Introduction
The world's poultry industry is growing at a reasonable pace, but those involved have to minimise the occurrence of diseases and immunosuppression to enhance the profit in this competitive industry. One of the most treacherous immunosuppressive diseases in poultry is infectious bursal disease (IBD), which threatens the poultry industry throughout the world (Mahgoub et al., Reference MAHGOUB, BAILEY and KAISER2012; Qi et al., Reference QI, CHEN, REN, ZHANG, GAO, WANG, QIN, WANG, GAO and WANG2014). IBD virus (IBDV), a highly immunosuppressive virus, characteristically replicates in the lymphoid organs and directly suppresses the functionality of the immune system (Zhai et al., Reference ZHAI, WANG, YU and HU2014).
IBDV is a double-stranded RNA virus, belonging to the genus Avibirnavirus within the family Birnaviridae (Müller et al., Reference MÜLLER, SCHOLTISSEK and BECHT1979; Mahgoub et al., Reference MAHGOUB, BAILEY and KAISER2012; Ndashe et al., Reference NDASHE, SIMULUNDU, HANG'OMBE, MOONGA, OGAWA, TAKADA and MWEENE2016). Double-stranded RNA of the IBDV genome is made up of two segments A and B ( Figure 1 ). Segment A has two partially overlapping open reading frames (ORFs), known as major and minor. Major ORF encodes a polyprotein, which via auto-proteolysis produce viral proteins VP2, VP4 and VP3 and the minor ORF encodes for VP5 (a non-structural protein). VP1 of the IBDV is encoded by the RNA-dependent RNA polymerase, an ORF present on the segment B of the IBDV (Ndashe et al., Reference NDASHE, SIMULUNDU, HANG'OMBE, MOONGA, OGAWA, TAKADA and MWEENE2016; Mahgoub et al., Reference MAHGOUB, BAILEY and KAISER2012). IBDV structure is a non-enveloped, single-shelled icosahedral symmetry capsid of about 70 nm in diameter, composed of 260 trimers of VP2 that form spikes projecting radially from the capsid. The peptides derived from pre-VP2 C-terminal cleavages remain associated within virion. VP3 forms a ribonucleoprotein complex with the genomic RNA. Minor amounts of VP1 are incorporated in the virion. Segmented linear dsRNA genome: 2 segments (A, B) encode for 5-6 proteins. VP1 is found in a free form and covalently attached at the 5’ genomic RNA end (VPg). Segments size is about 2.3-3 kb and genome total size is about 6 kb. Genomic segment A encodes for a structural polyprotein which is matured in cis by VP4. It also encodes an alternative ORF translated possibly by leaky scanning (VP5).Genomic segment B encodes for VP1 (http://viralzone.expasy.org/viralzone/all_by_species/572.html).
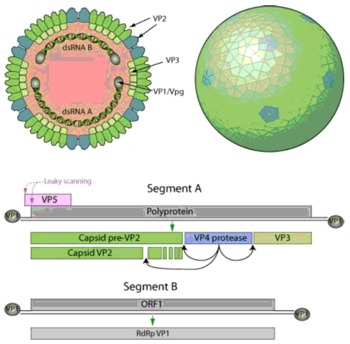
Figure 1 Structure and genetic coding for IBD virus.
When IBDV invade the body, the innate immune system is activated first and participates in the initial attack against the pathogens. Among the cells involved in innate immunity, dendritic cells (DCs) act as antigen-presenting cells and migrate from the infected tissue to the regional lymph nodes where they present the antigens to T cells (Akira, Reference AKIRA2011). Subsequently, the adaptive immune system is activated and antibody production and killer T cells are induced. The resulting antibodies and killer T cells specifically attack the pathogens ( Figure 2 ).

Figure 2 Interactions of innate and adaptive immunity when pathogens invade the body (Adopted from Akira, Reference AKIRA2011).
Immunosuppression decreases weight gain and production and increases carcass condemnation rates at processing plants. Moreover, immune dysfunction also increases the rate of mortality and morbidity mainly due to secondary viral and bacterial infections (Fussell, Reference FUSSELL1998). There are two serotypes reported so far for IBDV; IBDV 1 and IBDV 2. Clinical disease is only caused by the serotype 1 in chickens, but serotype 2 can infect turkeys, chicken, duck but does not cause clinical symptoms. On the basis of the virulence, serotype 1 is classified into classical virulent, antigenic variant, very virulent and attenuated strains ( Table 1 ).
Table 1 Viral proteins and their roles in pathogenesis of IBDV.

Chickens are highly susceptible to IBDV between ages of three and six weeks post-hatching (Aricibasi et al., Reference ARICIBASI, JUNG, HELLER and RAUTENSCHLEIN2010). Mechanisms of pathogenic and immunosuppressive aspects of IBDV are still not well known (Escaffre et al., Reference ESCAFFRE, LE NOUEN, AMELOT, AMBROGGIO, OGDEN, GUIONIE, TOQUIN, MULLER, ISLAM and ETERRADOSSI2013; Lee et al., Reference LEE, WU and LIN2014; Ouyang et al., Reference OUYANG, WANG, DU, LIU and ZHANG2015), however, it has been shown that IBDV causes the disruption of innate immune responses, macrophages (MΦ), T cells, DCs, and also the massive destruction of B cells (Mahgoub et al., Reference MAHGOUB, BAILEY and KAISER2012). A suppressed immune system is the frontrunner for suboptimal vaccine responses, thus leading to increased susceptibility to other diseases (Schat and Skinner, Reference SCHAT and SKINNER2014; Prandini et al., Reference PRANDINI, SIMON, JUNG, POPPEL, LEMIERE and RAUTENSCHLEIN2016). This review discusses current research regarding the immunotoxic and immunosuppressive nature of IBDV in the poultry and highlights areas requiring future research attentions that may help to establish foundations for effective and improved vaccines against IBDV.
Interaction of innate immune responses with IBDV
Innate immunity is the primary barrier against pathogens, and intestinal mucosa is the first barrier that prevents the invasion of the IBDV. Intestinal mucosa may have direct role in regulation of the adaptive immune responses (Wang et al., Reference WANG, ZHOU, SHE, XIONG, SUN, PENG, LIU and LIU2009b). IBDV uses its one of four structural proteins (pep46) to disturb the cell membrane and enter the target cells. This structural protein deforms the membrane and makes the pores open. The virus enters the target cells in a multiple step process; first of all it recognises the target cell, endocytoses in the presence of calcium and releases pep46. After this, membrane destabilisation starts and pores are formed 10 nm in size that can be seen through electron microscope. As the size of the virus is 70 nm, so it cannot enter into the cytoplasm, indicating that virus uses these pores only for the transfer of small molecules that initiate genetic transcription and subsequently translocation (Galloux et al., Reference GALLOUX, LIBERSOU, MORELLET, BOUAZIZ, DA COSTA, OULDALI, LEPAULT and DELMAS2007).
Innate immune responses depend on pattern-recognition receptors (PRR), such as toll-like receptors (TLR), retinoic acid-induced gene I (RIG-I)-like receptors (RLR), and nucleotide-binding oligomerisation-domain-like receptors (NLR) to identify the pathogen-associated molecular patterns (PAMP), such as dsRNA, lipopolysaccharide (LPS) and flagella. TLR3 and TLR7 are only involved in viral infection in chickens. TLR3, TLR4 and TRIF is upregulated in IBDV infection in chickens (Rauf et al., Reference RAUF, KHATRI, MURGIA, JUNG and SAIF2011a; Guo et al., Reference GUO, WANG, CUI, RUAN, LIU and LI2012; Lee et al., Reference LEE, KIM, WU and LIN2015a) and chick embryo fibroblasts cells (Wong et al., Reference WONG, HON, ZENG and LEUNG2007). In IBDV infections, expression of TLR2B, TLR4, TLR7 and MyD88 was down regulated (Rauf et al., Reference RAUF, KHATRI, MURGIA, JUNG and SAIF2011a; Guo et al., Reference GUO, WANG, CUI, RUAN, LIU and LI2012) in the bursa of Fabricius (BF). Down regulation of the TLR2B indicates decreased immune responses (Guo et al., Reference GUO, WANG, CUI, RUAN, LIU and LI2012). IBDV also utilises some cellular proteins and uses them for replication and to protect itself from the innate antiviral responses, for example VP3 of the IBDV use the ribosomal protein L4 (RPL4) for the replication (Chen et al., Reference CHEN, LU, ZHANG, GAO, WANG, GAO, WANG, LI, GAO, CUI, GAO, LIU, ZHANG, QI and WANG2016). IBDV protein (VP3) has a high affinity with the chicken melanoma differentiation-associated gene 5 (MDA5), which blocks the signalling pathway to IBDV genomic dsRNA. As a result MDA5 fails to recognise the viral RNA thus prohibiting the antiviral immune response (Ye et al., Reference YE, JIA, SUN, HU, WANG, LU and ZHOU2014).
cytokines and chemokines
Cytokines are essential for the activation, differentiation and control of the host immune system. Variation in the expression of the cytokines had been noted in IBDV infection depending on the cells, virus type, age, and different tissues (He et al., Reference HE, GENOVESE and KOGUT2011; Li et al., Reference LI, GAO, GAO, QI, GAO, QIN, WANG and WANG2013a; Lee et al., Reference LEE, WU and LIN2015b; Yasmin et al., Reference YASMIN, YEAP, TAN, HAIR-BEJO, FAKURAZI, KAISER and OMAR2015). IBDV infection in chicken promotes the expression of the pro-inflammatory cytokines and chemokines, Th1 cytokines, iNOS and MHC class I in HD11 cells in vitro (Rasoli et al., Reference RASOLI, YEAP, TAN, ROOHANI, KRISTEEN-TEO, ALITHEEN, RAHAMAN, AINI, BEJO, KAISER and OMAR2015) and upregulate chicken IL-12 and IL-18 in HD11 cells infected with IBDV (Khatri et al., Reference KHATRI, PALMQUIST, CHA and SHARMA2005; Lee et al., Reference LEE, WU and LIN2015b). IBDV also upregulates the IL-6, IL-8 and interferons (IFNs) expression in the BF (Carballeda et al., Reference CARBALLEDA, ZOTH, GOMEZ, LUCERO, GRAVISACO and BERINSTEIN2014) and IL-8 (Rauf et al., Reference RAUF, KHATRI, MURGIA, JUNG and SAIF2011a). Temporary up regulation of IFN-γ, IL-2, IL-6 and down regulation of the cytokine IL-1β and type I- IFNs were noted in IBDV infection (Rautenschlein et al., Reference RAUTENSCHLEIN, VON SAMSON-HIMMELSTJERNA and HAASE2007; Eldaghayes et al., Reference ELDAGHAYES, ROTHWELL, WILLIAMS, WITHERS, BALU, DAVISON and KAISER2006).
There are three main type of the IFNs which are IFNs type I (IFN-I; IFNα, IFNβ), Type II (IFN-II; IFN-γ) and Type III (IFN-III; IFNλ). Production of IFN-I is inhibited in IBDV infection in chickens (Eldaghayes et al., Reference ELDAGHAYES, ROTHWELL, WILLIAMS, WITHERS, BALU, DAVISON and KAISER2006) and is not induced in IBDV infected cells either in vivo or in vitro (Ye et al., Reference YE, JIA, SUN, HU, WANG, LU and ZHOU2014) although dsRNA induces IFNβ expression. IBDV infection in HD11 cells induced significantly upregulated expression levels in chicken, IFN-β (693-fold) (Lee et al., Reference LEE, WU and LIN2015b) also in BF (Rauf et al., Reference RAUF, KHATRI, MURGIA, JUNG and SAIF2011a) and IFN-γ in unvaccinated chickens (Lee et al., Reference LEE, KIM, WU and LIN2015a). IFN antiviral signal transduction pathway is controlled by the interferon regulatory factor 2 (IRF2). IBDV infection seriously obstructs the production of the IFNs (Ye et al., Reference YE, JIA, SUN, HU, WANG, LU and ZHOU2014) by inhibiting the expression of the IRF2 gene by the gga-miR-9, hence promoting the IBDV replication (Ouyang et al., Reference OUYANG, WANG, DU, LIU and ZHANG2015). However, there are some contradictory studies that indicate IRF-1 is upregulated in the IBDV challenged birds after three days post challenge (Lee et al., Reference LEE, KIM, WU and LIN2015a). These contradictory results may be due to age, pathogenicity of the virus, days post infection (DPI) when measuring levels and examination of either production or dsRNA expression levels. IFN-I production is not enhanced in cells in vitro or in vivo but the genomic dsRNA induces the IFN-β expressions which are triggered by blocking the MDA5 dependent signalling pathway (Ye et al., Reference YE, JIA, SUN, HU, WANG, LU and ZHOU2014), and IBDV is unable to inhibit this expression if these are activated (Ye et al., Reference YE, JIA, SUN, HU, WANG, LU and ZHOU2014). Another possible pathway is the use of VP4 for inhibition of the IFN-I via communication with the glucocorticoid-induced leucine zipper (Li et al., Reference LI, WANG, LI, LI, CAO and ZHENG2013b). So, it is clear that viral proteins affect the antiviral immune responses in IBDV infection by triggering different pathways.
dendritic cells
Dendritic cells (DCs) work like sentinels within the immune system (Levitz and Golenbock, Reference LEVITZ and GOLENBOCK2012) and present in all parts of the chicken's body, including lymphoid, non-lymphoid, interstitial tissues, skin epidermis, mucosal surfaces and peripheral blood (Liu, Reference LIU2001). DCs are a vital protagonist in the initiation of the primary immune responses by increasing proliferation of naïve T cells, differentiation to memory cells (Yasmin et al., Reference YASMIN, YEAP, TAN, HAIR-BEJO, FAKURAZI, KAISER and OMAR2015) and can direct the T cell response (Juul-Madsen et al., Reference JUUL-MADSEN, VIERTLBÖECK, HÄRTLE, SMITH and GÖBEL2014). They recognise pathogens by TLRs and cytoplasmic nucleotide-binding oligomerisation domain-like receptors for the initiation of the innate immune response (Mogensen, Reference MOGENSEN2009). Recently, Yasmin et al. (Reference YASMIN, YEAP, TAN, HAIR-BEJO, FAKURAZI, KAISER and OMAR2015) characterised bone marrow derived DCs (chBM-DCs) in chicken inoculated with LPS, inactivated vvIBDV and live vvIBDV. Interestingly, it was reported that vvIBDV infected BM-DC showed significantly higher numbers of apoptotic cells, increased expression of VP3 and VP4. Expression of IL-1β, IL-18 and CCR7 was upregulated by the LPS whereas live vvIBDV treatment significantly enhanced the expression of Th1-like cytokines, IFN-γ and IL-12α and TLR3. On the other hand, inactivated vvIBDV-treated BM-DC was unable to increase the expression of the IFN-γ, IL-12α and TLR3 (Yasmin et al., Reference YASMIN, YEAP, TAN, HAIR-BEJO, FAKURAZI, KAISER and OMAR2015).
In another study by Liang et al. (Reference LIANG, YIN, QIN and YANG2015) compared the effect of LPS, inactivated IBDV and IBDV on activation and maturation of chBM-DCs. These cells displayed the typical morphology of DCs after LPS or inactivated IBDV or IBDV inoculation. Significantly upregulated expression of chBM-DCs surface CD40 and CD86 molecules, and ability to induce T-cell proliferative response was found in LPS or virus stimulated chBM-DCs than non-stimulated. But, this stimulation was higher for inactive IBDV than live IBDV. In conclusion, IBDV replicate in DCs, alter the expression of immunity gene and trigger the development of specific immune response or memory.
natural killer cells
Natural killer (NK) cells are normally present in the dormitory phase in circulation but they start infiltration in the infected tissue by the activation of cytokines (Glas et al., Reference GLAS, FRANKSSON, UNE, ELORANTA, OHLEN, ORN and KARRE2000). Their major function is to recognise and kill virally-infected and neoplastic cells. When the ligand of the NK cells interact with the cell-surface receptors, they produce several cytokines like IFN-γ, which have an immunoregulatory role (Mandal and Viswanathan, Reference MANDAL and VISWANATHAN2015).
Studies related to the role of natural killer cells in IBD are limited. Sharma and Lee (Reference SHARMA and LEE1983) have studied the effect of IBDV on two genetic lines of chicken and found that cell cytotoxicity and mitogenic response of the NK cells were not affected by IBDV whereas, Kumar et al. (Reference KUMAR, DAS and RAO1998) found functional impairment of the NK cells in IBD cases. Interestingly, IBDV did not constantly boost NK cell levels in chickens, as Rauf et al. (Reference RAUF, KHATRI, MURGIA and SAIF2011b) found a down regulation of the natural killer cell lysin by IBDV.
macrophages
Macrophages (MΦ) are key cells of the innate immune system and are well equipped to destroy invading pathogens (Khatri and Sharma, Reference KHATRI and SHARMA2008; de Geus and Vervelde, Reference DE GEUS and VERVELDE2013; Scanes, Reference SCANES2015). MΦ play an important role in presenting the antigens to T cells and serve as a bridge between the cellular and humoral immune response. Some earlier reports indicated that MΦ may be susceptible to the IBDV infection (Inoue et al., Reference INOUE, YAMAMOTO, MATUO and HIHARA1992; Khatri et al., Reference KHATRI, PALMQUIST, CHA and SHARMA2005). It has been proposed in many studies that MΦ are the main cells that transfer the IBDV from the gut to the peripheral tissue (Kim et al., Reference KIM, KARACA, PERTILE, ERICKSON and SHARMA1998; Lam, Reference LAM1998; van den Berg et al., Reference VAN DEN BERG, ETERRADOSSI, TOQUIN and MEULEMANS2000; Palmquist et al., Reference PALMQUIST, KHATRI, CHA, GODDEERIS, WALCHECK and SHARMA2006; Khatri and Sharma, Reference KHATRI and SHARMA2006).
IBDV increase the expression of proinflammatory cytokines IL-1, IL-6, IL-18 and inducible nitric oxide synthase iNOS in the BF (Khatri et al., Reference KHATRI, PALMQUIST, CHA and SHARMA2005; Liu et al., Reference LIU, ZHANG, HAN, YUAN and LI2010) and spleen (Palmquist et al., Reference PALMQUIST, KHATRI, CHA, GODDEERIS, WALCHECK and SHARMA2006), and significantly higher levels of nitric oxide in the splenocytes (Kim et al., Reference KIM, KARACA, PERTILE, ERICKSON and SHARMA1998) and DH11 cells (Rasoli et al., Reference RASOLI, YEAP, TAN, ROOHANI, KRISTEEN-TEO, ALITHEEN, RAHAMAN, AINI, BEJO, KAISER and OMAR2015). Moreover, IBDV upregulate IFN-β stimulated genes, including those encoding oligoadenylate synthetase (OAS), dsRNA-dependent protein kinase (PKR), myxovirus resistance (Wong et al., Reference WONG, HON, ZENG and LEUNG2007; Lee et al., Reference LEE, WU and LIN2015b) cyclooxygenase-2 (COX-2), chemokine (IL-8) (Khatri and Sharma, Reference KHATRI and SHARMA2006) and antigen-presenting molecules (Li et al., Reference LI, HANDBERG, JUUL-MADSEN, ZHANG and JORGENSEN2007; Lee et al., Reference LEE, WU and LIN2015b).
IBDV infection enhances the expression of MDA5 (Lee et al., Reference LEE, WU and LIN2015b) and upregulates the pro-inflammatory cytokines IL-1β, pro-inflammatory chemokines CCL4, CXCLi1 and CXCLi2, and Th1 cytokines IL-12α and IL-18, and downregulates IL-10 in HD11 cells. This overexpression of pro-inflammatory cytokines and iNOS mRNA demonstrates that IBDV can affect the cellular immune responses, especially MΦ. The detailed mechanism of upregulation of the cytokines and iNOS in IBDV infections is unknown, but the inhibition of nuclear factor (NF)-ĸB and p38 mitogen-activated protein kinases (MAPK) inhibitor lessens the virus-induced iNOS, COX-2, IL-8 and NO production. This means that IBDV use the NF-ĸB and p38 MAPK to increase the activation of the MΦ (Khatri and Sharma, Reference KHATRI and SHARMA2006). This upregulation of the cytokines and decrease in the MΦ number (Palmquist et al., Reference PALMQUIST, KHATRI, CHA, GODDEERIS, WALCHECK and SHARMA2006) may leads to a decrease in number of the resident MΦ. After upregulation in cytokines and MΦ activation, recovery process from the disease is delayed, resulting in cellular immunosuppression depending on the breed, virus dose/strain, and age of the bird (Rauw et al., Reference RAUW, LAMBRECHT and VAN DEN BERG2007).
It is possible that macrophage cell line adapted IBD enhanced the antigen presenting ability of MΦ and improved the activation of the T cells (Khatri and Sharma, Reference KHATRI and SHARMA2008). IBDV induce the cytopathic effects and replicate in the primary stem cells, making them highly susceptible to replication of IBDV (Khatri and Sharma, Reference KHATRI and SHARMA2009b).
mast cells
Mast cells (MCs) are present everywhere in the body especially in the tissues associated with the structure like nerves and blood vessels, and in tissues that interface the environment. MCs are responsible for the production of inflammatory substances, which indicate that MCs play a vital role in immune responses (Wang et al., Reference WANG, LIU, SHE, XU, LIU, XIONG, YANG, SUN and PENG2009a; Reference WANG, XIONG, SHE, LIU, ZHANG, LUO, LI, HU, WANG, ZHANG and SUN2008). MCs recognise the viruses or dsRNA through TLR3 and produce a number of the cytokines and chemokines that leads to increase in the recruitment of the effector cells in that specific area (Abraham and St John, Reference ABRAHAM and ST JOHN2010; Moon et al., Reference MOON, ST LAURENT, MORRIS, MARCET, YOSHIMURA, SEKAR and BEFUS2010). Severe histological lesions, increased MCs (at 1, 2, 3rd DPI) and enhanced tryptase activity were observed in the thymus, spleen, glandular stomach, liver, kidney and especially in BF from infection with vvIBDV in chickens (Wang et al., Reference WANG, XIONG, SHE, LIU, ZHANG, LUO, LI, HU, WANG, ZHANG and SUN2008). Contrary to this finding, a decrease in the population of MCs were observed in the duodenum, jejunum and ileum ( Figure 3 ) at second and third DPI with vvIBDV in specific pathogen free chickens (Wang et al., Reference WANG, ZHOU, SHE, XIONG, SUN, PENG, LIU and LIU2009b). Large quantities of tryptase in the BF, brain and other organs (which was absent in negative control) indicated that it may be involved in inflammation. Tryptase is the most abundant product of the MCs and it‘s quantity is directly related to the number of MCs (Wang et al., Reference WANG, LIU, SHE, XU, LIU, XIONG, YANG, SUN and PENG2009a) and it can stimulate MCs secretion, causing a feedback cycle as the disease progresses (He et al., Reference HE, GACA and WALLS1998). Degranulated MCs release tryptase that may then attract neighbouring MCs, leading to inflammation and lesions (Wang et al., Reference WANG, LIU, SHE, XU, LIU, XIONG, YANG, SUN and PENG2009a). Treatment with ketotifen (a mast cell membrane stabiliser) in IBDV infected birds decreased MCs numbers in BF and reduced bursal damage (Wang et al., Reference WANG, LIU, SHE, XU, LIU, XIONG, YANG, SUN and PENG2009a). Tissue damage and mortality can be reduced by inhibiting the MCs degranulation and mediator release in IBDV infection (Graham et al., Reference GRAHAM, TEMPLE and OBAR2015; Wang et al., Reference WANG, LIU, SHE, XU, LIU, XIONG, YANG, SUN and PENG2009a).

Figure 3 An outline of pathogenic and immunosuppressive aspects of IBDV (adapted from Sharma et al., Reference SHARMA, KIM, RAUTENSCHLEIN and YEH2000).
heterophils
These are the equivalent to the neutrophils in mammals (Wu and Kaiser, Reference WU and KAISER2011) and belong to the polymorphonuclear leukocytes (granulocytes). Heterophils are mainly involved in the elimination of the pathogens. Granules present in the heterophils contain β-defensins (Gal-1 and Gal-2), cathepsinn, lysozyme, acid phosphatase, β-glucuronidase, and α-glucosidase (Genovese et al., Reference GENOVESE, HE, SWAGGERTY and KOGUT2013), which have the antimicrobial functions. Probably activated by T cells, granulocytes cause release of cytokines or oxidative burst (Kogut et al., Reference KOGUT, ROTHWELL and KAISER2002). Release of cytokines and nitric oxide has been suggested as part of the IBD syndrome that resembles septic shock (Berg, Reference BERG2000). The role played by the heterophils in IBDV infection is not well defined, and studies related to the functions of heterophils in IBD are lacking. The number of the heterophils entering the BF increase with acute IBDV infection (Lam, Reference LAM1998). Kabell et al. (Reference KABELL, IGYARTO, MAGYAR, HAJDU, BIRO, BISGAARD and OLAH2006) have studied the effect of IBDV after pretreating the three groups with 5-fluorouracil, a placebo and 5-fluorouracil, and the results indicated that heterophil granulocytes, together with the bursal secretory DCs, contribute to the outbreak and may progress clinical symptoms. IBDV in vitro alter the functioning of heterophils, with decreases in cell migration and phagocytic activity whilst it activates cellular oxidative bursts and increases cellular adherence to the IBDV-infected chicken embryo fibroblasts of the heterophils. Electron microscopy of heterophils has shown condensed chromatin and packets of intact granules (Lam, Reference LAM1997), either free or being phagocytosed by MΦ, indicating that the heterophils were undergoing apoptosis. (Lam, Reference LAM1998). So, it may be proposed that heterophils are one of the target cells of the immune system for IBDV.
Interaction of acquired immune responses with IBDV
This is mainly involved in the clearance, memory and production of antibodies. IBDV infection severely affects adoptive immune responses in chickens resulting in death and secondary infections. This system has the ability to distinguish self from the foreign particles, and their destructive response should be only targeted towards foreign particles. IBDV damage the B cells, leading to lower antibody production and T cells, resulting in impaired virus killing ability.
B- lymphocytes
IBDV replicates in the lymphoid cells, particularly in the BF of young chickens, affecting the activity of the B cells with respect to immunoglobulin (Ig) M expression (Kong et al., Reference KONG, OMAR, HAIR-BEJO, AINI and SEOW2004; Withers et al., Reference WITHERS, DAVISON and YOUNG2006). IgM is the main antibody that is produced in the serum during the primary immune response (Maroufyan et al., Reference MAROUFYAN, KASIM, EBRAHIMI, LOH, BEJO, ZERIHUN, HOSSENI, GOH and FARJAM2012). During lysis, inhibition of proliferation and induction of the apoptosis causes the depletion of B lymphocytes (Rodriguez-Lecompte et al., Reference RODRIGUEZ-LECOMPTE, NINO-FONG, LOPEZ, FREDERICK MARKHAM and KIBENGE2005).B cells are the main players in the humoral immune system and their depletion leads to the immunosuppression, increasing the birds susceptibility to secondary infections (Yasmin et al., Reference YASMIN, YEAP, TAN, HAIR-BEJO, FAKURAZI, KAISER and OMAR2015) and poor vaccine titres to other diseases (Ciccone et al., Reference CICCONE, MWANGI, RUZOV, SMITH, BUTTER and NAIR2014). IBDV do not have a significant effect on the humoral response in birds having the different genetic backgrounds (Aricibasi et al., Reference ARICIBASI, JUNG, HELLER and RAUTENSCHLEIN2010) and age (Rautenschlein et al., Reference RAUTENSCHLEIN, VON SAMSON-HIMMELSTJERNA and HAASE2007).
Drastic decreases in IgM-bearing B cells was noted by the cytolytic effects of IBDV (Hirai et al., Reference HIRAI, FUNAKOSHI, NAKAI and SHIMAKURA1981; Kim et al., Reference KIM, GAGIC and SHARMA1999), whereby infected chickens produce less antibodies for other pathogens (Kim et al., Reference KIM, GAGIC and SHARMA1999). The vvIBDV (UK661) causes the depletion of the Bu-1+, IgM+ cells from the BF, spleen and thymus resulting in losses of mature and immature B lymphocytes. A very small number of the Bu-1+ cells were repopulated after 14 days following the infection when they were again expressing the IgM or IgG. This recovery of the B-lymphocytes was considered due to the development of two type of follicles, undifferentiated and differentiated. Plenty of the undifferentiated follicles are required for the production of antibodies against IBDV (Mahgoub et al., Reference MAHGOUB, BAILEY and KAISER2012). After the acute phase of the disease, B lymphocytes begin to appear due to the repairing of the BF, but these birds show depressed primary antibody respaces until seven weeks post-infection. The age of the bird and virus pathotype were the main factors that affected the recovery process (Schat and Skinner, Reference SCHAT and SKINNER2014).
The detailed mechanism of the lysis of IBDV infected B cells is not known, but it has been assumed that IBDV affects the methylation process of the B cell genome. This highly methylated genome of B cells protect them from the mutagenic activity of activation-induced deaminase at off-target sites, as this enzyme is inefficient at deaminating 5-methylcytosine (Wijesinghe and Bhagwat, Reference WIJESINGHE and BHAGWAT2012). Activation-induced deaminase expressed in the BF is involved in antibody diversification by gene conversion in vivo, but advanced methylation may protect the reliability of the B-cell genome from over-expression of the deaminating enzyme (Ciccone et al., Reference CICCONE, MWANGI, RUZOV, SMITH, BUTTER and NAIR2014). IBDV interruption to genomic methylation processes and an increase in global 5-hydroxymethylcytosine leads to genomic instability, viral progress, cell death (Ciccone et al., Reference CICCONE, MWANGI, RUZOV, SMITH, BUTTER and NAIR2014) and immunosuppression.
T- lymphocytes
T cells are important for the clearance of the infected organs after IBDV infection. IBDV vaccinated (but T cell compromised) chickens were not protected during an IBDV challenge (Rautenschlein et al., Reference RAUTENSCHLEIN, YEH and SHARMA2002). T cell compromised chickens showed fewer antibodies, higher virus titres, extensive muscular haemorrhages and suppressed proliferative response (Poonia and Charan, Reference POONIA and CHARAN2001). So, T cells have an important role in preventing lesions and replication of the virus in infected birds.
Significant thymocyte depletion, apoptosis ( Figure 3 ) and decreases in the proliferation response has been observed by in ovo administration of classical virulent IBDV (Khatri and Sharma, Reference KHATRI and SHARMA2009a), and reduced lymphocyte proliferation was observed in cvIBDV infected chickens by Long et al. (Reference LONG, GUO, WANG, LIU, ZHANG and YANG2011). In addition, downregulation of CD132+CD8+, upregulation of CD132+CD25+ T cells in BF and functional disturbance in the secretion of cytokine γc in thymus was reported (Wang et al., Reference WANG, TENG, JIA, SUN, WU and ZHOU2014).
CD4 and CD8 Cells
CD4+ cells produce soluble T cell growth factors and stimulate B cell differentiation and proliferation. Perforin and granzyme-A dependant cytotoxic pathways of the cytotoxic T cell are mainly involved in the clearance of the IBDV from the BF, due to higher expression of these proteins in the CD4 and CD8 and BF cells (Rauf et al., Reference RAUF, KHATRI, MURGIA and SAIF2011b). CD4 and CD8 cells populations appeared to increase in the BF, decrease in the thymus (particularly in the cortex), and show an increasing trend for CD4 and decreasing CD8, which was observed in the spleen in vvIBDV infected chickens (Carballeda et al., Reference CARBALLEDA, ZOTH, GOMEZ, LUCERO, GRAVISACO and BERINSTEIN2014), although results from work by Rodenberg et al. (Reference RODENBERG, SHARMA, BELZER, NORDGREN and NAQI1994) were contradictory. It is not clear whether these higher populations are due to cell migration from the tissues to the periphery or contractions of the resident cells (Rasoli et al., Reference RASOLI, YEAP, TAN, ROOHANI, KRISTEEN-TEO, ALITHEEN, RAHAMAN, AINI, BEJO, KAISER and OMAR2015). Bird age and genetic background differentially modulate the activity of T cell in IBDV infections (Rautenschlein and Haase, Reference RAUTENSCHLEIN and HAASE2005, Tippenhauer et al., Reference TIPPENHAUER, HELLER, WEIGEND and RAUTENSCHLEIN2013). Recruitment of CD4 and CD8 T-lymphocytes enhanced damage in the BF by releasing cytokines and causing a cytotoxic effect (Wang et al., Reference WANG, XIONG, SHE, LIU, ZHANG, LUO, LI, HU, WANG, ZHANG and SUN2008) that leads to prolonged immune suppression after IBD.
It has been noted that the relative proportions of the CD4 and CD8 cells did not change in the BF after infection with cvIBDV (Rodenberg et al., Reference RODENBERG, SHARMA, BELZER, NORDGREN and NAQI1994), although converse to these results, Williams and Davison (Reference WILLIAMS and DAVISON2005) found that CD4 and CD8 cells were obvious at 3 and 5 dpi respectively and remained at higher levels until 14 days. The γδTCR+ T-cell population was not affected in the thymus and showed a slight increase (P< 0.05) only at 5 dpi in BF after vvIBDV (strain UK661) infection (Williams and Davison, Reference WILLIAMS and DAVISON2005).
regulatory T- cells
It has been well documented that CD4 CD25 regulatory T cells play an immunosuppressive role in many diseases (Yu et al., Reference YU, RUI, SHAO, LIU, LU, ZHANG and LI2015) and the chickens CD4 CD25 cells have the similar suppressive and cytokine production properties (Shanmugasundaram and Selvaraj, Reference SHANMUGASUNDARAM and SELVARAJ2011). Upon infection with Harbin-1 (very virulent) or Ts (moderately virulent) strains of the IBDV, CD4 CD25 cells migrated out from the thymus (observed up to 5dpi) and this was more than any other CD4 cells. Infiltration of the CD4 CD25 cells along with CD4 cells was observed in the BF after IBDV infection, totalling 44.3% ± 7.4% of the infiltrating CD4 cells (Yu et al., Reference YU, RUI, SHAO, LIU, LU, ZHANG and LI2015). Increased numbers of the CD4 CD25 cells were detected in the peripheral blood. Viral infection is the most probable cause of the migration of the CD4 CD25 cells to the periphery, away from their origin, which affect their suppressive roles (Yu et al., Reference YU, RUI, SHAO, LIU, LU, ZHANG and LI2015).
T cells upregulate IFN-γ after IBDV infection (Khatri and Sharma, Reference KHATRI and SHARMA2006) which inhibits the mitogenic response of the T cells in vitro (Sharma et al., Reference SHARMA, KIM, RAUTENSCHLEIN and YEH2000) and production of the IFN-γ from the spleenocytes from naïve chickens. IFN-γ reduces the production of IL-2 (Bradley et al., Reference BRADLEY, DALTON and CROFT1996). These activated T cells produce more IFN-γ, which stimulates MΦ to produce more cytokines. This delays the recovery process and results in T cell immunosuppression. The measurement of ChIFN-c after mitogen or antigen recall stimulation could be a good indicator of immunosuppression in chicken after IBDV infection or immunocompetence of flocks in general. IBDV infection downregulates the interferon regulatory factor (IRF) 4 and upregulates IRF1 (Guo et al., Reference GUO, WANG, CUI, RUAN, LIU and LI2012), leading to the differentiation of the T helper cell to Th1, resulting in an inflammatory response and antibody production that may lead to immunosuppression.
Immunosuppression interventions
The ultimate goal of intervention is the prevention of economic losses due to immunosuppressive viral infections. This will be achieved mainly through biosecurity, to prevent exposure to the causes of immunosuppressive diseases, and increasing the resistance to challenge from immunosuppressive agents through carefully chosen vaccination strategies and genetic selection. Recent cloning of avian cytokine genes has shown their potential usefulness as therapeutic agents for IBD in poultry as well as vaccine adjuvants, which will become more feasible in the future. Furthermore, certain immunomodulary products have been experimentally tested and shown to reduce the severity of IBD induced lesions and consequently immunosuppression.
Currently, the disease is controlled by live attenuated or inactivated IBDV but these can revert back to virulence and may not give full protection against the vvIBDV strain. The inactivated or killed viruses are usually given to birds in the pre-laying stage to induce higher levels of antibody production for at least two weeks. By virtue of greatly advanced molecular virology and the availability of genomic information on IBDV, there are opportunities to create novel concept vaccines. DNA vaccines have great potential as an alternative capable of inducing protective immune responses against a variety of infectious diseases. Several studies have been conducted for the development of a DNA vaccine encoding the VP2 gene of IBDV, with variable protection results (Pradhan et al., Reference PRADHAN, PRINCE, MADHUMATHI, ARUNKUMAR, ROY, NARAYANAN and ANTONY2014). However, a DNA vaccine encoding the VP2 gene alone has limited potency and results in only partial protection. Several methods have been employed to increase the efficacy of DNA vaccines including a ‘DNA prime–protein boost’ strategy wherein initial immunisation with plasmid DNA encoding the VP2 gene is followed by boosting with recombinant VP2 protein or killed vaccine virus, which resulted in complete protection (Gao et al., Reference GAO, LI, GAO, QI, GAO, QIN, WANG and WANG2013). Heat shock protein (HSP) is a member of the family of molecular chaperones that assists in protein folding and processing. Fusion of antigen to HSP70 of Mycobacterium tuberculosis has been explored as a potential strategy for enhancing vaccine potency. It has been previously reported that fusion of antigen to the C-terminal domain (amino acid residues 359–610) of M. tuberculosis has the ability to induce antigen specific humoral, cytotoxic T lymphocyte and Th1 responses (Ebrahimi et al., Reference EBRAHIMI, DABAGHIAN, TEBIANIAN and JAZI2012).
Conclusions
The poultry industry has economic losses due to long term IBDV mediated immunosuppression, mortality, morbidity and stunted growth. Recent developments in the understanding of immunotoxic and immunosuppressive effects of IBDV could potentially offer a way to prevent these conditions. One solution is to improve the ability of the bird to resist IBDV through genetic selection while maintaining industry standards. However, additional research is warranted to enhance understanding of immune cells and viral protein interactions to effectively design new vaccines that protect the birds from the newly emerging highly pathogenic strains, can regulate cytokine expressions and have the ability to activate cells of innate immune system. Future research should be focussed on developing vaccines that prevent drastic changes in cytokine expressions and activate the innate immune along with humoral immune responses to IBDV.