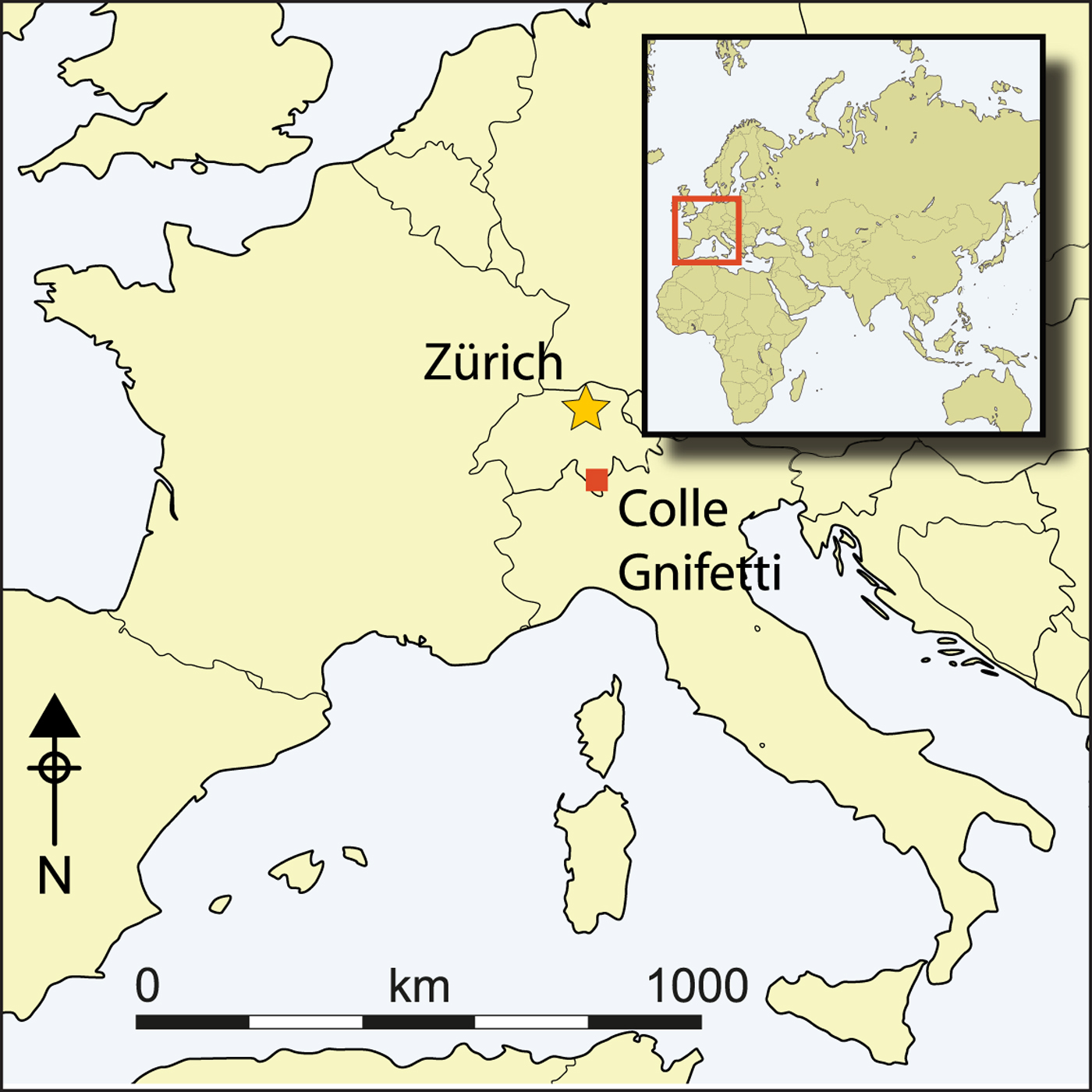
Introduction
During the second half of the seventh century AD, the coin-using regions of North-west Europe switched from gold to silver currencies. This change was a key element in the social and economic transformations that resulted in increased long-distance trade, greater specialisation and the growth of large port settlements (McCormick Reference McCormick2001; Loveluck Reference Loveluck2013). Yet the sources of the silver that allowed the shift from gold to silver coinage have proven enigmatic. In the rare cases when scholars have addressed this issue, they have assumed that the silver came primarily from existing European stocks of residual Roman bullion, or that silver was imported from the Mediterranean region (Scull Reference Scull, Hines and Bayliss2013: 546; Naismith Reference Naismith2014: 10). Recent archaeological exploration of an early medieval silver mine at Melle (Deux-Sèvres, France) and the isotopic profiling of its lead have indicated that exploitation of these deposits could have contributed to the epochal economic shift of the mid seventh century AD (Téreygeol Reference Téreygeol and Bourgeois2010: 251, 259). Data on lead pollution from a new ice core at Colle Gnifetti, Switzerland (Figure 1), provides evidence of the renewed smelting of silver-lead ores from c. AD 640–670. This unambiguously shows that, alongside any residual pool of Roman bullion and imported metal, new mining facilitated the production of the last post-Roman gold coins—debased with increasing amounts of silver—and the new silver coinages that replaced them. The high-resolution ice-core record offers a new and independent chronology for renewed silver production in the early medieval west.
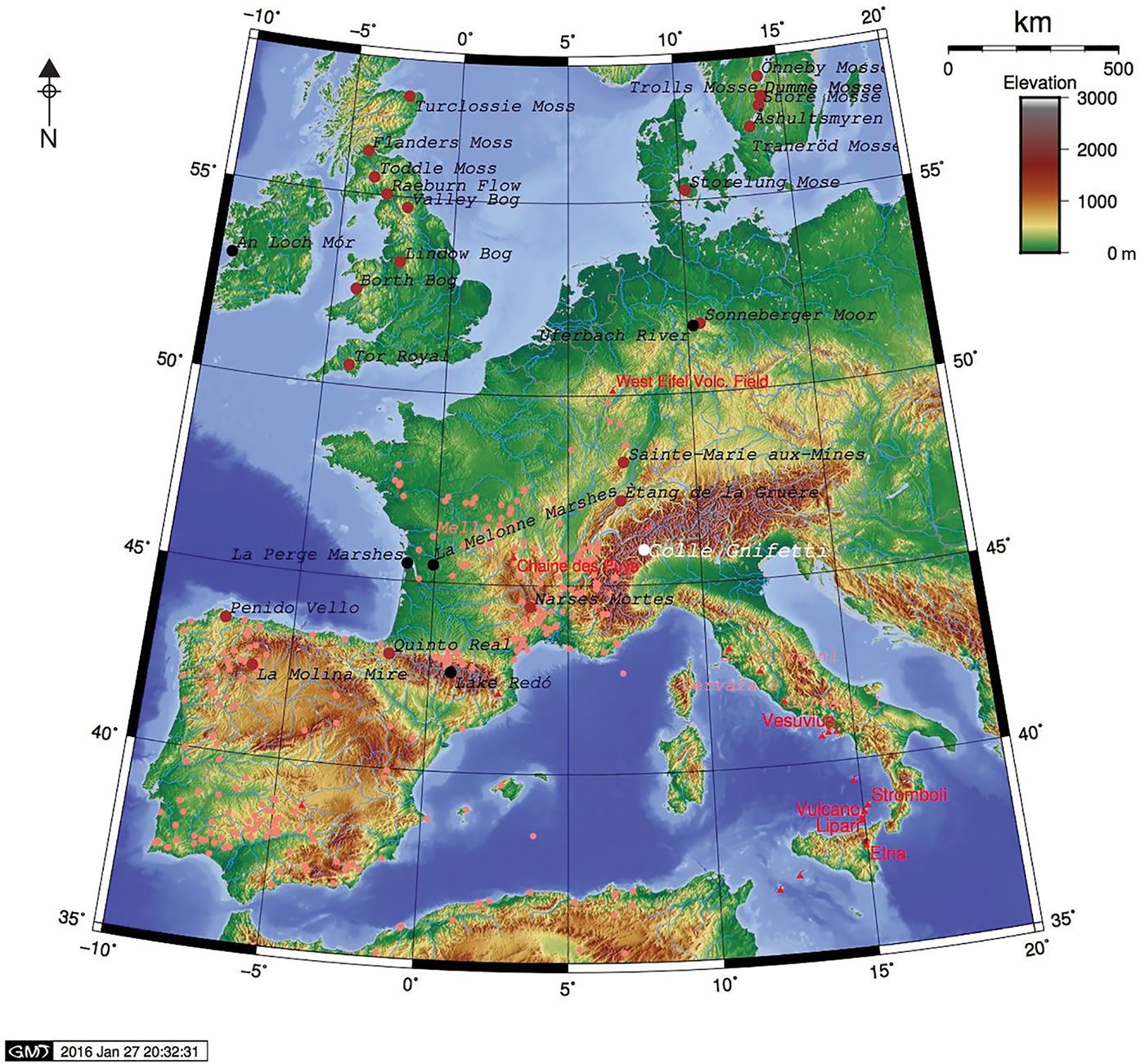
Figure 1. Location of the Colle Gnifetti ice-core and other geo-archaeological pollution records across Western Europe (figure by A.V. Kurbatov).
Previously published ice-core records, for example, from central Greenland (GRIP and GISP2), demonstrate trends in changing pollution and climate-driven atmospheric circulation in the northern hemisphere. These include evidence for the scale of northern hemisphere silver-lead smelting in the Roman period, and for the influences of climate change on marine and terrestrial air masses from the ‘Medieval Climate Anomaly’ (c. AD 950–1250) to the ‘Little Ice Age’ (c. AD 1400–1700) (Mayewski et al. Reference Mayewski, Meeker, Morrison, Twickler, Whitlow, Ferland, Meese, Legrand and Steffensen1993; Hong et al. Reference Hong, Candelone, Patterson and Boutron1994; Kreutz et al. Reference Kreutz, Mayewski, Meeker, Twickler, Whitlow and Pittalwala1997). In contrast, the new Alpine ice core from Colle Gnifetti (45°55′N, 7°52′E) provides a lead pollution record from the heart of Western Europe, with a high-resolution annual layer-counted chronology covering the last 2000 years (More et al. Reference More, Spaulding, Bohleber, Handley, Hoffmann, Korotkikh, Kurbatov, Loveluck, Sneed, McCormick and Mayewski2017; Bohleber et al. Reference Bohleber, Erhardt, Spaulding, Hoffmann, Fischer and Mayewski2018). The core documents the ‘signatures’ of a range of macro-economic and societal changes that are not evident in those Greenland ice cores published to date. One of these signatures is the renewed mining and smelting of silver-lead ores in Western Europe during the seventh century AD. Analysis of atmospheric circulation patterns, provided by Climate Re-analyzer™ visualisation software (Climate Change Institute, University of Maine—CCI), illuminates the potential sources that delivered lead aerosol to Colle Gnifetti. We compare the ice-core evidence to other pollution records and archaeological and historical data from specific mining sites and regions (Figure 1). We then consider the implications of this new evidence for understanding the transformation of the economy of mid seventh-century early medieval Europe.
The Colle Gnifetti ice core: analytical methods and chronological framework
The ice core was extracted in August 2013 from the Colle Gnifetti glacier (Monte Rosa, 4450m asl) in the Swiss-Italian Alps. The drill site was located on the north-facing slope of the glacier, on a flow-line trending towards its eastern flank (Figure 2). The core was drilled to bedrock, is 72m long and has an estimated net surface accumulation of approximately 0.2m of water equivalent per year. The chronological resolution derived from different elements and the measurement of lead pollution was established in a two-phase process: firstly, through continuous flow analysis and high-resolution discrete inductively coupled plasma mass spectrometry (ICP-MS) and ion chromatography; and, secondly, by annual layer-counting using ultra-high resolution laser ablation inductively coupled plasma mass spectrometry (LA-ICP-MS). (For the detailed methodological procedures, materials, specification and calibration details of the instruments used, see Sneed et al. Reference Sneed, Mayewski, Sayre, Handley, Kurbatov, Taylor, Bohleber, Wagenbach, Erhardt and Spaulding2015; More et al. Reference More, Spaulding, Bohleber, Handley, Hoffmann, Korotkikh, Kurbatov, Loveluck, Sneed, McCormick and Mayewski2017; Bohleber et al. Reference Bohleber, Erhardt, Spaulding, Hoffmann, Fischer and Mayewski2018.)
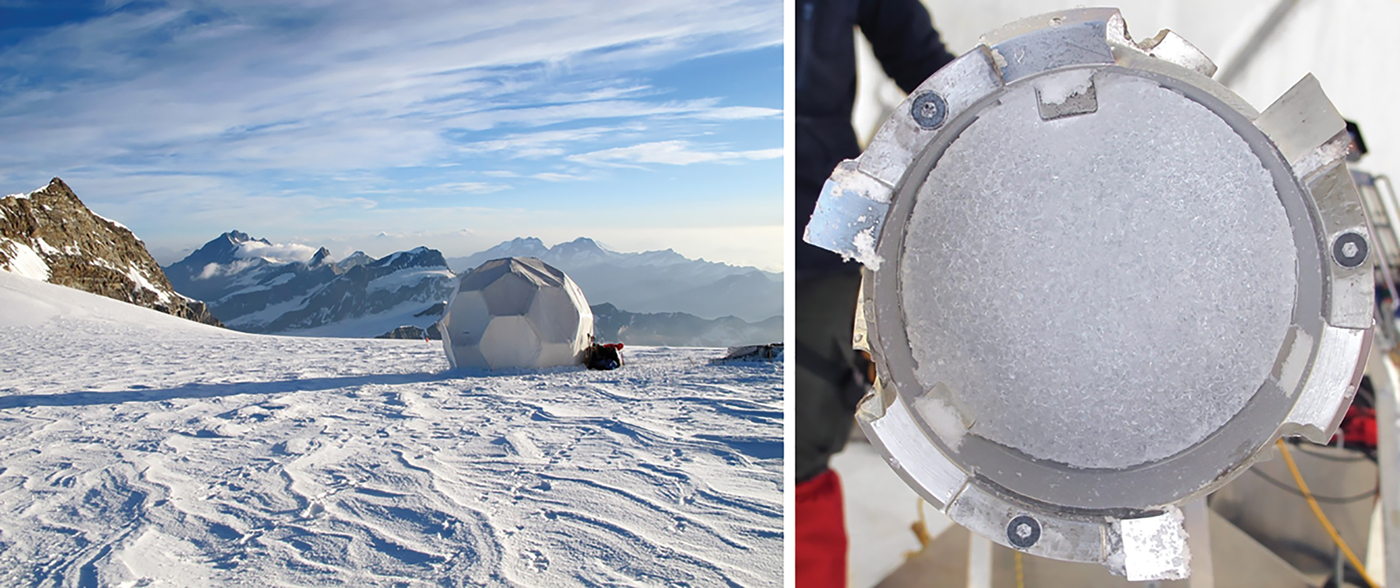
Figure 2. Left) the drilling site (under the dome tent) on Colle Gnifetti; right) a section of the core (photographs by N.E. Spaulding).
Ultra-high resolution sampling of the ice core by LA-ICP-MS occurred at intervals of 120 microns, and was undertaken at CCI (Sneed et al. Reference Sneed, Mayewski, Sayre, Handley, Kurbatov, Taylor, Bohleber, Wagenbach, Erhardt and Spaulding2015). This new method allows the counting of highly compressed and thinned annual ice layers not previously resolved by existing centimetre-resolution analyses of ice cores. Figure 3 shows an example of annual layer-counting with LA-ICP-MS for the years AD 700–704, using atmospheric dust-derived calcium (see also the online supplementary material (OSM) S2.1). Layers were identified as local maxima in the calcium profile, corresponding to mostly summer snow preservation (Preunkert et al. Reference Preunkert, Wagenbach, Legrand and Vincent2000: 998). Elements such as iron were used to corroborate further the calcium-based layer-counting. Since AD 1900, known time-markers, such as documented major Saharan dust events, were used to constrain the ice-core chronology. For the most recent 800 years or so, further corroboration was provided by direct time series comparisons with a neighbouring Colle Gnifetti ice core, dated with conventional centimetre-resolution analysis (Schwikowski et al. Reference Schwikowski, Barbante, Doering, Gaeggeler, Boutron, Shotterer, Tobler, Van de Velde, Ferrari, Cozzi, Rosman and Cescon2004). The discrete liquid-based ICP-MS measurement of multi-element (including lead) concentrations through the new core was also conducted at CCI, with an average resolution of 45mm from a water stream generated by the continuous melter system (Bohleber et al. Reference Bohleber, Erhardt, Spaulding, Hoffmann, Fischer and Mayewski2018).
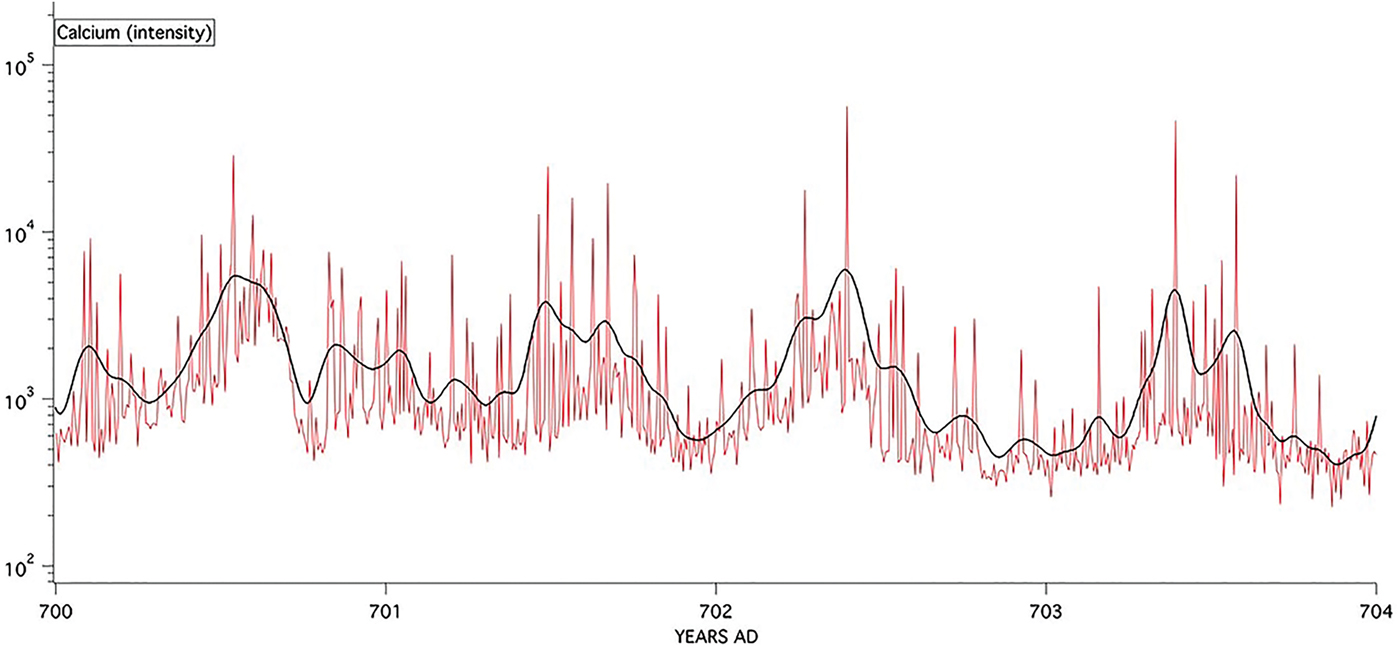
Figure 3. Example of annual layer-counting on the Colle Gnifetti ice core for the years AD 700–704, using ultra-high-resolution LA-ICP-MS analysis of calcium intensity (figure by P.A. Mayewski).
The ultra-high-resolution time series allows the application of the Colle Gnifetti layer-counting procedure back to at least the beginning of the first millennium AD. The layer-counting methodology had a theoretical error margin of ±72 years at c. AD 600, without absolute dating markers to tie the chronology more closely (Bohleber et al. Reference Bohleber, Erhardt, Spaulding, Hoffmann, Fischer and Mayewski2018). The chronology has been greatly refined and established to address the research questions of this paper by the use of three absolute chronological markers that reduce this theoretical error margin to an estimated ±10 years or fewer. The first absolute marker is provided by the Black Death signal (see More et al. Reference More, Spaulding, Bohleber, Handley, Hoffmann, Korotkikh, Kurbatov, Loveluck, Sneed, McCormick and Mayewski2017). More et al. (Reference More, Spaulding, Bohleber, Handley, Hoffmann, Korotkikh, Kurbatov, Loveluck, Sneed, McCormick and Mayewski2017) have demonstrated that despite a theoretical error of 35–40 years, between 1330 and 1370, the total collapse in lead pollution in layer-counted deposits, from 1349 to 1353, matches exactly the absolute chronology from textual sources for the Black Death epidemic, especially in Britain—the probable source of the pollution record for that period.
The second absolute marker comes from the geochemical evidence of the massive eruption of the Icelandic Eldgjá volcano between AD 934 and 939 (Thordason et al. Reference Thordason, Miller, Larsen, Self and Sigurdson2001). The discrete ICP-MS data from Colle Gnifetti present a large bismuth peak at AD 945, which probably indicates the Eldgjá eruption. Although this also corresponds with a large sulphate peak at Colle Gnifetti, sulphate cannot be used as a reliable volcanic marker there, as most of it arrives in the form of gypsum (calcium sulphate) as dust (Schwikowski et al. Reference Schwikowski, Barbante, Doering, Gaeggeler, Boutron, Shotterer, Tobler, Van de Velde, Ferrari, Cozzi, Rosman and Cescon2004). A similar contribution of sulphate from dust was encountered in the Mount Everest ice core, where bismuth was also used as the independent volcanic marker (Kaspari et al. Reference Kaspari, Mayewski, Kang, Sneed, Hou, Hooke, Kreutz, Introne, Handley, Maasch, Qin and Ren2007: 1–2). The identification of the large bismuth increase at c. AD 945 at Colle Gnifetti with the Eldgjá eruption corresponds closely with its eruption interval, suggested as AD 934 in Alpine tree rings and at 939 in the GISP2 Greenland ice core (Baillie & McAneney Reference Baillie and McAneney2015: 112). The occurrence of other Icelandic volcanic signals in the Colle Gnifetti core has also been confirmed by the publication of Icelandic tephra recovered from it (Luongo et al. Reference Luongo, Kurbatov, Erhardt, Mayewski, McCormick, More, Spaulding, Wheatley, Yates and Bohleber2017), and is further emphasised by the new discovery below.
The third absolute marker is provided by analysis of newly discovered tephra particles from the Colle Gnifetti core that are chemically very similar to the tephra from the NEEM-2011-S1 Greenland ice core—shard QUB-1859 (Sigl et al. Reference Sigl2015; see OSM S1). The latter has been interpreted as tephra corresponding to the AD 536 volcanic event. The NEEM-2011-S1 core is tied chronologically to the GICC05 Greenland ice core, which fixes the NEEM-2011 and the Colle Gnifetti tephra to AD 536±2 (Sigl et al. Reference Sigl, McConnell, Layman, Maselli, McGwire, Pasteris, Dahl-Jensen, Steffensen, Vinther, Edwards, Mulvaney and Kipfstuhl2013). The Colle Gnifetti annual layer-counting chronology places the AD 536-event tephra at AD 500, suggesting a maximum error of 36 years at AD 536 in the initial chronology (Bohleber et al. Reference Bohleber, Erhardt, Spaulding, Hoffmann, Fischer and Mayewski2018). Recounting of the annual layers from the Eldgjá event to AD 536 has ascertained that, due to poorly defined annual layers, the counting error occurred between c. AD 500 and 600. The current theoretical error margin between AD 600 and 700 is estimated at a maximum of ±10 years, and less through the eighth and ninth centuries AD.
Lead pollution: potential sources, historical events and societal trends
The lead pollution record reflected in the new discrete ICP-MS time series at Colle Gnifetti provides a unique archive of changes in the smelting of silver-lead ores for Western Europe, transported to the western Alps by long-distance, high-level tropospheric and low-level regional winds over the last 2000 years (More et al. Reference More, Spaulding, Bohleber, Handley, Hoffmann, Korotkikh, Kurbatov, Loveluck, Sneed, McCormick and Mayewski2017: fig. 1). It can be compared to less temporally resolved records provided by peat and lake sediment cores. The most reliably dated of the latter indicate a post-Roman decline in lead emissions through the fifth and early sixth centuries (Le Roux et al. Reference Le Roux, Weiss, Grattan, Givelet, Krachler, Cherbukin, Rausch, Kober and Shotyk2004: 507; Kylander et al. Reference Kylander, Weiss, Martinez-Cortizas, Spiro, Garcia-Sanchez and Coles2005: 480–81). By the mid sixth century, these records show a further steep decline across Western Europe. This possibly reflects the first ravages of the Justinianic plague pandemic and sudden climate change attributable to the ‘Late Antique Little Ice Age’, with commensurate decline in demand (Büntgen et al. Reference Büntgen, Myglan, Ljungqvist, McCormick, Di Cosmo, Sigl, Jungclaus, Wagner, Krusic, Esper, Kaplan, de Vaan, Lutterbacher, Wacker, Tegel and Kirdyanov2016: 234). From around AD 600, the discrete ICP-MS measurements show detailed patterns of fluctuating lead deposition at Colle Gnifetti.
A significant increase in lead aerosol pollution is evident from the mid AD 620s, which peaks in 640±10 (at 639; see OSM S2.2, but between 630 and 650 with the ±10-year error margin, see Figure 4). Lead deposition then reaches two further peaks in AD 660±10 and 695±10. Measurements of bismuth and sulphur were compared with the lead record to ascertain whether their probable origin was anthropogenic or volcanic. Both bismuth and sulphur occur in Western European lead-silver galena ores and are released as by-products during the smelting and cupellation processes (L'Héritier et al. Reference L'Héritier, Baron, Cassayre and Téreygeol2015: 58–59). They can, however, also be released with lead during volcanic eruptions. Most importantly, the lead peaks in AD 640 and 660 do not mirror high bismuth measurements and are, therefore, probable anthropogenic signals (Figure 4).

Figure 4. Changing pollution deposition by concentration (log scale) of lead, bismuth and sulphur in the Colle Gnifetti ice core, between AD 600 and 875, from high-resolution discrete ICP-MS (figure by H. Clifford).
The sudden reappearance of large anthropogenic lead peaks between AD 640 and 660±10 implies significant new smelting of galena ores in one or more regions capable of delivering pollution to the north-facing slopes of the Alps. Atmospheric circulation modelling using Climate Re-analyzer™ and averaged data from June, July and August 1979–2014 (Figure 5) suggests that air mass delivery to the drill site was most probably from the west. The closest source region lies in modern-day France. Other climate analyses over shorter durations have also shown the probability of delivery of aerosol pollutants from Britain to Colle Gnifetti (More et al. Reference More, Spaulding, Bohleber, Handley, Hoffmann, Korotkikh, Kurbatov, Loveluck, Sneed, McCormick and Mayewski2017: fig. 4). The circulation patterns suggest a reduced probability of pollution from Spain or known areas of later medieval mining, such as Sardinia and Tuscany. It has not yet been possible to measure lead isotopes to attempt to distinguish lead mining sources, due to the small size of the ice samples from the Colle Gnifetti core. While isotope analysis of peat and lake sediments has been possible, sourcing has been fraught with difficulties. Similar geology among different source regions within Europe often produces lead metal and aerosol pollution with overlapping isotope characteristics, rendering the sources indistinguishable. Furthermore, scrap metal from different origins was added during cupellation and casting, thereby mixing isotopic signatures (Baron et al. Reference Baron, Le-Carlier, Carignan and Ploquin2009; Pedersen et al. Reference Pedersen, Andersen, Simonsen and Erambert2016: 157–59).

Figure 5. Climate Re-analyzer™ model showing air-mass delivery from the west to Colle Gnifetti, between June, July and August 1979–2014 (CCI).
To date, only one place west of Colle Gnifetti has yielded archaeological evidence supported by radiocarbon dates that demonstrates mid seventh-century AD mining and smelting of silver-lead galena ore. That place is the site of the paramount silver mines of Western Europe prior to the tenth century, located at Melle (Deux-Sèvres, France; Figure 6). Thousands of coins were produced by the miners and moneyers at Melle during its ninth-century zenith (Bruand Reference Bruand2002; Coupland Reference Coupland, Naismith, Allen and Screen2014). Twenty years of research at Melle have produced a firm understanding of the mine galleries, and of the ore-processing, -smelting and -cupellation methods used to separate silver from lead (Téreygeol Reference Téreygeol and Henning2007). Twenty-six radiocarbon dates from charcoal range from the fifth to the later tenth centuries, although the majority of the trees converted into charcoal date from the early seventh century onwards (Téreygeol Reference Téreygeol and Bourgeois2010: 253, fig. 2). Isotopic studies show that different mints across western France struck numerous pennies that used silver from Melle from a point in time dated numismatically to c. AD 675 onwards (Clairand & Téreygeol Reference Clairand, Téreygeol, Clairand and Hollard2009; Téreygeol Reference Téreygeol and Bourgeois2010: 253–59; Figures 6–7).

Figure 6. Places and sites discussed in the text with archaeological or textual evidence relating to the period, c. AD 640–725 (figure by S. Troadec & C.P. Loveluck).

Figure 7. Penny minted at Melle (left: obverse; right: reverse), probably struck from c. 660. Found at Ashford, Kent (EMC 2016.0039, image courtesy of T. Abramson).
An origin from Melle for the seventh-century lead pollution finds further support in the close correspondence between the lead deposition patterns and known smelting and minting at Melle during the Carolingian period, particularly from c. AD 790–875. Figure 4 shows ice-core lead peaks at AD 805±10 and 825±10, dates which fall within the numismatic chronology for the minting of the Metullo coinage issue of Charlemagne at Melle, between AD 793 and 814 (Coupland Reference Coupland2015: 61), and the Melle issues of his son, Emperor Louis the Pious, between AD 816 and 823 (Coupland Reference Coupland, Naismith, Allen and Screen2014: 265–68). The sharp drop in lead deposition in AD 850±10 mirrors the recorded sack of Melle by Vikings in AD 848 and a civil war in the region between Charles the Bald and Pepin of Aquitaine during the mid 850s (Grat et al. Reference Grat, Vieillard and Clémencet1964: 55; Nelson Reference Nelson1992: 162–64, 171–74, 209–12). The subsequent peak at AD 860±10 fits with renewed minting of Charles's Metullo coinage at Melle in the 860s, prior to the political and economic fragmentation of the west Frankish kingdom following his death in 877. The correspondence between known minting and political events affecting Melle and the Colle Gnifetti ice-core record is startling, even with the time error margin of the discrete ICP-MS data. It reinforces the probability that Melle was the source for the seventh-century peaks.
Mining regions to the west of Colle Gnifetti, in Spain and France, have not yet yielded high-resolution evidence of silver-lead ore smelting between c. AD 550 and 700 (Kylander et al. Reference Kylander, Weiss, Martinez-Cortizas, Spiro, Garcia-Sanchez and Coles2005; Martinez-Cortizas et al. Reference Martinez-Cortizas, López-Merino, Bindler, Mighall and Kylander2013). Only in one case—at Lake Redó in the Pyrenees—do sediments show a peak in lead concentration at c. AD 660. These, however, were not deposited in annual varve layers, and so are dated absolutely within the radiocarbon error margin (approximately ±150 years when stated, with a rise in lead beginning in c. AD 467 and ending in c. 1105); there are also high levels of lead in local granitic rocks around Redó (Camarero et al. Reference Camarero, Masqué, Devos, Ani-Ragolta, Catalan, Moor, Pla and Sanchez-Cabeza1998: 442–44). The earliest radiocarbon dates from archaeological surveys of over 70 lead mining/smelting sites, and peat evidence from central and southern France, show new lead mining only from the eleventh to thirteenth centuries onwards (Baron et al. Reference Baron, Lavoie, Ploquin, Carignan, Pulido and De Beaulieu2005, Reference Baron, Carignan, Laurent and Ploquin2006; Bailly-Maître et al. Reference Bailly-Maître, Minivielle-Larousse, Kammenthaler, Gonon and Guionova2013). In Britain, the surprising wealth in silver and gold within furnished graves in the Peak District (Derbyshire), from c. AD 640–660, may hint at contemporaneous lead and silver extraction (Loveluck Reference Loveluck, Bintliff and Hamerow1995: 90). Currently, however, the earliest unambiguous early medieval evidence for lead production in Britain comes from an Anglo-Norman rendition of a charter dated to AD 835, when an aristocrat, Humberht, was obliged to pay a rent in lead for land he held at Wirksworth (Kelly & Brooks Reference Kelly and Brooks2013: 636–38; Figure 6). Exploitation of silver-lead sources in Germany cannot be proven until the ninth century, when very limited mining occurred in the Harz Mountains region, prior to its later zenith from c. AD 950–1050 (Kempter & Frenzel Reference Kempter and Frenzel2000: 100).
Context, purpose and implications of new silver production, c. AD 640–680
The lead peak dated to c. AD 640±10 at Colle Gnifetti occurred at a crucially important moment in the post-Roman monetary history of Western Europe. A recent archaeometric study has confirmed that, beginning in the reign of Dagobert I (AD 629–639), the gold content of the tremissis coinage issued in the Merovingian kingdoms of Gaul declined progressively and substantially. This was due to the intentional addition of silver, and is unambiguously shown by the concurrent presence of significant amounts of lead in the coinage arising from galena ore processed to extract new silver (Blet-Lemarquand et al. Reference Blet-Lemarquand, Bompaire and Morrisson2010: 189). Measurements by proton activation analysis (PAA, 35 coins) and by LA-ICP-MS (9 coins, one of which was also assayed by PAA) have indicated that coins struck by the Provence and Banassac mints declined from 92–94 per cent gold to 31–61 per cent at c. AD 640 (Blet-Lemarquand et al. Reference Blet-Lemarquand, Bompaire and Morrisson2010: 179–82). The swiftness and extent of this change argue against a measure to compensate for the gradual depletion of gold content that might accompany ‘wear and tear’ and repeated recycling, or a diminishing gold supply (Grierson & Blackburn Reference Grierson and Blackburn1986: 95, 109). Instead, they suggest a conscious decision to increase the amount of gold, albeit alloyed with silver, available for striking. Expanding money supply may, in turn, hint at an increased demand for coinage—a consideration that numismatists could test by estimating from die patterns whether the volume of production did indeed increase in these years (Metcalf Reference Metcalf2006: 383–88). The end of Byzantine gold subsidies to the Frankish kings c. AD 600—exacerbated by declining or less favourable Mediterranean trade from the AD 620s—has been suggested as the trigger for the sudden adulteration with silver of the Merovingian gold coinage, a situation also observed in Anglo-Saxon England c. AD 640 (Blet-Lemarquand et al. Reference Blet-Lemarquand, Bompaire and Morrisson2010: 191, 195; Williams Reference Williams2010: 58). In any event, the new alloy coins used silver, and the Colle Gnifetti ice core confirms a surge in lead pollution in c. AD 640±10. This reflects lead-silver freshly mined and smelted to produce at least a proportion of this new coinage.
The second large seventh-century peak at 660±10 occurs when pure silver issues replaced gold-silver alloy coinage. Numismatists have estimated that the first silver coinage in the western Merovingian kingdoms was minted c. AD 675 (Depeyrot Reference Depeyrot2001: 6; Lafaurie & Pilet-Lemière Reference Lafaurie and Pilet-Lemière2003: 11). If, as seems probable, Ebroin—the ‘mayor of the palace’—decided on the shift to silver, the change will have occurred between c. AD 658 and his death in c. 680 (Ewig Reference Ewig1986); the new coins are mentioned in texts from 682 (Grierson & Blackburn Reference Grierson and Blackburn1986: 94). The use of the earliest silver coins—known today as deniers in France (here called pennies)—could conceivably have overlapped with the last use of the gold-silver alloy issues (McCormick Reference McCormick, Bompaire and Sarah2018: 439). Numismatists have also suggested a contemporaneous change in England c. 675–80, from the use of debased Anglo-Saxon gold coins to silver replacements, known as sceattas (Archibald Reference Archibald, Hines and Bayliss2013; Gannon Reference Gannon2013: 93–97). A new chronology for dating Anglo-Saxon furnished graves, involving high-resolution carbon-dating, artefact seriations and Bayesian modelling, however, challenges the numismatic date for the first sceattas; the earliest sceattas (almost exclusively series B) from graves were dated by the modelling to c. AD 660 (Hines & Scull Reference Hines, Scull, Hines and Bayliss2013; Scull & Naylor Reference Scull and Naylor2016: 213–14).
An association between the radiocarbon-based Bayesian date for the first Anglo-Saxon silver coins (c. AD 660) and contemporaneous lead-silver smelting, most probably occurring at Melle in France, probably reflects an almost simultaneous minting of silver coinage in France and England from the early AD 660s. Its immediate economic and social impact may have been limited by both geography and the range of social actors who found it useful. Merovingian mints concentrated in three regions: around the Mediterranean ports of Arles and Marseilles; within central-western France, between Melle, Poitiers and Tours; and in the Paris basin, extending into upper Burgundy (Depeyrot Reference Depeyrot2001; Kluge Reference Kluge, Jarnut and Strothmann2013; Figure 6). Although the minting location(s) of series B sceattas are currently unknown, their initial use was largely confined to south-eastern England. Deposition of these early silver coinages soon concentrated in the key exchange zones of the Channel and southern North Sea coasts of Europe. Early Merovingian pennies have been found at the seasonal beach-trading settlement at Domburg, Walcheren, in the Scheldt estuary (Dhénin & Schiesser Reference Dhénin and Schiesser2007: 294), and in the south-east of England (Figure 7). Series B sceattas occur in small numbers in the Pas-de-Calais and Picardy; and in the vicinity of the port of Quentovic (Leroy Reference Leroy and Soulat2009: 201); they are also found in a hoard of mainly Merovingian pennies, buried in c. AD 720 at Cimiez, near Nice, on the Mediterranean coast of France (Grierson & Blackburn Reference Grierson and Blackburn1986: 142–43; Figure 6). Key features of these silver coinages were their high silver content and close similarity in weight (Metcalf & Northover Reference Metcalf and Northover1989: 106), making them interchangeable from the North Sea to the Mediterranean.
Conclusions
Preliminary analysis of the Colle Gnifetti ice core, using ultra-high-resolution layer-counting chronology, high-resolution analysis of pollution proxies for metal production and atmospheric circulation modelling to assess source regions, permits a new appreciation of macro-economic and societal change during the seventh century AD. Integrated analysis of the ice-core data with other environmental, archaeological, numismatic and textual records from Western Europe has identified a pollution record c. AD 640±10 and 660±10, probably stemming from new, large-scale silver mining and bullion production in those years. The evidence indicates that the mines at Melle in western France were the most probable source. The Colle Gnifetti ice core provides a chronology independent of archaeological and numismatic dates for the two major monetary transformations of seventh-century North-west Europe. In the early AD 660s, the port settlements of the Channel and North Sea were in their infancy, whereas by the 680s, multiple lines of evidence show that the large ports at London (Lundenwic) and Quentovic were firmly established (Bede HE IV.22; Colgrave & Mynors Reference Colgrave and Mynors1969; Malcolm et al. Reference Malcolm, Bowsher and Cowie2003; Lebecq et al. Reference Lebecq, Béthouart and Verslype2010). The striking of silver coinage from c. AD 660, rather than from c. 675–680 as previously suggested by numismatists, allows this more utilitarian silver currency an extra 15–20 years of use within the mercantile agency that made these ports vibrant by c. 685. Combined with ongoing advances in numismatic studies and archaeological research, high-precision environmental records provide a unique new insight into the dynamics of metal and coin production, and contemporaneous social transformations in the early medieval West.
Acknowledgements
This is contribution six of the ‘Historical Ice Core’ project funded by the Arcadia Fund (AC3862) of Peter Baldwin and Lisbet Rausing, whose support we gratefully acknowledge. C.L. also thanks the History Department, Dumbarton Oaks, and the Initiative for the Science of the Human Past, Harvard University, for funding a Visiting Professorship for this research, and the University of Nottingham. Ice-core and tephra analyses were conducted at the W.M. Keck Laser Ice Facility and ICP-MS laboratory at CCI, funded by the W.M. Keck Foundation and the National Science Foundation (PLR-1042883, PLR-1203640, PLR-1142007). We also thank Pascal Bohleber for his research on the ice-core chronology. The Colle Gnifetti core was collected by a team from the Institut für Umweltphysik, Universität Heidelberg, CCI-University of Maine and the Climate and Environmental Physics Institute, University of Bern, with support from the Alfred-Wegener-Institute, Bremerhaven. Thanks are also due to Solenn Troadec, Martin Allen and Tony Abramson.
Supplementary material
To view supplementary material for this article, please visit https://doi.org/10.15184/aqy.2018.110