Introduction
Since its origins, environmental education is closely linked to problem-solving activities and specifically to the goal of developing problem-solving competences. Early, in the last years of the 1960’s, environmental education was already fostering a literate environmental citizenship, with citizens who are competent in environmental problem solving (Stapp, Reference Stapp1969). In the late 70´s, the Declaration and Recommendations of the Intergovernmental Conference on Environmental Education, organized by UNESCO and UNEP, included an objective fostering “to help social groups and individuals acquire the skills for identifying and solving environmental problems” (UNESCO, 1978, p. 27). In the 21st century, Sauvé (Reference Sauvé2005) presents the problem-solving current as a traditional and contemporary environmental education focus, in which “The goal is to (…) learn about environmental issues, as well as develop the attitudes and skills for solving them” (p. 15). In this way, environmental education problem solving is linked to real problems, which can be remote or local. In either case, environmental education problem solving requires diagnosing and monitoring the environmental problem in a specific environmental system, making use of real data sets (Lombardi, Reference Lombardi2007). These data sets are nowadays made available by remote data acquisition systems and ubiquitous data connectivity (McGrath & Scanaill, Reference McGrath, Scanaill, McGrath and Scanaill2013b), but are also being produced locally with plug and play affordable and accessible environmental electronic sensors (Silva, Reference Silva2020). In this context, environmental education problem solving involves environmental knowledge building, which requires acquiring environmental information, using human senses and tools, such as electronic sensors (Marchand, Reference Marchand and Marchand2010).
Electronic sensors are devices that can be used to measure or detect a vast variety of physical, chemical, and biological quantities in the real world (McGrath & Scanaill, Reference McGrath, Scanaill, McGrath and Scanaill2013a). Accordingly, human senses are biological sensors. Diversely, transducers transform the output of the sensing process into an electrical or optical measurable signal (McGrath & Scanaill, Reference McGrath, Scanaill, McGrath and Scanaill2013a). Sensors and transducers are usually linked in scientific and everyday devices. The origin of sensors, as digital devices, goes back to the 80’s (McGrath & Scanaill, Reference McGrath, Scanaill, McGrath and Scanaill2013b) and, since then, they came to be smarter, smaller, portable, affordable and wireless (Knight, Reference Knight2005; Shuler, Reference Shuler2009), becoming in this way available to be used in everyday school activities.
The problem-based learning, the inquiry-based learning, and the authentic learning models are three constructivist, student-centred didactic approaches, which have fundamental affordances to develop learning about the real-world environmental problems, as well as to develop the attitudes and skills to solve such problems. The problem-based learning approach is an iterative process that integrates a problem analysis phase, an autonomous research phase and a phase of communication and assessment of the solution/s to the problem (Hung, Reference Hung and Phillips2014; Yew & Goh, Reference Yew and Goh2016).
In environmental education, the problem-based learning approach is often merged with the inquiry-based learning method, to include an investigative strategy and empirical techniques, with the collection and analysis of data, as well as with evidence-based knowledge acquisition and creation (Cobern et al., Reference Cobern, Schuster, Adams, Applegate, Skjold, Undreiu and Gobert2010; Song, Reference Song2014). Besides, in environmental education, those two approaches are interlinked with the authentic learning model, when the analysed problems are complex real-world problems, and the practices that create knowledge (epistemic practices) are embodied, situated (Kelly & Licona, Reference Kelly, Licona and Matthews2018) and use multiple resources, including digital technologies, such as the electronic sensors (Chinn & Malhotra, Reference Chinn and Malhotra2002; Reeves, Herrington, & Oliver, Reference Reeves, Herrington and Olivers2002; Herrington, Reference Herrington2006; Lombardi, Reference Lombardi2007).
Electronic sensors, integrated in mobile digital devices, can be used, with human senses, as epistemic mediators, that is, external devices that support knowledge creation in action (Magnani, Reference Magnani2004).
In this way, sensors and senses can facilitate and enhance the embodied and situated observation of the environment, as well as other epistemic practices, such as collection and analysis of data, communication and sense making of the findings (Woodgate, Stanton Fraser, & Crellin, Reference Woodgate, Stanton Fraser and Crellin2007), in problem-based, inquiry-based and authentic didactic activities. In this context, the purpose of this paper is to present a model, named EcoSolvingS (Solving Environmental Problems, using Senses and Sensors), to support teachers in creating didactic activities that use senses and sensors as epistemic mediators in authentic environmental education problem-based learning.
Following related theoretical and empirical works, the EcoSolvingS model aims to support teachers in exploring the affordances of human senses and electronic sensors in authentic knowledge creation (epistemic) activities with investigative environmental education problem-based learning.
In the next section of this paper, the fundamentals of the use of senses and sensors in investigative environmental education problem-based learning is presented. In the subsequent section, the results of a cross analysis of a set of environmental education problem-solving case studies are presented and related to the components of the EcoSolvingS model. Finally, the model is described, and its utility and developments are discussed.
The Use of Senses and Sensors in Authentic Investigative Environmental Education Problem-Based Learning
Human senses are our interface with the environment, the interface of our environmental experiences, the basis of our embodied experience (Classen, Reference Classen1997). Human senses make it possible to observe the environment and, consequently, they are fundamental to our everyday life (Mason & Davies, Reference Mason and Davies2009) and learning.
Social norms influence how and what people see, touch or smell, and perception is influenced by social practices and ideologies (Classen, Reference Classen2020). In this way, the social construction of sensory perception affects our experience and understanding of our bodies and the world (Classen, Reference Classen1997).
There is a social ranking of senses, which results from cultural traditions and values, and influences, in a decisive way, which sensory information should be considered more important, and which should be filtered or ignored (Classen, Reference Classen2020). In Western culture, vision, followed by hearing, have been the most valued senses since antiquity, with visual and auditory information and practices being more valued (due to their cultural link to rationality and science) than those related to the other senses, such as smell, taste and touch that are considered more linked to physicality and animality (Classen, Reference Classen2020). The multisensory exploration of the environment requires the challenge of the social ranking of the human senses, overcoming the overvaluation of vision and hearing, and using smell, the balance vestibular sense and all the somatic senses, including senses of pain, temperature, itching and proprioception (Gardner & Martin, Reference Gardner and Martin2000), to acquire environmental information. Vision is a primary sense in the perception of the environment and in the use of sensors, but it must be considered, like any other sense, as contributing to a multisensory environmental perception. In recent years, the social and human sciences have carried out a change from unisensory to multisensory approaches (Howes, Reference Howes2006).
Human beings are always involved in a matrix of environmental factors that have an impact on their thoughts and actions, and on the construction of knowledge, in the process of exploring and solving problems (Howes, Reference Howes2010; Marchand, Reference Marchand and Marchand2010). In this sense, knowledge is not a defined object/entity, it is rather a continuous activity, inseparable from practice and everyday life, with the construction of knowledge being shared between people and with the world (Marchand, Reference Marchand and Marchand2010).
Sensory learning, as emphasized by Piaget (Reference Piaget2010) and Montessori (Reference Montessori1987), is a necessary element in the development of formal operations, namely in the transition from concrete thinking to abstract thinking (Minogue & Jones, Reference Minogue and Jones2006). Hands-on activities, in which students use their different senses to acquire environmental information, can scaffold the progress from more concrete to more complex and abstract levels of thought, in the development of essential competences in the 21st century, such as problem solving with critical thinking (Turiman, Omar, Daud, & Osman, Reference Turiman, Omar, Daud and Osman2012).
For instance, when addressing the specific problem of noise pollution at school, it is worthwhile to promote multisensory familiarization of students with sound, its propagation and with its impacts on the environment. The joint use of senses such as hearing, vision and touch is essential for familiarization with sound propagation, as when hearing and touch are mobilized to hear the sound, feel the vibration of a tuning fork and see the wave it creates in the water. On the other hand, hitting with a pencil a wire hanger touching a student’s ear allows him/her to feel the hanger’s vibration, while listening to the sound produced, which is much stronger than if the hanger is not touching the hear. In this case, hearing and touch are being perceived together.
This kind of multisensory explorations constitutes a concrete basis for learning concepts (such as sound level and propagation media) related to the experienced processes (such as vibration and sound propagation), allowing the creation of bridges between the concrete and the more abstract conceptual learning. In this way, a multisensory approach can constitute a concrete basis that facilitates and guides the use (placement and operation) of sensors in acquiring sound level data in different school locations and contexts (with different sound sources and propagation media), as well as the interpretation of such data, which can be related to the located multisensory experience.
In this way, the use of sensors in Environmental Education problem solving can be enhanced by a multisensory approach. Multisensory environmental exploration can support students in (Silva, Lopes & Silva, Reference Silva, Lopes and Silva2013):
-
Learning the properties of natural objects and phenomena, this way supporting the abstract learning on the conceptual web related to the environmental problem that should be explored and solved;
-
Searching for the more adequate conditions — such as location, point of view and point of hearing — to use sensors;
-
Building the concrete experiences that can constitute a basis for the development of the numerical, digital and scientific competences needed to make sense of sensors’ data;
-
Comparing sensory assessments with the sensors’ data, to enhance the use of senses and sensors.
Furthermore, the use of sensors can trigger multisensory exploration, this way contributing to overcome the social hegemony of vision and hearing. For instance, when studying thermal comfort, not only vision, but predominantly touch and the sense of temperature are fundamental human senses to the sensorial familiarization with the temperature magnitude and to heat exchange processes between human body, air, water and objects in the environment (Nogueira, Reference Nogueira2012; Silva, Lopes & Silva, Reference Silva, Lopes and Silva2013). The temperature sense can be used by students to familiarize themselves with thermal comfort in different locations and conditions in the school. Thus, children can be challenged to feel the temperature, and the associated (dis)comfort, in the classroom, outside the classroom and in the corridor (Gomes, Novais & Abrantes, Reference Gomes, Novais and Abrantes2019; Monteiro, Reference Monteiro2018) while measuring temperature with a sensor. Students can also be challenged to feel the water temperature as well as their own body temperature, also relating the sensations to the measured temperature and to the environmental factors affecting it (Nogueira, Reference Nogueira2012; Teixeira, Silva & García-Rodeja, Reference Teixeira, Silva and García-Rodeja2015).
In problem-based learning, the first phase is the problem analysis phase that usually produces a question problem to guide the autonomous research phase, in which the observation practices took place. The joint use of senses and sensors enhance the observation practices, which are fundamental investigative and problem-solving practices. In educational environments, collaboration and participation (with shared conversations) improve observation practices, making them more productive and scientific (Eberbach & Crowley, Reference Eberbach and Crowley2009).
The Observation practices are epistemic practices, that is, practices that build knowledge. This construction of knowledge occurs, over time, through interactions, not only interactions based on data collection, but also in conversations about interpretation, and in processes of communication, evaluation and legitimation, in specific contexts (Kelly & Licona, Reference Kelly, Licona and Matthews2018). Thus, to develop knowledge, during the observation process, students need experiences, domain knowledge and tools, such as sensors (Eberbach & Crowley, Reference Eberbach and Crowley2009).
The joint use of senses and sensors also seems to facilitate and enhance the epistemic practice of “making predictions”, as reported by Nogueira (Reference Nogueira2012) and Teixeira, Silva and García-Rodeja, (Reference Teixeira, Silva and García-Rodeja2015) referring to activities of air and water temperature exploration, developed with 2nd and 5th grade children. In these activities, children improved their estimates by comparing their previous estimates with sensors’ data (Nogueira, Reference Nogueira2012; Teixeira, Silva & García-Rodeja, Reference Teixeira, Silva and García-Rodeja2015).
The practices of observation (with data collection), description (collect and use of information to characterize what was observed) and interpretation (sense making of what have been described) are important steps to develop abstraction in inquiry practices. The joint use of senses and sensors makes it possible the concreteness fading strategy, in which the used representations increase their degree of formality and abstraction, while maintaining the connection to the represented situation (Goldstone & Son, Reference Goldstone and Son2005). Silva, Lopes and Silva (Reference Silva, Lopes and Silva2013) present a set of six case studies on the joint use of senses and sensors, in which the strategy of progressive reduction of concreteness was used to develop abstract thinking. The use of senses makes it possible to anchor sensors’ graphical representations of information acquired through vision, audition, touch, smell and sense of temperature. Furthermore, recordings of such perceptions, such as notes, drawings, photos and videos, can link concrete sensations to graphical and numerical sensors’ data representations. For instance, audios can bridge hearing sensations to sound level graphs.
In the last 20 years, electronic sensors have been increasingly used by students in school activities, namely in authentic investigative science and environmental education. This use has been twofold: (i) the use of sensors with physical computing robotic environments, such as Lego/Logo, Crickets and Arduino (Blikstein, Reference Blikstein, Walter-Herrmann and Büching2013); (ii) the use of plug and play sensors in mobile devices to acquire and visualize environmental data. Nevertheless, there is a lack of use of mobile devices by students and teachers in science education to produce, collaborate and create knowledge (Crompton et al., Reference Crompton, Burke, Gregory and Grabe2016; Oliveira et al., Reference Oliveira, Feyzi Behnagh, Ni, Mohsinah, Burgess and Guo2019). The model proposed in this paper addresses the need of supporting environmental educators in scaffolding students during the embodied use of senses and sensors. This use of senses and sensors can (Oliveira et al., Reference Oliveira, Feyzi Behnagh, Ni, Mohsinah, Burgess and Guo2019): (i) mediate the students’ environmental perception and experience, as epistemic mediators that co-constitute the reality being investigated; (ii) create opportunities for epistemic action, enhancing observation, measurement, interpretation and evidence-based decision making in environmental education problem-based learning.
In this paper, the classes are framed as communities of practice, which include people, tools and culture of practice (Stroupe, Reference Stroupe2014), and where teachers and students negotiate and produce epistemic practices (Kelly & Licona, Reference Kelly, Licona and Matthews2018). Furthermore, in the development of the EcoSolvingS model, sensors are envisioned as epistemic mediators that (France et al., Reference France, Lee, Maclachlan and McPhee2021): (i) can be used in a situated way, contributing to the continuous construction of the learning spaces; (ii) contribute to a more fluid instead of a more complex learning experience; (iii) should allow students to collaborate with the teacher, and other students; (iv) integrate data produced in the problem-solving tasks.
Cross-Case Analysis of Case Studies on the Use of Senses and Sensors in Authentic Environmental Education Problem-Solving Activities
In this section, the results of a cross-case analysis of a set of case studies (Yin, Reference Yin2003) on the use of senses and sensors in authentic environmental education problem-solving activities are presented (see Tables 1, 2 and 3). The analysed case studies were supervised by the author of this paper, implemented by pre-service and in-service teachers, and centred on the agency of students in solving authentic environmental problems in their schools. They were guided by a previous framework (Silva, Lopes & Silva, Reference Silva, Lopes and Silva2013), which was also based on a previous set of case studies, developed from 2010 to 2012 by in-service teachers, with schoolchildren from 7 to 12 years old. That framework is grounded on (Silva, Lopes & Silva, Reference Silva, Lopes and Silva2013): (i) the joint use of senses and sensors by children, in contexts of Environmental Education; (ii) the development of epistemic practices by children, as a result of the use of senses and sensors in experimental work; (iii) teaching mediation of the use of senses and sensors in investigative and experimental work, supporting the prediction and sense making of acquired data, and bridging the gap between sensory experiences and the most abstract representations of environmental information.
Table 1. Identified components of two case studies on noise pollution with 1st and 4th grade schoolchildren

Table 2. Identified components of two case studies on Thermal Comfort with 6th grade schoolchildren
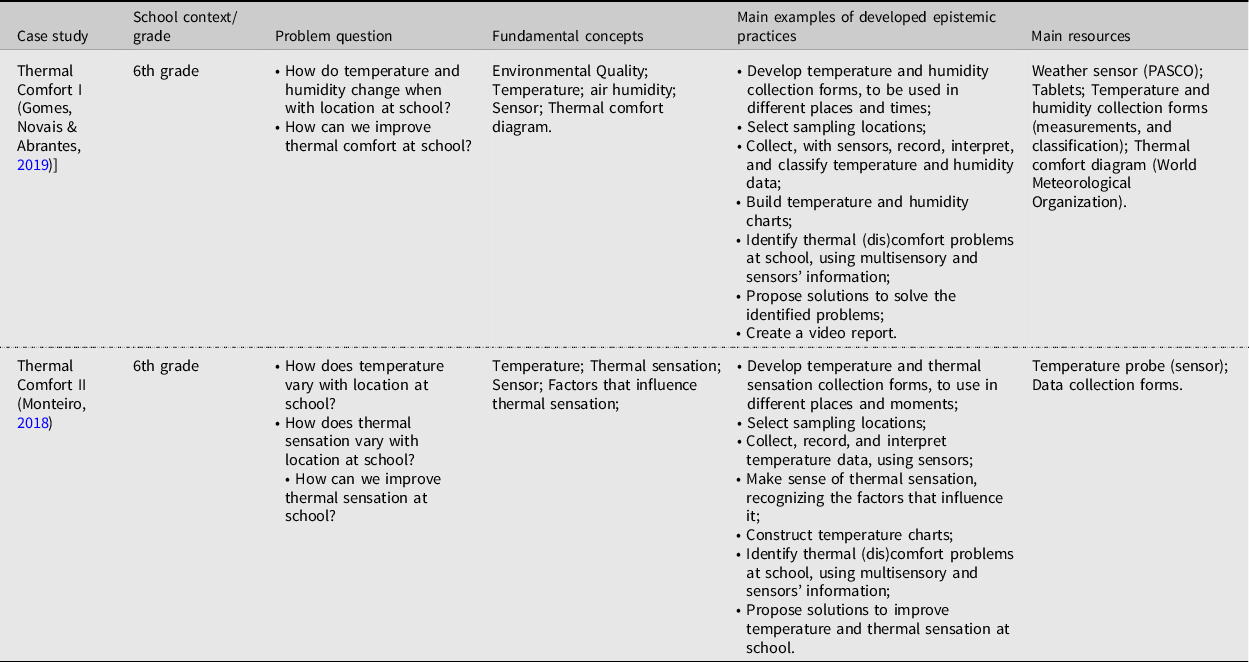
Table 3. Identified components of three case studies on air quality with 6th grade schoolchildren, Professional High School students, and pre-service teachers

The cross-case analysis, whose results are here presented, is data grounded and searched for the fundamental components of case studies, to inform the modelling of the environmental education problem-based learning activities, in the construction of the EcoSolvingS model.
All the problem-solving activities, implemented in the context of the analysed case studies, were mediated by an educator. In three cases (Thermal Comfort cases, and Air Quality I case), the activities were mediated and assessed by pre-service teachers, in the context of their internship, while in four cases (Noise Pollution cases, and Air Quality II and III cases) the activities were mediated and assessed by in-service teachers.
In problem-based learning, learning begins with a problem that needs solving (Yew & Goh, Reference Yew and Goh2016). The seven analysed case studies (see Tables 1, 2 and 3) are centred on three main environmental problems of schools (WHO, 2015): noise pollution; thermal (dis)comfort and air pollution. These are real problems that ask for students’ complex reasoning and decision making. In problem-solving, problem questions, often presented by teachers, drive and scaffold students in inquiry processes and complex reasoning (Tawfik et al., Reference Tawfik, Graesser, Gatewood and Gishbaugher2020). In Tables 1, 2 and 3, it is possible to examine the problem questions that guided the diverse case studies, and which were established by teachers and students in the beginning of the problem-solving processes. In each case, the first question/s are testing questions focused on meaning-making and interrelations between the variables (Tawfik et al., Reference Tawfik, Graesser, Gatewood and Gishbaugher2020). The last question/s are deep/complex questions that support complex reasoning to establish causality and decision making (Tawfik et al., Reference Tawfik, Graesser, Gatewood and Gishbaugher2020).
Differing transmissive approaches, the problem-based learning approaches scaffold students in acquiring, applying and construct content knowledge (Hung, Reference Hung and Phillips2014). In this approach, the content knowledge is organized around problems, and is learned in the context of the problem-solving tasks, to be usable in real life situations (Hung, Reference Hung and Phillips2014). Furthermore, problem-based learning is an iterative process, in which students understand the problem space through multiple comprehension cycles (Tawfik et al., Reference Tawfik, Graesser, Gatewood and Gishbaugher2020). In each of the analysed case studies, it is possible to identify a set of integrated fundamental concepts (Tables 1, 2 and 3), linked both to the phenomena being inquired, as well as to the inquiry tasks in problem solving. For instance, in case study Noise Pollution II, the concepts of sound, sound propagation, sound level and decibel are interconnected and linked to the noise pollution phenomenon, while the concepts of sound scale, chart, average, maximum and minimum are related to the processes of data classification, visualization and processing.
In performing authentic inquiry tasks, students, with teacher mediation, iteratively construct knowledge trough epistemic practices, such as planning procedures, selecting variables, controlling variables, observing/sensing, recording data, creating multiple representations, discussing, interpreting, proposing solutions and making decisions. The analysis of such epistemic practices, in authentic (real word) inquiry, developed in meaningful environmental education problem-solving contexts, can constitute an authentic assessment technique (Figueiredo, Reference Figueiredo2005; Chinn & Malhotra, Reference Chinn and Malhotra2002; Silva, Lopes & Silva, Reference Silva, Lopes and Silva2013; Silva et al., Reference Silva, Ferreira, Souza, Alves and Batista2018a).
The activities of all the case studies were curricular activities in formal contexts. In these contexts, the pre and in-service teachers assess students’ learning, in what concerns content and process learning, with a focus on the analysis of students’ epistemic practices. The results of such assessment were very positive (as described in detail in the reports referenced in Tables 1, 2 and 3), this way validating the structure of the cases’ activities. The analysis of the epistemic practices was centred on the recordings produced by students in the data collection and interpretation forms, during such practices. In the Noise Pollution II case, which was developed with eight 4th grade classes, the assessment was also based on a pre and post-test that allowed the confirmation of content and process learning. In all the cases, that assessment of the epistemic practices allowed the recognition of procedural and content knowledge acquisition (learning) and of knowledge creation (new local data and information about the environmental problems).
In addition to human senses, the main resources used in the analysed case studies were sensors, data collection and interpretation forms, together with scales with safety limits of the monitored variables. Sensors have been successfully used, as epistemic mediators, in all the mentioned cases. As in the related case studies, presented and analysed in Fenton (Reference Fenton2008), van den Berg, Schweickert, and van den Berg (Reference van den Berg, Schweickert and van den Berg2010) and Silva, Lopes and Silva (Reference Silva, Lopes and Silva2013), sensors, as epistemic mediators, allowed students to collect and signify quantitative environmental information that would not be otherwise accessible (Magnani, Reference Magnani2004). In all those case studies, the use of human senses to observe objects and processes not only informed the students’ search for the appropriate conditions for an effective use of the sensors, but also created a concrete basis that facilitates the interpretation of abstract information acquired by the sensors.
Registration forms, which can be broadly defined as documents with an organized set of fields for information input, were also important epistemic mediators, supporting knowledge creation in action (Magnani, Reference Magnani2004). In the presented case studies, the set of fields in the used registration forms scaffolded students, during problem exploration and solving, in developing epistemic practices, such as the control of variables, organization, classification, interpretation and use of acquired environmental data.
In the case studies presented in Tables 1, 2 and 3, the registration forms played an important role in the promotion, explanation and visibility of epistemic practices. In the different case studies, those forms were diverse: (i) collaborative experiment plans, to create the problem question and plan experimental activities with control of the variables (Aboim, Reference Aboim2014; Santos, Reference Santos2018; Silva, Reference Silva2020); (ii) data collection forms, which include tables, just for registering the data acquired with the sensors (Monteiro, Reference Monteiro2018), or more complex ones, to register the predictions/estimates/sensations or the classification of acquired data (Gomes, Novais & Abrantes, Reference Gomes, Novais and Abrantes2019; Santos, Reference Santos2018; Silva & Rodrigues, Reference Silva and Rodrigues2019; Silva, Reference Silva2020) using security scales of the different environmental factors; (iii) and the interpretation forms to register qualitative interpretation of the data (Melo, Silva & Valente, Reference Melo, Silva and Valente2019; Santos, Reference Santos2018; Silva, Reference Silva2020), identify environmental problems and make decisions in problem solving (Melo, Silva & Valente, Reference Melo, Silva and Valente2019; Santos, Reference Santos2018; Silva, Reference Silva2020).
Except for the case study Thermal Comfort II, which used a stand-alone temperature probe, in all the case studies under analysis, the sensors, linked to tablets/mobile phones and using the SPARKvue app, allowed students to visualize and explore the acquired environmental data. The joint use of those tools and of the registration forms made it possible for students to bridge multiple representations (see Figure 1), while performing the desired epistemic practices, such as observing/sensing, recording data, creating multiple representations, discussing, interpreting, proposing solutions and making decisions.

Figure 1. Sound sensor, tablet showing data charts, data collection and interpretation form, filled by a 4th grade student, and his/her drawing, during Noise Pollution II case study.
In the case studies on Sound Pollution II (Silva, Reference Silva2020) and Thermal Comfort I (Gomes, Novais & Abrantes, Reference Gomes, Novais and Abrantes2019), developed in the context of the Eco-Sensors4Health Project (https://eco-sensors4health.site/), a collaborative platform (http://www.eco-sensors4health.pt/) was used to share and visualize the data acquired with the sensors in the diverse schools and activities (Silva et al., Reference Silva, Ferreira, Souza, Alves, Rito and Gomes2018b). Children used the platform to register, share and visualize the acquired data, in a very positive way. The ease of use of the platform was increased by the consistency of the data collection forms with the platform’s data entry fields. Furthermore, the acquired data charts, in the SPARKvue app, were consistent with the visualization of the [blind] platform’s data graphs, this way facilitating interpretation and sense making.
In the projects that were pioneers in using sensors to solve environmental problems, such as the Globe (Smoľáková, Švajda, Koróny, & Činčera, Smoľáková et al., Reference Smoľáková, Švajda, Koróny and Činčera2016), the Ambient Wood (Rogers & Price, Reference Rogers and Price2004), the LillyPad (Rogers, Connelly, Hazlewood, & Tedesco, Reference Rogers, Connelly, Hazlewood and Tedesco2010) and the SENSE (Fraser et al., Reference Fraser, Smith, Tallyn, Kirk, Benford, Rowland and Fitzpatrick2005) projects, data visualization and data sharing tools were also fundamental resources to problem-solving inquiry activities. In the same way, at present and in the last years, such tools are recognized as fundamental to develop visualization, information and data literacy in formal education, since early education (Chevalier et al., Reference Chevalier, N., Alper, Plaisant, Boy and Elmqvist2018) and in environmental education citizen science projects (Castell et al., Reference Castell, Grossberndt, Gray, Fredriksen, Skaar and Hoiskar2021; Golumbic, Fishbain, & Baram-Tsabari, Reference Golumbic, Fishbain and Baram-Tsabari2020). Nowadays, the importance of the use of data visualization and data sharing tools in environmental education is strengthened by the recent exploration of the potential of the Internet of Things in schools, which complements the use of local sensors (Davies, Beauchamp, Davies, & Price, Reference Davies, Beauchamp, Davies and Price2020).
EcoSolvingS: A Model to Support Teachers in Developing Environmental Problem-Based Learning Activities
The EcoSolvingS model (Solving Environmental Problems, using Sensors) is presented in this section. The design of this model was informed, as described in the previous sections, by related theoretical and empirical works and by the cross-case analysis of multiple case studies on the use of electronic sensors to solve environmental problems, in school contexts. It aims at promoting critical thinking in environmental problem solving, using senses and sensors, and at supporting teachers, from primary to higher schools, in developing environmental problem-based learning activities.
In Figure 2, it is possible to observe the structure of the EcoSolvingS model, with the integrated set of components and their relationships:

Figure 2. EcoSolvingS model (Solving Environmental Problems, using Sensors), a model to support teachers in developing environmental problem-based learning activities.
-
The problem questions should be designed and reified in an experiment plan, by students with teacher mediation, in a first phase of the problem-solving planning, in order to guide the following activities;
-
The network of concepts and processes, related to the problem, should be explored in an active way, by students with teacher mediation, during the problem-solving activities. This exploration can be supported by multimedia resources in an initial approach, and/or can be approached, in an integrated way, during the development of the various students’ tasks;
-
The sensorial practices are the practices, asked to students by teacher mediation and guided by the problem questions, in which the different senses are mobilized, together with everyday material resources, to explore, signify and concretize the concepts and processes related to the problem, this way creating learning outcomes;
-
The students’ epistemic practices are the practices that produce knowledge. These practices are scaffolded by teacher mediation and resources, such as sensors, data forms, data visualization and data sharing tools, and should be designed on the basis of the Science and Technology practices that produce scientific and technological knowledge;
-
Students’ decision making is the set of students’ practices that create solutions to the identified problems, based on the results of previous epistemic practices;
-
In the EcoSolvingS model, the multiple resources (mediators in teachers’ and students’ practices) are diverse — the human senses, everyday objects and materials, different tools, such as electronic sensors and visualization tools, and documents, such as data collection forms and multimedia presentations;
-
Teacher mediation mobilizes resources and creates interactions with students, allowing them to overcome challenges that otherwise they would not be able to reach. It includes the assignment of tasks and the scaffolding of students´ practices;
-
The learning outcomes are consequences of students’ practices with teacher mediation, and include learning of content knowledge, changing attitudes, and developing competences. Learning outcomes can be assessed in the process, or at the end of it, both formatively and summatively.
The EcoSolvingS model integrates the joint use of senses and sensors, with a concreteness fading strategy, from sensorial to epistemic practices, and from the everyday observations to iconic and abstract representations, in order to improve: (i) students’ awareness of environmental processes and of the potential of senses and sensors to their exploration; (ii) sensorial observation, through the comparison of the data acquired by the sensors to what is perceived by senses; (iii) the observation of processes and descriptions, as well as the interpretations, predictions and decisions in environmental education problem-solving.
The EcoSolvingS model can support the design of environmental education problem-solving activities, from the beginning of school years to Higher Education. However, in the first years of schooling, teacher mediation should be more intensive, reducing degrees of freedom and more closely guiding the practices to the desired goal, this way reducing the complexity of the problem-solving process (Reiser, Reference Reiser2004). Furthermore, for early childhood, sensorial practices should play a major role, and the design of children’ epistemic practices with sensors should consider the previous children’s experiences, namely to choose the adequate data representation (configurable in sensors’ apps), and the data to be organized and interpreted (Silva & Rodrigues, Reference Silva and Rodrigues2019; Teixeira, Silva & García-Rodeja, Reference Teixeira, Silva and García-Rodeja2015).
Conclusion
This paper is centred on the EcoSolvingS model (Solving Environmental Problems, using Senses and Sensors), a model to support the use of human senses and electronic sensors, as epistemic mediators, in environmental education problem-solving activities.
In this model, the use of senses challenges the social hegemony of vision and hearing, looking for the integration of all the human senses, including touch, sense of temperature, and smell, in multisensory environmental exploration. Such approach addresses the potential of sensorial activities to support the development of abstract thinking, needed to collect and analyse environmental information.
The EcoSolvingS model was developed based on diverse educational approaches, such as the authentic, problem-based, sensory, experiential and inquiry approaches to learning. In this context, previous and related theoretical and empirical works revealed that with teacher mediation, the joint use of senses and sensors allows students to develop epistemic practices, such as observing/sensing, recording data, creating multiple representations, discussing and interpreting the acquired information. In this context, scaffolding strategies include the concreteness fading strategy that starts with sensory approaches to problems and progresses to increasingly abstract analysis, this way linking concrete sensations to iconic and symbolic representations of environmental information.
The cross-case analysis of a set of case studies that were based on the aforementioned assertions allowed the identification of key components of environmental education problem-solving activities, using senses and sensors: problem questions; content knowledge related to the problem; sensorial and epistemic practices; assessment of students’ practices and resources, including senses, sensors, data forms, and data visualization and sharing tools. These key components informed the structure of the EcoSolvingS model that is centred on students’ sensorial, epistemic and decision-making practices. In this model, students’ practices make use of the asserted resources, are guided by problem questions and content knowledge, mediated by teachers, and produce learning outcomes, such as learning of content knowledge, changing attitudes and developing competences.
Even being the only case study that was analysed regarding gender questions, it is noteworthy that the results of the assessment of the case study “Noise Pollution II” revealed no significant gender differences (Silva, Ferreira & Batista, Reference Silva, Ferreira and Batista2019). The EcoSolvingS model is intended to support a gender-neutral use of digital technologies in environmental education problem-solving activities. The mentioned model recommends the use of mobile electronic sensors, which are not gender marked devices, as evidenced by catalogues, guides and papers that present photos of both boys and girls using them (Fenton, Reference Fenton2008; Kedzierska, Reference Kedzierska2017; van den Berg et al., Reference van den Berg, Schweickert and van den Berg2010). Furthermore, the EcoSolvingS model includes other elements, such as hands-on activities, project-based learning and scientific inquiry, with real-world experience, which are reported as more attractive to girls UNESCO (2017). Additionally, the EcoSolvingS model aims at supporting the development of environmental problem-based learning activities in formal educational contexts, from primary to higher schools, where activities are designed for all (boys and girls).
To explore their potentialities, the future use of the EcoSolvingS model should be centred on Teacher Training. The development of this model was informed by a set of case studies developed by pre-service and in-service teachers. Furthermore, since its development, the EcoSolvingS model has been used to develop Teacher Training Workshops, aiming at empowering pre and in-service teachers to support students in using senses and sensors to environmental problem solving in schools. In those Workshops, which have a short duration (12 h), this model has been successfully used to design and implement sessions to familiarize teachers with the potentialities of the joint use of senses and sensors, as well as to support those teachers in creating, implementing and assessing didactic sequences in schools (Silva & Rodrigues, Reference Silva and Rodrigues2020).
Acknowledgements
None.
Conflicts of Interest
Nothing to declare.
Financial Support
The work presented in this paper was partially funded by the FEDER (PORTUGAL2020) and Portugal State Budget (FCT) [LISBOA-01-0145-FEDER-023235].
Ethical Standards
Nothing to note.
Maria João Silva, Coordinator Professor with Aggregation and Tenure in Polytechnic of Lisbon, School of Education, since 2015; Professor at the Polytechnic of Porto School of Education, from 1987 to 2015; Aggregation in Education (Lusofona University, Degree and PhD in Environmental Engineering (Nova University Lisbon), Master's and Post-doctorate degree in Information Systems and Technologies in Education (Coimbra University). Coordinator and member of diverse research projects in environmental education. Coordinator of the Eco-Sensors4Health Project (from 12/10/2017 to 11/3/2019), funded by Portugal2020 and FCT. She has several publications on environmental education.