Introduction
Over the past 40 years, ART has been steadily on the rise, allowing millions of infertile couples to conceive. Currently, it has been estimated that ∼9 million children have been conceived globally following ART, and in some European countries, it represents ∼5% of all births (De Geyter et al., Reference De Geyter, Calhaz-Jorge, Kupka, Wyns, Mocanu, Motrenko, Scaravelli, Smeenk, Vidakovic and Goossens2020; European IVF Monitoring Consortium (EIM), 2022). Infertility problems affect ∼15% of couples and represent the main driver of IVF utilization. However, there is currently a continuous rise in the number of individuals who freeze their eggs or embryos (European IVF Monitoring Consortium (EIM), 2022) for future use, with an estimation of 309,475 frozen embryo transfers (FET) performed only in Europe in 2018 (European IVF Monitoring Consortium (EIM), 2022; Table 1). ART is generally considered safe; however, several reports have described an association between ART and an increased incidence of low birth weight, birth defects, altered growth and metabolic disorders (Hart and Norman, Reference Hart and Norman2013; Ventura-Juncá et al., Reference Ventura-Juncá, Irarrázaval, Rolle, Gutiérrez, Moreno and Santos2015). These findings might be secondary to epigenetic dysregulation of gametes and embryos, which potentially can be transferred to the future generation (Hirasawa and Feil, Reference Hirasawa and Feil2010; Smith et al., Reference Smith, Chan, Humm, Karnik, Mekhoubad, Regev, Eggan and Meissner2014; Vrooman and Bartolomei, Reference Vrooman and Bartolomei2017) Embryo cryopreservation by ‘slow freezing’ started in the 1980s (Chen, Reference Chen1986) and later was replaced by the ‘vitrification’ protocol (Kuwayama et al., Reference Kuwayama, Vajta, Kato and Leibo2005) that has been considered a real breakthrough in ART (Potdar et al., Reference Potdar, Gelbaya and Nardo2014; Rienzi et al., Reference Rienzi, Gracia, Maggiulli, LaBarbera, Kaser, Ubaldi, Vanderpoel and Racowsky2017) Practically, cryopreservation enables the long-term preservation of tissue or cells at ultra-low temperatures in a state of suspended animation. This state can be obtained by vitrification, using a high concentration of cryoprotective agents (CPAs) to increase viscosity and inhibit the ice crystals formation, and finally induce the solution to enter a ‘glassy state’ (Hubel et al., Reference Hubel, Spindler and Skubitz2014). One of the most used CPAs is dimethyl sulfoxide (DMSO): an amphipathic chemical compound. Exposure to this molecule might cause unexpected changes in cellular functions and metabolism, enzyme activities, cell growth and apoptosis, as well as might induce alterations in microRNAs (miRNA) and epigenetic changes (Santos et al., Reference Santos, Figueira-Coelho, Martins-Silva and Saldanha2003; Iwatani et al., Reference Iwatani, Ikegami, Kremenska, Hattori, Tanaka, Yagi and Shiota2006; Verheijen et al., Reference Verheijen, Lienhard, Schrooders, Clayton, Nudischer, Boerno, Timmermann, Selevsek, Schlapbach, Gmuender, Gotta, Geraedts, Herwig, Kleinjans and Caiment2019) Animal studies have suggested that oocyte cryopreservation can be associated with increased levels of reactive oxygen species (ROS) and apoptotic events (Kohaya et al., Reference Kohaya, Fujiwara, Ito and Kashiwazaki2013; Zhao et al., Reference Zhao, Hao, Du, Zhao, Wang, Wang, Wang, Liu, Qin and Zhu2016; Christou-Kent et al., Reference Christou-Kent, Dhellemmes, Lambert, Ray and Arnoult2020) that might alter epigenetic mechanisms correlated with oocyte competence and subsequent embryo viability (Sendžikaitė and Kelsey, Reference Sendžikaitė and Kelsey2019; Christou-Kent et al., Reference Christou-Kent, Dhellemmes, Lambert, Ray and Arnoult2020) Therefore, the vitrification protocol and eventually the consequences of chilling might impair genome integrity and transcripts stability, and potentially affect the future offspring health (Albertini and Olsen, Reference Albertini and Olsen2013; Sendžikaitė and Kelsey, Reference Sendžikaitė and Kelsey2019) Therefore, given the increasing number of babies conceived after embryo vitrification, it is critical to clarify and established that cryopreservation is not harmful to the future health of children. In this manuscript, we will illustrate the current state of knowledge of the effect that vitrification and high concentration of cryoprotectants might have on potential epigenetic modification and consequences for future offspring health.
Table 1. Main indications for application of human embryo cryopreservation

Signs on cryopreservation
The cryopreservation protocol permits cell or tissue freezing and subsequent storage in liquid nitrogen (−196°C), to interrupt all biological activities and maintain their viability and physiological competencies for future use. The first report of live birth following the transfer of a cryopreserved–thawed embryo was recorded in Australia by Trounson and Mohr in 1983 through the ‘slow freezing’ procedure (Trounson and Mohr, Reference Trounson and Mohr1983) Later in the 1990s, a great advancement in the field was achieved with the introduction of the ‘vitrification’ method in Japan and Australia (Mukaida et al., Reference Mukaida, Wada, Takahashi, Pedro, An and Kasai1998; Kuwayama et al., Reference Kuwayama, Vajta, Kato and Leibo2005; Li et al., Reference Li, Wang, Ledger, Edgar and Sullivan2014; Rienzi et al., Reference Rienzi, Gracia, Maggiulli, LaBarbera, Kaser, Ubaldi, Vanderpoel and Racowsky2017; Sciorio et al., Reference Sciorio, Thong and Pickering2018, Reference Sciorio, Thong and Pickering2019) The success of vitrification is correlated with several factors, such as the temperature at the vitrification and warming steps, which depends on the choice of the carrier used (open and or closed vitrification), and most importantly the concentration and the type of CPAs used (Table 2). Seki and Mazur have demonstrated how the cryo-damage might also be induced by re-crystallization at the warming step, reporting that the warming rate is as important as the cooling rate. They examined the relationship between cooling versus warming rates in a mouse model and concluded that a warming rate of at least 3000°C/minute was imperative to obtain an acceptable survival rate above 80% (Seki and Mazur, Reference Seki and Mazur2009). The choice and concentration of CPAs play a critical role in cryopreservation success. They are supplemented to the vitrification medium to defend cells from the cryo-injuries and are classified into two categories: ‘permeating and non-permeating’ (Figure 1). The first group includes small molecular weight compounds, (less than 400 Da) that can cross cell membranes and once inside protect the cell from cryo-induced damage. This group includes ethylene glycol (EG), dimethyl sulfoxide (DMSO), propylene glycol or 1,2 propanediol (PG), glycerol (GLY), formamide (FMD), methanol (METH), and butanediol (BD; 2,3-butanediol). DMSO and glycerol are the two most used (Table 3). Non-penetrating CPAs are non-diffusible, normally have a higher molecular weight, and therefore cannot cross the cell membrane. Examples are trehalose, sucrose, glucose, mannitol, galactose, and polyvinylpyrrolidone (PVP). They have a high molecular weight and are able to induce an osmotic gradient that removes water from inside to outside the cell (dehydration), reducing the temperature at which ice starts to form, and preserving membranes and intracellular structures (Karlsson and Toner, Reference Karlsson and Toner1996; Fuller, Reference Fuller2004).
Table 2. Membrane permeability coefficient of some cryoprotectants (Times 10 -5cm/s)

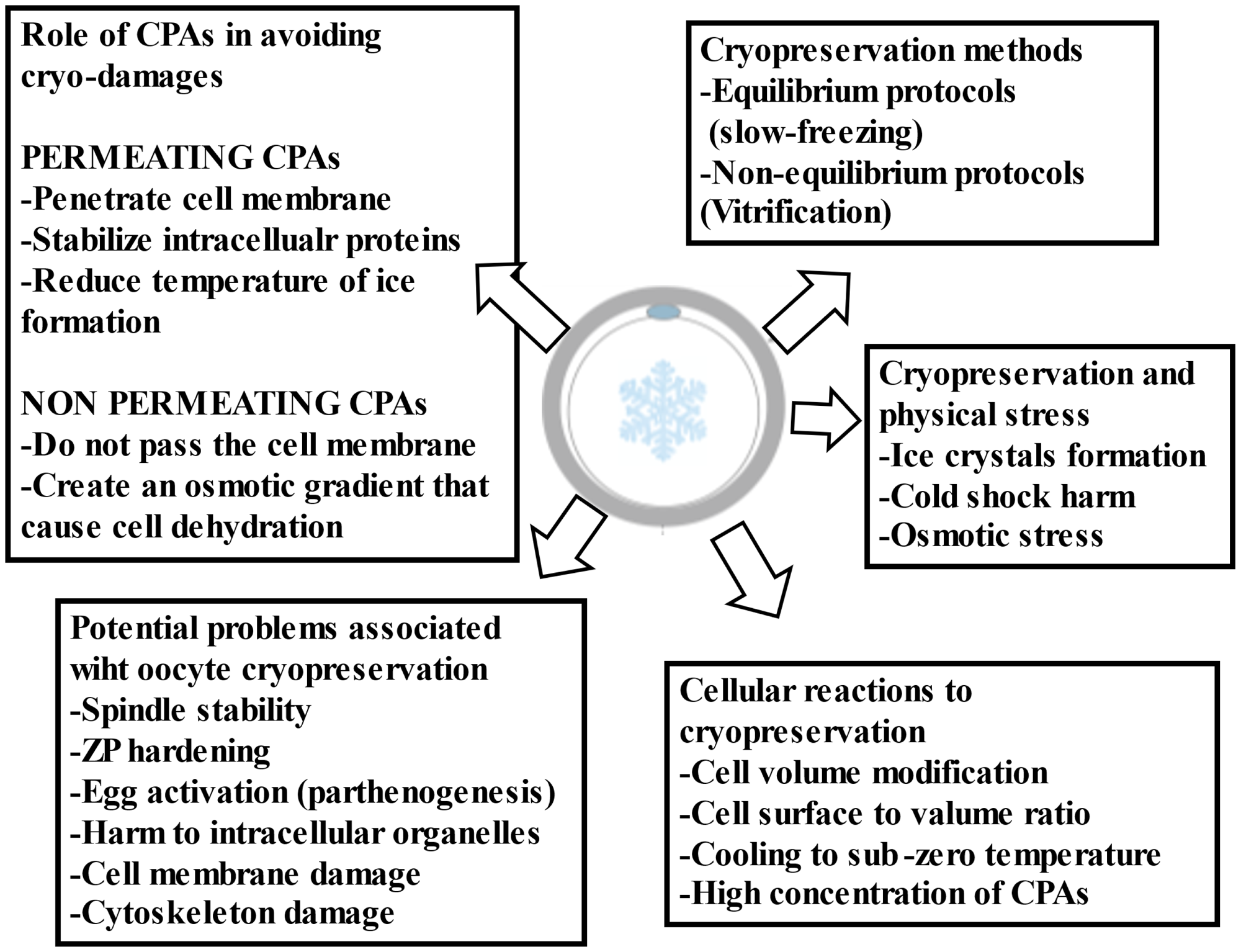
Figure 1. General criticalities associated with oocyte cryopreservation.
Table 3. Minimal concentration required to vitrify (C-Vit) for some permeating cryoprotectants at pressure of 1 atmosphere according to Fahy and colleagues 1984 (Fahy et al., Reference Fahy, MacFarlane, Angell and Meryman1984)

Potential cryo-damage associated with the vitrification procedure
The principal physical feature to address with cryopreservation is the formation of ice crystals. Human embryo and even more so the oocyte contains a high content of water, which might be converted to ice causing irreversible damage and death. This concern has been elegantly described by Mazur (Mazur, Reference Mazur1963) The sharp reduction in temperature, might also lead to cold-shock harm and impair the function of several sensitive structures located in the oocyte cytoplasm, including membrane permeability, cytoskeleton architecture and importantly the meiotic spindle (MS) apparatus (Smith and Silva E Silva, Reference Smith and Silva E Silva2004; Best, Reference Best2015) The MS is a cytoskeletal structure, formed from microtubules and associated proteins, and considered an indicator of oocyte health; its stability is linked with normal fertilization and is directly responsible for the correct segregation of chromosomes, avoiding errors in chromatin division, accountable for aneuploidies and miscarriage rate (Feuer and Rinaudo, Reference Feuer and Rinaudo2012; Dal Canto et al., Reference Dal Canto, Guglielmo, Mignini Renzini, Fadini, Moutier, Merola, De Ponti and Coticchio2017) It is well established that temperature changes can debilitate MS stability (Wang et al., Reference Wang, Meng, Hackett, Oldenbourg and Keefe2002) At a temperature of 33°C or lower, the MS starts to depolymerize, and only a few minutes of exposure to non-physiologic pH or temperature are sufficient to induce the spindle to disassemble (Montag and van der Ven, Reference Montag and van der Ven2008) Several studies on both animals and humans have demonstrated a negative association between temperature and spindle alterations (Pickering et al., Reference Pickering, Braude, Johnson, Cant and Currie1990; Wang et al., Reference Wang, Meng, Hackett, Oldenbourg and Keefe2002; Montag and van der Ven, Reference Montag and van der Ven2008; Feuer and Rinaudo, Reference Feuer and Rinaudo2012) Additional impairment following cooling and warming may be depicted by a premature hardening of the zona pellucida (ZP), which is indispensable for physiologically support fertilization and prevent polyspermy (Pickering et al., Reference Pickering, Braude, Johnson, Cant and Currie1990; Larman et al., Reference Larman, Sheehan and Gardner2006) Additional biological functions that might be harmed by the cryopreservation process include cryo-damage to intracellular organelles, as well as being described an increased risk of parthenogenetic activation of the oocytes (Gook et al., Reference Gook, Osborn and Johnston1995) Oocyte exposure to CPAs might cause ultrastructural modification of mitochondria and of the smooth endoplasmic reticulum complex, which are involved in the physiological mechanism of calcium signalling triggered by the sperm entrance at the time of fertilization (Jones et al., Reference Jones, Van Blerkom, Davis and Toledo2004; Katkov et al., Reference Katkov, Kim, Bajpai, Altman, Mercola, Loring, Terskikh, Snyder and Levine2006; Diaferia et al., Reference Diaferia, Dessì, DeBlasio and Biunno2008; Gualtieri et al., Reference Gualtieri, Iaccarino, Mollo, Prisco, Iaccarino and Talevi2009; Kader et al., Reference Kader, Agarwal, Abdelrazik, Sharma, Ahmady and Falcone2009; Cheng et al., Reference Cheng, Fu, Zhang, Jia, Hou and Zhu2014; Kopeika et al., Reference Kopeika, Thornhill and Khalaf2015) As vitrification becomes widely used in modern ART cycles, it is extremely important to examine whether its use is associated with potential genomic and epigenetic dysregulation (Bouquet et al., Reference Bouquet, Selva and Auroux1993; Katkov et al., Reference Katkov, Kim, Bajpai, Altman, Mercola, Loring, Terskikh, Snyder and Levine2006; Xu et al., Reference Xu, Cowley, Flaim, James, Seymour and Cui2010; Wagh et al., Reference Wagh, Meganathan, Jagtap, Gaspar, Winkler, Spitkovsky, Hescheler and Sachinidis2011; Estill et al., Reference Estill, Bolnick, Waterland, Bolnick, Diamond and Krawetz2016; Li et al., Reference Li, Feng, Gu, He and Wei2017; Cui et al., Reference Cui, Dong, Lyu, Zheng and Ai2021).
Main application of embryo vitrification in ART practice
Embryo cryopreservation plays an active role in ART, with ∼310,000 FET cycles completed only in Europe in 2018 (Table 1; European IVF Monitoring Consortium (EIM), 2022). If in the past embryo cryopreservation was only adopted to store a surplus number of embryos following the fresh transfer, at this time, elective embryo cryopreservation includes fertility preservation for cancer patients when these women are in a stable relationship (Somigliana et al., Reference Somigliana, Viganò, Filippi, Papaleo, Benaglia, Candiani and Vercellini2015; Sciorio and Anderson, Reference Sciorio and Anderson2020) Preimplantation genetic testing also relies on embryo cryopreservation and overcomes the time interval between blastocyst biopsy and genetic result (Coates et al., Reference Coates, Kung, Mounts, Hesla, Bankowski, Barbieri, Ata, Cohen and Munné2017). As well, in cases of other medical reasons such as severe endometriosis or elevated progesterone in the late follicular phase, which has been reported to have a negative effect on pregnancy outcomes, cryopreservation of all available embryos has been suggested so that FET could be performed in a future cycle (Santos-Ribeiro et al., Reference Santos-Ribeiro, Polyzos, Haentjens, Smitz, Camus, Tournaye and Blockeel2014). To prevent the risk of ovarian hyperstimulation syndrome, a potentially life-threatening complication, FET cannot be performed (Sullivan et al., Reference Sullivan, Wang, Hayward, Chambers, Illingworth, McBain and Norman2012; Groenewoud et al., Reference Groenewoud, Cohlen and Macklon2018; Sciorio and Esteves, Reference Sciorio and Esteves2020). Finally, culturing embryos until the blastocyst stage, and vitrifying every single blastocyst for future use, represents a valid alternative to reduce the incidence of multiple pregnancies, which is one of the most critical complications of ART. This application has been reported in several studies by others (Groenewoud et al., Reference Groenewoud, Cohlen and Macklon2018; Sciorio et al., Reference Sciorio, Thong and Pickering2018, Reference Sciorio, Thong and Pickering2019; Liebermann, Reference Liebermann2021).
Oocyte vitrification in the ART programme
The advancements in cryopreservation technology can be perfectly applied in fertility preservation for both social reasons and for cancer patients. Social modifications drive women to look for pregnancy in their late thirties or early forties when age-related issues significantly reduce the chance of success. Therefore, for that group of women, it is considered a valid option to undergo ovarian stimulation to induce multiple gametes production, and freeze all the mature oocytes collected, to be used in the future when the carrier will be established or when the right partner is found. The same procedure can be applied to cancer patients. Unfortunately, currently, cancer diseases are much more common compared with in the past, and those malignancies, including breast cancer, and cervical and ovarian cancer, might negatively affect future fertility (Stearns et al., Reference Stearns, Schneider, Henry, Hayes and Flockhart2006; Sung et al., Reference Sung, Ferlay, Siegel, Laversanne, Soerjomataram, Jemal and Bray2021). A study by Cobo and colleagues examined the pregnancy outcome in fertility preservation after oocyte freezing for age-related fertility decline and for patients before cancer treatment. The authors reported that overall oocyte survival was comparable between the two groups, but implantation, ongoing pregnancy and live birth rates were lower in cancer patients (Cobo et al., Reference Cobo, García-Velasco, Domingo, Pellicer and Remohí2018). A live birth rate of 61.9% was reported from 12 cryopreserved oocytes in women ≤35 years, and 43.4% from 10 oocytes in those >35 years, illustrating the importance of both the number of oocytes that can be collected and cryopreserved. Another application of oocyte cryopreservation has been observed in the donation programme. This practice has increased in the last decade worldwide, as a result of a high prevalence of age-related infertility. Currently, oocyte donation (OD) is well established for age-related female infertility, in which the oocyte quality is compromised (Adams et al., Reference Adams, Clark, Davies and De Lacey2016). In the USA, OD treatments increased by more than 100% in the last decade, from 10,801 in the year 2000 to 24,300 in 2016 (Sauer and Kavic, Reference Sauer and Kavic2006). Similar results have been reported by the European Society of Human Reproduction and Embryology (ESHRE) (De Geyter et al., Reference De Geyter, Calhaz-Jorge, Kupka, Wyns, Mocanu, Motrenko, Scaravelli, Smeenk, Vidakovic and Goossens2020) The first successful pregnancy obtained in a recipient woman following OD was described by Trouson and colleagues in Australia (Trounson et al., Reference Trounson, Leeton, Besanko, Wood and Conti1983). Since then, OD has become more commonly accepted and it has been used for severe female infertility, such as repeated implantation failure, recurrent miscarriages, and premature ovarian failure or for women of advanced maternal age (Sauer and Kavic, Reference Sauer and Kavic2006; Perheentupa and Huhtaniemi, Reference Perheentupa and Huhtaniemi2009; Cobo et al., Reference Cobo, Garrido, Pellicer and Remohí2015) However, it is sometimes difficult to complete the donation of fresh oocytes due to the lack of donors, as a result ART units have started to cooperate with oocyte banks located even abroad to manage the high demand for OD. Therefore, in this setting, the oocyte bank performs the egg retrieval and cryopreservation of these gametes, which can be lately transported to the receiving clinic (Figure 2). Then, the imported oocytes, in the IVF laboratory of the recipient centre, are warmed, and fertilized with the ICSI technique using fresh or frozen sperm, followed by embryo culture and transfer to the recipient’s uterus (Cobo et al., Reference Cobo, Meseguer, Remohí and Pellicer2010; Debrock et al., Reference Debrock, Peeraer, Fernandez Gallardo, De Neubourg, Spiessens and D’Hooghe2015; Rienzi et al., Reference Rienzi, Cimadomo, Maggiulli, Vaiarelli, Dusi, Buffo, Amendola, Colamaria, Giuliani, Bruno, Stoppa and Ubaldi2020). Much evidence has been published on the efficiency of oocyte vitrification even in OD cycles (Cobo et al., Reference Cobo, Meseguer, Remohí and Pellicer2010, Reference Cobo, Garrido, Pellicer and Remohí2015; Rienzi et al., Reference Rienzi, Romano, Albricci, Maggiulli, Capalbo, Baroni, Colamaria, Sapienza and Ubaldi2010, Reference Rienzi, Cimadomo, Maggiulli, Vaiarelli, Dusi, Buffo, Amendola, Colamaria, Giuliani, Bruno, Stoppa and Ubaldi2020; Debrock et al., Reference Debrock, Peeraer, Fernandez Gallardo, De Neubourg, Spiessens and D’Hooghe2015; Sciorio et al., Reference Sciorio, Antonini and Engl2021) One study was performed by Rienzi and colleagues, who demonstrated that oocyte vitrification provided high survival rates after warming, and similar pregnancy outcomes compared with cycles with fresh oocytes (Rienzi et al., Reference Rienzi, Romano, Albricci, Maggiulli, Capalbo, Baroni, Colamaria, Sapienza and Ubaldi2010). Similar results were described by Cobo and collaborators in a vast study that counted ∼3500 oocytes donation cycles (Cobo et al., Reference Cobo, Garrido, Pellicer and Remohí2015).
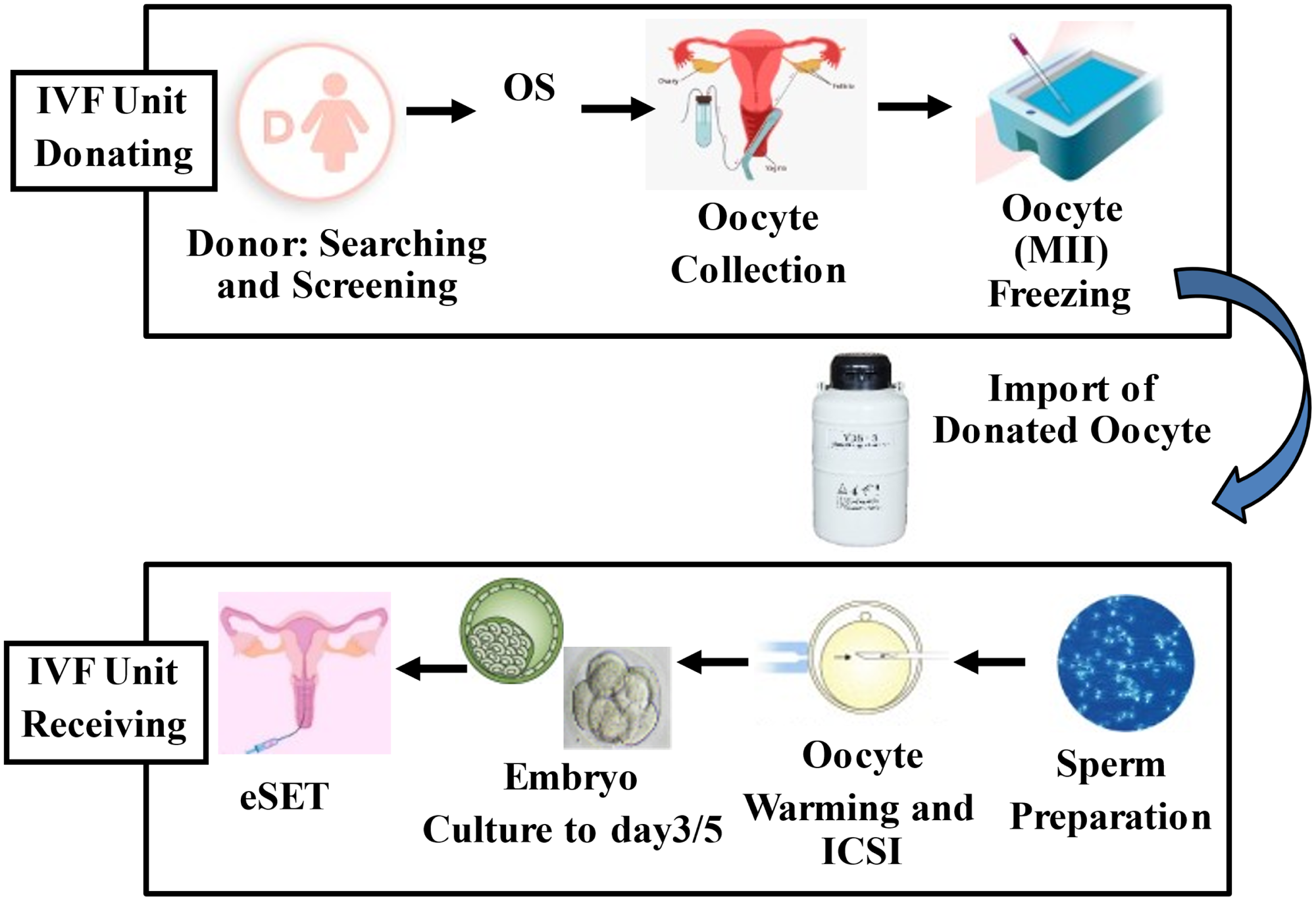
Figure 2. Description of the imported oocyte donation programme from a foreign oocyte bank. eSET, elective single embryo transfer; ICSI, intracytoplasmic sperm injection; MII, metaphase II oocyte; OS, ovarian stimulation.
Signs on epigenetics
Waddington Conrad, a biologist at Edinburgh University in 1942, was the first to underline the importance of environmentally directed changes during the early stages of mammalian embryo development and introduced the term ‘Epigenetics’, which indicates a gene-regulatory mechanism that leads to heritable changes in gene function that are not associated with changes in DNA sequence (Waddington, 2012). For many years it has been thought that the heritability of epigenetic information was only restricted to mitotic cellular division. However, it is now evident that epigenetic processes can be transmitted meiotically in organisms from one generation to another (Russo et al., Reference Russo, Martienssen and Riggs1996). During gametogenesis and early embryo development, sweeping epigenetic modifications are introduced to both male and female inherited chromatin as two terminally differentiated cells (i.e., the spermatozoon and oocyte) that unite to form a totipotent zygote (Figure 3). There are two main epigenetic reprogramming phases: one that reset epigenetic marks in germ cells when primordial germ cells (PGCs) develop and migrate to the fetal gonadal ridge. The second is during the early stage of embryo development, following fertilization; the parental genome experiences an extensive second wave of demethylation (the paternal genome is actively demethylated, and the maternal genome is passively demethylated). This is essential to provide the pluripotency required to develop embryonic lineages and future development (Mak et al., Reference Mak, Weaver and Bartolomei2010; Skinner, Reference Skinner2011; Marcho et al., Reference Marcho, Cui and Mager2015). DNA encased around histones makes the nucleosome formation, which is part of the chromatin pattern (Figure 4). Accordingly, specific DNA disposition will establish if a gene will be transcriptionally expressed or remain silent. Portions of highly compacted DNA are termed heterochromatin and are transcriptionally silent, and tracts that are weakly bonded to histones are defined as euchromatin and are transcriptionally active. Indeed, during early embryo development, the epigenome is highly susceptible to external and internal modification, or any contact with chemical compounds such as CPAs that can alter the reprogramming machinery and cause long-term risk of disease, even in the future generation (Klose and Bird, Reference Klose and Bird2006; Bannister and Kouzarides, Reference Bannister and Kouzarides2011; Skinner, Reference Skinner2011; Marcho et al., Reference Marcho, Cui and Mager2015). Accumulating evidence, much from animal studies, has indicated that this can, in turn, lead to alterations in developmental processes resulting in congenital abnormalities with a longer term predisposition to certain diseases in adulthood (Klose and Bird, Reference Klose and Bird2006; Weaver et al., Reference Weaver, Susiarjo and Bartolomei2009; Bannister and Kouzarides, Reference Bannister and Kouzarides2011; Faulk and Dolinoy, Reference Faulk and Dolinoy2011; Skinner, Reference Skinner2011).

Figure 3. Epigenetic reprogramming during early stage of embryo development. The scheme illustrates the stage of development at which different ART techniques are used. ICSI, intracytoplasmic sperm injection; IVF, in vitro fertilization; OS, ovarian stimulation. Adapted from Sciorio and El Hajj (Reference Sciorio and El Hajj2022).

Figure 4. Diagram depicts the epigenetic marks in the DNA structure.
Epigenetic marks and imprinting genes
DNA methylation is the most investigated epigenetic process and involves the addition of a methyl group at the 5′ carbon position of the cytosine pyrimidine ring in the context of CG dinucleotide (CpG sites). Those epigenetic modifications are maintained by daughter cells throughout cell divisions by DNA methyltransferases (DNMTs). To date, five different types of DNMTs have been identified: Dnmt1, Dnmt2, Dnmt3a, Dnmt3b, and Dnmt3L (Rivera and Ren, Reference Rivera and Ren2013; Gujar et al., Reference Gujar, Weisenberger and Liang2019). DNA methylation is generally correlated with gene silencing, but it is also involved in other regulatory mechanisms such as imprinting or X-chromosome inactivation and silencing of centromeric sequences (Goldberg et al., Reference Goldberg, Allis and Bernstein2007; Rivera and Ren, Reference Rivera and Ren2013; Gujar et al., Reference Gujar, Weisenberger and Liang2019) Additional epigenetic regulations comprise post-translational histone modifications, involving lysine acetylation of histones, which is normally regulated by histone acetyltransferases (HATs) and histone deacetylases (HDACs) (Gallinari et al., Reference Gallinari, Di Marco, Jones, Pallaoro and Steinkühler2007) Acetylation leads to open chromatin configuration, enhances transcriptional activity, and encourages transcription factor binding to DNA. Conversely, deacetylation is correlated with transcriptional inactivation and gene silencing (Liu et al., Reference Liu, Lu, Yang, Fan, Yang, Liu, Korolev and Nordenskiöld2011). An additional mark is represented by the ubiquitination/deubiquitination system, which is relevant for the degradation of proteins, cell cycle progression, transcriptional regulation and oocyte maturation (Swain et al., Reference Swain, Ding, Brautigan, Villa-Moruzzi and Smith2007; Bassermann et al., Reference Bassermann, Eichner and Pagano2014; Mtango et al., Reference Mtango, Latham, Sutovsky and Sutovsky2014). On the same line, SUMOylation and de-SUMOylation marks indicate the addition and removal of SUMO (small ubiquitin-related modifier) polypeptides on lysine residues (Schatten and Sun, Reference Schatten and Sun2014) which are essential for the occurrence of oocyte maturation, meiotic resumption and spindle formation (Schatten and Sun, Reference Schatten and Sun2014; Feitosa et al., Reference Feitosa, Hwang and Morris2018; Rodriguez et al., Reference Rodriguez, Briley, Patton, Tripurani, Rajapakshe, Coarfa, Rajkovic, Andrieux, Dejean and Pangas2019) Furthermore, in the female reproductive system, several proteins such as LH, FSH, AMH, and GDF9 are glycosylated, the mark represents one of the most frequent post-translational modifications, which involves protein folding, activity and stability (Bousfield and Harvey, Reference Bousfield and Harvey2019). Finally, another epigenetic modification has been newly identified as lactylation, which represents a new type of histone mark that can be stimulated by lactate, and directly stimulates gene transcription (Zhang et al., Reference Zhang, Tang, Huang, Zhou, Cui, Weng, Liu, Kim, Lee, Perez-Neut, Ding, Czyz, Hu, Ye, He, Zheng, Shuman, Dai and Ren2019). Collectively, these epigenetic marks play a major role in the specific and complex process of regulation of a small subset of genes defined as ‘imprinted genes’ (Glaser et al., Reference Glaser, Ramsay and Morison2006; Skaar et al., Reference Skaar, Li, Bernal, Hoyo, Murphy and Jirtle2012). Currently, ∼150 genes have been identified in mice, and less than 100 in humans (Glaser et al., Reference Glaser, Ramsay and Morison2006). A list of the current mammalian imprinted genes is available online at http://Igc.Otago.Ac.Nz/Home.html. These genes are characterized by a monoallelic expression that is dependent on the parental origin of the allele. The parental imprint is linked to differential epigenetic labelling of parental alleles and, importantly, is established during gametogenesis, and maintained during the early stage of preimplantation embryo development (Das et al., Reference Das, Lee, Strogantsev, Jin, Lim, Ng, Lin, Chng, Yeo, Ferguson-Smith and Ding2013; Li, Reference Li2013; Thamban et al., Reference Thamban, Agarwaal and Khosla2020). The correct expression of those imprinted genes depicts a critical role in growth, development, and are prevalently located in the placenta and brain (Hiura et al., Reference Hiura, Okae, Chiba, Miyauchi, Sato, Sato and Arima2014; Kalish et al., Reference Kalish, Jiang and Bartolomei2014; Lazaraviciute et al., Reference Lazaraviciute, Kauser, Bhattacharya, Haggarty and Bhattacharya2014; Eggermann et al., Reference Eggermann, Perez de Nanclares, Maher, Temple, Tümer, Monk, Mackay, Grønskov, Riccio, Linglart and Netchine2015) Along these lines, embryonic epigenetic disruptions of imprinting control regions might result in serious consequences and induce imprinting disorders (Kalish et al., Reference Kalish, Jiang and Bartolomei2014). Examples include loss of imprinted DNA methylation at the Kvdmr icr, found in ART-conceived babies with Beckwith–Wiedemann syndrome (BWS) (Azzi et al., Reference Azzi, Abi Habib and Netchine2014) or gain of methylation because of maternal uniparental disomy on chromosome 7 at the Mest icr in ∼10% of Silver–Russell Syndrome (SRS) cases, as well as Angelman syndrome (AS) and Prader–Willi syndrome (DeBaun et al., Reference DeBaun, Niemitz and Feinberg2003; Gicquel et al., Reference Gicquel, Gaston, Mandelbaum, Siffroi, Flahault and Le Bouc2003; Halliday et al., Reference Halliday, Oke, Breheny, Algar and Amor2004; Mabb et al., Reference Mabb, Judson, Zylka and Philpot2011; Cassidy et al., Reference Cassidy, Schwartz, Miller and Driscoll2012; Azzi et al., Reference Azzi, Abi Habib and Netchine2014; Hiura et al., Reference Hiura, Okae, Chiba, Miyauchi, Sato, Sato and Arima2014; Kalish et al., Reference Kalish, Jiang and Bartolomei2014; Lazaraviciute et al., Reference Lazaraviciute, Kauser, Bhattacharya, Haggarty and Bhattacharya2014; Eggermann et al., Reference Eggermann, Perez de Nanclares, Maher, Temple, Tümer, Monk, Mackay, Grønskov, Riccio, Linglart and Netchine2015).
DMSO: toxicity effect and epigenetic aberration
The vitrification protocol currently available uses a high concentration of CPAs: for example, a combination of 15% DMSO associated with 15% EG (Table 3). DMSO as a penetrating cryoprotectant is able to pass the cell membrane, and it enhances the permeability of other low-molecular-weight chemical compounds, thereby making them facilitate the crossing of the membrane or going deeper into the cell (Yu and Quinn, Reference Yu and Quinn1994). DMSO is not only applied in ART but also widely used in other fields such as regenerative and stem cell research and studies have shown that DMSO has temperature, time and concentration-dependent toxic effects (Marks and Breslow, Reference Marks and Breslow2007; Hunt, Reference Hunt2011). Studies have reported that DMSO interferes with the activity of the enzyme DNMT3a, even though the specific mechanism is unknown (Santos et al., Reference Santos, Figueira-Coelho, Martins-Silva and Saldanha2003; Verheijen; et al., Reference Verheijen, Lienhard, Schrooders, Clayton, Nudischer, Boerno, Timmermann, Selevsek, Schlapbach, Gmuender, Gotta, Geraedts, Herwig, Kleinjans and Caiment2019) A study by Verheijen and colleagues investigated the biological effect that DMSO induces on DNA methylation profile in human cardiac microtissues. The pathway analysis of cardiac gene expression showed dysregulation of DNA methylation pathways. Methyltransferases DNMT1, a key factor for maintenance of DNA methylation, as well as DNMT3A, essential for both de novo and maintenance of DNA methylation, were upregulated, whereas TET1, which has an important role in active de-methylation, was downregulated (Verheijen; et al., Reference Verheijen, Lienhard, Schrooders, Clayton, Nudischer, Boerno, Timmermann, Selevsek, Schlapbach, Gmuender, Gotta, Geraedts, Herwig, Kleinjans and Caiment2019) This study although performed on fetal-like cardiac cells, demonstrated the depth of epigenetic modification induced by DMSO. The vigorous activity of DMSO has been also investigated in other cellular compartments, such as changes in mitochondrial pathways associated with the formation of ROS and alteration of ATP production, which might also impair embryo division and cell cycle activity. DMSO is a known radical scavenger, and as an antioxidant helps to protect cells from the damage caused by free radicals, and against high levels of ROS. However, at normal or decreased levels of ROS, it may restrict cell metabolism by scavenging electrons needed for ATP production, which is positively associated with embryo viability, especially at the cleavage stage. Therefore DMSO-induced ATP decrease might cause downstream effects that may disrupt cellular function, fetal development and implantation potential (Yu and Quinn, Reference Yu and Quinn1994; Marks and Breslow, Reference Marks and Breslow2007; Hunt, Reference Hunt2011; Zorov et al., Reference Zorov, Juhaszova and Sollott2014).
The potential effect of vitrification on epigenetics alteration
In the past few years, several research groups have investigated the relationship between vitrification and epigenetics disruption in early embryo development. Preliminary results mainly from animal models suggest that the vitrification process might induce some epigenetic modification and, by doing so, impair embryonic gene expression. This alteration could lead to placenta potential modifications in growth patterns and metabolic parameters, which might be the cause of obstetric alteration at birth, such as intrauterine growth restriction and preterm delivery, but also in disease at later stages and in adult life, especially if the alteration involves the ‘imprinted genes’ (Fleming et al., Reference Fleming, Watkins, Velazquez, Mathers, Prentice, Stephenson, Barker, Saffery, Yajnik, Eckert, Hanson, Forrester, Gluckman and Godfrey2018; Verheijen et al., Reference Verheijen, Lienhard, Schrooders, Clayton, Nudischer, Boerno, Timmermann, Selevsek, Schlapbach, Gmuender, Gotta, Geraedts, Herwig, Kleinjans and Caiment2019; Kaneko-Ishino and Ishino, Reference Kaneko-Ishino and Ishino2022). Studies analyzing microRNA (epigenetic regulators of gene expression) in different placentas have found different expression between miRNAs in FET placentae versus those from fresh ET, which potentially can be an answer to the increased birthweight and perinatal complications, whereas no difference was observed in expression between placentae from fresh ET and spontaneous pregnancies (Hiura et al., Reference Hiura, Hattori, Kobayashi, Okae, Chiba, Miyauchi, Kitamura, Kikuchi, Yoshida and Arima2017; Marjonen et al., Reference Marjonen, Auvinen, Kahila, Tšuiko, Kõks, Tiirats, Viltrop, Tuuri, Söderström-Anttila, Suikkari, Salumets, Tiitinen and Kaminen-Ahola2018). Additional investigations in animals have shown that, following the vitrification–warming of mouse oocytes, the expression of the imprinted gene Kcnq1ot1 decreased significantly (Ma et al., Reference Ma, Long, Liu, Bai, Ma, Bai, Zuo and Li2022). Chen and collaborators reported that after the vitrification of mature bovine oocytes, the expression of imprinted genes Peg10, Kcnq1ot1 and Xist in blastocysts obtained by ICSI raised abnormally (Chen et al., Reference Chen, Zhang, Deng, Zou, Wang, Quan and Zhang2016). The same group in a subsequent paper found that vitrification of mouse MII oocytes affected the expression of some imprinted genes, such as the maternally imprinted genes Peg3, Peg10 and Igf2r in oocytes, and maternally imprinted genes Peg3 and Peg10 and paternal imprinted gene Gtl2 in cleavage stage embryo obtained after IVF (Chen et al., Reference Chen, Zhang, Wang, Chang, Xie, Fu, Zhang and Quan2019). Another study also reported that methylation of imprinted genes H19, Peg3 and Snrpn was decreased in blastocysts obtained by IVF after vitrified mouse oocytes (Wang et al., Reference Wang, Xu and He2010). Comparable results have been reported by other authors, showing a reduction in the overall DNA methylation level in oocytes and early embryos following the vitrification process (Ying et al., Reference Ying, Xiang-Wei, Jun-Jie, Dian-Shuai and Shi-En2014; Zhao et al., Reference Zhao, Wang, Zhang, Hao, Pang, Wang, Du, Zhao, Ruan, Zou, Hao, Zhu and Zhao2020). Therefore, collectively according to published evidence, especially in animal models, it seems that vitrification may affect the normal expression of imprinted genes by changing the DNA methylation level, affecting the regulatory region of those genes (Wang et al., Reference Wang, Xu and He2010; Ying et al., Reference Ying, Xiang-Wei, Jun-Jie, Dian-Shuai and Shi-En2014; Chen et al., Reference Chen, Zhang, Deng, Zou, Wang, Quan and Zhang2016, Reference Chen, Zhang, Wang, Chang, Xie, Fu, Zhang and Quan2019; Zhao et al., Reference Zhao, Wang, Zhang, Hao, Pang, Wang, Du, Zhao, Ruan, Zou, Hao, Zhu and Zhao2020) (Table 4).
Table 4. Summary of both human and animal studies showing the effects of vitrification on DNA methylation and histones modifications. DMR; differentially methylated regions; GV; oocyte at germinal vesicle stage, IVM; in vitro maturation, MII; oocyte at metaphase II stage; 5hmC; 5-hydroxymethylCytosine, 5mC; 5-methylCytosine

Human studies
Currently, only very few reports have been produced on humans, and overall have found no changes in DNA methylation and imprinted gene expression following vitrification. A study by Al-Khtib and co-authors stated that the methylation of imprinted genes H19 and Kcnq1ot1 was not affected in oocyte MII after in vitro maturation from the GV stage (Al-Khtib et al., Reference Al-Khtib, Perret, Khoueiry, Ibala-Romdhane, Blachère, Greze, Lornage and Lefèvre2011). Similar results have been observed by Liu and colleagues analyzing vitrification and warming of mature oocytes (MII) (Liu et al., Reference Liu, Zhou, Chu, Fu, Sha and Li2017). Furthermore, De Munck and collaborators reported no significant change in the overall DNA methylation level of in vitro cultured 8-cell embryos derived from vitrified oocytes (De Munck et al., Reference De Munck, Petrussa, Verheyen, Staessen, Vandeskelde, Sterckx, Bocken, Jacobs, Stoop, De Rycke and Van de Velde2015). Huo and colleagues using 16 donated human MII oocytes observed that, in total, 1987 genes were differentially expressed following oocyte vitrification/warming compared with the fresh mature oocytes and found that ∼82% of these genes were downregulated, while 18% were upregulated (Huo et al., Reference Huo, Yuan, Qin, Yan, Yan, Liu, Li, Yan and Qiao2021). Those genes were involved in several critical biological processes, such as two meiosis-related genes, Ncapd2 and Tubgcp5 were significantly downregulated following oocyte vitrification. Similar data were found in other genes whose activities are correlated with physiological processes including oogenesis, cellular response to heat, microtubule-based processes, methylation, ubiquinone biosynthetic processes, sister chromatid migration, DNA repair, oxidative phosphorylation, and ATP metabolic processes, which are overall correlated with oocytes quality and future embryo viability. The authors also investigated the time of storage of vitrified oocytes in nitrogen and found no alteration in gene expression, suggesting that, overall, the potential damage resulting after oocyte vitrification might be associated with the cryopreservation process itself rather than the storage (Huo et al., Reference Huo, Yuan, Qin, Yan, Yan, Liu, Li, Yan and Qiao2021). These findings are in agreement with others (Monzo et al., Reference Monzo, Haouzi, Roman, Assou, Dechaud and Hamamah2012; Stigliani et al., Reference Stigliani, Moretti, Anserini, Casciano, Venturini and Scaruffi2015; Xu and Xie, Reference Xu and Xie2018; Table 4).
Further concerns and concluding remarks
The goal of ART should be to generate healthy offspring without increased prevalence of disease, and an expectation of a long healthy life. The periconceptional period is extremely important for providing and maintaining epigenetic modelling, and any exposure to chemical or toxic compounds might cause persistent epigenetic aberrations, which can be transmitted to future generations, and might result in a long-term disease (Laprise, Reference Laprise2009; Stigliani et al., Reference Stigliani, Moretti, Anserini, Casciano, Venturini and Scaruffi2015; Xu and Xie, Reference Xu and Xie2018). Over the last 4 decades, we have witnessed a true advancement in ART, with an average rate of pregnancy of 40%. However, an association has been observed between ART-conceived babies and risk for congenital birth defects (Davies et al., Reference Davies, Moore, Willson, Van Essen, Priest, Scott, Haan and Chan2012; Sciorio and Esteves, Reference Sciorio and Esteves2022), low birth weight (Poikkeus et al., Reference Poikkeus, Gissler, Unkila-Kallio, Hydén-Granskog and Tiitinen2007), and placental dysfunction (Shevell et al., Reference Shevell, Malone, Vidaver, Porter, Luthy, Comstock, Hankins, Eddleman, Dolan, Dugoff, Craigo, Timor, Carr, Wolfe, Bianchi and D’Alton2005). Also, enough evidence has shown that FET is associated with a higher birth weight compared with fresh embryo replacement (Belva et al., Reference Belva, Bonduelle, Roelants, Verheyen and Van Landuyt2016; Ainsworth et al., Reference Ainsworth, Wyatt, Shenoy, Hathcock and Coddington2019; Hwang et al., Reference Hwang, Dukhovny, Gopal, Cabral, Diop, Coddington and Stern2019). A meta-analysis including 26 studies by Maheshwari and collaborators reported that singleton children born from vitrified-warmed cycles were associated with a higher risk of high birth weights, large for gestational age and above all faced an increased risk of hypertensive disorders during pregnancy (Maheshwari et al., Reference Maheshwari, Pandey, Amalraj Raja, Shetty, Hamilton and Bhattacharya2018). An increased birthweight in ART babies conceived following FET has been reported by several authors (Pelkonen et al., Reference Pelkonen, Koivunen, Gissler, Nuojua-Huttunen, Suikkari, Hydén-Granskog, Martikainen, Tiitinen and Hartikainen2010; Sazonova et al., Reference Sazonova, Källen, Thurin-Kjellberg, Wennerholm and Bergh2012; Pinborg et al., Reference Pinborg, Henningsen, Loft, Malchau, Forman and Andersen2014; Maheshwari et al., Reference Maheshwari, Raja and Bhattacharya2016). It was not easy to confirm whether the vitrification procedure itself was responsible for the higher birthweight offspring, or if other factors might have contributed, such as the use of CPAs or the drug used for endometrial preparation, or parental infertility, as no difference was observed in the birthweight when embryos were transferred in a natural cycle, speculating that drugs applied for endometrial preparation might be responsible for that condition (Mann et al., Reference Mann, Lee, Doherty, Verona, Nolen, Schultz and Bartolomei2004; Wang et al., Reference Wang, Xu and He2010; Donjacour et al., Reference Donjacour, Liu, Lin, Simbulan and Rinaudo2014; Shi et al., Reference Shi, Sun, Hao, Zhang, Wei, Zhang, Zhu, Deng, Qi, Li, Ma, Ren, Wang, Zhang, Wang, Liu, Wu, Wang and Bai2018; Ma et al., Reference Ma, Ma, Wen, Lei, Chen and Wang2019; Yao et al., Reference Yao, Huang, Huang, Lin, Guo, Hua, Wu, Hu and Li2020; Estudillo et al., Reference Estudillo, Jiménez, Bustamante-Nieves, Palacios-Reyes, Velasco and López-Ornelas2021). The debate is very active (Cheng et al., Reference Cheng, Fu, Zhang, Jia, Hou and Zhu2014; De Munck et al., Reference De Munck, Petrussa, Verheyen, Staessen, Vandeskelde, Sterckx, Bocken, Jacobs, Stoop, De Rycke and Van de Velde2015; Sermondade et al., Reference Sermondade, Hesters, De Mouzon, Devaux, Epelboin, Fauque, Gervoise-Boyer, Labrosse, Viot, Bergère, Devienne, Jonveaux, Levy and Pessione2023) and, overall, the epigenome establishment in cryopreserved embryos or oocytes remains still to be fully clarified (Fahy et al., Reference Fahy, MacFarlane, Angell and Meryman1984; Wang et al., Reference Wang, Xu and He2010; Jahangiri et al., Reference Jahangiri, Shahhoseini and Movaghar2014; Bouillon et al., Reference Bouillon, Léandri, Desch, Ernst, Bruno, Cerf, Chiron, Souchay, Burguet, Jimenez, Sagot and Fauque2016; Chen et al., Reference Chen, Zhang, Wang, Chang, Xie, Fu, Zhang and Quan2019; Barberet et al., Reference Barberet, Barry, Choux, Guilleman, Karoui, Simonot, Bruno and Fauque2020; Movahed et al., Reference Movahed, Shabani, Hosseini, Shahidi and Salehi2020; Barberet et al., Reference Barberet, Romain, Binquet, Guilleman, Bruno, Ginod, Chapusot, Choux and Fauque2021; Cantatore et al., Reference Cantatore, George, Depalo, D’Amato, Moravek and Smith2021; Ma et al., Reference Ma, Long, Liu, Bai, Ma, Bai, Zuo and Li2022; Sciorio and El Hajj, Reference Sciorio and El Hajj2022; Shafqat et al., Reference Shafqat, Kashir, Alsalameh, Alkattan and Yaqinuddin2022; Manna et al., Reference Manna, Barbagallo, Sagnella, Farrag and Calogero2023; Sciorio et al., Reference Sciorio, Tramontano, Rapalini, Bellaminutti, Bulletti, D’Amato, Manna, Palagiano, Bulletti and Esteves2023; Yan et al., Reference Yan, Zhang, Yang, Zhou, Ni and Yan2023). It is worth mentioning that the number of reports available on the topic is currently limited, and this lack of information needs to be urgently clarified. It is imperative to be proactive as vitrification and cryopreservation are routinely and rapidly gaining clinical use in modern ART treatments. At this time, novel advancements in molecular technologies allow scientists to perform a large-scale investigation of DNA methylation from even a single cell. Follow-up studies are urgently needed to clarify, eventually, the potential epigenetic risk associated with the vitrification procedures. In addition, epidemiological studies performed on a large scale are necessary to evaluate the implications that cryopreservation and CPAs might have on the offspring’s health, not only at the time of delivery but also during later adult life. Finally, we agree with a review paper recently published by Barberet and collaborators (Barberet et al., Reference Barberet, Barry, Choux, Guilleman, Karoui, Simonot, Bruno and Fauque2020) concluding that epigenetic and transcriptomic profiles are susceptible to the stress induced by ART manipulation and the cryopreservation protocol, and highlights the demand to increase our knowledge in the field.
Data availability statement
No data are available.
Author Contributions
RS contributed to the conception and designed the manuscript. RS, GC, LT, FMB, GMB, and MV wrote sections of the manuscript and revised it for content. All the authors contributed to the manuscript revision, read, and approved the submitted version.
Funding
This research received no external funding.
Institutional review board statement
All procedures performed in studies involving human participants were in accordance with the ethical standards of the institution and with the 1964 Helsinki Declaration and its later amendments. For this type of study, formal consent is not required.
Informed consent statement
Not applicable.
Competing interests
The authors have no conflict of interest to declare.