Introduction
There are reports of 34 surges in the Karakoram since the 1860s involving 23 glaciers (Reference HewittHewitt, 1969, Reference Hewitt1998). Twelve of these are tributaries, detached from or joined to main glaciers that are not known to surge. Many surges, especially of the less accessible tributaries, may have gone undetected. All but one of the main glaciers reach inhabited areas or terminate near important trails, making reports of their activity more likely.
Since 1985, more surges have been recorded than in any comparable period in records since the 1850s (Reference MasonMason, 1930; Reference HewittHewitt, 1969). Of thirteen events, ten have been tributary glacier surges; improved observation may be a factor. However, equivalent numbers are not evident in satellite imagery before 1985, suggesting a real increase in events since then. Moreover, four independent tributaries of Panmah Glacier have surged in the past decade, three of them in quick succession between 2001 and 2005 (Fig. 1). Landsat imagery shows that two tributaries of the adjacent Sarpo Laggo Glacier, and another in an unnamed basin nearby, surged some time after 1995 (Table 1). This concentration of events is unprecedented.

Fig. 1. Map of Panmah Glacier showing four recent surges. The heads of ice streams are shown schematically to reflect how most of the basin above 5000 m consists of rock walls, seasonally or perennially snow-covered, subject to all-season avalanching, and with countless small, steep ice masses that contribute to the trunk streams through ice avalanches.
Table 1. Surging tributaries: dimensions of Panmah and other glaciers with tributaries that recently surged in the central Karakoram

The perennial snow and ice cover of the Greater Karakoram Range and adjacent Hindu Raj is about 18 500 km2 (von Reference von Wissmann and FlohnWissmann and Flohn, 1959). It is dominated by large glaciers with no record of surges. The 12 largest basins comprise almost half the cover. The glaciers are at globally extreme elevations and have exceptional elevation ranges. Generally, they differ in elevation and climate regime from most of the glaciers used to track contemporary glacier change (Reference Oerlemans, Lisse and BalkemaOerlemans, 2001). Recent events raise two broad issues: the nature of surging tributaries and reasons for this sudden concentration of them. These issues are addressed after describing the surges in the Panmah Glacier system.
Recent Panmah Glacier Tributary Surges
Panmah Glacier is the sixth largest glacier in the region and is composed of two main branches joining 7 km above the terminus. All four recent surges are in west- or southflowing, east-bank tributaries of the Nobande Sobande Glacier branch (Fig. 1). Before any surges were reported, three of these tributaries were classified as ‘suspected’ surging glaciers by Reference KotlyakovKotlyakov (1997, plate 406). The four surges affect Chiring, Maedan, Shingchukpi and Drenmang Glaciers. The first three had detached and retreated from the main glacier since the 1930s, but all four now contribute ice to it.
The 1995 Chiring Glacier surge was described by Reference HewittHewitt (1998). By the summer of 2005, marked thickening was observed in its accumulation zone. The entire ablation zone had thinned to 10–50 m below the 1996 profile. It was no longer heavily crevassed but remained active throughout. Tributaries sheared off in the surge have now adjusted to enter at the present ice level.
Maedan Glacier surged some time after 2002, causing major thickening and break-up of its lower 8 km (Fig. 2). Along the side-walls were masses of dirty ice, in places more than 100 m above pre- and post-surge levels, evidence of a ‘mid-glacier zone’ of the surge, with ‘compressive followed by extensive flow’ (Reference Murray, Dowdeswell, Drewry and FrearsonMurray and others, 1998, p. 271). When observed in July 2005, the surge was over but the lower Maedan Glacier surface remained a mass of seracs. A smoother lobe of its ice, ~3 km2 in area, had entered the main Nobande Sobande Glacier and formed a series of concentric ridges, with the highest at the outer rim, reflecting a zone of compression continuing after the surge. Opposite the junction, Chiring Glacier was compressed to a narrow, heavily crevassed thread. The lobe of the 1995 Chiring Glacier surge ice in the main valley was distorted by Maedan Glacier, but its outer rim was still a well-defined, steep-sided ridge of ice ~40–60 m high.

Fig. 2. Overview of Maedan (M) surge in July 2005 where it enters the Chiring (C) and Nobande Sobande (NS). The lobe Chiring (C) surge ice is also seen and the conspicuous pressure ridge at its rim. The outer part of the Shingchukpi (S) surge is visible in the middle distance.
The Maedan Glacier surge was preceded by a steady advance. In September 1992 its terminus was ~3 km from Chiring Glacier, but had advanced 1.5 km by 1996. It reached Chiring Glacier in 2000, 2 years before the surge.
Shingchukpi Glacier lies parallel to Maedan Glacier, its basin having a similar aspect and morphology. In 1996 the terminus was ~2.5 km from the main glacier. Like Maedan Glacier, it advanced steadily almost 2 km before the surge, which began in the fall or winter of 2004/05 and was still in progress in July 2005 (Fig. 3). Again, sections of dirty marginal ice were stranded tens of metres up the valley walls. The seracs of Shingchukpi Glacier remained distinct from Nobande Sobande Glacier ice, which had been pushed into a series of relatively smooth concentric ridges, broken systematically by transverse crevasses. The three innermost ridges had cupola-like crests, curved bridges of highly stressed ice that shattered at a slight touch. Masses of ice breccia occurred along the crests or beneath the cupolas (Fig. 4).

Fig. 3. Shingchukpi Glacier surge, July 2005. The surface consists almost wholly of seracs, and dirty ice is thrust up irregularly at margins. View is from Nobande Sobande ice in foreground.
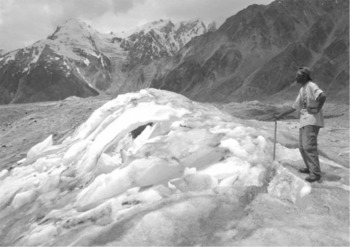
Fig. 4. Ice ridge on Nobande Sobande Glacier, 100 m from contact with the Shingchukpi Glacier surge ice (to left of photograph). Cupola-like bridges are shown and ice breccia beneath.
The Drenmang Glacier surge probably began in the fall or winter 2004/05, coming from the east branch, the larger of Drenmang Glacier’s two main sources. When visited in 1996, and on Landsat imagery to 2001, ice from the shorter western branch occupied more than half the combined area beyond their junction. By the summer of 2005 this relationship was reversed. There were steep ice cliffs along both flanks of the eastern branch, and broken masses of dirty ice were overriding lateral moraines that had been ice-free for some decades (Fig. 5). The central areas were more crevassed than in 1996, but not as severely broken as on Shingchukpi or Maedan Glacier, perhaps because the surge was in an early stage.

Fig. 5. Drenmang in July 2005, looking up the left-flank surging tributary from Nobande Sobande. Arrows identify surge-generated disturbances.
Features of tributary surges
Each of the Panmah tributary glacier surges involved the usual sudden acceleration, severe break-up of the glacier surface, rapid thickening and terminus advance. In the accumulation basins, surface collapse marked the upper limits of the surges and new or expanded crevasses. The highest of the disturbances were at 5400 m a.s.l. for Chiring Glacier, 5200 m for Maedan and Shingchukpi Glaciers and, to July 2005, 5100 m for Drenmang Glacier. These limits are ~1200 to 1600 m lower than the watershed maxima, so the surge events were confined to about the 1000 m range in elevation. The impeded run-out suggests the elevation ranges and ‘surge lengths’ are different from main glacier surges. Moreover, a distinctive three-fold partitioning of surge-transferred ice occurred between the tributary and main glaciers and post-surge stagnant ice.
As described for Shingchukpi Glacier, there are large, immediate impacts on the main glacier, but half or more of the surge ice remained in the lower tributary valleys to enter the main glacier in a post-surge, or relaxation, phase. As with all observed Karakoram examples, actual surges were short-lived, lasting a few months, unlike those reported from Svalbard, for example, which last several years (Reference Dowdeswell, Hamilton and HagenDowdeswell and others, 1991). However, at Chiring Glacier, ‘quiescent’ phase conditions were not resumed in the ablation zone for at least 6 years. Until then, flow rates and crevassing remained greater than before the surges or at present. Likewise, 2–3 years after the Maedan Glacier surge, one-third of the surge ice, estimated relative to the pre-surge surface, remained in the tributary ice stream. An even larger fraction was observed in Shingchukpi Glacier after the surge front had passed its full length. Thus, in July 2005, the four events represent different surge and post-surge phases from Chiring Glacier, essentially returned to quiescent or ‘normal’ behaviour, to Drenmang Glacier, then in mid-surge. Similarly extended periods of post-surge adjustments were observed by the author in the years following the tributary surges of Pumarikish Glacier into Hispar Glacier in 1989 (Reference Wake and SearleWake and Searle, 1993), and Liligo Glacier into Baltoro Glacier in the mid-1990s (Reference Pecci and SmiragliaPecci and Smiraglia, 2000). This extends and complicates the notion of surge episodes, or their impact in mountain valleys, suggesting a need to define a condition intermediate between surging and ‘normal’ flow behaviour.
Major, post-surge adjustments of the main glacier also continue for some years. They include progressive distortions of pre-existing surface features, including the looped and ‘teardrop’ forms widely associated with surging glaciers (Reference LawsonLawson, 1996). In the Panmah Glacier system, developments were complicated by interactions among the several surges occurring in rapid succession (Fig. 6). However, the boundaries of the ice from each surge were still well defined years after the surges.

Fig. 6. Panmah Glacier surface changes associated with surges: (a) 1993, prior to Chiring surge; ice of 1977–8 Drenmang surge was just passing the mouth of Chiring indicating an average annual movement of about 500 m; (b) 1996, conditions following the 1994–5 Chiring surge; (c) 2005, showing complex interactions of older Drenmang and Chiring surges with ongoing Maedan and Shingchukpi events. The new Drenmang surge has barely affected the main glacier.
Only one case is known of a surging tributary directly causing advance of the main glacier: the Lokpar Glacier surge, which occurred sometime between 1989 and 1993. It caused a rapid advance of the main Aling Glacier terminus of ~1.5 km (Reference HewittHewitt, 1998), and a further 2 km of post-surge advance when observed in 2001. However, this tributary entered within 1 km of the snout, and the main glacier did not surge. Indeed, a reverse slope of over 100 m developed between the surge-elevated terminal ice and trunk stream, which has continued thinning as in previous decades.
The Panmah Glacier surges deposited large volumes of stagnant ice along tributary margins and their lateral moraines. These marked the exceptional heights reached in each surge but were subsequently sheared off, as described below. They differ from reports for main trunk surges where post-surge stagnant ice is found in lower tongue and terminus areas (Reference Benn and EvansBenn and Evans, 1998). For example, this was observed after the Karakoram surges of Karambar Glacier in 1993 and Bualtar Glacier in 1986, while negligible amounts of ice were stranded along their lateral margins (Reference HewittHewitt, 1998). In the Panmah Glacier system, some millions of cubic metres of ice, super-elevated during the surge, were left along tributary margins, having a significant influence on moraine development and ice budget (Fig. 7). Conversely the outer lobes remain active parts of the main glacier.

Fig. 7. Stagnant surge ice (arrows) along the post-surge Chiring margins, 1996. The heavily crevassed active ice (A) is 30 m below surge height but 50 m thicker than in 2005 when several other bands of dead ice were present below that shown here (cf. Fig. 2 and Reference HewittHewitt, 1998).
The stagnant ice involved a phenomenon widely reported during surges: a well-defined line of shear or ‘fault’ near the ice edge or between active ice and stagnant ice, or moraine, at the glacier margin. In fact, an ice-edge line of shear is almost universal in the ablation zones of Karakoram glaciers, but undergoes exceptional development during major thickening and surges. In the rising phase of the latter, the ‘fault’ extends upwards and outwards and commonly projects to a vertical or overhanging ice cliff. However, following the Chiring Glacier surge the line of shear was reestablished stepwise within the ice, as the active ice stream thinned and contracted. Successive bands of dead ice recorded the changes (Fig. 2, centre foreground).
These surges did not reach the Panmah Glacier terminus, but, between 1996 and 2005, Nobande Sobande Glacier thickened for several kilometres below Shingchukpi Glacier, and reactivated ice around the junction with Choktoi Glacier. The main terminus continues to thin and stagnate, as it has since the 1930s, but in time the surges may lead to a delayed advance.
In general, Karakoram surging tributaries are steeper than most reported elsewhere. The mean elevation range for the whole set is 2620 m, and the average length 11 km, compared to 2156 m and 10.9 km for the five known Panmah surging tributaries, and 3910 m and 12.2 km, respectively, for all Karakoram surging glaciers. The 20 km long Hassanabad Glacier, and 16.5 km Minapin Glacier in Hunza, evidently have the greatest elevation ranges for mountain surging glaciers: 5570 and 5400 m, respectively, to the lower limit of their last surges (Reference HewittHewitt, 1998). The 41 km long Khurdopin Glacier is the tenth longest glacier in the region and the longest known to surge. All the others are less than 21 km long, while 35 not known to surge are longer than this. These data seem to differ from key findings in the Alaska–Yukon Ranges, North America, (Reference Clarke and BlakeClarke, 1991) and Svalbard (Reference Jiskoot, Murray and BoyleJiskoot and others, 2000), where length is positively correlated with likelihood of surging. However, the Karakoram lacks such detailed inventories for comparable statistical analysis, and reports from those regions do not suggest equivalent numbers or significance of surging tributaries.
Interpreting The Events
Surges are generally isolated developments involving single glaciers (Reference SharpSharp, 1988; Reference Murray, Dowdeswell, Drewry and FrearsonMurray and others, 1998). The Karakoram record to 2000 was of widely scattered events, each seemingly unrelated to what was happening at that time elsewhere in the region, in adjacent basins or in other glaciers known to surge (Reference HewittHewitt, 1998). Models explain surging in terms of internal, mainly thermal and hydrological, developments affecting the base of the glacier, possibly involving soft basal sediments (Reference KambKamb, 1987; Reference Clarke and BlakeClarke and Blake, 1991; Reference Fowler, Murray and NgFowler and others, 2001).
Nevertheless, response to climate change seems the only explanation for events at Panmah Glacier. Unlike the Susitna Glacier tributaries in Alaska (Reference Clarke and BlakeClarke, 1991), these surges are not linked. Initially, three of the glaciers were not connected to the main glacier. Their known surge intervals do not support a mere coincidence. Historical evidence shows Maedan Glacier surged sometime between 1856 and 1861, and Chiring Glacier in 1886–87, implying recurrence intervals of roughly 155 and 120 years respectively if the recent surges are taken as ‘regular’. Drenmang Glacier surged in 1930–31 and 1977–78, suggesting a recurrence interval of almost 50 years. Of course, the interval from 1978 to 2005 is barely half of that. Since surges appear to come from Drenmang’s east and west branches alternately, their surge intervals could be different or, since they have very similar characteristics, may have been of the order of 100 years each until affected by recent conditions. Drenmang Glacier surges could be from the east and west branches at roughly 100 year intervals. No past surges of Shingchukpi Glacier are recorded, but local shepherds had identified it as a surging glacier.Footnote *
Compared with the mid- and high-latitude surging glaciers that have led theoretical discussions and modelling, investigations of Karakoram examples provide limited data. However, conditions that distinguish the region from other Himalayan and inner Asian ranges that lack surging glaciers help to bracket the interpretive issues (Fig. 8). The area–altitude distribution of the glaciers is exceptional. All originate over 6500 m a.s.l.; nine over 7000 m a.s.l. More important for glacier mass balance and climate response, about 80% of total ice area lies between 4000 and 6000 m, comprising most of the accumulation and ablation zones, and all of the surging tributaries.

Fig. 8. Area–altitude relations of glacier environments in the central Karakoram identify regime factors that could affect surging or climatic influences on it. The air temperatures shown differ from accumulation zone snow and ice. In open, avalanche-free areas at 5000–6000 m a.s.l., firn temperatures at depth in pits and cores were at least 5°C warmer (Reference WakeWake, 1989).
There is a five- to ten-fold increase in precipitation from glacier termini ~2500 m a.s.l. to accumulation zones above 4800 m a.s.l. (Hewitt, unpublished information). Maximum precipitation occurs between 5000 and 6000 m (Reference Hewitt and ShroderHewitt and others, 1993), i.e. 2000–3000 m higher than generally reported from the tropics (Reference BarryBarry, 1992, p.234). Most glaciers, and all those known to surge, are nourished mainly or wholly by avalanched snow. High-altitude snowfall, or the relative proportions of summer vs winter, can change the scale, pattern and qualities of avalanches. Mass balance could be altered in this way, and ice temperatures as well as firn temperatures and debris characteristics.
Three different climatic regimes affect the region. A ‘sub-Mediterranean’, westerly circulation and cyclonic storms dominate the winter, accounting for two-thirds of high-altitude snowfall (Reference Hewitt, Wake, Young and DavidHewitt and others, 1989). One-third derives from summer snowfall and the Indian Ocean, mainly due to monsoon incursions (Reference WakeWake, 1989). Continental anticyclones over inner Asia affect the behaviour of the other two systems and the incidence of clear weather, critical for ablation. Mass balance is intermediate between the ‘summer accumulation’ types of the Greater Himalaya, and winter accumulation farther west (Reference Ageta and FujitaAgeta and Fujita, 1996). Glacier climate is affected by changes in the relative strength, character and seasonality of these systems.
High-altitude climate and thermal change
There is growing evidence elsewhere that climate can influence surge incidence and event triggers. It may occur in three ways: through mass-balance change; episodes of exceptional ablation and free water ponding; or ice thermal change. Surge intervals have been shown to reflect the net rate of accumulation over intervening periods at Variegated Glacier, Alaska, and at Medvezhiy Glacier, 450 km northwest of Panmah Glacier (Reference Eisen, Harrison and RaymondEisen and others, 2001). Climatestation records in and around the Karakoram show statistically significant increases in winter and summer precipitation since 1961 (Reference Archer and FowlerArcher and Fowler, 2004). They also indicate winter warming, but declining summer temperatures and temperature ranges, attributed to greater summer cloudiness and storms (Reference Archer and FowlerArcher and Fowler, 2004). The changes suggest conditions favouring positive mass balance.
However, a huge loss of ice mass and glacier recessions were observed in almost all Karakoram glaciers for most of the 20th century until the mid-1990s. Since then there has been thickening and advances in many non-surging glaciers, but confined to the highest watersheds of the central Karakoram (Reference HewittHewitt, 2005). Glaciers in the rest of the region continue to decline, including in most valleys where the climate stations are located. Also, the expansions occurred suddenly and irregularly while the climate data suggest long-term, relatively small and gradual trends, but large, short-term fluctuations.
The absence of comparative data from high altitudes makes it difficult to extrapolate changes observed at weather stations to the glaciers. Most stations are located well below 3000 m. Moreover, mass-balance change alone seems unlikely to cause the sudden convergence of surges in glaciers formerly out-of-phase by decades. Rather, this suggests some critical threshold(s) being reached. Reference PatersonPaterson’s (1994, p.337) observation seems pertinent here, that ‘Only in temperate glaciers is the effect of a climate change restricted to a change in mass balance...’ Changes in ice thermal regimes, or meltwater availability, can also induce accelerated changes in glacier behaviour and extent. All four classes of thermal regime recognized by Paterson are present in the Karakoram, sometimes in the same glacier, i.e. ‘warm’ ice throughout or sub-zero, ‘cold’ ice throughout, with the melting point reached only at the base, or only in surface layers. Measurements are few, but show variations dependent on elevation and orientation (Batura Glacier Investigation Group, 1979). Thermal shifts would most probably occur in a vertical sense, supporting the possibility of threshold changes at critical elevations.
If cold ice is warmed, flow rates may increase. The transition from frozen to wet bed conditions can dramatically affect movement by sliding, whether directly or through softening of subglacial till. It is not clear what the climatestation evidence of summer cooling means for high-altitude snow and ice temperatures. Summer precipitation at the arid valley-floor climate stations is liquid, and evaporative cooling occurs during and after rainfall, although katabatic winds as well as cloud cover tend to buffer them from intense nocturnal cooling. Conversely, accumulation zone snowfall occurs almost wholly within the cloud layer and reflects its temperature, suggesting that increased summer snowfall would increase firn temperatures. However, periods of clear weather in summer are associated with intense daytime warming, and melting of firn as high as 6000 m to depths of tens of centimetres. This does alternate with nocturnal freezing. Radiative cooling refreezes firn from the surface downwards, while a second freezing front develops at depth where percolating meltwater is deposited as ice lenses and layers in sub-zero firn. The latter tend to insulate firn at depth from superficial temperature changes and further moisture percolation, but with net warming from release of latent heat. Without monitoring, the situation is too complex to say what climate changes would mean in terms of the net effect on firn temperatures. Meanwhile, there are no studies of thermal conditions in the allimportant, all-season avalanche cascade that nourishes most glaciers and links a wide range of elevations; hence, no basis to say how climate change would affect it. However, winter warming and increased summer snowfall make it more likely that sub-zero ice moving out of the accumulation zones is being warmed.
Ice thermal changes are rarely invoked even for century-long variations, since ice at depth has been found mainly to reflect the temperature of accumulation zone snow. It may take centuries for the latter to reach and affect the base of the glacier, kilometres away. However, conditions in these steep, avalanche-fed glaciers with large icefalls (Fig. 8) may accelerate response to thermal change (Reference HewittHewitt, 2005).
Climate change here, as elsewhere, affects variability and not just trends in mean conditions. Reference Eisen, Harrison, Raymond, Echelmeyer, Bender and GordeevEisen and others (2005, p.404) argue that episodes of exceptional melting and influxes of free water may be the precursors of some surges and of their termination. In the Karakoram, the impact of ablation on glacier behaviour is reflected by a 20–30% increase in normal summer movement rates compared to winter (Reference Hewitt, Wake, Young and DavidHewitt and others, 1989). Two kinds of anecdotal report from the surge-triggering zones may be relevant. Between 1998 and 2005, the writer has observed midsummer slush flow activity originating above 4000 m a.s.l., of previously unrecorded scope. Many flows travelled several kilometres, and there was ponding in slush swamps. One of these, near the equilibrium-line altitude on Biafo Glacier in 1999, covered more than 2 km2 and two-thirds the width of the ice. The activity was associated with heavy, late-spring snowfalls, with wet snow, and water ponded or flowing over ice lenses beneath the firn surface as high as 5600 m. Also involved were short-term episodes of exceptional warmth despite, perhaps related to, stormier summer weather. Meanwhile, as in the Qomolangma region, mountaineers report changes in the quality of high-altitude ice and snow towards a ‘more tropical’ or a ‘rotten’ consistency. This suggests an increase in high-elevation snow temperatures, at odds with evidence of summer cooling at lower elevations.
Progressive warming of ice over the past century, and/or short-term, intensive melting events in critical areas, have the potential to accelerate or trigger surges. They may preempt the surge cycle and cause a convergence of surges in different glaciers. Perhaps tributaries, the smaller glaciers at high elevations, are especially susceptible. Finally, there are the implications of the sudden and irregular expansions in many non-surging Karakoram glaciers since the mid-1990s (Reference HewittHewitt, 2005). A shift to positive mass balance may be taking place, in keeping with weather-station records and gauging stations that show reduced run-off from the most heavily glacierized Hunza basin (Reference Archer and FowlerArcher and Fowler 2004). Again, however, the suddenness of the changes must be considered and their confinement to the highest watersheds. This suggests thermal and/or hydrological threshold(s) being crossed that trigger downslope redistribution of ice by normal as well as surging flow, with or without mass-balance changes.
Acknowledgements
I thank M.J. Hambrey for helpful comments on an early version and I. Evans on Figure 8, two anonymous reviewers for many helpful suggestions and P. Schaus for preparing the figures. Earlier baseline studies drawn on here were funded by the International Development Research Centre, Ottawa, Canada; Pakistan’s Water and Power Development Authority; and Wilfrid Laurier University’s Office of Research. Mountain guides Mohammed Khan of Hushe, Ghulam Mohammad and Mohammad Ibrahim of Haldi, greatly assisted in the field.