Impact statement
This study provides an overview of recent literature related to groundwater use in coastal urban areas, especially as an emergency resource during drought periods. The primary threats to coastal aquifers in the past, present and future are highlighted, as well as potential sustainable management solutions. A global comparison of risks and possible solutions regarding coastal aquifer management is imperative given increasing pressures on these systems related to socio-economic development, population growth and climate change. This is especially important in the context of droughts as these events affect both the quantity and quality of water available to densely populated coastal urban areas, which are vulnerable to resource degradation from both catchment and marine processes. Droughts coupled with groundwater abstraction also increase saltwater intrusion in aquifers with marine connectivity. As an area susceptible to severe droughts, a South African case study is used to illustrate the historical reliance of two coastal metropolitan municipalities on surface water resources and how groundwater abstraction has been used to supplement diminishing water supply. To our knowledge, this is the first study to review the literature available on the water crises experienced by both coastal municipalities. This assessment reveals priority management principles linked to sustainable water resource use during drought disasters in the coastal zone.
Introduction
Sustainable and reliable potable freshwater supply has been a limiting feature for human occupation of landscapes historically, especially at the coast (Rishworth et al., Reference Rishworth, Cawthra, Dodd and Perissinotto2020a; Rosinger, Reference Rosinger2021), and will restrict emergent economic development in the future in all regions (Garrick et al., Reference Garrick, Hall, Dobson, Damania, Grafton, Hope, Hepburn, Bark, Boltz, De Stefano, O’Donnell, Matthews and Money2017). Behavioural changes surrounding how water use is minimised across all sectors, how its lifecycle is optimised to restrict wastage and how alternative water supplies are utilised present solutions to managing water scarcity. The latter of which includes sources such as groundwater which have been used for millennia as dependable freshwater sources but are not always well-understood locally in terms of hydrological cycling.
Many groundwater reserves have been unsustainably managed and are at risk of depletion globally (Famiglietti and Ferguson, Reference Famiglietti and Ferguson2021; Jasechko and Perrone, Reference Jasechko and Perrone2021), although in other areas, such as Sub-Saharan Africa, groundwater resources are generally underdeveloped (Cobbing, Reference Cobbing2020). Coastal aquifers (groundwater resources situated along the coast and linked to or influenced by coastal or marine ecosystems) face additional threats driven by climate change and urbanisation compared to their inland counterparts (e.g., Boretti and Rosa, Reference Boretti and Rosa2019). These include sea-level rise (SLR), reduced recharge, storm surges (including hurricanes and cyclones), coastal flooding and erosion (Ferguson and Gleeson, Reference Ferguson and Gleeson2012; Barbier, Reference Barbier2015; Erostate et al., Reference Erostate, Huneau, Garel, Ghiotti, Vystavna, Garrido and Pasqualini2020; Chesnaux et al., Reference Chesnaux, Marion, Boumaiza, Richard and Walter2021). Coastal urbanisation is more concentrated compared to inland, and this is exacerbated by the increasing trend of coastal tourism, migration and population growth to coastal areas (Neumann et al., Reference Neumann, Vafeidis, Zimmermann and Nicholls2015; Parisi et al., Reference Parisi, Monno and Fidelibus2018). As a result, increased anthropogenic pressures at the coast may accelerate pollution, over-abstraction and compaction of coastal aquifers causing water quality degradation, seawater intrusion and land subsidence (e.g., Ferguson and Gleeson, Reference Ferguson and Gleeson2012; Chang et al., Reference Chang, Zheng, Zheng, Zhang, Sun and Walther2019; Han and Currell, Reference Han and Currell2022). Thus, the urbanisation of continental coastlines and islands coupled with increasing threats of climate change, coastal vulnerability and associated extreme events such as droughts adds pressure to an already constrained system of freshwater supply (Boretti and Rosa, Reference Boretti and Rosa2019; see Figure 1). Therefore management and supply of alternative resources such as groundwater are becoming more crucial under these circumstances along coastlines.
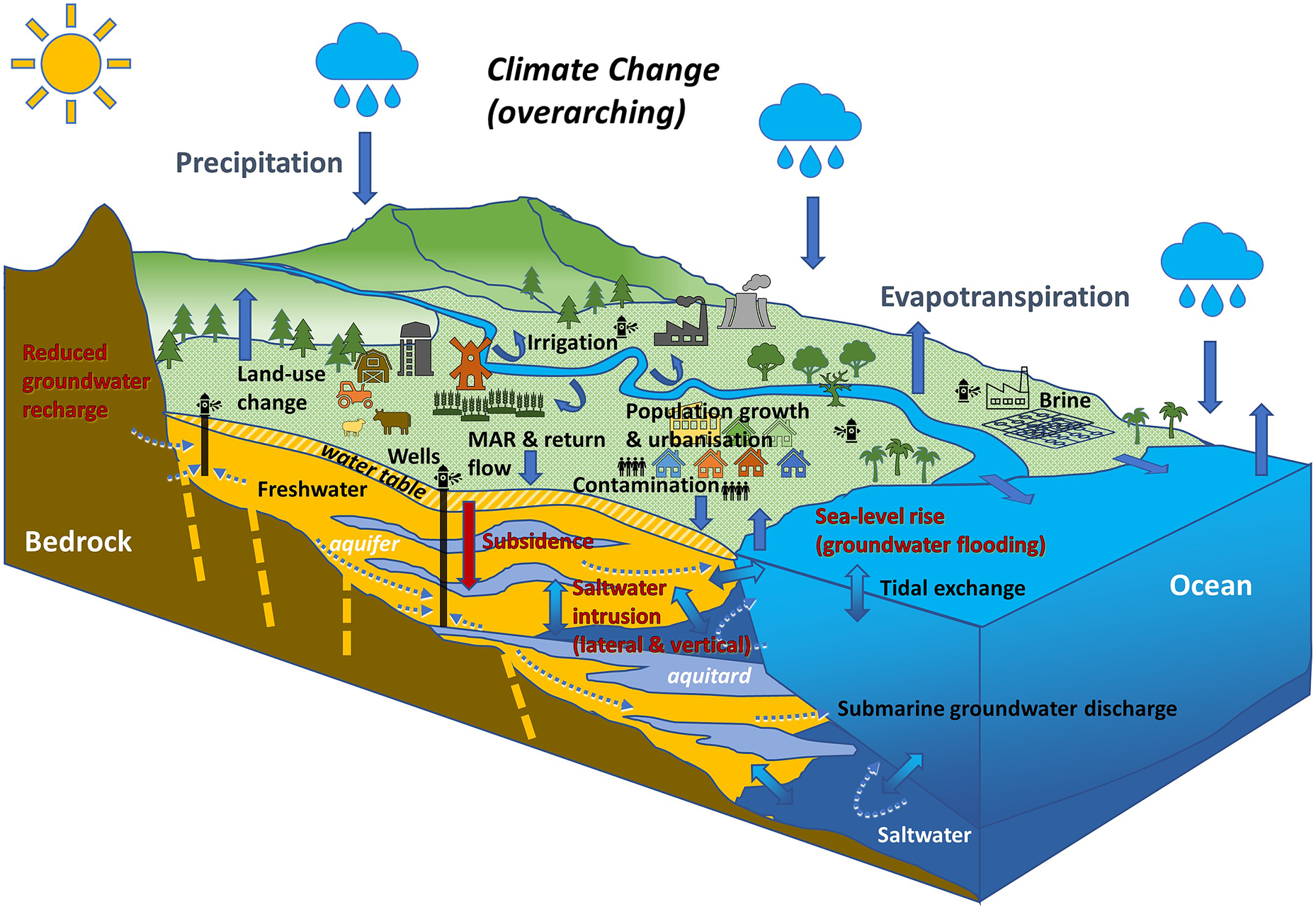
Figure 1. Schematic portraying the issues, opportunities and dynamics related to ground water and its management at the coast in light of future climate change, sustainable development and drought threats. This demonstrates key issues relevant to coastal urban reliance on groundwater. Based on and adapted from graphical abstract of Han and Currell (Reference Han and Currell2022).
Droughts may be classified according to their origin (e.g., meteorological drought) or effect (e.g., agricultural, hydrological). A prolonged meteorological drought, which is caused by below-average precipitation for a given time, may develop into an agricultural or hydrological drought. An agricultural drought is characterised by deficits in soil moisture and often has negative effects on crop yields. On the other hand, a hydrological drought can be sub-categorised (e.g., groundwater drought) and refers to reductions in water levels and reservoir storage (e.g., Schreiner-McGraw and Ajami, Reference Schreiner-McGraw and Ajami2021). In this assessment, “drought” generally refers to a persistent meteorological drought, which has manifested as a hydrological drought.
This study evaluates and reviews recent literature discussing the drivers, pressures, states and impacts influencing coastal aquifer functioning and potential management responses, especially in a drought context.
Perspective
Groundwater use and risks in the coastal zone
As surface water resources become more limited and progressively degraded, coastal groundwater abstraction has increased markedly and is expected to increase even further under predicted drier conditions in the future with more frequent, long-lasting and intense droughts anticipated (e.g., Parisi et al., Reference Parisi, Monno and Fidelibus2018; Erostate et al., Reference Erostate, Huneau, Garel, Ghiotti, Vystavna, Garrido and Pasqualini2020). These climatic changes (higher temperatures, evapotranspiration and decreased precipitation) will further affect groundwater resources through decreased recharge (e.g., Stigter et al., Reference Stigter, Nunes, Pisani, Fakir, Hugman, Li, Tomé, Ribeiro, Samper, Oliveira, Monteiro, Silva, Tavares, Shapouri, Cancela da Fonseca and El Himer2014; Unsal et al., Reference Unsal, Yagbasan and Yazicigil2014). The consequent impacts of declines in precipitation and groundwater recharge are expected to be especially pronounced in arid and semi-arid regions and coastal regions, such as small islands, that rely exclusively on rainwater harvesting (RWH) and groundwater resources for water supply (e.g., Stigter et al., Reference Stigter, Nunes, Pisani, Fakir, Hugman, Li, Tomé, Ribeiro, Samper, Oliveira, Monteiro, Silva, Tavares, Shapouri, Cancela da Fonseca and El Himer2014; Unsal et al., Reference Unsal, Yagbasan and Yazicigil2014; Kopsiaftis et al., Reference Kopsiaftis, Tigkas, Christelis and Vangelis2017). Urbanisation may further decrease groundwater recharge by the replacement of natural permeable landscapes with hard, impermeable surfaces such as roads. These urban surfaces linearly direct surface runoff to lentic waterways more effectively and therefore reduce infiltration opportunities (Han et al., Reference Han, Currell, Cao and Hall2017; Lorenzo-Lacruz et al., Reference Lorenzo-Lacruz, Garcia and Morán-Tejeda2017; Minnig et al., Reference Minnig, Moeck, Radny and Schirmer2018; Frommen et al., Reference Frommen, Groeschke, Nölscher, Koeniger and Schneider2021). As such, this will contribute to the coastal squeeze of coastal urban aquifers since these systems are wedged between developed urban areas and rising seas (Vitousek et al., Reference Vitousek, Barnard and Limber2017).
Coastal threats and changes (e.g., SLR, storm surges, coastal erosion and land subsidence) may occur simultaneously and/or have knock-on effects leading to the occurrence or acceleration of other pressures, usually intensified through urbanisation and development. The onset of coastal pressures may, however, also be triggered inland such as in the case of coastal erosion caused by dam construction and the resultant changes in sediment source and coastal sediment dynamics (e.g., Huang and Jin, Reference Huang and Jin2018 and references therein; Hzami et al., Reference Hzami, Heggy, Amrouni, Mahé, Maanan and Abdeljaouad2021). Coastal erosion may accelerate land losses beyond those caused by SLR, or erosion may amplify the effects of SLR, causing groundwater salinisation and reductions in groundwater recharge (Baharuddin et al., Reference Baharuddin, Masirin, Hazreek, Azman and Madun2018; Chesnaux et al., Reference Chesnaux, Marion, Boumaiza, Richard and Walter2021). This is of further concern since the decrease in groundwater recharge may be a more important issue than SLR in flat low-lying coastal areas (Parisi et al., Reference Parisi, Monno and Fidelibus2018). Diminished groundwater discharge levels due to declines in groundwater levels, whether induced by abstraction or reductions in recharge, affect the rest of the hydrologic system through decreased river baseflows, lake and spring levels and ultimately result in landscape desiccation (de Graaf et al., Reference De Graaf, Gleeson, (Rens) Van Beek, Sutanudjaja and Bierkens2019). Furthermore, seaward erosion during storm surges of coastal barriers (e.g., dunes), could result in breaching events that may subsequently affect coastal aquifers kilometres inland through vertical saltwater infiltration, with the delayed recovery of the aquifers to a freshwater state lasting longer than the storm event (Giambastiani et al., Reference Giambastiani, Colombani, Greggio, Antonellini and Mastrocicco2017; Elsayed et al., Reference Elsayed, Oumeraci and Goseberg2018).
Many coastal hazards, whether caused by anthropogenic or climatic drivers, ultimately lead to coastal inundation (permanent) or flooding (temporary) (sensu Flick et al., Reference Flick, Chadwick and Harper2012) (hereafter collectively referred to as submergence). Three types may be recognised, namely: 1) marine submergence (surface submergence with marine water through, e.g., tidal flooding, SLR, storm drain backflow); 2) groundwater submergence (rise in groundwater levels as groundwater is displaced in response to, e.g., SLR or wastewater network leaks) and 3) precipitation-driven submergence (through large rainfall events) (Han et al., Reference Han, Currell, Cao and Hall2017; Minnig et al., Reference Minnig, Moeck, Radny and Schirmer2018; Habel et al., Reference Habel, Fletcher, Anderson and Thompson2020; Rahimi et al., Reference Rahimi, Tavakol-Davani, Graves, Gomez and Valipour2020; Su et al., Reference Su, Liu, Beheshti and Prigiobbe2020; Frommen et al., Reference Frommen, Groeschke, Nölscher, Koeniger and Schneider2021). Furthermore, these submergence types may compound each other, which can result in “snowballing” impacts compared to isolated occurrences (Habel et al., Reference Habel, Fletcher, Anderson and Thompson2020; Rahimi et al., Reference Rahimi, Tavakol-Davani, Graves, Gomez and Valipour2020). For example, modelled scenarios of the Oakland Flatlands, a low-lying coastal area of California, USA indicate that SLR alone will not pose significant threats to urban infrastructure, but coupled with groundwater submergence more than 310 ha will be negatively affected (Rahimi et al., Reference Rahimi, Tavakol-Davani, Graves, Gomez and Valipour2020). Excluding the damage to coastal infrastructure and threats to human life, submergence is usually accompanied by groundwater salinisation through seawater intrusion and pollution of aquifers (Habel et al., Reference Habel, Fletcher, Anderson and Thompson2020; Rakib et al., Reference Rakib, Sasaki, Matsuda, Quraishi, Mahmud, Bodrud-Doza, Atique Ullah, Fatema, Newaz and Bhuiyan2020), which exacerbates the degradation of aquifers already under pressure from over-abstraction and droughts by decreasing potability (e.g., in California, USA – Rahimi et al., Reference Rahimi, Tavakol-Davani, Graves, Gomez and Valipour2020).
Despite being identified as a hazard to drinking water supply systems more than a century ago (Michael et al., Reference Michael, Post, Wilson and Werner2017), seawater intrusion and resultant groundwater salinisation are still the primary threat related to coastal groundwater abstraction (Chang et al., Reference Chang, Zheng, Zheng, Zhang, Sun and Walther2019). The source of salinity, however, may be natural (seawater trapped in pores from earlier sea level transgressions and dissolution of evaporite minerals, e.g., halite and gypsum) or anthropogenic (related to over-extraction and agricultural return flow) (Nogueira et al., Reference Nogueira, Stigter, Zhou, Mussa and Juizo2019).
Seawater intrusion triggered by over-abstraction from coastal aquifers can result in both landward (lateral) and vertical (upconing) intrusion (Parisi et al., Reference Parisi, Monno and Fidelibus2018). Coastal aquifers are increasingly at risk from bidirectional contamination as shorelines migrate landward with SLR and seawater intrusion and with pollution pressure from land sources – especially agricultural and industrial (Michael et al., Reference Michael, Post, Wilson and Werner2017; summarised in Figures 1 and 2). Not only does this leave groundwater resource unsuitable for human use but may also have dire effects on coastal groundwater-dependent ecosystems (GDEs) such as estuaries, lagoons, microbialite ecosystems and coral reefs (Michael et al., Reference Michael, Post, Wilson and Werner2017; Erostate et al., Reference Erostate, Huneau, Garel, Ghiotti, Vystavna, Garrido and Pasqualini2020; Rishworth et al., Reference Rishworth, Dodd, Perissinotto, Bornman, Adams, Anderson, Cawthra, Dorrington, Du Toit, Edworthy, Gibb, Human, Isemonger, Lemley, Miranda, Peer, Raw, Smith, Steyn, Strydom, Teske and Welman2020b). These GDEs are also at risk of state changes or even localised extinction as groundwater levels are reduced or depleted and surface-groundwater connections are disturbed. This is especially relevant in arid or semi-arid coastal regions with a Mediterranean climate, such as those of the Mediterranean basin and the southwestern coasts of Australia, Chile, California (USA) and South Africa (Erostate et al., Reference Erostate, Huneau, Garel, Ghiotti, Vystavna, Garrido and Pasqualini2020). For example, salinity increases in the microbialite-bearing Lake Clifton, Western Australia has been observed since the 1990s. This is attributed to hydrological changes driven by increased evaporation and decreased freshwater recharge to Lake Clifton due to lower rainfall, groundwater abstraction in the catchment areas and the construction of the Dawesville Channel (Forbes and Vogwill, Reference Forbes and Vogwill2016; Warden et al., Reference Warden, Coshell, Rosen, Breecker, Ruthrof and Omelon2019). The higher salinity, in addition to higher nutrient loads, is thought to have contributed to a change in the microbial communities of the Lake, although this does not necessarily translate to unfavourable conditions for microbialite formation (Gleeson et al., Reference Gleeson, Wacey, Waite, O’Donnell and Kilburn2016; Warden et al., Reference Warden, Coshell, Rosen, Breecker, Ruthrof and Omelon2019). The effects of coastal water crises associated with groundwater salinisation globally may be summarised as conflict of uses between different water users, wetland degradation, loss of crops, soil salinisation, aridity, desertification and health issues (Parisi et al., Reference Parisi, Monno and Fidelibus2018).
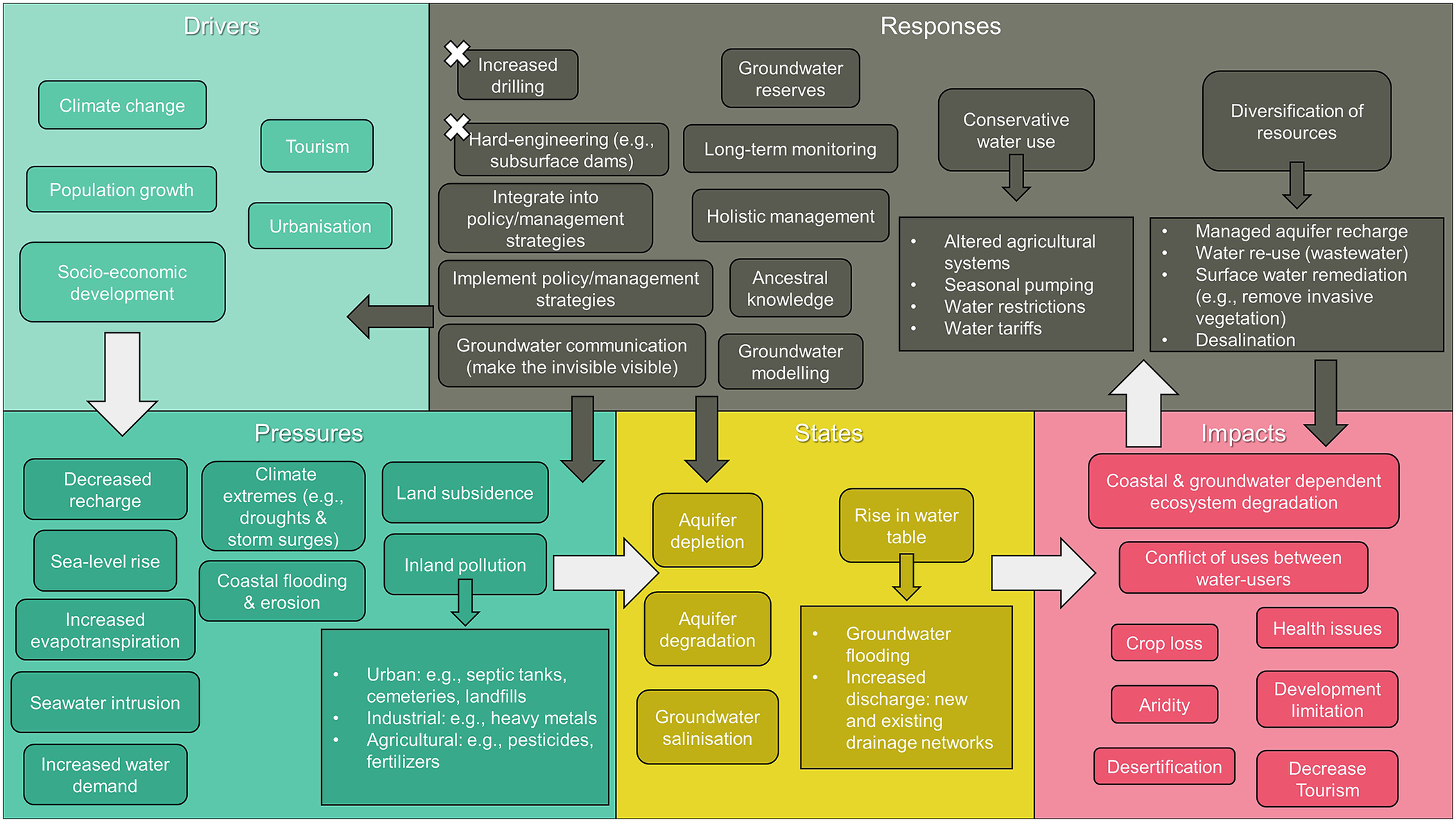
Figure 2. Driver-Pressure-State-Impact-Response (DPSIR) framework regarding groundwater use in coastal urban areas. Responses with a cross indicate there are large negative implications reported with the use thereof.
Furthermore, an emerging issue related to coastal groundwater use is that of land subsidence and increased rates of relative SLR. Natural land subsidence of unconsolidated coastal sediments may be accelerated by groundwater abstraction and urban development (see below) and when combined with SLR and storm surges increase the risk of seawater intrusion into aquifers (Huang and Jin, Reference Huang and Jin2018; Shirzaei et al., Reference Shirzaei, Freymueller, Törnqvist, Galloway, Dura and Minderhoud2021). This will likely be exacerbated by drought conditions and climate change. For example, model predictions underestimated land subsidence in Yuanchang, Taiwan for the 2011–2012 period due to increased groundwater pumping and reduced recharge during a dry spell (Shirzaei et al., Reference Shirzaei, Freymueller, Törnqvist, Galloway, Dura and Minderhoud2021). Relative SLR may pose a further risk to coastal communities through groundwater flooding as unconfined coastal aquifer levels will increase in response to sea level changes. It is anticipated that water tables will either rise the same amount as sea levels, where the additional groundwater can be accommodated, or that the seawater intrusion will displace fresh groundwater, which will discharge through original or new drainage systems (Befus et al., Reference Befus, Barnard, Hoover, Hart and Voss2020). For instance, coastal cities in Indonesia such as Semarang and the Indonesian capital, Jakarta, are already experiencing the impacts of land subsidence, coastal flooding during high tides and seawater intrusion into aquifers (Abidin et al., Reference Abidin, Andreas, Gumilar, Sidiq and Gamal2015). This is partly due to the demand for clean water outstripping surface water supply and consequently the over-abstraction of groundwater to make up the water supply deficit. Furthermore, urban development results in the consolidation of sediments, increased surface runoff and decreased groundwater recharge during rain events (Abidin et al., Reference Abidin, Andreas, Gumilar, Sidiq and Gamal2015; Pramono, Reference Pramono2021; Taftazani et al., Reference Taftazani, Kazama and Takizawa2022). As a result, more than 80% of groundwater samples from monitoring wells in northern Jakarta’s groundwater basins exceed the recommended limits of salinity for human consumption (Taftazani et al., Reference Taftazani, Kazama and Takizawa2022) and aquifers of entire small islands are brackish or saline during dry seasons (Cahyadi, Reference Cahyadi2018).
Water quality degradation through anthropogenic and geogenic pollution linked to increased water demand will further decrease both surface and groundwater resources available for use at the coast (e.g., Han and Currell, Reference Han and Currell2022). Urban aquifers are often associated with pollution by emerging contaminants (e.g., pharmaceuticals and personal care products) and nutrient loading through aqua- and agricultural activities, septic tanks, cemeteries and landfills (e.g., Lapworth et al., Reference Lapworth, Nkhuwa, Okotto-Okotto, Pedley, Stuart, Tijani and Wright2017; Boretti and Rosa, Reference Boretti and Rosa2019; Burri et al., Reference Burri, Weatherl, Moeck and Schirmer2019; Preziosi et al., Reference Preziosi, Frollini, Zoppini, Ghergo, Melita, Parrone, Rossi and Amalfitano2019; Han and Currell, Reference Han and Currell2022). Slow-moving aquifers and groundwater-fed rivers can release persistent organic pollutants, such as anthropogenic per- and polyfluoroalkyl substances (PFAS), into marine environments long after its initial release into the upstream environment (Sunderland et al., Reference Sunderland, Hu, Dassuncao, Tokranov, Wagner and Allen2019; Zhang et al., Reference Zhang, Lohmann and Sunderland2019a; Ruyle et al., Reference Ruyle, Pickard, Leblanc, Tokranov, Thackray, Hu, Vecitis and Sunderland2021). Coastal point sources include airports and ports (Li et al., Reference Li, Zhang, Gibbes, Wang and Lockington2022), while wastewater treatment works, landfills and urban runoff also contribute to the transportation of PFAS (e.g., Hepburn et al., Reference Hepburn, Madden, Szabo, Coggan, Clarke and Currell2019; Cui et al., Reference Cui, Li and Quinete2020). The residence time of certain PFAS species may be further lengthened by adsorption driven by tidal and salinity effects in the coastal zone resulting in bioaccumulation in benthic organisms. In fact, seafood is considered the primary human dietary exposure pathway to PFAS compounds (Sunderland et al., Reference Sunderland, Hu, Dassuncao, Tokranov, Wagner and Allen2019; Zhang et al., Reference Zhang, Lohmann and Sunderland2019a). However, PFAS adsorption in coastal sandy aquifers may act as a natural attenuation process (Li et al., Reference Li, Zhang, Gibbes, Wang and Lockington2022). These pathways are therefore not fully understood but present an emerging research priority and likely threat to coastal aquifers.
Management of water crises and groundwater use in the coastal zone
When facing a water scarcity crisis, there are two management strategies that can be employed, namely, supply management (i.e., increase the available resources) and demand management (i.e., decrease water use) (Lam et al., Reference Lam, Lant, O’Brien and Kenway2016). Although droughts occur naturally as a part of the hydrological cycle, their frequency and intensity are increasing under climate change scenarios. Emergency procedures during droughts often mean that more wells are drilled, which may accelerate aquifer depletion (Petersen-Perlman et al., Reference Petersen-Perlman, Aguilar-Barajas and Megdal2022) and are therefore likely not a sustainable solution (Figure 2). Water supply management in urbanised coastal areas during droughts cannot rely on a “wait ‘til it rains’” approach (Parisi et al., Reference Parisi, Monno and Fidelibus2018). Rather, socio-ecological and collaborative strategic management of water supply is required to effectively manage water resources and prevent aquifer salinisation (Parisi et al., Reference Parisi, Monno and Fidelibus2018).
Some problems related to drought and groundwater management are caused by governance misalignments (Petersen-Perlman et al., Reference Petersen-Perlman, Aguilar-Barajas and Megdal2022). For example, the Salento aquifer in Italy is experiencing groundwater salinisation and despite droughts in the area, salinity effects are not considered in groundwater management practices and no systematic monitoring takes place (Parisi et al., Reference Parisi, Monno and Fidelibus2018). Furthermore, although the national legislation of selected water-scarce and drought-prone countries protects GDEs (e.g., Australia) or advocates for environmental requirements (e.g., South Africa) (Rohde et al., Reference Rohde, Froend and Howard2017; Erostate et al., Reference Erostate, Huneau, Garel, Ghiotti, Vystavna, Garrido and Pasqualini2020), monitoring of actual regional groundwater abstraction is often lacking or management regulations are not met (Bekesi et al., Reference Bekesi, McGuire and Moiler2009; Erostate et al., Reference Erostate, Huneau, Garel, Ghiotti, Vystavna, Garrido and Pasqualini2020; Kent et al., Reference Kent, Pandey, Turner, Dickinson and Jamieson2020; Robertson, Reference Robertson2020). That being said, in Australia progress in GDE management has been achieved through comprehensive adaptive management frameworks that are informed by legislation as well as scientific and technological advancements coupled with regional datasets (Rohde et al., Reference Rohde, Froend and Howard2017).
Other accounts of successful groundwater management strategies and implementation of policy during droughts in coastal urban areas include the employment of managed aquifer recharge (MAR). The main applications of MAR are to increase water storage and provide a water resource more resilient to climate change, while water quality is also often improved by pollutant attenuation during infiltration (e.g., Kazakis, Reference Kazakis2018; Dillon et al., Reference Dillon, Escalante, Megdal and Massmann2020). This strategy of MAR is often implemented in regions with seasonal precipitation cycles to mitigate surface-water shortages during the dry months, such as in India (Glendenning and Vervoort, Reference Glendenning and Vervoort2011). A small-scale example, stemming from ancestral knowledge, is that of coastal Ecuador using constructed “tapes” or artisanal dikes to increase groundwater storage during periods of water scarcity (Carrión et al., Reference Carrión, Herrera, Briones, Sánchez and Limón2018). Furthermore, MAR has been widely applied to prevent seawater intrusion into coastal aquifers by maintaining groundwater levels and driving the saltwater wedge seaward or decreasing aquifer salinisation through blending with lower salinity surface water, such as treated wastewater (e.g., Masciopinto, Reference Masciopinto2013; Bachtouli and Comte, Reference Bachtouli and Comte2019; Alam et al., Reference Alam, Borthakur, Ravi, Gebremichael and Mohanty2021). For example, in Jakarta, Indonesia the effects of land subsidence and seawater intrusion are combatted by increasing groundwater recharge through the construction of water traps and drainage reservoirs and increasing vegetation cover (Pramono, Reference Pramono2021). However, if not managed properly MAR can introduce pollutants and pathogens into groundwater resources (e.g., Raicy et al., Reference Raicy, Parimala Renganayaki, Brindha, Elango, Elango, Goyal and Thomas2012; Casanova et al., Reference Casanova, Devau, Pettenati, Jakeman, Barreteau, Hunt, Rinaudo and Ross2016; Alam et al., Reference Alam, Borthakur, Ravi, Gebremichael and Mohanty2021). For example, a review of studies using stormwater for MAR revealed that dissolved organic carbon, selected metals and E. coli are effectively removed from recharge water during the infiltration process, but that trace organics and Enterococcus bacteria were still present (Alam et al., Reference Alam, Borthakur, Ravi, Gebremichael and Mohanty2021).
Policy-driven groundwater demand management has been successful in the drought-prone Central Coast region of California (USA) where groundwater supplies 90% of the drinking water (Langridge and Van Schmidt, Reference Langridge and Van Schmidt2020). In response to the severe drought of 2012–2016 experienced in the area, a Sustainable Groundwater Management Act (SGMA) was passed to mitigate groundwater storage loss (Langridge and Van Schmidt, Reference Langridge and Van Schmidt2020). Although the SGMA does not take into account groundwater losses prior to the passing of the Act, mitigation strategies related to groundwater include using flood flows rather than groundwater for MAR and irrigation purposes and developing local groundwater drought reserves to avoid irreversible groundwater losses during drought periods. The latter is achieved through, for example, using agricultural return flow for recharge, of which a portion is then available to farmers for irrigation during droughts (Langridge and Van Schmidt, Reference Langridge and Van Schmidt2020).
Another example of a coastal urban area that is prone to severe droughts and is predicted to be impacted by increased temperatures and decreases in surface water resources is that of Perth, Australia (population of 1.6 million) (Serrao-Neumann et al., Reference Serrao-Neumann, Renouf, Kenway and Choy2017). During the Millennium Drought (1996–2010) in southern Australia, it was proposed that the water supply in Perth be further augmented from the West Yarragadee aquifer, however, desalination of seawater was implemented instead (Lam et al., Reference Lam, Lant, O’Brien and Kenway2016). During 2013–2014 about 42% of Perth’s water supply was from groundwater, while desalination provided 39% of supply. More recently, MAR is also being considered to supplement water supply. Interestingly, the urban water use in Perth remained lower post-drought than that of pre-drought use, despite increased urban population, suggesting the value of social awareness and behavioural shifts in managing water scarcity. However, the energy use of the water supply system has doubled (Lam et al., Reference Lam, Lant, O’Brien and Kenway2016).
Several other interventions have been successful in decreasing/managing pollution and salinisation risks such as alternating/seasonal pumping regimes, pumping limits and hard-engineering approaches (e.g., subsurface dams, cut-off walls, semi-pervious subsurface barriers; Chang et al., Reference Chang, Zheng, Zheng, Zhang, Sun and Walther2019), rehabilitation of dune systems and the removal of alien trees (Giambastiani et al., Reference Giambastiani, Colombani, Greggio, Antonellini and Mastrocicco2017), and identifying sources of groundwater-borne pollutants (Michael et al., Reference Michael, Post, Wilson and Werner2017). Some of these interventions are not without adverse effects. For example, sub-surface dams can result in the accumulation of landward pollutants behind the barrier, increase inland soil salinisation and prevent groundwater discharge to the coastal zone (Chang et al., Reference Chang, Zheng, Zheng, Zhang, Sun and Walther2019).
Depending on the scale of a drought event, groundwater demand may be effectively managed by a range of options in the short to medium term (Parisi et al., Reference Parisi, Monno and Fidelibus2018), including alternative sources such as RWH, the recycling of water and desalinisation. RWH is perhaps the most universally applied method (Wurthmann, Reference Wurthmann2019) and can be used at a household (e.g., tank) or catchment (impoundment or for groundwater recharge) scale. For example, in Bangladesh, rainfall in coastal areas is higher than inland and RWH in urban areas is considered a suitable resource for drinking and residential purposes (Islam et al., Reference Islam, Afrin, Redwan and Rahman2015). Conversely in Florida USA, RWH used for residential outdoor irrigation could meet more than half the future water demand under a high population growth scenario and may perform better than other alternative sources such as reclaimed water (Wurthmann, Reference Wurthmann2019). The long-term reliability of RWH is, however, a concern since harvesting units are usually designed for current precipitation patterns and future rainfall variability is imminent due to climate change (Islam et al., Reference Islam, Afrin, Redwan and Rahman2015). In addition, at a catchment scale RWH through impoundments could reduce streamflow (Glendenning and Vervoort, Reference Glendenning and Vervoort2011).
The recycling of storm- and/or wastewater for irrigation purposes (Lavrnić et al., Reference Lavrnić, Zapater-Pereyra and Mancini2017), MAR (Alam et al., Reference Alam, Borthakur, Ravi, Gebremichael and Mohanty2021 and references therein) and even potable use (Lee and Tan, Reference Lee and Tan2016) is a climate-resilient water source. However, integration into water supply systems is not as readily accepted due to public perceptions as well as contamination and health risks (Gu et al., Reference Gu, Chen, Pody, Cheng, Zheng and Zhang2015; Garcia-Cuerva et al., Reference Garcia-Cuerva, Berglund and Binder2016). The use of reclaimed water for irrigation is considered a suitable option since the water already contains nutrients and therefore reduced fertiliser application is required (Lavrnić et al., Reference Lavrnić, Zapater-Pereyra and Mancini2017). Other innovations such as altered agricultural water supply systems (e.g., drip irrigation) or drought-resistant crops will be essential for future agricultural practices (Oude Essink, Reference Oude Essink2001; Lavrnić et al., Reference Lavrnić, Zapater-Pereyra and Mancini2017). Finally, desalination of brackish and seawater is a viable option for coastal urban water supply but is often expensive, energy-intensive and can have negative impacts on coastal biodiversity through brine by-products (Singh et al., Reference Singh, Jain, Sukhwani and Shaw2021 and see below case study).
Other management solutions include aquifer protection, through for example financial incentives to reduce groundwater abstraction and associated negative impacts. For instance, groundwater abstraction tax has been implemented in Jakarta and it was suggested that groundwater abstraction is spatially controlled (e.g., limiting abstraction to volcanic lithologies rather than subsidence-prone sedimentary lithologies) (Taftazani et al., Reference Taftazani, Kazama and Takizawa2022).
Drought management successes have also been achieved through adjusted water tariffs, integrated management of water resources, conservative water-use practices (residents and tourists), as well as the re-definition of management and policy systems (Parisi et al., Reference Parisi, Monno and Fidelibus2018; Singh et al., Reference Singh, Jain, Sukhwani and Shaw2021 and the references therein). Furthermore, it is crucial that groundwater modelling of water supply systems is included into drought policies and long-term planning. These models must take into account the present status of the aquifer(s) and be informed by baseline monitoring assessments and extraction rate information. However, a lack of monitoring and unmetered groundwater use poses a major challenge for groundwater management modelling (Hunter et al., Reference Hunter, Brooking, Reading and Vink2016; Keir et al., Reference Keir, Bulovic and McIntyre2019; Rochford et al., Reference Rochford, Ordens, Bulovic and McIntyre2022). Recent innovations in groundwater modelling have meant that hydrological deficits can be distinguished from the effects of over-abstraction and that aquifer responses can be linked to decision-making (Petersen-Perlman et al., Reference Petersen-Perlman, Aguilar-Barajas and Megdal2022). In addition, advances have been made in the understanding of aquifer vulnerability in terms of both volume and quality during droughts related to pumping and pollution. However, the effects of augmentation strategies such as MAR need to be incorporated more sufficiently (Petersen-Perlman et al., Reference Petersen-Perlman, Aguilar-Barajas and Megdal2022). Finally, it is essential that issues related to groundwater use during droughts are effectively communicated and that local stakeholders participate in managing groundwater resources (Petersen-Perlman et al., Reference Petersen-Perlman, Aguilar-Barajas and Megdal2022).
Both land and water management need to be proactive rather than reactive to ensure that coastal groundwater resources are managed adequately for the economy, health and environment (Michael et al., Reference Michael, Post, Wilson and Werner2017). Integrated coastal groundwater management needs to take into account geological, hydrological and biogeochemical complexity whilst also accounting for human complexity in terms of economic, cultural and decision-making factors (Michael et al., Reference Michael, Post, Wilson and Werner2017). Thus, environmental and hydrological connections between cities and the surrounding areas, future uncertainties related to water availability, and a holistic landscape view is required in planning (Serrao-Neumann et al., Reference Serrao-Neumann, Renouf, Kenway and Choy2017; see Figure 2).
Case study: A comparison of two South African metropolitan municipalities
Several South African urban areas are struggling to meet water supply requirements due to growing demand, decreased rainfall and poor governance (e.g., Olivier and Xu, Reference Olivier and Xu2019; Mahlalela et al., Reference Mahlalela, Blamey, Hart and Reason2020; Pamla et al., Reference Pamla, Thondhlana and Ruwanza2021). Two large, coastal metropolitan municipalities, the City of Cape Town (CoCT) in the Western Cape province and the Nelson Mandela Bay Metropolitan Municipality (NMBM) in the Eastern Cape province (see Figure 3), have faced or are facing “Day Zero” where the water supply systems are expected to fail (e.g., Pamla et al., Reference Pamla, Thondhlana and Ruwanza2021).
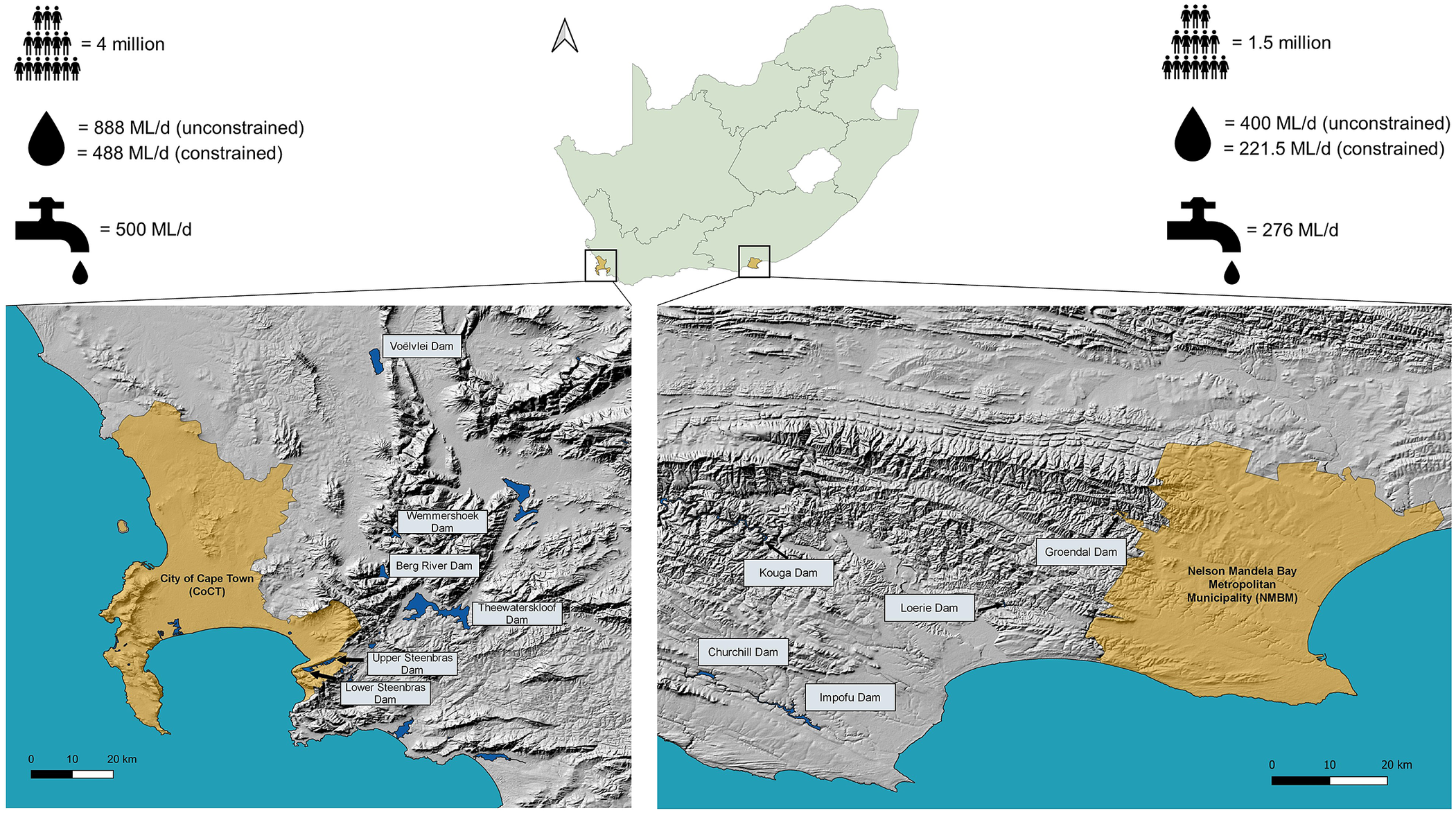
Figure 3. Locations of City of Cape Town and Nelson Mandela Bay Metropolitan Municipalities and their major supply dams. Urban population size, unconstrained and constrained surface water supplies available to the municipalities and water use during the drought are also indicated.
Although all droughts do not necessarily evolve into water crises (Wolski, Reference Wolski2018), both municipalities rely primarily on rain-fed surface water resources (e.g., Luker and Harris, Reference Luker and Harris2019; NMBM, 2022), which makes them vulnerable to reduction in precipitation in the short to medium term (see Table 1). This is especially pertinent given the coastal setting of these municipalities and the additional climate change challenges that they face (e.g., SLR, coastal flooding and erosion) (e.g., Bornman et al., Reference Bornman, Schmidt, Adams, Mfikili, Farre and Smit2016; Williams and Lück-Vogel, Reference Williams and Lück-Vogel2020; Dube et al., Reference Dube, Nhamo and Chikodzi2022). Consequently, groundwater reserves are viable alternative freshwater supplies for the metros (e.g., Miller et al., Reference Miller, Dunford, Swana, Palcsu, Butler and Clarke2017; NMBM, 2022) to mitigate the unsustainable water supply scenario presented by anticipated climate change effects and population growth.
Table 1. Direct comparison of the case-study scenarios which highlight the relevant regional context and management responses to local drought-related water scarcity
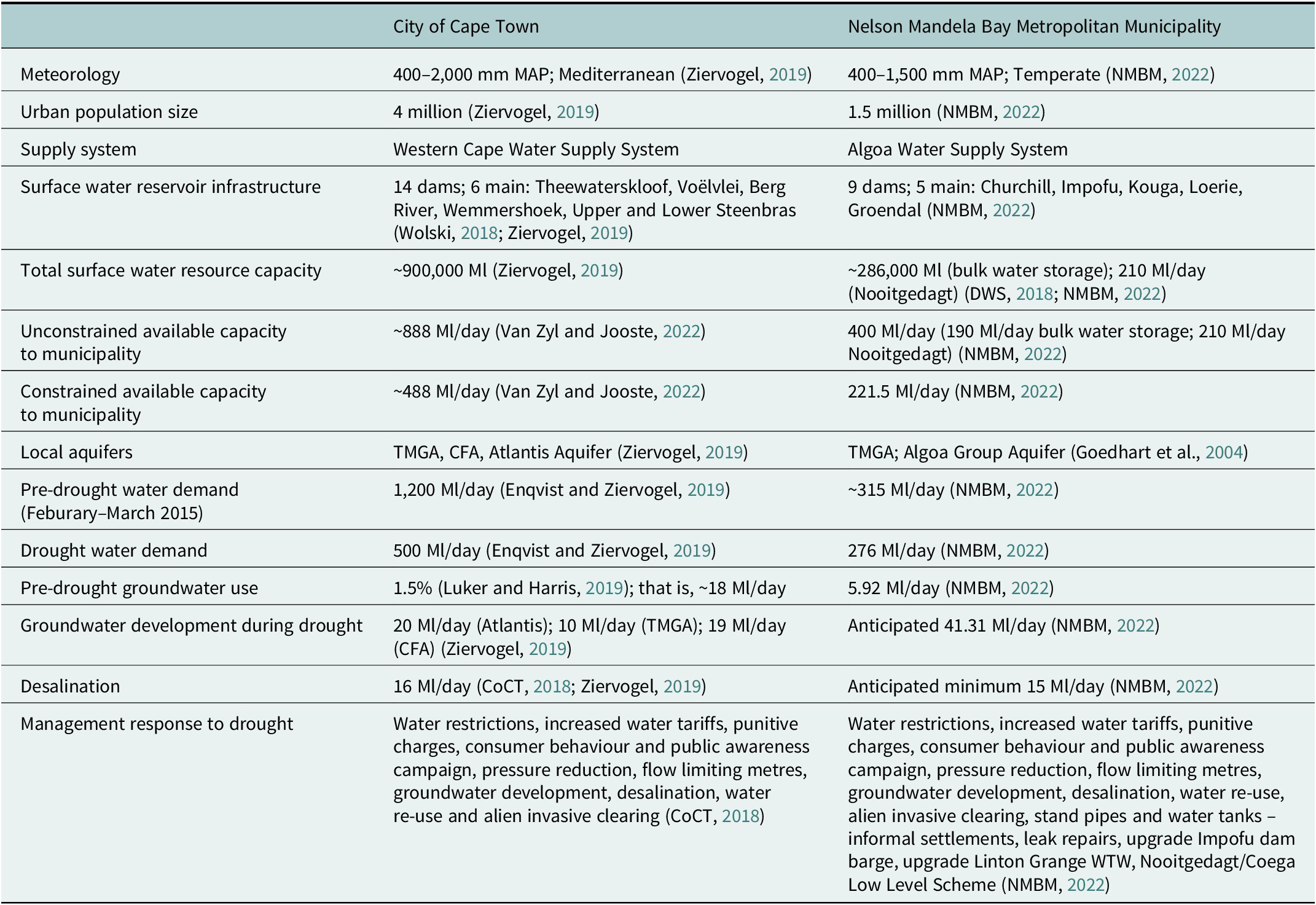
Note: Historical usage of groundwater and potential mitigation and alleviating opportunities provided by groundwater are also given.
Large-scale, systematic development of groundwater resources for water supply in the CoCT and NMBM was generally limited to times of drought (e.g., 1982–1994 drought in NMBM; Lomberg et al., Reference Lomberg, Rosewarne, Raymer and Devey1996) or where no other water supply options were available (e.g., rural/small town settings) as groundwater was/is predominantly considered an emergency resource (Cobbing et al., Reference Cobbing, Eales, Gibson, Lenkoe and Cobbing2015; Luker and Harris, Reference Luker and Harris2019). Furthermore, groundwater resource development for private use was likely driven by socio-economic factors rather than hydrogeological features (Lomberg et al., Reference Lomberg, Rosewarne, Raymer and Devey1996). This is despite both municipalities having access to suitable aquifers for potential development or water supply diversification (e.g., Pietersen and Parsons, Reference Pietersen and Parsons2002). The recent interest in large-scale abstraction from regional and local aquifers, therefore, provides the municipalities with a comparatively “clean slate” and an opportunity to learn from management successes and failures of other coastal urban areas reliant on groundwater (as discussed above). This case study highlights the groundwater resources available for development in the CoCT and NMBM, municipal groundwater schemes already in place, anticipated/experienced climate change threats and management priorities for sustainable groundwater development in these coastal cities.
For both the CoCT and NMBM an important regional aquifer is that of the Palaeozoic Table Mountain Group aquifer (TMGA), which predominantly consists of quartzitic sandstones that generally yield good quality water (e.g., Rosewarne, Reference Rosewarne, Pietersen and Parsons2002; Figure 4). Water is stored in pore space formed by secondary deformation associated with faults, bedding planes and joints (e.g., Pietersen and Parsons, Reference Pietersen and Parsons2002). Shallow, unconfined Cenozoic intergranular aquifers, where water is stored in the primary pore space between interstices of sand grains of fluvial, marine and aeolian deposits, also occur in both municipalities (e.g., Saayman and Adams, Reference Saayman and Adams2002; Goedhart et al., Reference Goedhart, Small and Hulley2004; Jovanovic et al., Reference Jovanovic, Bugan, Tredoux, Israel, Bishop and Marinus2017; DWS, 2022). The most important for development being the Atlantis and Cape Flats Aquifers (CFA) for the CoCT (Sandveld Group) (e.g., Enqvist and Ziervogel, Reference Enqvist and Ziervogel2019; Ziervogel, Reference Ziervogel2019) and the Algoa Group aquifer for the NMBM (e.g., Goedhart et al., Reference Goedhart, Small and Hulley2004; DWA, 2010; Figure 4).
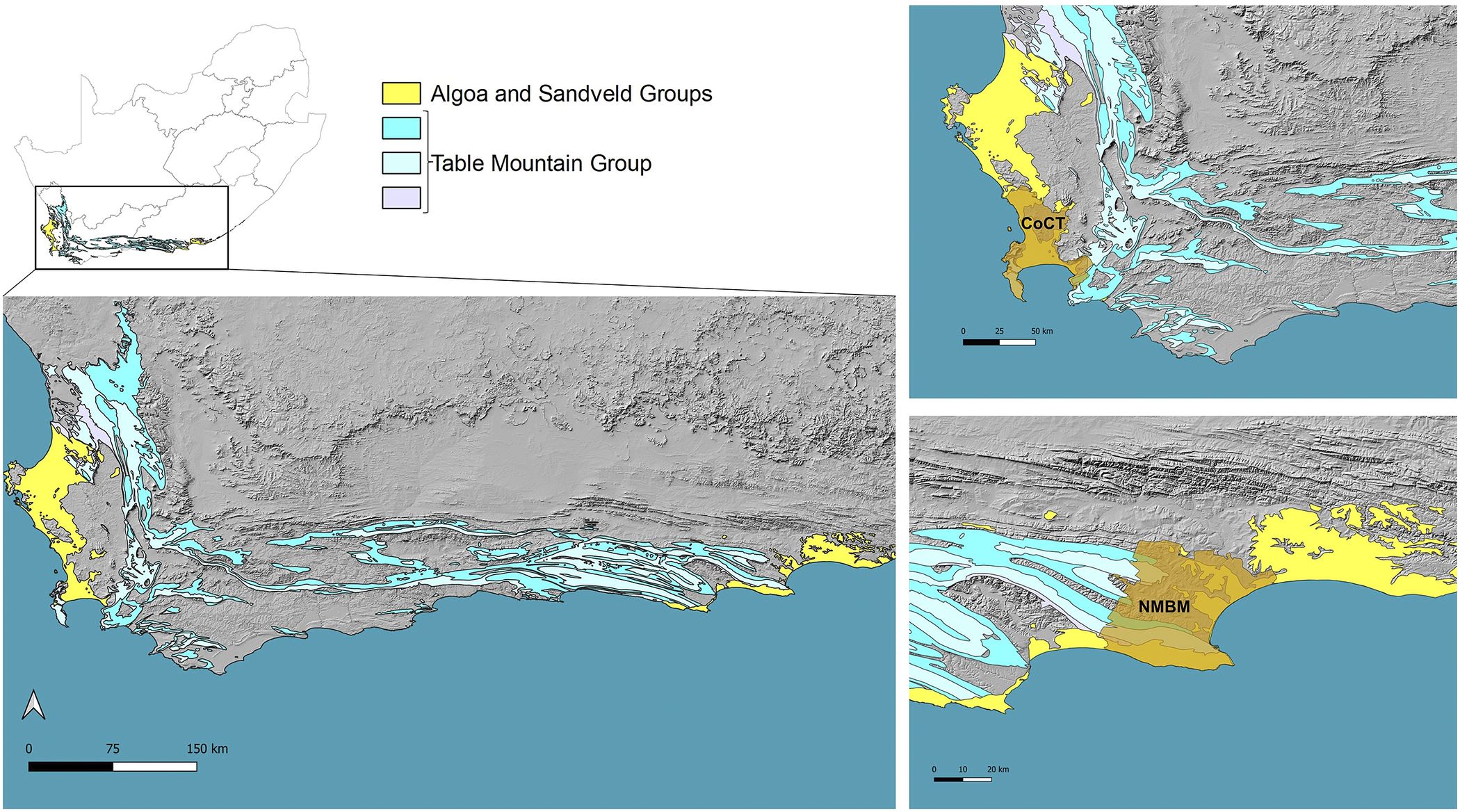
Figure 4. Location and regional extent of the lithological groups of interest for groundwater development in the City of Cape Town (CoCT) and Nelson Mandela Bay Metropolitan (NMBM) municipal areas.
In NMBM, about 10–15% of supply for the town of Kariega (formerly Uitenhage) is derived from the Uitenhage Springs groundwater (Baron, Reference Baron2000; DWA, 2010), which equates to about 1.6% of the NMBM water demand (NMBM, 2022). According to the municipal water outlook reports, it is expected that a further 35 Ml of groundwater can be sustainably abstracted per day from five production well-fields that are being developed in response to the current drought (NMBM, 2022). However, the local NMBM aquifers are poorly understood in terms of inflow sources, recharge, and residence time with scant available hydrogeological literature (e.g., Goedhart et al., Reference Goedhart, Small and Hulley2004; Murray et al., Reference Murray, Goedhart and Baron2008). There is, therefore, a risk that groundwater abstraction during droughts may be a short-term solution should abstraction exceed recharge and aquifer storage. Furthermore, the ecological implications of reduced groundwater discharge (e.g., related to GDEs) are either uncertain or have not been well-considered (e.g., Rishworth et al., Reference Rishworth, Dodd, Perissinotto, Bornman, Adams, Anderson, Cawthra, Dorrington, Du Toit, Edworthy, Gibb, Human, Isemonger, Lemley, Miranda, Peer, Raw, Smith, Steyn, Strydom, Teske and Welman2020b). Pre-drought groundwater use accounted for merely 1.5% of the CoCT water supply and was largely limited to the town of Atlantis (Luker and Harris, Reference Luker and Harris2019). However, groundwater development became an important augmentation strategy during the recent drought for the CoCT, as well as the surrounding agricultural regions, due to the lack of upstream surface water releases and agricultural releases (Watson et al., Reference Watson, Miller, Künne and Kralisch2022). Large-scale groundwater projects prompted by the 2015–2018 drought included the proposition to abstract 100 Ml/day from the TMGA and CFA and further MAR schemes in the West Coast area (Luker and Harris, Reference Luker and Harris2019; Zhang et al., Reference Zhang, Xu and Kanyerere2019b). However, the former was considered unfeasible by experts given the timeline (De Villiers, Reference De Villiers2017; Luker and Harris, Reference Luker and Harris2019) with production well-fields still under development years later (Blake et al., Reference Blake, Hartnady, Hay, Riemann, Abrunhosa, Chambel, Peppoloni and Chaminé2021; McGibbon et al., Reference McGibbon, Hugman, Towers, Riemann, Hay, Hartnady, Abrunhosa, Chambel, Peppoloni and Chaminé2021) and operational issues due to over-recharge and groundwater flooding were previously encountered with the latter (Zhang et al., Reference Zhang, Xu and Kanyerere2019b).
South Africa boasts the most reported cases of MAR in Africa of which one of the most notable is the Atlantis scheme (e.g., Dillon et al., Reference Dillon, Escalante, Megdal and Massmann2020; Ebrahim et al., Reference Ebrahim, Lautze and Villholth2020). The scheme has operated since the 1970s (Bugan et al., Reference Bugan, Jovanovic, Israel, Tredoux, Genthe, Steyn, Allpass, Bishop and Marinus2016; LaVanchy et al., Reference LaVanchy, Adamson, Kerwin, Mukherjee, Scanlon, Aureli, Langan, Guo and McKenzie2021) and uses stormwater, domestic and industrial effluent for recharge to meet domestic demand and simultaneously prevent seawater intrusion. Stormwater and treated domestic wastewater are used in the main recharge basins for the former, while industrial wastewater is released into coastal recharge basins for the latter before discharging into the Atlantic Ocean (Bugan et al., Reference Bugan, Jovanovic, Israel, Tredoux, Genthe, Steyn, Allpass, Bishop and Marinus2016). It is estimated that up to 30% of the Atlantis Town water is supplied from the recharge scheme and future expansion has been proposed to increase water resilience (Ebrahim et al., Reference Ebrahim, Lautze and Villholth2020). Although a largely successful example of MAR, groundwater abstraction from the scheme decreased following access to surface water resources and issues related to borehole clogging and turbidity (Bugan et al., Reference Bugan, Jovanovic, Israel, Tredoux, Genthe, Steyn, Allpass, Bishop and Marinus2016). Decreased abstraction and continued MAR resulted in groundwater level increases, which pose threats in terms of groundwater pollution, flooding risks and ecosystem functioning (Bugan et al., Reference Bugan, Jovanovic, Israel, Tredoux, Genthe, Steyn, Allpass, Bishop and Marinus2016; LaVanchy et al., Reference LaVanchy, Adamson, Kerwin, Mukherjee, Scanlon, Aureli, Langan, Guo and McKenzie2021). The management of the scheme has since been improved including actions to prevent iron clogging through ozone injection, monitoring of groundwater levels and quality, and addressing illegal discharges (Bugan et al., Reference Bugan, Jovanovic, Israel, Tredoux, Genthe, Steyn, Allpass, Bishop and Marinus2016). More recently, it has been proposed that MAR is also implemented in the CFA (e.g., Hay et al., Reference Hay, Holmes, Atkins, Coetzee, Hugman, Flügel, Snyman and De Jager2018; LaVanchy et al., Reference LaVanchy, Adamson, Kerwin, Mukherjee, Scanlon, Aureli, Langan, Guo and McKenzie2021). This would entail that stormwater and domestic wastewater were intercepted before discharging to the ocean. Treated water would be directly injected into the aquifer or recharge could occur through infiltration at wetland areas (Hay et al., Reference Hay, Holmes, Atkins, Coetzee, Hugman, Flügel, Snyman and De Jager2018). Similarly to the Atlantis scheme, this would increase groundwater storage and a total sustainable yield of 18,000 Ml/a is suggested as feasible (Mauck and Winter, Reference Mauck and Winter2021).
Threats of groundwater integration into the water supply systems of the CoCT and NMBM include overexploitation and drawdown. Furthermore, contamination of groundwater resources is a concern (Rosewarne, Reference Rosewarne, Pietersen and Parsons2002; Luker and Harris, Reference Luker and Harris2019). For example, seawater intrusion in the coastal suburb of Summerstrand in the NMBM was already reported in the 1990s (Lomberg et al., Reference Lomberg, Rosewarne, Raymer and Devey1996) and continues to be a concern associated with groundwater use in coastal regions (Rosewarne, Reference Rosewarne, Pietersen and Parsons2002). Developed coastal areas and estuaries within the CoCT and NMBM are also amongst the most threatened areas in South Africa in terms of SLR and the anticipated increase in storm surges (e.g., Theron and Rossouw, Reference Theron and Rossouw2008; Bornman et al., Reference Bornman, Schmidt, Adams, Mfikili, Farre and Smit2016; Raw et al., Reference Raw, Adams, Bornman, Riddin and Vanderklift2021; Allison et al., Reference Allison, Palmer and Haigh2022). Resultant saltwater intrusion into estuaries is expected to be amplified by decreased freshwater inflows and altered sediment sources (e.g., Bornman et al., Reference Bornman, Schmidt, Adams, Mfikili, Farre and Smit2016) and aquifer salinisation and higher groundwater levels are expected (Williams and Lück-Vogel, Reference Williams and Lück-Vogel2020). Coastal flooding has also increased in the CoCT region and is driven by high-intensity rainfall events and larger tidal amplitudes (Dube et al., Reference Dube, Nhamo and Chikodzi2022). Already certain suburbs (e.g., Strand) are subject to frequent coastal flooding and erosion (Williams and Lück-Vogel, Reference Williams and Lück-Vogel2020) and SLR has caused significant economic losses and damage to infrastructure, threatening tourism activities and World Heritage sites (e.g., Robben Island) (Dube et al., Reference Dube, Nhamo and Chikodzi2021), while dune erosion leaves coastal aquifers vulnerable to seawater intrusion (LaVanchy et al., Reference LaVanchy, Adamson, Kerwin, Mukherjee, Scanlon, Aureli, Langan, Guo and McKenzie2021).
In addition, biofouling and iron-related clogging of boreholes may occur (e.g., Maclear, Reference Maclear2001; Fortuin et al., Reference Fortuin, Woodford, Rosewarne and Low2004; Luker and Harris, Reference Luker and Harris2019). Geogenic contamination of the TMGA by high concentrations of radionuclides and metals is a further concern (Mohuba et al., Reference Mohuba, Abiye, Demlie and Nhleko2022). Other issues include a lack of institutional knowledge, the human capacity for maintenance and monitoring, and the absence of regulatory requirements and implementation of restrictions for domestic groundwater use in South Africa (e.g., Wright and Jacobs, Reference Wright and Jacobs2016; Luker and Harris, Reference Luker and Harris2019).
Given the coastal setting of the CoCT and NMBM, it is worth noting that desalination of seawater has been proposed as a potential long-term water supply augmentation strategy for both municipalities that is resilient in terms of climate change (Blersch and Du Plessis, Reference Blersch and Du Plessis2017; NMBM, 2022). For example, this has been employed as a drought management option at another coastal South African town, Mossel Bay (Sorensen, Reference Sorensen2017). However, desalination is an expensive, energy-intensive option with a high carbon footprint compared to other more conventional sources (Gobin et al., Reference Gobin, Sparks, Okedi, Armitage and Ahjum2019). The high electricity costs and frequent electricity outages (“loadshedding”) in South Africa is problematic for both desalination as well as groundwater abstraction and pumping, although this may be mitigated through renewable energy supply in the future (Sorensen, Reference Sorensen2017; Hattingh, Reference Hattingh2022).
From a demand management perspective, both the CoCT and NMBM effectively used several measures to curb water use/loss from the surface water supply systems. These measures included water restrictions, stepped water tariffs, water metres and fixing of water leaks, to mention a few (e.g., Enqvist and Ziervogel, Reference Enqvist and Ziervogel2019; Ziervogel, Reference Ziervogel2019; NMBM, 2022). However, mismanagement of water supply systems, ageing infrastructure and maintenance issues contributed to the water crises. For instance, alien invasive vegetation significantly reduces surface runoff and although clearing programmes (viz. Working for Water) exist and is listed as a demand management strategy (NMBM, 2022), it is essential that routine follow-up clearing is conducted in catchments (Enqvist and Ziervogel, Reference Enqvist and Ziervogel2019; Ziervogel, Reference Ziervogel2019; Holden et al., Reference Holden, Rebelo, Wolski, Odoulami, Lawal, Kimutai, Nkemelang and New2022). Clearing invasives from dune systems may also have a positive impact on shallow unconfined aquifers, as indicated elsewhere along the coast (Görgens and van Wilgen, Reference Görgens and Van Wilgen2004). Of concern is that in contrast to surface water resources, systematic management of groundwater resources, restriction of use at a municipal level and detailed information on private groundwater use is lacking (Ziervogel, Reference Ziervogel2019). For example, the number of registered private boreholes in the CoCT increased from 1,500 to 23,000 within the calendar year of 2017 (Visser, Reference Visser2018).
Therefore, sustainable groundwater abstraction in the CoCT and NMBM requires comprehensive groundwater monitoring, especially related to baseline datasets for future groundwater scheme locations, and offers the opportunity for knowledge building and the advancement of groundwater management institutions (Luker and Harris, Reference Luker and Harris2019). Routine long-term monitoring of groundwater resources and GDEs is a crucial management priority. The removal of alien vegetation from catchments and dune systems is likely to be beneficial for both surface- and groundwater resources. Furthermore, previous economic valuations of water remediation programs in the case study areas (e.g., Hosking and Du Preez, Reference Hosking and Du Preez2002) should be updated and included in a comprehensive cost–benefit analysis of the development of groundwater monitoring programs for the CoCT and NMBM water supply systems. Finally, sustainable groundwater use may be achieved by the conjunctive use of groundwater resources. This is especially needed during supply deficiencies, surface water infrastructure failures (e.g., blocked/damaged pipes) and contamination issues (Luker and Harris, Reference Luker and Harris2019), such as experienced in the Voëlvlei Dam, CoCT (Luker and Harris, Reference Luker and Harris2019) and in the NMBM (Capa, Reference Capa2022).
Strategies and solutions linked to managing hydrological variability relevant to metropolitan cities on dynamic coastlines could include inland MAR schemes that effectively would then allow for higher dependency on accessible groundwater aquifers at the coast while minimising risks of saltwater intrusion and groundwater submergence or land subsidence (Dillon et al., Reference Dillon, Escalante, Megdal and Massmann2020; Alam et al., Reference Alam, Borthakur, Ravi, Gebremichael and Mohanty2021; Pramono, Reference Pramono2021). An urgent research priority should be directed towards quantifying the inland point sources of groundwater recharge, both spatially and volumetrically (e.g., Hall et al., Reference Hall, Currell and Webb2020), as well as through legislative policies regulating and monitoring the quantities of residential groundwater abstraction (e.g., Langridge and Van Schmidt, Reference Langridge and Van Schmidt2020) – one cannot manage what one does not measure. Such research is now underway regionally in South Africa. Knowledge of source-to-sink hydrological connectivity of coastal groundwater cycles would allow interventions to be more effectively directed, such as the removal of water-intensive alien invasive plants in the areas that most directly recharge coastal groundwater-fed aquifers to both improve coastal supply and quality.
Concluding remarks
Coastal aquifers are under increasing pressure due to a myriad of threats affecting the quality and quantity of fresh groundwater resources, often associated with anthropogenic drivers and magnified by climate change and climate extremes. In addition to the “standard” climate change driven impacts, hazards specific to coastal aquifers include SLR, storm surges, seawater intrusion, coastal flooding and erosion. Furthermore, although not unique to coastal aquifers, pressures may be aggravated in coastal areas such as land subsidence due to the unconsolidated nature of sediment and pollution risks since coasts are a nexus for inland and marine contaminants.
Remediation of over-abstracted and contaminated groundwater resources is difficult, costly and in some cases irreversible. Proactive protection of aquifers is therefore crucial for sustainable management of urban water supply. These issues are arguably compounded in coastal cities because of increased water demand due to rapid population growth and urbanisation coupled with decreased recharge. On the other hand, several management solutions and strategies, such as MAR and desalination are practical and potentially the most cost-effective in coastal settings.
To emphasise the issues and management imperatives related to coastal urban water supply and the mitigating role of groundwater during drought-related crises, the investigation of the water supply systems of two South African metropolitan municipalities provides examples of large cities (population > 1 million) experiencing water crises due to anthropogenic (e.g., urbanisation, socio-economic development) and environmental (e.g., decreased precipitation and runoff, increased evapotranspiration) pressures. We specifically chose to highlight this scenario given the regional climate-related future predictions related to increased drought severity. Furthermore, these coastal municipalities rely primarily on surface water resources and therefore have the opportunity to develop integrated water resource management through incorporating alternative water sources, including easily accessible groundwater, into water supply systems. However, the unique coastal pressures facing these groundwater aquifers (e.g., saltwater intrusion and reduced recharge to GDEs) necessitate unique solutions and strategies. Both municipalities implemented several interventions to decrease water demand and increase water supply and although several of these were effective to postpone “Day Zero,” it may not be enough to prevent water supply failure in the future.
Future research priorities related to groundwater at the coast need to account for the following most especially: (1) updating and implementing existing policies to develop integrated groundwater management; (2) long-term groundwater monitoring in terms of quantity and quality for both urban and natural systems, especially related to salinity effects and (3) communication of issues, such as land subsidence, seawater intrusion and coastal ecosystem degradation through, for example, decreased discharge related to large-scale groundwater use during droughts, to seek a balance between human-driven water needs and the sustainability of coastal aquifers both in terms of supply quantity and quality.
Open peer review
To view the open peer review materials for this article, please visit http://doi.org/10.1017/cft.2022.11.
Acknowledgements
Ms Larika van Vuuren is thanked for providing administrative support during the writing of this manuscript. The Editors, Dr Matthew Forbes and an anonymous reviewer are thanked for their valuable input and feedback, which especially helped strengthen the coastal focus of the manuscript.
Author contributions
Conceptualisation: C.D., G.M.R.; Funding acquisition: G.M.R.; Investigation: C.D.; Visualisation: C.D., G.M.R.; Writing – original draft: C.D., G.M.R.
Financial support
This research is funded by the Water Research Commission (WRC) through grant number 2022/2023-00833, “Coastal seeps and groundwater connectivity” awarded to G.M.R. C.D. is funded by the National Research Foundation of South Africa and the Deutscher Akademischer Austausch Diens (UID: 131592). Opinions expressed herein are those of the authors and not necessarily also those of the funders.
Competing interest
The authors declare none.
Comments
Dear Editor,
Attached is an original review manuscript entitled: "Coastal urban reliance on groundwater during drought cycles: opportunities, threats and state of knowledge" by Carla Dodd and Gavin M. Rishworth. This is being submitted as a commissioned review article under several Coastal Futures themes, including Coastal Development (Sustainability and Resilience); Geomorphology (Coupled natural and human dynamics) and Conservation & Restoration (Habitat Conservation). We would be sincerely grateful if this manuscript is considered for publication in Coastal Futures. This study outlines a global overview of threats and opportunities related to groundwater use in coastal urban areas. This is especially important given future socio-economic development and climate change scenarios, which will result in demand outstripping supply in constrained water supply systems globally, as is already being experienced in South Africa. As such, a South African case-study is provided to articulate a water-crisis scenario where integrated groundwater supply may alleviate drought effects in two large coastal metropolitan municipalities. Anticipated threats and possible solutions surrounding large-scale groundwater use is also highlighted. We believe that this review will be of interest to a variety of members of the scientific community, including those involved in coastal development, disaster management, coastal ecology and human sciences.
Kind regards,
Carla