (plane polarized light), CL photomicrographs of sandstone and micro-conglomerate samples of the Pestehligh Formation. (a) Thin-section from the studied sandstone samples; blue arrow shows the inter-grains calcite cement, and yellow arrow indicates the blocky cement that has filled the space between the grains. (b) The CL photomicrograph from (a); blue arrow shows dark luminescent calcite cement, green arrow shows light luminescent calcite cement, and yellow arrow shows the difference between the inter-grains cement and carbonate inside a rock fragment. (c) Photomicrograph of a thin-section in natural light provided from the studied sandstone samples; yellow arrow shows a fracture that has been filled by cement. (d) The CL photomicrograph of Figure 3c yellow arrow shows the dark luminescence of the filled fracture; green arrows and areas show the bright luminescent inter-grains blocky cements; blue arrows and areas show the centre of the pores between grains that have been filled by dark luminescent blocky cements; and the white arrows show the dull luminescence. The scale bar is 1 mm.
4.b. Cathodoluminescence (CL)
In this study, CL images were used to interpret redox conditions of fluids in diagenetic environments. A geochemistry sampling guide map from the CL images is provided (Fig. 4). The blocky cement type in the studied samples shows different luminescence under the CL microscope, which may indicate formation in different diagenetic phases and environments (Fig. 4b). In CL terms, the meniscus and pendant calcite cement types show dark luminescence (Fig. 4b).
Based on the positions of the formation and the luminescence, calcite cements in the studied samples were categorized into four types: (1) the cement around the grains with dark luminescence (such as rim, pendant gravity and meniscus); (2) inter-grain cements with dark luminescence (such as some of the blocky and poikilotopic cements); (3) inter-grain cements with bright luminescence (such as some of the blocky and poikilotopic cements); and (4) the cement-filled fractures with dark luminescence (some of the blocky cements). Each of these four types of cements was sampled and analysed for elemental content as well as oxygen and carbon isotope ratios. Analysis results also confirmed their geochemical differences (Table 1; Fig. 5). Using the CL microscope, the difference in luminescence between the grain and the filled fractures luminescence is quite obvious (Fig. 4d).
Table 1. Geochemical data of the Danian Pestehligh Formation in the west part of Kopet-Dagh Basin


Fig. 5. The geochemical cross-plots from three studied stratigraphic sections. The data belong to four cement types:, dark luminescence around the grains (rim, meniscus and pendant gravity), bright luminescent inter-grain cements, dark luminescent inter-grain cement, and dark luminescent vein fillings cements. (a) Cross-plot of δ18O/ δ16O ratios vs δ13C/ δ12C. The different ratios of each group are clear. (b) Cross-plot of Sr/Mn ratios vs δ13C/ δ12C; the highest Sr/Mn ratios are recorded from the dark luminescent inter-grain and the dark luminescent vein-filling cements, both of which most probably precipitate in meteoric environments after uplifting. This cross-plot indicates that the oxic conditions in the Earth’s surface diagenetic environments cause the Mn to cease entering the mineralogical network of calcite cements. On the other hand, the lightening trend of the carbon isotope ratios vs the increasing of the Sr/Mn ratios confirms the meteoric fluids flow in oxic conditions. (c) Cross-plot of Sr values vs δ13C/ δ12C. The effects of the meteoric fluids in reducing Sr values in dark luminescence around the grains (including the rim, pendant gravity and meniscus), the dark luminescent inter-grains and the dark luminescent vein fillings are obvious. The lighter ratios of the carbon isotope with lower values of Sr are a sign of the influence of meteoric diagenetic fluids. (d) Cross-plot of Sr vs Mn values in most cases shows negative trends. (e) Cross-plot of Fe/Mn ratios vs δ18O/δ16O. In all cements except the dark luminescent, inter-grains show a negative trend. (f) Cross-plot of Fe values vs Mn values in four cement types that show a negative trend for the bright luminescent inter-grain cement, but positive trends for the three other cement types.
4.c. Geochemical analysis
In this study, 29 samples from three stratigraphic sections were analysed to obtain isotopic (O, C) and elemental (Ca, Mg, Sr, Na, Fe, Mn) data.
-
Magnesium (Mg): Its value varies from 0.24 % to 0.81 % in the analysed calcite cement types.
-
Strontium (Sr) and sodium (Na): In the examined samples, Sr values range from 24.31 to 1363.87 ppm. The lower amounts belong to surrounding grains cement type (including rim, pendant gravity and meniscus cements), and the higher values were seen in the cements, which formed inter-grain spaces (including blocky and poikilotopic cements). Na values in the studied calcite cements varied from 201.65 to 1008.77 ppm. The highest amounts of Na belonged to the bright luminescent inter-grain cements. The lowest values belong to grain rim cement types without luminescence. The values of Na in fracture-filling cements are between two previous types of cements.
-
Manganese (Mn) and iron (Fe): The value of Mn in the studied calcite cements varied between 14.28 and 335.14. The values of Fe fluctuated between 514.17 and 1305.36. The highest values of Fe and Mn were found in the inter-grain cement type with bright luminescence and the fracture-filling cement, respectively, and the lowest values were reported from the rim cement with dark luminescence.
-
Oxygen and carbon isotopes: The oxygen isotope ratios in the studied samples varied between 1.14 ‰ VPDB and −6.48 ‰ VPDB, and the carbon isotope ratios ranged from 2.14 to −7.06 ‰ VPDB. Among the studied samples of the Pestehligh Formation, the lightest ratios of carbon isotope belonged to the Sheikh section (Table 1; Fig. 5).
5. Discussion
5.a. Interpretation
In the early stages of diagenesis, water squeezes out of relatively soft sediments, resulting in a closer arrangement of grains in the rock (Tucker, Reference Tucker2001). The performance of the physical compaction process depends on the sedimentation rate, burial depth and sediment volume (Einsele, Reference Einsele2000; Xi et al. Reference Xi, Cao, Liu, Wu, Yuan, Zhu, Kashif and Zhao2019). The loose arrangement of the sediments in the early stages of sedimentation increases porosity.
In the chemical compaction process, the pressure applied to the sediments is higher than that of physical compaction and occurs at temperatures of c. 100 °C to 200 °C and burial of 1 to 2 km deep (Tucker, Reference Tucker2001; Ahmad et al. Reference Ahmad, Bhat and Azim Khan2006). During this process, the grains’ contacts become convex–concave and zigzag, and the silica dissolves at points of the quartz grains’ contact (Ahmad et al. Reference Ahmad, Bhat and Azim Khan2006). The most probable reason for there being little development of chemical compaction is that the quartz grains floated in a mud matrix, which changes the sediment fabric and arrangement. In some cases, however, increasing the dense arrangement ultimately leads to chemical compaction (e.g. Parcerisa et al. Reference Parcerisa, Gómez-Gras and Travé2005). Moreover, the deposition of primary cements around the grains prevented severe chemical compaction in the Pestehligh Formation siliciclastic rocks.
After depositing sediments in different sedimentary environments, the diagenetic fluids flow through the sediment pores, causing cementation in the sediments. Depending on the diagenetic fluids and environments, numerous types of cement form in sedimentary rocks (Heidari et al. Reference Heidari, Mahboubi, Moussavi-Harami, Gonzalez and Ludvigson2014, Reference Heidari, Shokri, Ghasemi-Nejad, Gonzales and Greg2015).
Usually, iron oxide cement precipitates in vadose, phreatic and shallow burial zones after tectonic uplifts. They also form in deep burial diagenetic environments under the influence of alkaline oxidizing diagenetic fluids (Einsele, Reference Einsele2000). The iron oxide cement type represents semi-arid (Weibel, Reference Weibel1998) and oxic conditions (Reed et al. Reference Reed, Eriksson and Kowalewski2005). Because of the weathering of other rocks, iron is released and dissolves in pore fluids in the fluvial siliciclastic deposits. This process in an oxidant environment forms iron oxide cement (Moussavi-Harami & Brenner, Reference Moussavi-Harami and Brenner1993).
The calcite cement types precipitate in most sedimentary and diagenetic environments. These cement types could form in many sandstone sequences such as continental red bed sandstones formed in semi-arid to arid climates (Burley & Worden, Reference Burley and Worden2003). These types of cement precipitate as calcite minerals in the pore spaces between sediment grains. They occur when the amount of the dissolved calcium and bicarbonate ions in the diagenetic fluids reaches a supersaturated level. The precipitation of calcite cement is possible in all stages of diagenesis: for example, at lower burial depths due to inter-grain fluid evaporation; at deeper burial environments due to higher temperatures and pH levels; and as a substitution of silica by meteoric water rich in calcium carbonate (Tucker & Wright, Reference Tucker and Wright1990; Burley & Worden, Reference Burley and Worden2003). If the pH of the environment is acidic, the formation of calcite cement is usually triggered by a higher concentration of calcium and bicarbonate ions in the pore fluids (Worden & Morad, Reference Worden, Morad, Worden and Morad2000).
Many of the sandstone beds of the studied formation are composed of sedimentary rock fragments such as limestone and mudstone grains (Heidari, Reference Heidari2008). The dissolution of these rock fragments can be a source of calcium ions and may saturate the diagenetic fluids to form poikilotopic and blocky cements in late diagenesis stages.
The formation of poikilotopic cement can be related to the slow nucleation of calcite crystals and their low growth rate. They could form in burial and meteoric diagenetic environments and occur by alkaline fluids. They may form after the quartz and feldspar dissolution in the siliciclastic succession (Tucker & Wright, Reference Tucker and Wright1990; Heidari et al. Reference Heidari, Mahboubi, Moussavi-Harami, Gonzalez and Ludvigson2014, Reference Heidari, Shokri, Ghasemi-Nejad, Gonzales and Greg2015; Xiong et al. Reference Xiong, Azmy, Nigel and Blamey2016).
Blocky cement confirms the activities of the diagenetic fluids in meteoric and burial conditions. During the precipitation of this type of cement in meteoric conditions, the dissolved pits and pores are first formed by the infiltration of atmospheric fluids with acidic pH. Subsequently, the primary pores and the voids created by dissolution are filled by this type of cement. Blocky cement may also form in burial environments (Choquette & James, Reference Choquette and James1987; Heidari et al. Reference Heidari, Mahboubi, Moussavi-Harami, Gonzalez and Ludvigson2014; Javanbakht et al. Reference Javanbakht, Wanas, Jafarian, Shahsavan and Sahraeyan2018). This type of cement is often referred to as the second generation of cements (Tucker, Reference Tucker2001; Flügel, Reference Flugel2010).
The meniscus cement type is among the first to form between the grains and the grains’ contact points (Tucker, Reference Tucker2001; Defliese & Lohmann, Reference Defliese and Lohmann2016). The edges of this type of cement are rounded, possibly indicating the effects of gravity. The position of these two cement formations indicates that they are first-generation calcite cements in the studied siliciclastic rocks. Pendant cement formed as a hanging cement beneath the grains. Its shape indicates the effect of gravity in an environment unsaturated with water (Defliese & Lohmann, Reference Defliese and Lohmann2016).
In marine diagenetic environments, the rim type of cement forms in needle and fibrous shapes with two small x, y and one long z-axis (Flügel, Reference Flugel2010). Their fibrous and needle shape is due to high magnesium/calcium ratios in the seawater. In terrestrial environments with fresh water, the reduction of magnesium/calcite ratios and the dimensions of cement crystals become closer and more equant (Defliese & Lohmann, Reference Defliese and Lohmann2016). Furthermore, their uniform distribution around the grains indicates that the diagenetic environment was saturated with diagenetic fluid. Therefore, the early phreatic meteoric environments were the best match to these conditions (e.g. Defliese & Lohmann, Reference Defliese and Lohmann2016).
The alteration process acts predominantly in deeper burial diagenetic environments (Worden & Morad, Reference Worden, Morad, Worden and Morad2000). The passage of diagenetic fluids with different compositions from the sedimentary sequences along with changes in the diagenetic environment temperature and pressure causes the transformation of unstable minerals into stable minerals (Einsele, Reference Einsele2000). The alteration of feldspar grains is controlled by fluids that pass through the sediment pores. The pH of the environment, the activity of the sodium and potassium cations and the presence of hydrogen ions lead to alterations in the feldspar grains (Worden & Morad, Reference Worden, Morad, Worden and Morad2000). Dynamic modelling of diagenetic environments shows that feldspar minerals are typically altered at temperatures of c. 90 °C to 150 °C (Chuhan et al. Reference Chuhan, Bjorlykke and Lowery2000; Reed et al. Reference Reed, Eriksson and Kowalewski2005). Usually, feldspars convert to sericite at high concentrations of potassium cation versus lower silica concentrations (Lanson et al. Reference Lanson, Beaufort, Berger, Bauer, Cassagnabere and Meunier2002; Reed et al. Reference Reed, Eriksson and Kowalewski2005; Weber & Ricken, Reference Weber and Ricken2005). The absence of any silica cement in the sandstone sequences in the studied interval revealed that poor silica fluids passed through the succession. The surviving unaltered feldspar grains in the studied rocks reflect the arid climate of the Danian age in the study area (Fig. 6; Suttner & Dutta, Reference Suttner and Dutta1986). Moreover, a high sedimentation volume at the time of sedimentation helped preserve the feldspar grains (e.g. Suresh et al. Reference Suresh, Ghosh, Kumar and Sangode2004). On the other hand, the placement of some feldspar grains in the matrix field can protect them from contact with diagenetic fluids.

Fig. 6. Reconstruction of the palaeoclimate using the petrography composition achieved from the studied thin-sections and grain counting. The average composition of the studied Danian interval of the west Kopet-Dagh Basin shows the sedimentation in the arid and semi-arid climates, which is consistent with the Kopet-Dagh Basin palaeo-latitude (between 20° and 30° of the northern semi-globe. The palaeo-situation of the Basin is coincident with the deserts belt of the Earth in the Palaeocene Epoch.
Dissolution occurs when there is no other mineral to form during the dissolution. Otherwise, the substitution process occurs and no porosity develops. The dissolution process and substitution take place from the surface conditions in the meteoric diagenetic phase (Xiong et al. Reference Xiong, Azmy, Nigel and Blamey2016) up to burial depths with a temperature of c. 125 °C (Lewis & McConchie, Reference Lewis and McConchie1994). The dissolution of silica and the precipitation of calcite are usually controlled by the pH and time. This process is possible in a pH range from 9 to 10. It works at the meteoric and burial diagenesis by sub-oxic and alkaline fluids (Morad et al. Reference Morad, Ketzer and DeRos2000; Xiong et al. Reference Xiong, Azmy, Nigel and Blamey2016).
The late porosity process may occur during the burial phase of diagenesis, but it may also occur because of meteoric waters (Reed et al. Reference Reed, Eriksson and Kowalewski2005). During the burial stage of diagenesis and after uplift, late porosity expanded due to the infiltration of meteoric fluids.
Fractures usually occur during the uplift of sedimentary sequences in the final stages of diagenesis (Cooke et al. Reference Cooke, Simo, Underwood and Rijken2006; Graaf et al. Reference Graaf, Reijmer, Bertotti, Bezerra, Cazarin, Bisdom and Vonhof2017).
Major reasons for the low development of fractures in the studied succession are the mud matrix of the background and the abundance of rock fragment grains, both of which caused the rock to bend and prevented the fracture from spreading (e.g. Suresh et al. Reference Suresh, Ghosh, Kumar and Sangode2004).
5.b. The CL interpretation
The presence of manganese (Mn) and iron (Fe) in the calcite cement types is related to the redox condition of diagenetic fluids, which may be affected by sedimentary and diagenetic environments. The CL is a very useful tool for detecting diagenesis phases and considering porosity evolution in sedimentary rocks. All calcite cement types are products of the diagenesis process (e.g. Bathurst, Reference Bathurst1975; James & Choquette, Reference James and Choquette1990). Mn is the main known activator element of luminescence (Götte & Richter, Reference Götte and Richter2009; Götze, Reference Götze2012). Other cations, such as lead and some other rare earth elements, may also play a role in the formation of luminescence (Machel et al. Reference Machel, Mason, Mariano, Mucci, Barker and Kopp1991; Habermann et al. Reference Habermann, Neuser and Rochter1996). On the other hand, iron, nickel and cobalt play main roles in quenching luminescence (Mason & Mariano, Reference Mason and Mariano1990). Budd et al. Reference Budd, Hammes and Ward(2000) found that values greater than 17 ppm of Mn could cause a bright luminescence. Lower values could even be sufficient to create a slight luminescence in calcite cements (Habermann et al. Reference Habermann, Neuser and Richter1998). In general, it seems that as long as the Fe concentration is less than 200 ppm, even low levels of Mn can produce a luminescence (Budd et al., Reference Budd, Hammes and Ward2000). As Fe enters the calcite and dolomite crystal networks, the luminescence changes from yellow to orange, red and brown. The red luminescence and very dark brown to black (without luminescence) will appear if the Fe concentration exceeds a few thousand parts per million. Values lower than 100 ppm will not have a negative effect on the luminescence (Budd et al., Reference Budd, Hammes and Ward2000; Götte & Richter, Reference Götte and Richter2009).
Measuring Fe and Mn concentrations in calcite cement types is a useful approach for detecting the redox conditions of the mineral formation, because their redox potentials are different (Cander, Reference Cander1994; Vahrenkamp & Swart, Reference Vahrenkamp, Swart, Purser, Tucker and Zenger1994; Kyser et al. Reference Kyser, James and Bone2002). Under reduction conditions, the Fe and Mn elements easily enter the trigonal crystal network of the carbonates and replace calcium in calcite minerals. By reducing the oxygen level below that of the atmosphere, Mn quickly enters the diagenetic calcite cements.
Various geochemical regimes cause different luminescence in calcite cements. The dark luminescence in calcite cement is interpreted as being formed by oxic fluids with minimum values of Fe and Mn (Barker et al. Reference Barker, Higley, Dalziel, Barker and Kopp1991; Li et al. Reference Li, Goldstein and Franseen2017). In contrast, the bright luminescence reflects the flow of anoxic fluids, which leads to an increase in Mn (Li et al. Reference Li, Goldstein and Franseen2017). The changes in the Fe and Mn values are evident in the analysed samples of the different cement types of the studied formation (Table 1; Fig. 5).
5.c. Geochemistry
The values of Mg in the carbonate samples from tropical regions varied between 0.11 % and 3.2 %. Aragonite minerals contain less than 1 % Mg, while calcite can contain from 1 % to more than 12 % (Rao, Reference Rao1996). The comparison of calcite cements with carbonate cements, which were precipitated in the siliciclastic deposits, is provided here to point out their differences.
In tropical carbonates, Sr values from 813 to 9000 ppm have been reported. In many cases, changes in Sr values occur because of relative changes in the volumes of aragonite and calcite (Rao, Reference Rao1996). Because of the larger dimension of the aragonite inter-crystalline spaces, the Sr element that is larger in size is more consistent with the aragonite crystal structure. Therefore, Sr values are higher in carbonate with higher portions of aragonite mineralogy. The values of Na in carbonate sediments are usually positively correlated with the amount of Sr (Rao & Adabi, Reference Rao and Adabi1993; Rao, Reference Rao1996).
Mn and Fe have low values in aragonite specimens but relatively high ones in calcite (Rao, Reference Rao1996).
Sedimentary environments control the calcite cement types in the early stages of diagenesis. The sedimentary environments of some carbonate cements have already been determined as proximal areas of the alluvial and fluvial systems (Heidari, Reference Heidari2008). In these types of cements, the values of trace elements in the diagenetic fluids are meagre, and the oxic condition is dominant. Many studies have confirmed a link between sedimentary environment and diagenesis (e.g. Ketzer et al. Reference Ketzer, Holz, Morad and Al‐Aasm2003; Dill et al. Reference Dill, Botz, Luppold and Henjes-Kunst2005; Parcerisa et al. Reference Parcerisa, Gómez-Gras and Travé2005). The presence of the Mn and Fe in calcite cements is dealing with the redox conditions of the diagenetic fluids and environments. Greater distance from the source results in more alkaline fluids. Therefore, the Mn and Fe values increase in the calcite cements (Parcerisa et al. Reference Parcerisa, Gómez-Gras and Travé2005). However, later cements such as in mesodiagenesis phases, such as burial, are less affected by the sedimentary environments. They often contain Mn because of the redox conditions. The higher values of Mn often help create a bright luminescence in calcite cements in the mesodiagenesis phase in terrestrial siliciclastic successions (Parcerisa et al. Reference Parcerisa, Gómez-Gras and Travé2005). In burial diagenetic environments, sources of Fe and Mn could be the shale horizons of a formation. Moreover, at temperatures above 50 °C, the transformation of clay minerals releases some Fe and Mn (e.g. Emery, Reference Emery and Marshall1987). The concentrations of some trace elements which are mainly present in seawater, such as Na, Mn and Sr, are severely reduced by the flow of atmospheric fluids (Winefield et al. Reference Winefield, Nelson and Hodder1996; Javanbakht et al. Reference Javanbakht, Wanas, Jafarian, Shahsavan and Sahraeyan2018). Because the studied Danian succession was deposited in the alluvial and fluvial systems, the effects of seawater are absent in the early diagenetic features. Therefore, the initial fluids were depleted of trace elements. This is especially evident in proximal parts of the river system in the study area (such as the Jozak section), which are closer to the source settings. The amounts of major and trace elements are lower in Jozak early cements than in other stratigraphic sections (Table 1). In the later phases of diagenesis, such as mesodiagenesis and telodiagenesis, the effects of the sedimentary environments gradually diminished because of the distance from the early meteoric fluids. The studied siliciclastic succession is sandwiched between two marine carbonate formations known as the Kalat (Maastrichtian in age) and Chehelkaman (Thanetian in age) (Fig. 2). Therefore, in the burial diagenetic environments, the diagenetic fluids from the lower and/or upper carbonate sequences have both been enriched by passing through the marine sequences. Hence, the values of some marine trace elements such as Sr and Na are increased in the burial calcite cements of the studied succession. Consequently, the diagenetic fluids in the burial environments were expected to be affected mainly by marine carbonate sequences, even after the uplift environments. A significant increase in the amounts of Sr, Na and Mn in the bright luminescent inter-grain cements confirms the effects of the diagenetic fluids which crossed the upper and lower carbonate sequences (Table 1).
The Sandberg studies revealed that the aragonite seas were dominant in the Cenozoic Era (Sandberg, Reference Sandberg1975). The original mineralogy of the aragonite accelerates the dissolution process of carbonates and causes the release of marine trace elements. In the Pestehligh Formation siliciclastic sequences, because of the carbonate dissolution in the lower and upper marine successions, the released marine elements such as Sr, Na and Mg are raised in many of the calcite cements. The stable isotopic data indicate that in the cements that are enriched with marine trace elements, oxygen isotope ratios range from −8 to −10 ‰ Vienna Pee Dee Belemnite (VPDB) (Table 1). This result confirms the cement precipitation in burial diagenetic environments (e.g. Heidari et al. Reference Heidari, Mahboubi, Moussavi-Harami, Gonzalez and Ludvigson2014, Reference Heidari, Shokri, Ghasemi-Nejad, Gonzales and Greg2015). The values of the carbonate succession’s main and trace elements could be clues to identifying mineralogy, sedimentation rate, water temperature, redox conditions, element distribution or diffusion coefficient, fluid composition, PCO2 value and salinity (Veizer, Reference Veizer1983).
In the studied cement samples, the highest ratios of Sr/Mn were reported from the dark luminescent fracture fillings and dark luminescent cements around the grains (including the rim, pendant gravity and meniscus cements). These higher Sr/Mn ratios are not due to the increase in Sr values, but are due to the decrease in Mn content in oxic conditions of diagenetic environments near the Earth’s surface (Fig. 5b; Table 1). Furthermore, the negative correlation of the Sr/Mn ratios versus the carbon isotope indicates a lightening trend in carbon isotope versus decreases in Mn content. It confirms the inhibitory influences of surface oxic conditions on the entrance of Mn into calcite cement types. Consequently, a dark luminescence is made in the fracture-filling cements which formed close to the Earth’s surface. Some other fracture-filling cements that show a bright luminescence can be considered in connection with greater depths before reaching the Earth’s surface (Figs 4, 6).
The comparison of main and trace elements of the studied succession also showed two types of positive and negative regression trends. Sr, Mg and Na each displayed a positive regression trend versus each other (Fig. 5c). On the other hand, they showed a negative regression trend versus Fe and Mn (Fig. 5f). By increasing the Mn and Fe contents in the early phases of meteoric conditions, the Sr and Na values decreased (e.g. Bathurst, Reference Bathurst1975; Winefield et al. Reference Winefield, Nelson and Hodder1996; Heidari et al. Reference Heidari, Mahboubi, Moussavi-Harami, Gonzalez and Ludvigson2014, Reference Heidari, Shokri, Ghasemi-Nejad, Gonzales and Greg2015). This process is made more possible by the flow of the meteoric fluids in the sediments. It leads to a decreasing trend in Sr and Mg and an increasing trend in Fe and Mn (Budd & Land, Reference Budd and Land1990).
Oxygen and carbon isotopes are very useful for understanding the nature and timing of fluids that precipitate calcite cement types (Li et al. Reference Li, Goldstein and Franseen2017; Javanbakht et al. Reference Javanbakht, Wanas, Jafarian, Shahsavan and Sahraeyan2018). The probable explanation for the lightest ratios of the carbon isotope in the Sheikh section is the lack of limestone deposits of the Thanetian Chehelkaman Formation in this section and the direct sedimentation of the Khangiran Formation (Ypresian in age) dark shale deposits on the Danian Pestehligh Formation (Fig. 2). In other words, the higher organic content of the Ypresian dark shales has affected the diagenetic fluids and caused the carbon isotope ratios in the Sheikh section to be lightened (Table 1).
An accurate study of diagenetic history from sedimentation to uplifting and sampling by a geologist requires a geochemical analysis of the successions. The diagenetic fluids in various phases, i.e. eodiagenesis, mesodiagenesis and telodiagenesis, have different properties and varying effects on sedimentary successions. These fluids usually precipitate different cement types. It is not possible to detect the diagenetic phases without quantity and semi-quantity analytical data. Concerning the carbonate cements, trace elements, oxygen and carbon isotopes have a good performance (e.g. Mazzullo, Reference Mazzullo2000; Heidari et al. Reference Heidari, Mahboubi, Moussavi-Harami, Gonzalez and Ludvigson2014, Reference Heidari, Shokri, Ghasemi-Nejad, Gonzales and Greg2015).
5.d. The paragenetic sequence
After sedimentation, the various diagenetic processes start to change the deposits. A complex of diagenetic features occurs that can be identified and deciphered using petrographic and geochemical data. After the identification and interpretation of the diagenetic features and processes, a paragenetic sequence can be reconstructed. Based on the interpreted diagenetic processes and their geochemical properties, the paragenetic sequence of the interval studied herein was reconstructed. Early diagenesis involves the syn-sedimentary processes to shallow burial environments. Middle and late diagenesis includes a variety of processes that occur in the deeper burial to after the uplifting of diagenetic environments.
5.d.1. The early meteoric diagenetic environment (Eodiagenesis phase)
The palaeoclimate had a major influence on early terrestrial diagenesis (Reed et al. Reference Reed, Eriksson and Kowalewski2005). The petrographic data of the studied succession (Heidari, Reference Heidari2008) have been plotted on Suttner and Dutta’s palaeoclimate diagram (Suttner & Dutta, Reference Suttner and Dutta1986). The data of this study revealed deposition in the semi-arid to arid climates (Fig. 6). Early diagenesis is coincident with the meteoric regime (Galloway, Reference Galloway, McDonald and Surdam1984), in which sediments infiltrated by way of meteoric fluids. In this regime type, the fluid flow rate is high and controlled by the permeability and homogeneity of the sandstone masses (Galloway, Reference Galloway, McDonald and Surdam1984). At this stage of diagenesis, processes such as physical compaction and early cementation (iron oxide and calcite grains rim, meniscus and pendant cement types) affected the studied siliciclastic rocks (Fig. 7). The iron oxide cement in this phase had deposited a thin layer in grains’ points of contact, indicating that before the initial compaction of the sandstone sequences, an early cement of iron oxide had formed and covered the surface of the sandstone grains. Due to the relatively higher sedimentation rate in the alluvial and fluvial systems in active tectonic areas, the sandstones at the bottom of the studied stratigraphic sections underwent more compaction, which ultimately led to a decrease in the initial porosity. From a geochemical view, the fluids that precipitate the cements in this phase of diagenesis are depleted of trace elements. The red and brown colours of the studied siliciclastic rocks reflect the oxic fluids in this phase. As mentioned earlier, the meteoric fluids were more depleted in the Jozak section than in other stratigraphic sections due to the proximity to source areas of the alluvial and fluvial systems.

Fig. 7. Schematic diagram of the early meteoric conditions of the Danian age in west Kopet-Dagh Basin. There was not enough time for early meteoric diagenetic fluids to be enriched with trace elements, so they were depleted. Their relevant cements contain the lowest values of trace elements. These fluids are also more under-saturated than calcite, making them suitable for the development of dissolution in carbonate grains and cements. The early meteoric consists of two diagenetic environments: upper, and under the underground water table surface, known as the vadose and phreatic zones, respectively. (a) Because of vadose cement precipitation in the free fluid pores, gravity forms the cements as pendants and hangs them under the grains. Some others, such as the meniscus cements, follow the cohesion of the water molecules with grains and precipitate between grains. (b) Under the underground water table, rim cements surround the grains. The lower development of rim cements in continental diagenetic fluids compared to marine ones is due to the under-saturation conditions more than the carbonates in terrestrial fluids.
The oxic conditions of the Earth’s surface environments prevent the entrance of Mn into the mineralogy network. Thus, some cement types such as the grains rim, pendant and meniscus types that were formed in this phase show a dark luminescence (Fig. 4b, d). Isotopic data showed that the cement types of this diagenetic environment had relatively heavy oxygen ratios (between −1.35 and −1.82 ‰ VPDB) and relatively light carbon isotopes (between −5.32 and −7.06 ‰ VPDB). These isotopic ratios reflect the meteoric conditions in this phase of diagenesis, which could be subdivided into vadose and phreatic diagenetic environments (Fig. 7a, b). The situation and form of the vadose and pheriatic cement types are different. Rim grain cements form in the pheriatic early meteoric, while gravity pendant and meniscus types form in the vadose early meteoric phase (Fig. 7a, b).
5.d.2. The burial diagenetic environment (Mesodiagenesis phase)
In this phase of diagenesis, the studied siliciclastic rocks were subjected to more burial and pressure. The chemical composition of the diagenetic fluids within the pore spaces changed in this phase of diagenesis, which correlates with the compaction regime of Galloway (Galloway, Reference Galloway, McDonald and Surdam1984). In this regime, sediments gradually move away from the Earth’s surface and are affected by the deeper diagenetic environments. A variety of processes have acted on the siliciclastic deposits of the studied succession in this phase, including physical compaction, chemical compaction, cementation, alteration and dissolution of the feldspar and quartz grains, dissolution and substitution of the calcite, and developing secondary porosity (Fig. 8). In the relatively deeper diagenesis phases, the pressure on the rock increases, and the grains’ dissolution starts at the grains’ points of contact and makes zigzag and convex–concave contacts. Some of the grains such as quartz and feldspar are then dissolved by the penetration of alkaline fluids, which are rich in calcium and bicarbonate. In this diagenetic environment, the feldspar and quartz grains tend to be dissolved and substituted by calcite. During the mesodiagenesis phase, some processes, such as dissolution and alteration of the feldspar and quartz grains, can lead to an increase in secondary porosity.

Fig. 8. The burial diagenetic environments of the studied siliciclastic succession. The studied siliciclastic interval is sandwiched between two marine carbonate successions, the Maastrichtian carbonate beneath and the Thanetian carbonate above. Both upward and downward fluids have to cross a marine carbonate pack to touch the Pestehligh Formation. Therefore, some marine trace elements such as Sr and Na have enriched the burial cements of the studied succession.
A marked increase in trace elements contents, a lightening of the oxygen isotope ratios and a relatively heavier carbon isotope ratio are the geochemical properties of this diagenetic phase. In the burial environment cement types, there is a marked increment in seawater-derived trace elements, such as Sr, Na and Mg. The downward- and upward-penetrating fluids in the studied succession were enriched by crossing through the marine carbonate deposits of the upper and lower formations (Fig. 8). The origin of some trace elements, such as Sr, Na and Mg, is not marine fluids, but it is fluids that passed through and were enriched by the marine carbonate rocks (Fig. 8).
The increasing trend of temperature, because of the burial environments deepening, has also caused a lightening in oxygen isotope ratios. However, the maximum of −6.72 in the oxygen isotope ratios in the studied interval indicates a moderate burial depth. The mentioned oxygen isotope maximum was accompanied by relatively heavy ratios of the carbon isotope, indicating the influence of burial diagenesis (Fig. 5a). The characteristic ‘reverse J shape trend’ of the oxygen and carbon isotope ratios discloses the burial diagenetic environments (Fig. 5a). The reduction in alkaline conditions and enrichment of trace elements in burial fluids were facilitated by the entrance of Mn into the mineralogy network of the calcite cement types of this phase. Therefore, the calcite cement types of this phase, including blocky and poikilotopic, which were deposited mostly in the centre parts of the pores, display a bright luminescence (Fig. 4b, d).
5.d.3. After uplift of diagenetic environment (Telodiagenesis phase)
This phase of the diagenesis is in accordance with the Galloway meteoric regime (Galloway, Reference Galloway, McDonald and Surdam1984), in which the palaeoclimate is very important. Some diagenetic processes, including fractures, fillings and dissolution, developed during this diagenetic phase. After the burial diagenetic phase, the Pestehligh Formation was lifted up by the tectonic activities and folding. Because of that uplift, the fractures have to some extent expanded. In this phase, some fractures are filled by calcite cements. Those fillings diminished the porosity and permeability of the studied sequence. In terms of redox conditions, in the later steps of the after uplifting, the meteoric oxic conditions of the near Earth’s surface make a dark luminescence in the fracture-filling cements. The positions of these phase cements, often in fractures, indicate the meteoric environments. These meteoric cements are different from those of the early meteoric environment of the eodiagenesis. It is often difficult to differentiate the early meteoric environments, because their evidence is mostly overprinted by the next diagenetic fluids and processes. The geochemical properties of cements of this stage show relatively light carbon isotopes and heavy oxygen isotopes (Table 1; Fig. 5a). The trace element contents of cements of this phase are higher than the early meteoric cements but less than the burial types. Figure 9 shows the reconstructed paragenetic sequence of the Pestehligh siliciclastic rocks. The carbon isotope of the cement of this stage is lighter than the early meteoric ones in the eastern section known as Sheikh. The reason for the lighter carbon ratios is related to the upper Khangiran black shale formation, which is different from two other stratigraphic sections. The higher organic matter contents of the upper shale formation in the Sheikh section caused the lighter carbon ratios.

Fig. 9. The paragenetic sequence of the Danian-age siliciclastic rocks of the western part of the Kopet-Dagh Basin. The diagenetic processes affected the sediments in three stages: Eodiagenesis, Mesodiagenesis and Telodiagenesis. These phases happened in three diagenetic environments: early meteoric; burial; and late meteoric after uplift.
6. Conclusions
Many processes affect deposits after their sedimentation. Those processes that may be physical, chemical or biological can create some diagenetic features in sedimentary rocks. Discriminating between these diagenetic features and relating them to the diagenetic environments help the reconstruction of the diagenetic para-sequence. Many macro-scale factors, such as changes in sea level, base level, climate, sedimentary environments, sedimentation rate, burial depth, etc., control the diagenetic history. The lower Palaeocene sequence was deposited along with significant changes in sedimentary environments in the Kopet-Dagh Basin, East Tethys, at the K/Pg boundary. Several diagenetic features have been identified, including physical and chemical compaction, cementation, alteration, dissolution, fractures and filling. According to petrographic and CL studies, the studied calcite cements are subdivided into four types: (1) dark luminescence around the grains (the grains rim, gravity pendant and meniscus cement types); (2) dark luminescent inter-grains (some of the blocky and poikilotopic cement types); (3) bright luminescent inter-grains (some of the blocky and poikilotopic cement types); and (4) dark luminescent filling fractures (some of the blocky cement types). Each of the four categorized cement types has different geochemical properties that have been recognized in this study. The geochemical data of this study revealed the precipitation of categorized cement types in several diagenetic environments, including the early meteoric, the low depth burial, the deeper burial, and the late meteoric after the uplift. The qualitative petrographic studies, semi-quantitative CL studies and quantitative ICP-MS analysis made it possible to evaluate the diagenetic history of the Danian interval in the western Kopet-Dagh Basin, East Tethys.
The study of the sedimentary environments of the Danian interval revealed considerable changes that occurred from the alluvial and fluvial systems flowing into marine sedimentary environments at the K/Pg boundary. The Danian siliciclastic formation is sandwiched between two marine carbonate sequences, i.e. the Maastrichtian carbonate and Thanetian carbonate successions. The transgression in the sea level in the late Palaeocene led to precipitation of many calcite cement types in the studied formation. Moreover, the upward-penetrating diagenetic fluids crossed the lower carbonate formation and facilitated the formation of calcite cements. Finally, the diagenetic sequence was reconstructed. In this study, a data source from the petrography, oxygen and carbon stable isotopes, CL and trace elements has been reproduced for considering the diagenetic history of the siliciclastic sequences.
Acknowledgements
The authors express their appreciation to the Deputies of Research and Technology of both Shahid Chamran University of Ahvaz and Ferdowsi University of Mashhad. The study of samples was carried out at Ferdowsi University of Mashhad Petrography Lab and at the Keck Paleoenvironmental and Environmental Stable Isotope Laboratory (KPESIL) at Kansas University. The authors also express their thanks to the Vice-Chancellor of Research of Shahid Chamran University of Ahvaz for financial support of this study with a research grant and grant number SCU.E99.110.