Introduction
Pesticide resistance has always been a global concern, and in May 2018, Science published a special issue focusing on the rise of resistance (Ash Reference Ash2018; Atashgahi et al. Reference Atashgahi, Sánchez-Andrea, Heipieper, van der Meer, Stams and Smidt2018; Baker et al. Reference Baker, Thomson, Weill and Holt2018; Fisher et al. Reference Fisher, Hawkins, Sanglard and Gurr2018; Gould et al. Reference Gould, Brown and Kuzma2018). Herbicide resistance causes substantial yield loss, agroecosystem imbalance, and food safety issues. Since their initial introduction in the late 1950s, herbicides have dominated weed control practices in China. Herbicide resistance, inevitably, has become the biggest threat to the sustainability of chemical-based weed management in China. Given that China has followed the same laissez-faire approach toward resistance management that has been practiced in developed countries, which emphasizes private incentives and voluntary actions (Davis and Frisvold Reference Davis and Frisvold2017), herbicide resistance has constantly/rapidly evolved and steadily increased over the past decade. According to the International Herbicide-Resistant Weed Database (Heap Reference Heap2019), cases of herbicide resistance have increased remarkably in China between 2009 and 2019. There were only 15 resistance cases documented before 2009, but 45 were recorded by 2019 (Figure 1). A total of 27 weed species (13 dicots and 14 monocots) have evolved resistance to 13 different herbicide modes of action (MOAs) in 10 major crops in China (Liu et al. Reference Liu, Xiang, Zong, Ma, Wu, Liu, Zhou and Bai2019). These resistant weeds pose a serious threat to chemical control in China and have therefore caught Chinese researchers’ attention. Many studies seek to monitor levels of resistance or decipher the causes of resistance. However, the general understanding of herbicide resistance in China is still in its early stages (Liu et al. Reference Liu, Xiang, Zong, Ma, Wu, Liu, Zhou and Bai2019). Previously, we carried out a systematic review to quantitatively assess herbicide-resistance issues in China (Liu et al. Reference Liu, Xiang, Zong, Ma, Wu, Liu, Zhou and Bai2019). In this review, however, we focus on mechanistic studies and management practices to document the (1) history of herbicide application in China; (2) resistance mechanisms of major herbicide groups; and (3) herbicide-resistance management strategies currently practiced in China.

Figure 1. The development of herbicide-resistant cases in China. Data from 1985 to 2019 were provided from the International Survey of Herbicide-Resistant Weeds (Heap Reference Heap2019).
History of Herbicide Application in China
Before the introduction of herbicides, weed control in China was generally limited to manual weeding, which is labor-intensive and not suitable for modernized agriculture (Zhang Reference Zhang2003). China began to study chemical weed control in the mid-1950s (Zhang Reference Zhang1997). The first introduced herbicide was 2,4-D, used to control broadleaf weeds in wheat (Triticum aestivum L.) (Zhang Reference Zhang1997). Subsequently, nitrofen and sodium pentachlorophenate were tested for barnyardgrass [Echinochloa crus-galli (L.) P. Beauv.] control in rice (Oryza sativa L.) in the early 1960s (Zhang Reference Zhang1997). In the 1970s, herbicide field trials and field demonstrations were carried out. Several herbicides, including thiobencarb, molinate, butachlor, chlortoluron, trifluralin, alachlor, and linuron, were applied in rice, wheat, soybean [Glycine max (L.) Merr.], cotton (Gossypium hirsutum L.), and other major crops (Zhang Reference Zhang2003). Use of herbicides steadily increased, becoming an important and attractive form of weed control for farmers (Zhang Reference Zhang1997, Reference Zhang2003). At the end of the 1990s, herbicides were employed extensively to control weeds in China, and by 2015 China had become one of the most important herbicide-producing and herbicide-consuming nations in the world (GACC 2015). Figure 2 presents the annual Chinese usage of insecticides, fungicides, herbicides, plant growth regulators, and rodenticides between 2012 and 2016. Herbicides were the second most used class of pesticides, only surpassed by insecticides. The amount of herbicides applied increased continuously, while insecticide applications decreased, even during the implementation of the Action Plan for Zero Increase in Pesticide Use by 2020 (Zhang et al. Reference Zhang, Mccarl, Luan and Kleinwechter2018c) released by the Chinese government to limit pesticide consumption without decreasing crop yields. There are several factors that influence the current situation in China. One major factor is the development of domestic herbicide production capacity, which allows herbicide prices to be reduced by more than 50%. Another factor is the shortage of workers to hand weed fields due to the spontaneous migration of rural labor to cities in pursuit of employment opportunities and increased wages (Gianessi Reference Gianessi2013; Haggblade et al. Reference Haggblade, Minten, Pray, Reardon and Zilberman2017; Huang et al. Reference Huang, Wang and Xiao2017). As a result, herbicides are in high demand and are viewed as the most reliable form of weed control in China.
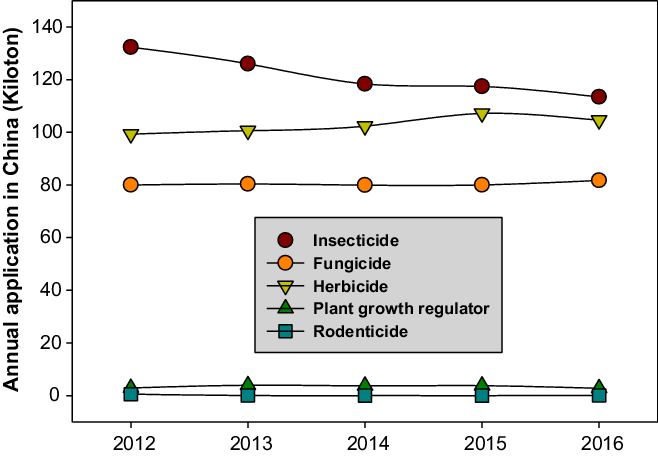
Figure 2. Pesticide application between 2012 and 2016 in China. Each data point represents the amount of active ingredient in pesticides. Data were collected from the Institute for the Control of Agrochemicals, Ministry of Agriculture, China.
Resistance Mechanisms of Major Herbicide Groups in China
Herbicide resistance commonly refers to the inherited ability of a weed population to survive and reproduce at regularly applied herbicide doses, with the final result being weed control failure (Norsworthy et al. Reference Norsworthy, Ward, Shaw, Llewellyn, Nichols, Webster, Bradley, Frisvold, Powles and Burgos2012). It is widely recognized that exposure to herbicides causes changes in weed populations over time via natural selection and the adaptive evolution of weeds (Beckie et al. Reference Beckie, Warwick and Sauder2012; Powles and Yu Reference Powles and Yu2010). Several factors influence herbicide-resistance evolution: (1) genetic factors, including frequency, number, dominance, and fitness cost of resistance genes; (2) biology of weed species, including cross-pollination versus self-pollination, seed production capacity, seed longevity in the soil seedbank and seed/pollen movement capacity; (3) herbicides used, including chemical structure, MOAs, and residual activity; and (4) operational factors, including herbicide dose, agroecosystem factors (nonchemical weed controls, such as crop rotation), and skills of the operator (treatment machinery, timing, and environmental conditions) (Powles and Yu Reference Powles and Yu2010). To effectively manage herbicide resistance, it is important to understand possible resistance mechanisms. Two major resistance mechanisms, non–target–site–based resistance (NTSR) and target–site–based resistance (TSR), are broadly grouped to explain the ability of herbicide-resistant (HR) weeds to survive herbicide treatments. TSR mechanisms focus on gene amplification of target enzymes or mutations that change enzyme or protein conformation and herbicide binding. NTSR mechanisms typically focus on reduced translocation in vascular tissue, sequestration in cell walls or vacuoles, reduced penetration, and enhanced metabolism (Délye et al. Reference Délye, Jasieniuk and Le Corre2013).
Currently, at least 11 weed species in China have evolved NTSR associated with changes in cytochrome P450 monooxygenase (P450) (Li et al. Reference Li, Zhang, Zhao, Guo, Liu, Li and Wang2017c), glutathione-S-transferase (GST) (Li et al. Reference Li, Wu, Yu, Cang, Chen, Zhao, Cai and Wu2013), glycosyltransferases (GTs) (Zhao et al. Reference Zhao, Li, Bai, Guo, Yuan, Wang, Liu and Wang2017b), ethylene biosynthesis (EB) (Xu et al. Reference Xu, Lv, Wang, Li and Dong2013b), ATP-binding cassette (ABC) transporters (Yang et al. Reference Yang, Deng, Li, Yu, Bai and Zheng2016), polyamines (An et al. Reference An, Shen, Ma, Yang, Liu and Chen2014), micro-RNAs (miRNAs), and several typical antioxidant enzymes (Pan et al. Reference Pan, Wang, Cai, Gao, Zhao and Dong2016, Reference Pan, Zhao, Yu, Bai and Dong2017) (Table 1). NTSR endowing herbicide resistance is less often reported, and its resistance mechanisms are poorly understood because of its more complexity and diversity compared with TSR mechanisms. However, it poses a greater challenge to HR weed management because of the potential for cross/multiple resistance to develop. GST- and P450-mediated metabolic resistance have been recognized as the primary NTSR mechanisms. In China, evolved P450-mediated resistance has been reported in several weeds, including American sloughgrass [Beckmannia syzigachne (Steud.) Fernald] (Li et al. Reference Li, Zhang, Zhao, Guo, Liu, Li and Wang2017c), shortawn foxtail (Alopecurus aequalis Sobol.) (Zhao et al. Reference Zhao, Li, Bai, Guo, Yuan, Wang, Liu and Wang2017b), water chickweed [Myosoton aquaticum (L.) Moench] (Liu et al. Reference Liu, Wu, Guo, Du, Yuan and Wang2015b), flixweed [Descurainia sophia (L.) Webb ex Prantl] (Yang et al. Reference Yang, Deng, Li, Yu, Bai and Zheng2016), perennial ryegrass (Lolium perenne L.) (Zhang et al. Reference Zhang, Wu, Xu, Gao, Zhang and Dong2017b), rice barnyardgrass [Echinochloa phyllopogon (Stapf) Koso-Pol.] (Zuo et al. Reference Zuo, Zang, Chen and Ji2016), threeleaf arrowhead (Sagittaria trifolia L.) (Zhao et al. Reference Zhao, Fu, Yu, Huang, Yan, Li, Shafi, Zhu, Wei and Ji2017a), redroot pigweed (Amaranthus retroflexus L.) (Wang et al. Reference Wang, Guo, Zhang, Zhao, Ge, Lv, Liu and Wang2017), large crabgrass [Digitaria sanguinalis (L.) Scop.] (Mei et al. Reference Mei, Si, Liu, Qiu and Zheng2017), and annual bluegrass (Poa annua L.) (Wang et al. Reference Wang, Li, Lv, Lou and Dong2013). Enhanced activity of GST and/or P450 alongside target-site gene mutations often contribute to herbicide resistance, as in M. aquaticum resistance to tribenuron-methyl (Liu et al. Reference Liu, Wu, Guo, Du, Yuan and Wang2015b).
Table 1. Weeds with a non–target site based resistance mechanism(s) in China. a

a Abbreviations: HRAC, Herbicide Resistance Action Committee; ABC, ATP-binding cassette; ACCase, acetyl-CoA carboxylase; ALS, acetolactate synthase; EPSPS, 5-enolpyruvylshikimate-3-phosphate synthase; GST, glutathione-S-transferase; GT, glycosyltransferases; miRNAs, micro-RNAs; PSI, photosystem I; PSII, photosystem II; P450, cytochrome P450 monooxygenase.
A recent review on herbicide resistance in China indicated that acetolactate synthase (ALS) inhibitors, acetyl-CoA carboxylase (ACCase) inhibitors, synthetic auxin herbicides, 5-enolpyruvylshikimate-3-phosphate synthase (EPSPS) inhibitors, protoporphyrinogen oxidase (PPO) (also known as protoporphyrinogen IX oxidase) inhibitors, photosystem I (PSI) electron diverters, photosystem II (PSII) inhibitors, and long-chain fatty-acid (LCFA) inhibitors are the herbicide groups most prone to developing resistance, accounting for 97% of developed resistance in China (Liu et al. Reference Liu, Xiang, Zong, Ma, Wu, Liu, Zhou and Bai2019). To manage HR weeds in farmland, it is very important to understand the mechanisms of these eight herbicide groups.
ALS Inhibitors
ALS-inhibiting herbicides include five groups based on molecular structure: sulfonylureas (SUs), imidazolinones (IMIs), pyrimidinylthiobenzoates (PTBs), triazolopyrimidines (TPs), and sulfonylaminocarbonyltriazolinone (SCT). These herbicides control weeds by inhibiting ALS, which is a key enzyme for biosynthesis of branch-chained amino acids. Almost all studies on the mechanisms of ALS inhibitor resistance focus on modifications of the ALS enzyme. A total of 160 weed species (62 monocots and 98 dicots) have evolved resistance to ALS inhibitors globally due to eight mutations and 29 distinct amino acid substitutions located in the ALS gene: Ala-122-Thr/Val/Tyr/Ser/Asn, Pro-197-Thr/His/Arg/Leu/Gln/Ser/Ala/Ile/Asn/Glu/Tyr, Ala-205-Val/Phe, Asp-376-Glu, Arg-377-His, Trp-574-Leu/Gly/Met/Arg, Ser-653-Thr/Asn/Ile, and Gly-654-Val/Asp (Heap Reference Heap2019). Generally, ALS inhibitors of different groups are associated with different amino acid substitutions in resistant weeds. Substitutions of Ala-122 or Ser-653 result in IMI but not in SU resistance, whereas substitutions of Pro-197 usually result in SU but not in IMI resistance. In some cases, low to moderate levels of IMI resistance have also been found in biotypes with the Pro-197 substitution. Substitutions of Trp-574 result in high levels of both IMI and SU resistance. Substitutions of Pro-197 and Ala-205 usually lead to cross-resistance (Tranel and Wright Reference Tranel and Wright2002). In China, there are five mutations with 13 amino acid substitutions in 10 weed species that provide known TSR to ALS inhibitors. The most common amino acid substitutions providing TSR to ALS inhibitors are found at residue Pro-197 in pickerelweed [Monochoria vaginalis (Burm. f.) C. Presl. ex Kunth] (Pro-197-Ser) (Wang et al. Reference Wang, Lin, Li, Ito and Itoh2004), D. sophia (Pro-197-Ser/Leu/Ala/Thr/His/Tyr) (Cui et al. Reference Cui, Zhang, Wei, Zhang, Li, Zhang and Wang2011; Deng et al. Reference Deng, Liu, Yang, Mei, Li and Zheng2015), shepherd’s purse [Capsella bursa-pastoris (L.) Medik.] (Pro-197-Ser/Leu/Thr/His/Tyr/Arg/Ala) (Zhang et al. Reference Zhang, Guo, Li, Wu, Zhao, Liu and Wang2017a), M. aquaticum (Pro-197-Ser/Glu) (Liu et al. Reference Liu, Bi, Li, Yuan, Du and Wang2013, Reference Liu, Yuan, Du, Guo, Li, Bi and Wang2015a), and A. aequalis (Pro-197-Thr/Arg) (Guo et al. Reference Guo, Lv, Zhang, Li, Wu, Lu, Liu and Wang2016; Xia et al. Reference Xia, Pan, Li, Wang, Feng and Dong2015). The Trp-574-Leu mutation is also linked in several weed species to TSR to ALS inhibitors, including A. aequalis (Guo et al. Reference Guo, Yuan, Liu, Bi, Du, Zhang, Li and Wang2015), D. sophia (Deng et al. Reference Deng, Yang, Zhang, Jiao, Mei, Li and Zheng2017), A. retroflexus (Chen et al. Reference Chen, Huang, Zhang, Huang, Wei, Chen and Wang2015b), and Japanese foxtail (Alopecurus japonicus Steudel) (Bi et al. Reference Bi, Liu, Guo, Li, Yuan, Du and Wang2016) (Table 2). These mutations at different sites or domains render ALS inhibitors unable to bind at the catalytic site of the target enzyme, resulting in increased resistance to ALS-inhibiting herbicides (Powles and Yu Reference Powles and Yu2010). In many resistance cases, however, NTSR and TSR can coexist. For example, the ALS gene mutation Pro-197-Thr in combination with two non–target site based genes, CYP96A13 and ABCC1, confers resistance to tribenuron-methyl, an ALS inhibitor, in D. sophia (Yang et al. Reference Yang, Deng, Li, Yu, Bai and Zheng2016). In M. aquaticum, GST/P450-mediated metabolic resistance combined with a Pro-197-Ser mutation is involved in herbicide resistance to ALS inhibitors (Liu et al. Reference Liu, Wu, Guo, Du, Yuan and Wang2015b).
Table 2. Mutations in weeds resistant to acetolactate synthase inhibitors in China.

a Amino acid followed by the location/site of substitution.
ACCase Inhibitors
ACCase-inhibiting herbicides can be divided into three classes based on chemical structure: aryloxyphenoxypropionates (APPs, also known as FOPs), cyclohexanediones (CHDs, also known as DIMs), and phenylpyrazolins (PPZs, also known as DENs) (Kaundun Reference Kaundun2014). ACCase inhibitors are used to control Poaceae weeds by inhibiting ACCase, which is a critical enzyme in fatty-acid synthesis. Likewise, the resistance mechanisms of ACCase inhibitors generally belong to TSR. A total of 10 mutations at codon positions 1734, 1738, 1739, 1781, 1999, 2027, 2041, 2078, 2088, and 2096 are determined by dominant alleles and confer resistance to ACCase inhibitors. The most common mutations are located at residue 1781 (Powles and Yu Reference Powles and Yu2010). Seventeen distinct amino acid substitutions located in the carboxyl transferase (CT)domain of the plastidic ACCase gene have been reported worldwide: Arg-1734-Gly (Tang et al. Reference Tang, Li, Dong, Dong, Lu and Zhu2012); Met-1738-Leu (Tang et al. Reference Tang, Li, Dong, Dong, Lu and Zhu2012); Thr-1739-Ser (Tang et al. Reference Tang, Li, Dong, Dong, Lu and Zhu2012); Ile-1781-Leu (Xia et al. Reference Xia, Pan, Li, Wang, Feng and Dong2015), -Val (Collavo et al. Reference Collavo, Panozzo, Lucchesi, Scarabel and Sattin2011), -Thr (Kaundun et al. Reference Kaundun, Hutchings, Dale and McIndoe2013); Trp-1999-Cys (Xu et al. Reference Xu, Li, Zhang, Cheng, Jiang and Dong2014), -Leu (Xu et al. Reference Xu, Li, Zhang, Cheng, Jiang and Dong2014), -Ser (Yuan et al. Reference Yuan, Liu, Bi, Du, Guo and Wang2015); Trp-2027-Cys (Du et al. Reference Du, Liu, Yuan, Guo, Li and Wang2016; Li et al. Reference Li, Du, Liu, Yuan and Wang2014; Yu et al. Reference Yu, Gao, Pan, Yao and Dong2017); Ile-2041-Asn (Tang et al. Reference Tang, Zhou, Zhang and Chen2015), -Val (Délye et al. Reference Délye, Zhang, Chalopin, Michel and Powles2003), -Thr (Guo et al. Reference Guo, Zhang, Wang, Li, Liu and Wang2017); Asp-2078-Gly (Guo et al. Reference Guo, Yuan, Liu, Bi, Du, Zhang, Li and Wang2015); Cys-2088-Arg (Yu et al. Reference Yu, Collavo, Zheng, Owen, Sattin and Powles2007); and Gly-2096-Ala (Du et al. Reference Du, Liu, Yuan, Guo, Li and Wang2016), -Ser (Beckie et al. Reference Beckie, Warwick and Sauder2012). These different amino acid substitutions can confer diverse cross-resistance to different ACCase-inhibiting herbicides. In Chinese cropping systems, at least seven weed species have evolved target-site ACCase resistance: A. japonicus (Tang et al. Reference Tang, Li, Dong, Dong, Lu and Zhu2012), A. aequalis (Xia et al. Reference Xia, Pan, Li, Wang, Feng and Dong2015), B. syzigachne (Du et al. Reference Du, Liu, Yuan, Guo, Li and Wang2016), keng stiffgrass [Pseudosclerochloa kengiana (Ohwi) Tzvelev] (Yuan et al. Reference Yuan, Liu, Bi, Du, Guo and Wang2015), Chinese sprangletop [Leptochloa chinensis (L.) Nees] (Yu et al. Reference Yu, Gao, Pan, Yao and Dong2017), Asia minor bluegrass (Polypogon fugax Nees ex Steud.) (Tang et al. Reference Tang, Zhou, Chen and Zhou2014), and E. crus-galli (Huan et al. Reference Huan, Jin, Zhang and Wang2011a). Table 3 shows that a total of nine mutations at codon positions and 11 distinct amino acid substitutions confer resistance to ACCase inhibitors in China.
Table 3. Mutations in weeds resistant to acetyl-CoA carboxylase inhibitors in China.

a Amino acid followed by the location/site of substitution.
In addition to amino acid substitutions, various forms of NTSR can also confer resistance to ALS and ACCase inhibitors. These NTSR mechanisms include increases in metabolism, reductions in herbicide absorption, and impaired translocation of herbicide molecules. P450s, ABC transporters, GT, and protective enzymes, such as superoxide dismutase, catalase, and peroxidase, could play important roles in conferring NTSR to weed species in China (Table 1). Mechanisms of both TSR and NTSR to mesosulfuron-methyl and fenoxaprop-P-ethyl were identified in an A. aequalis population (Zhao et al. Reference Zhao, Li, Bai, Guo, Yuan, Wang, Liu and Wang2017b). These concurrent forms of resistance pose significant challenges to the management of resistance. Additionally, in comparison to TSR, mechanisms of NTSR to ACCase and ALS inhibitors are much less well known, because they can confer resistance to herbicides with different target sites. As a result, resistance to ACCase- and ALS-inhibiting herbicides has major impacts on agriculture in China.
Synthetic Auxin Herbicides
In contrast to resistance to ACCase and ALS inhibitors, the incidence of weed resistance to synthetic auxin herbicides is much lower, although these herbicides have been in use for more than 30 yr in China. There have been relatively few research advances involving molecular resistance mechanisms of weeds to synthetic auxins, because elucidating the mechanisms of resistance to this class of herbicides is particularly difficult (Powles and Yu Reference Powles and Yu2010). In China, 12 weed species have developed resistance to synthetic auxins such as quinclorac, MCPA (4-chloro-2-ethyphenoxyacetate), 2,4-D butylate, and fluroxypyr (Liu et al. Reference Liu, Xiang, Zong, Ma, Wu, Liu, Zhou and Bai2019). Quinclorac is widely used for weed control during rice production in China and is the most resistance-prone synthetic auxin herbicide used. Its intensive use has selected for herbicide resistance to quinclorac in eight Echinochloa species (Peng et al. Reference Peng, Han, Yang, Bai, Yu and Powles2019). The mechanisms of resistance to quinclorac center on components of the ethylene biosynthesis pathway, including 1-aminocyclopropane-1-carboxylic acid synthase, 1-aminocyclopropane-1-carboxylic acid oxidase, as well as those of the cyanide detoxification pathway via β-cyanoalanine synthase (Gao et al. Reference Gao, Pan, Sun, Zhang, Dong and Li2017, Reference Gao, Li, Pan, Liu, Napier and Dong2018; Xu et al. Reference Xu, Lv, Wang, Li and Dong2013b). There are few known mechanisms of resistance to MCPA, 2,4-D butylate, and fluroxypyr. Resistance to MCPA generally involves changes in translocation or metabolism (Busi et al. Reference Busi, Goggin, Heap, Horak, Jugulam, Masters, Napier, Riar, Satchivi and Torra2018). It is hoped that the mechanistic biochemical and molecular basis of evolved resistance to synthetic auxin herbicides will be deciphered in the next few years.
EPSPS Inhibitors
Of the commonly used EPSPS inhibitors, glyphosate is the only member of the group for which resistance has been observed. Worldwide, a total of 47 weed species have evolved resistance to glyphosate (Heap Reference Heap2019; Liu et al. Reference Liu, Xiang, Zong, Ma, Wu, Liu, Zhou and Bai2019). In China, a total of eight weed species have developed resistance to glyphosate within three crops: goosegrass [Eleusine indica (L.) Gaertn.], D. sanguinalis, L. chinensis, A. retroflexus, and common purslane (Portulaca oleracea L.) in cotton; E. indica, horseweed [Conyza canadensis (L.) Cronquist], Asian copperleaf (Acalypha australis L.), and C. canadensis in orchard crops; and C. canadensis in ramie (Liu et al. Reference Liu, Xiang, Zong, Ma, Wu, Liu, Zhou and Bai2019). Both TSR and NTSR to glyphosate have been confirmed. Overexpression of EPSPS, a target enzyme, and mutations at amino acid positions Thr-102 and Pro-106 confer glyphosate TSR in E. indica, coatbuttons (Tridax procumbens L.), and C. canadensis in Chinese crop fields (Chen et al. Reference Chen, Huang, Zhang, Wei, Huang, Chen and Wang2015a; Gherekhloo et al. Reference Gherekhloo, Fernández-Moreno, Alcántara-de la Cruz, Sánchez-González, Cruz-Hipolito, Domínguez-Valenzuela and De Prado2017; Mei et al. Reference Mei, Xu, Wang, Qiu and Zheng2018; Yu et al. Reference Yu, Jalaludin, Han, Chen, Sammons and Powles2015). Glyphosate NTSR is endowed by restricted glyphosate translocation and sequestration to vacuoles in addition to glyphosate metabolization, controlled by the aldo-keto reductase (AKR) gene (Ge et al. Reference Ge, d’Avignon, Ackerman and Sammons2010; Lorraine-Colwill et al. Reference Lorraine-Colwill, Powles, Hawkes, Hollinshead, Warner and Preston2002; Pan et al. Reference Pan, Yu, Han, Mao, Nyporko, Fan, Bai and Powles2019). It has been demonstrated that the AKR gene EcAKR4-1 from jungle rice [Echinochloa colona (L.) Link] confers glyphosate resistance (Pan et al. Reference Pan, Yu, Han, Mao, Nyporko, Fan, Bai and Powles2019).
PPO Inhibitors
Currently, PPO-inhibiting herbicides include nine groups based on molecular structure: N-phenylphthalimides, diphenylethers, phenylpyrazoles, pyrimidindiones, oxadiazoles, oxazolidinediones, thiadiazoles, triazinones, and triazolinones. These herbicides control weeds by inhibiting PPO, a crucial enzyme in the biosynthesis of heme and chlorophyll, and catalyzing the oxidation of protoporphyrinogen to protoporphyrin IX. Some PPO inhibitors have been used for many years, but there has been little observed evolution of resistance to these herbicides. The possible reason for the slow evolution of weeds resistant to PPO inhibitors is the two target sites (PPO1 and PPO2) used by PPO inhibitors, compared with the single target site used by other enzyme-inhibiting herbicides (e.g., ALS inhibitors, ACCase inhibitors).To date, only 14 weed species have evolved resistance to PPO inhibitors globally (Heap Reference Heap2019). Deletion of amino acid Gly-210 in waterhemp [Amaranthus tuberculatus (Moq.) Sauer] (Lee et al. Reference Lee, Hager and Tranel2008; Patzoldt et al. Reference Patzoldt, Hager, McCormick and Tranel2006) and amino acid substitutions Arg-98-Leu in common ragweed (Ambrosia artemisiifolia L.) (Rousonelos et al. Reference Rousonelos, Lee, Moreira, VanGessel and Tranel2012), Arg-98-Gly and Arg-98-Met in Palmer amaranth (Amaranthus palmeri S. Watson) (Giacomini et al. Reference Giacomini, Umphres, Nie, Mueller, Steckel, Young, Scott and Tranel2017), Gly-399-Ala in A. palmeri (Rangani et al. Reference Rangani, Salas-Perez, Aponte, Knapp, Craig, Mietzner, Langaro, Noguera, Porri and Roma-Burgos2019), and Ala-212-Thr in E. indica (Bi et al. Reference Bi, Wang, Coleman, Porri, Peppers, Patel, Betz, Lerchl and McElroy2020) contribute to TSR to PPO-inhibiting herbicides. There are few reported cases of evolved NTSR to PPO inhibitors (Dayan et al. Reference Dayan, Owens, Tranel, Preston and Duke2014). In China, seven weed species, including E. crus-galli, barnyardgrass (Echinochloa glabrescens Munro ex Hook. f.), rough barnyardgrass [Echinochloa crus-galli var. mitis (Pursh) Peterm.], Chinese arrowhead [Sagittaria trifolia var. sinensis (Sims) Makino], D. sophia, Chinese melon [Cucumis melo var. agrestis (L.) Naudin], Asiatic dayflower (Commelina communis L.), and A. retroflexus, have evolved resistance to five PPO-inhibiting herbicides, including oxadiazon, oxyfluorfen, carfentrazone-ethyl, fluoroglycofen, and fomesafen (Liu et al. Reference Liu, Xiang, Zong, Ma, Wu, Liu, Zhou and Bai2019). These cases of resistance to PPO inhibitors are brief reports without deciphered information on the resistance mechanisms. Although natural resistance to PPO-inhibiting herbicides has evolved slowly (Powles and Yu Reference Powles and Yu2010), it may occur more frequently in weed species with large populations under continuous and extensive herbicide use (Jasieniuk et al. Reference Jasieniuk, Brûlé-Babel and Morrison1996).
Photosystem I Electron Diverters
PSI electron diverters are primarily non-translocated herbicides. These herbicides control weeds by accepting electrons from the PSI protein complex and subsequently transferring them to molecular oxygen to generate herbicide radicals. These radicals promote the formation of dangerous molecules such as reactive oxygen species (ROS), which can destroy membrane lipids, damage chlorophyll, and induce cell death (UCIPM 2020). Globally, 73 unique cases (species by site of action) of resistance to PSI electron diverters have been reported (Heap Reference Heap2019). Almost all of these cases involve paraquat resistance (Heap Reference Heap2019). In China, a total of six weed species, including A. japonicus, tall fleabane [Conyza sumatrensis (Retz.) E. Walker], E. indica, Asian mazus [Mazus fauriei Bonati], common hardgrass [Sclerochloa dura (L.) P. Beauv.], and Oriental false hawkbear [Youngia japonica (L.) DC], have developed resistance to paraquat (Dong et al. Reference Dong, Hu, Peng and Feng2015). At least five NTSR mechanisms have been proposed for paraquat resistance in plant cells, including uptake, efflux, sequestration, detoxification, and catabolism of the ROS produced by the herbicide (Xi et al. Reference Xi, Xu and Xiang2012). Vacuolar sequestration has been recognized and extensively reported as the major mechanism of paraquat resistance in weeds (Brunharo and Hanson Reference Brunharo and Hanson2017; Hawkes Reference Hawkes2014). Several functional genes that play important roles in sequestering paraquat away from chloroplasts and into the vacuole have been identified, but further research is required to understand how these genes are regulated (Fujita and Shinozaki Reference Fujita and Shinozaki2014; Luo et al. Reference Luo, Wei, Dong, Shen and Chen2019). Regarding the possibility of TSR to paraquat, it is debatable whether paraquat has any specific binding site from which it accepts electrons (Hawkes Reference Hawkes2014). To date, a binding site mutation-based mechanism for paraquat resistance has not been identified (Hawkes Reference Hawkes2014; Luo et al. Reference Luo, Wei, Dong, Shen and Chen2019; Powles and Yu Reference Powles and Yu2010).
Photosystem II Inhibitors
PSII inhibitors control weeds by inhibiting photosynthesis. This is accomplished by the herbicide binding to D1 proteins located in the photosystem II complex (UCIPM 2020). The binding occurs at three different attachment sites. Based on their attachment sites, PSII-inhibiting herbicides are classified into three groups by the Herbicide Resistance Action Committee (HRAC). Group C1 includes pyridazinone, triazine, triazinone, and uracil herbicides; group C2 includes ureas and amides; and group C3 includes benzothiadiazinone, nitrile, and phenylpyridazine herbicides. To date, there have been 107 unique cases (species by site of action) of resistance to PSII-inhibiting herbicides globally (Heap Reference Heap2019). In China, a total of five weed species (manyflower redstem [Ammannia multiflora Roxb.], common chickweed [Stellaria media (L.) Vill.], catchweed bedstraw (Galium aparine L.), A. japonicus, B. syzigachne) have developed resistance to PSII-inhibiting herbicides (Liu et al. Reference Liu, Xiang, Zong, Ma, Wu, Liu, Zhou and Bai2019). TSR to PSII-inhibiting herbicides is mainly due to seven mutations within the psbA gene encoding the D1 protein that results in nine distinct amino acid substitutions in resistant plants: Phe-274-Val, Ser-264-Gly/Thr, Asn-266-Thr, Phe-255-Ile, Ala-251-Val/Thr, Val-219-Ile, and Leu-218-Val (Lu et al. Reference Lu, Yu, Han, Owen and Powles2019b; McMurray et al. Reference McMurray, Preston, Vandenberg, Mao, Bett and Paull2019; Powles and Yu Reference Powles and Yu2010; Thiel and Varrelmann Reference Thiel and Varrelmann2014). The most common amino acid substitution providing TSR to PSII inhibitors is Ser-264-Gly. NTSR to PSII-inhibiting herbicides is due to enhanced metabolism mediated by P450s and/or GSTs (Anderson and Gronwald Reference Anderson and Gronwald1991; Busi et al. Reference Busi, Vila-Aiub and Powles2011). Commonly, TSR along with NTSR induces resistance in many weed species to PSII-inhibiting herbicides, such as P. annua resistance to atrazine, amicarbazone, and diuron (Svyantek et al. Reference Svyantek, Aldahir, Chen, Flessner, McCullough, Sidhu and McElroy2016) and wild radish (Raphanus raphanistrum L.) resistance to metribuzin (Lu et al. Reference Lu, Yu, Han, Owen and Powles2019a).
Long-Chain Fatty-Acid Inhibitors
LCFA inhibitors include acetamide, chloroacetamide, oxyacetamide, and tetrazolinone herbicides. These herbicides control weeds by inhibiting LCFA synthesis. Cases of resistance to LCFA-inhibiting herbicides have been reported in many countries (Heap Reference Heap2019). In China, a total of eight weed species are currently resistant to LCFA-inhibiting herbicides, including pretilachlor and butachlor (Liu et al. Reference Liu, Xiang, Zong, Ma, Wu, Liu, Zhou and Bai2019). Pretilachlor resistance is attributed to high cytochrome P450 activity in some perennial weeds (Shim et al. Reference Shim, Yun, Kobayashi and Usui2002), but there are no reports on the exact mechanisms of resistance to pretilachlor. However, several mechanisms conferring resistance to butachlor have been elucidated. Butachlor resistance can be caused by α-amylase activation (Huang et al. Reference Huang, Lin and Xiao1995), increased hydrolase activity (Huang and Lin Reference Huang and Lin1993), and increased protease activity (Kumar and Prakash Reference Kumar and Prakash1994) in resistant plants. Another possible resistance mechanism may be related to the accumulation of phytoalexins, which is considered a defense mechanism in higher plants. After treatment with pretilachlor and butachlor, the phytoalexin momilactone was greatly increased in rice. The accumulation of momilactone may be required for rice protection and growth regulation. However, the mechanism of resistance of pretilachlor/butachlor-resistant E. crus-galli has not yet been determined (Tamogami et al. Reference Tamogami, Kodama, Hirose and Akatsuka1995).
Resistance Mechanisms of Major Herbicide Groups in China
Overall, for the herbicide groups most prone to resistance in China presented here, more research has focused on mechanisms of TSR than on those of NTSR. The possible reason is that NTSR mechanisms are more complex than those of TSR. Generally, target-site mutations provide high-level resistance and can be identified rapidly by molecular markers. NTSR is often ignored, because it generally confers lower resistance levels and is often linked with TSR (Liu et al. Reference Liu, Wu, Guo, Du, Yuan and Wang2015b; Pan et al. Reference Pan, Li, Zhang, Zhang and Dong2015; Wang et al. Reference Wang, Guo, Zhang, Zhao, Ge, Lv, Liu and Wang2017; Zhang et al. Reference Zhang, Wu, Xu, Gao, Zhang and Dong2017b; Zhao et al. Reference Zhao, Li, Bai, Guo, Yuan, Wang, Liu and Wang2017b). In addition, due to the limited genomic information available for weeds, fewer NTSR mechanisms have been elucidated at the molecular level. Only a few CytP450 genes have been identified as contributing to resistance in weeds such as B. syzigachne, D. sophia, and A. aequalis (Yang et al. Reference Yang, Deng, Li, Yu, Bai and Zheng2016; Zhao et al. Reference Zhao, Li, Bai, Guo, Yuan, Wang, Liu and Wang2017b). However, NTSR could be more concerning, because it could confer resistance to multiple classes of herbicides. Farmers will face a reduced number of herbicide alternatives, because weeds will gradually evolve resistance to existing, new, or yet-to-be-discovered herbicides (Powles and Yu Reference Powles and Yu2010). Therefore, NTSR is currently unpredictable and a troublesome problem for weed control. In the near future, herbicide-resistance research should center on the evolution and management of NTSR.
Herbicide-Resistance Management in China
Based on the occurrence of HR weeds and advances in resistance mechanisms, weed researchers in China have made great efforts in developing resistance management strategies during the last few decades. We searched through literature published from 1980 to 2018 using the China National Knowledge Infrastructure (CNKI) and Web of Science databases, then manually screened for resistance management strategies. A total of 158 records were retrieved from the two databases; among those records, strategies generally involved a combination of early preventative measures and one or more major management strategies once resistance was detected. Major management strategies include chemical, cultural, biological, and physical practices; of those resistance management strategies, approximately 46.4% were chemical, 25.0% were cultural, 17.9% were biological, 5.4% were early preventative, and 5.3% were physical (Figure 3). Based on these results, chemical strategies are the mainstay for managing herbicide resistance in China.

Figure 3. Major herbicide-resistance management practices in China. The current management practices used to combat herbicide-resistance issues in China include early preventative, chemical, cultural, biological, and physical strategies. Based on percentages (in parentheses), synthetic herbicides (~50%) have dominated control practices.
Early Preventative Measures
Early preventative measures include resistance detection, risk assessment, and government policy, which can provide important scouting information for resistance management. Currently, a series of rapid and simple resistance-detection technologies have been developed. Resistance of E. crus-galli to quinclorac can be rapidly identified using label cards in combination with a water-culture method for growing plants without soil (Li et al. Reference Li, Tang, Liu, Liu, Bai, Ma and Peng2015b) or with DNA technologies coupled with sequencing information (Chen et al. Reference Chen, Zhang, Fang and Dong2017a). Computational models are often used in the development of novel herbicides (Choe et al. Reference Choe, Choe, Lee, Wu and Liu2015; Qu et al. Reference Qu, Yang, Liu, Chen, Hao, Niu, Xi and Yang2017b; Zhu et al. Reference Zhu, Yang, Yu, Yang and Yang2011). In a simulation of the evolution of ALS inhibitor resistance associated with the ALS Pro-197-Leu mutation, a series of novel derivatives of 2-benzoyloxy-6-pyrimidinyl salicylic acid were designed and displayed strong inhibitory activity against highly-resistant D. sophia (Qu et al. Reference Qu, Yang, Devendar, Kang, Liu, Chen, Niu, Xi and Yang2017a). However, modeling is only a preliminary tool used to compare the theoretical advantages of management practices. It is also necessary that researchers implement long-term field trials to evaluate novel strategies, such as studies on the population dynamics and possible future populations of weeds (Jones and Medd Reference Jones and Medd2000) or on the potential and capacity for soil mineralization after anti-resistance herbicide application (Cheyns et al. Reference Cheyns, Martin-Laurent, Bru, Aamand, Vanhaecke, Diels, Merckx, Smolders and Springael2012; Jones and Medd Reference Jones and Medd2005; Neve et al. Reference Neve, Norsworthy, Smith and Zelaya2011a, Reference Neve, Norsworthy, Smith and Zelaya2011b). In addition, government policies such as exotic pest and weed quarantine policies contribute to the prevention of herbicide resistance and limit the spread of invasive resistant species (Davis and Frisvold Reference Davis and Frisvold2017). Furthermore, to delay or relieve the development of herbicide resistance, diverse management strategies, including chemical, cultural, biological, and physical practices, as well as the use of HR crops, have been established (Norsworthy et al. Reference Norsworthy, Ward, Shaw, Llewellyn, Nichols, Webster, Bradley, Frisvold, Powles and Burgos2012; Vencill et al. Reference Vencill, Nichols, Webster, Soteres, Mallory-Smith, Burgos, Johnson and McClelland2012).
Chemical Management
Chemical management mainly includes herbicide sequence; herbicide rotation; development of novel herbicides and herbicide mixtures; use of synergists, new formulations, or new adjuvants; and use of full herbicide rates. When a resistance mechanism endows TSR, herbicide sequences, herbicide rotations, and herbicide mixtures have generally played a key role in delaying resistance (Gressel and Segel Reference Gressel and Segel1990). Herbicide sequence refers to more than one application of herbicides with different MOAs within one cropping system during a single growing season, whereas herbicide rotation refers to the application of herbicides with different MOAs to multiple crops over multiple growing seasons in a field (Beckie Reference Beckie2006). In recent years, adoption of herbicide sequences and rotations has increased markedly in China. However, the two tactics urgently require different herbicides with new MOAs. Disappointingly, no commercial herbicides with new MOAs have been released over the past 30 yr (Davis and Frisvold Reference Davis and Frisvold2017), prompting growers and herbicide companies to shift from new herbicides to herbicide mixtures, which are more effective at managing resistance than herbicide rotations (Beckie and Harker Reference Beckie and Harker2017). According to the website of the China Pesticide Information Network (www.icama.org.cn), Chinese agricultural chemical industries have developed a series of mixtures, such as penoxsulam mixed with mesotrione/fenoxaprop-P-ethyl/fluroxypyr-meptyl, cyhalofop-butyl with bispyribac-sodium/metamifop/pyribenzoxim, and mesotrione with pyrazosulfuron-ethyl/pretilachlor/simetryn, that are used to control resistant populations of E. crus-galli, L. chinensis, M. vaginalis, and smallflower umbrella sedge (Cyperus difformis L.) in rice. Additionally, the mixture of bentazone with fomesafen is used to delay or prevent the development of ALS and/or PPO resistance in weeds. Although herbicide mixtures are less costly, there are no effective tank mixes for contact and systemic herbicides because of antagonism. It is worth noting that cross-resistance and multiple resistance would be delayed or relieved when herbicide sequence, rotation, and/or mixture are used concurrently. When weeds develop NTSR, synergist application, formulation changes, and the use of new adjuvants also aid in managing herbicide resistance by increasing the concentration of herbicidal ingredients at the target site. In China, a series of new formulations (microcapsules, ionic liquids, etc.) (Tang et al. Reference Tang, Niu, Zhang, Yang, Tang, Tang, Zhou, Li and Cao2020; Zhang et al. Reference Zhang, Zhang, Luo, Li, Wei and Liu2018a) and adjuvants (adjuvant methylated seed oil, KAO® adjuvants A-134 [Kao Corporation, Tokyo, Japan] and A-178 [Kao Corporation, Shanghai, China], etc.) (Hao et al. Reference Hao, Zhang, Xu, Gao, Zhang and Tao2019a, Reference Hao, Zhang, Xu, Gao and Tao2019b; Zhang et al. Reference Zhang, Zheng, Jack, Yan, Zhang, Gerhards and Ni2013) have been applied to combat herbicide resistance. In addition, herbicide application at the full recommended rate is key to preventing weeds from rapidly evolving resistance. Research has demonstrated that lower than recommended dose selection favors the rapid evolution of polygenic resistance (Lagator et al. Reference Lagator, Vogwill, Mead, Colegrave and Neve2013; Manalil et al. Reference Manalil, Busi, Renton and Powles2011; Neve and Powles Reference Neve and Powles2005; Yu et al. Reference Yu, Han, Cawthray, Wang and Powles2013). Neve and Powles (Reference Neve and Powles2005) demonstrated that susceptible annual ryegrass (Lolium rigidum Gaudin) can evolve resistance to diclofop-methyl after only three recurrent rounds of selection.
Herbicide-Resistant Crop Management
Another chemical strategy is the introduction and adoption of HR crops, which can theoretically reduce the use of herbicides and achieve better yields than conventional crops. However, existing data suggest that this is not the case, and in fact HR crops may negatively affect agronomy, agricultural practices, and weed management, as well as lead to biodiversity loss (Schütte et al. Reference Schütte, Eckerstorfer, Rastelli, Reichenbecher, Restrepo-Vassalli, Ruohonen-Lehto, Saucy and Mertens2017). During continuous glyphosate/glufosinate-resistant crop cultivation, weed scientists recommend that farmers apply other herbicides (e.g., synthetic auxins) in tank mixtures with glyphosate/glufosinate rather than simply using higher rates of glyphosate/glufosinate for increased control efficiency (Schütte et al. Reference Schütte, Eckerstorfer, Rastelli, Reichenbecher, Restrepo-Vassalli, Ruohonen-Lehto, Saucy and Mertens2017). It is also noted that gene flow by pollen or seed dispersal from HR crops to weeds may cause the rise of HR superweeds, greatly jeopardizing the potential benefits of HR crops (Bain et al. Reference Bain, Selfa, Dandachi and Velardi2017). In China, glyphosate-resistant soybean, cotton, and maize (Fan et al. Reference Fan, Feng, Weaver, Delaney, Wehtje and Wang2017; Ren et al. Reference Ren, Cao, Zhang, Liu and Liu2015; Zhang et al. Reference Zhang, Tang, Wang and Wang2017c) and glufosinate-resistant rice and canola (Brassica napus L.) (Cai et al. Reference Cai, Zhou, Guo, Dong, Hu, Hou and Liu2008; Cui et al. Reference Cui, Liu, Li, Zhou, Chen and Lin2016), have been extensively studied. However, no commercial HR crops are currently approved for cultivation by the Chinese government.
Cultural Management
To increase crop competitiveness, maximize the growth of crops, and diminish weed germination and emergence, diverse cultural management is applied through crop rotation and intercropping, cultivar selection, water and fertilizer use practices, mulching, optimization of timing and density of crop planting, and use of allelopathic and cover crops. These cultural practices disrupt the life cycles of weeds and change the surrounding weed community based on the growth characteristics of each crop (Buhler Reference Buhler2002). In Chinese agricultural production, crop rotation is usually adopted by rotation of a grass crop (e.g., corn, wheat, rice) with a broadleaf crop (e.g., soybean, cotton, canola), or rotation of a wetland crop (e.g., rice) with a dryland crop (e.g., wheat) (Liu et al. Reference Liu, Zong, Li, Zhou and Bai2018; Zhang Reference Zhang2003). In addition, growers often attempt to enhance crop competitiveness through the use of mulching (Yang et al. Reference Yang, Mi, Tan, Wu and Onipchenko2018), allelopathy (Li et al. Reference Li, Liu, Zhou, Li and Bai2019), cover crops, and full-season cultivars combined with suitable planting timing and planting density (Deng et al. Reference Deng, Chen, Meng, Wu and Zhang2010). These methods aid in inhibiting the emergence and growth of HR weeds, thus reducing seed yield of the weeds and decreasing the soil seedbank. These cultural tactics are important for resistance management in China. However, cultural management tactics utilizing suppression, competition, and shading do not provide complete weed control.
Biological Management
Biological management is achieved by using living organisms or their products to reduce population, growth, and reproduction of weeds (Charudattan Reference Charudattan2005). Classical biological methods making use of a natural enemy of weeds, such as herbivorous animals, insects, and pathogenic microorganisms, have proven to be practical and effective strategies for HR weed control in China. In Chinese rice production, considerable success has been observed by raising fish, ducks, geese, and other herbivorous animals in paddy fields. The use of the leaf beetle Agasicles hygrophila, to control alligatorweed [Alternanthera philoxeroides (Mart.) Griseb.], is another successful case of biological control in China (Ma et al. Reference Ma, Wang and Ding2003). In addition, the use of microbial herbicides to control weeds has a long history in China (Li et al. Reference Li, Sun, Zhuang, Xu, Chen and Li2003). The first microbial herbicide in China was ‘Lubao No. 1’, used against Peruvian dodder (Cuscuta australis R. Br.) in the 1960s (Li et al. Reference Li, Sun, Zhuang, Xu, Chen and Li2003). Subsequently, considerable research has been conducted on the development of bioherbicides from microorganisms, including bacterial, fungal, and actinomycete-derived products (Li et al. Reference Li, Sun, Zhuang, Xu, Chen and Li2003). However, these biological agents have not been accepted for mass production because of their action conditions and potentially insignificant economic profit. Our research team produced a novel bioorganic fertilizer composed of kitchen garbage, maize straw, wood-destroying fungi dregs, rice straw, tobacco straw, plant ash, and chicken and sheep manure that not only provides biological control of HR weeds but also supplies nutrients to the crop. Scientific data indicated the fertilizer can effectively control grass (E. crus-galli) and broadleaf weeds (M. vaginalis) in rice fields with an average rate of more than 80% weed suppression, while increasing rice yield by 16.3% to 29.8% relative to controls (Li et al. Reference Li, Li, Zhou, Zhou and Bai2018). Biological management is an active research area pursued by weed scientists but is generally not used as a broad part of resistance management, depending rather on local agricultural environments. Therefore, effective use of biological management is context dependent.
Physical Management
Physical management of HR weeds includes deep plowing in winter, cleaning crop seeds before planting, tillage during the growth process of crops, damaging weed seeds at crop harvest (achieved by chaff carts), hay baling, windrow collection, and cleaning mechanical harvesters using mechanical equipment or manual labor. Physical management is beneficial, increasing output of seedbanks by stimulating weed seed germination, leading to rapid loss of weed seeds from the soil (Buhler Reference Buhler1995; Yenish et al. Reference Yenish, Doll and Buhler1992), and decreasing input to seedbanks by preventing weed seeds from entering the soil. It is documented that physical practices can prevent more than 95% of weed seeds from entering the soil and thus dramatically reduce weed seedling emergence in following crops (Harrington and Powles Reference Harrington and Powles2012; Walsh et al. Reference Walsh, Broster, Schwartz-Lazaro, Norsworthy, Davis, Tidemann, Beckie, Lyon, Soni, Neve and Bagavathiannan2018; Walsh and Powles Reference Walsh and Powles2007).
Generally, physical practices focus on tillage, which buries small weeds and disrupts or severely damages weeds through harrowing, moldboard plowing, or some other technique at the early stage of weed development, resulting in weed mortality (Mohler et al. Reference Mohler, Frisch and Pleasant1997; Rasmussen Reference Rasmussen2004; Van der Weide et al. Reference Van der Weide, Bleeker, Achten, Lotz, Fogelberg and Melander2008). For example, winter plowing to a depth of 15 to 20 cm can expose overwintering underground parts (e.g., tubers) of HR perennial weeds (e.g., S. trifolia) to cold air, freezing them to death or inactivating them. However, as a major mechanical control method, tillage may not be desirable, because it is labor-intensive with high labor costs, in addition to causing soil erosion, especially when plowing is used. Moreover, although hoeing can be effective on younger weeds, it becomes difficult after weeds reach the cotyledon stage (Kurstjens and Perdok Reference Kurstjens and Perdok2000).
Integrated Herbicide-Resistance Management
Current herbicide-resistance management strategies are beneficial for preventing or at least delaying the development of herbicide resistance, but each has its respective limitations. Therefore, the establishment of integrated herbicide-resistance management systems combining chemical, cultural, biological, and physical strategies is a pressing need. In this review, “integrated herbicide-resistance management systems” refers to combinations of more than one strategy of HR weed management (chemical, cultural, biological, or physical) during or surrounding a crop life cycle in a given field. In China, integrated herbicide-resistance management systems may include several different combinations of management practices, representing low to medium levels of risk for the development of herbicide resistance (Table 4). Many integrated herbicide-resistance management systems are helpful to farming systems (Llewellyn et al. Reference Llewellyn, Lindner, Pannell and Powles2007). However, these integrated practices for managing herbicide resistance are not widely adopted by Chinese farmers for several reasons. First, management of HR weeds is still in the preliminary stage in China (Liu et al. Reference Liu, Xiang, Zong, Ma, Wu, Liu, Zhou and Bai2019). Current knowledge of most management systems is still at the nascent stage and is incomplete. Second, chemical management offers significant advantages in the short term. Most integrated herbicide-resistance management systems are based on use of herbicides in combination with agricultural measures, which increases agricultural production costs compared with chemical management only. These economic constraints directly influence farmers’ decisions of whether and when to implement integrated herbicide-resistance management systems. Third, chemical management is more popular for weed control because herbicides are considered to be easier to use, relatively cheaper, more effective, and less labor-intensive. In addition, most Chinese farmers are less aware of the evolution of resistance promoted by continuous and extensive use of herbicides. Many farmers even believe that alternative herbicides with novel MOAs exist for control of HR weeds if and when herbicide resistance occurs. For these reasons, integrated management systems are rarely adopted in China. Therefore, the first step is to modify integrated herbicide-resistance management systems to better fit the diverse local ecological regions of China. Then, area-wide field demonstrations should be conducted to increase farmers’ confidence that integrated herbicide-resistance management systems can be successful. Additionally, integrated management programs should be developed to enhance farmers’ awareness of herbicide resistance, to educate farmers effectively on the purposes of integrated management, and to cover the measures necessary to prevent or delay herbicide-resistance evolution.
Table 4. Risk level associated with the current herbicide-resistance management practices.

a Number of applications of the same MOA herbicides within one cropping season.
b The number of applications per year required for weeds to develop resistance to herbicides with different MOAs varies. For resistance-prone herbicides (e.g., acetolactate synthase/acetyl-CoA carboxylase inhibitors), however, weeds only need two to three applications per year.
c Chemical paired with cultural, biological, and physical practices (Liu et al. Reference Liu, Sun, Fu and Zhong2015c).
Case Study: Integrated Herbicide-Resistance Management in Rice and in Wheat
The heaviest infestation of HR weeds in China is E. crus-galli in rice, followed by G. aparine and D. sophia in wheat (Liu et al. Reference Liu, Xiang, Zong, Ma, Wu, Liu, Zhou and Bai2019). For managing E. crus-galli multi-resistant to penoxsulam, bispyribac-sodium, and quinclorac, an integrated herbicide-resistance management is adopted as follows. Before rice planting, growers make use of high-quality weed-free seeds, clean out weeds on field margins, consider rotation with wheat/canola/cotton, and then optimize planting timing and planting density of rice. After transplanting, a 10- to 20-cm-deep water layer is maintained for a long time, which is beneficial in reducing infestation of annual grass resistant weeds (e.g., E. crus-galli). Next, a three-step herbicide application strategy is employed. Specifically, the first step is to use different MOA PRE herbicides (e.g., pretilachlor) before weed-seed germination. The second step is to apply different MOA POST herbicides (e.g., metamifop) or herbicide mixtures to control E. crus-galli at the 3-leaf stage. Whether the third step should proceed or not depends on the density of E. crus-galli in the field after the previous two treatment steps. If necessary, foliar-applied herbicides will be used to control E. crus-galli at the 4- to 5-leaf stage. After chemical control, the remaining multi-resistant E. crus-galli might start to produce seeds, at which point a physical practice such as pulling weeds by hand will be utilized to prevent the seeds from spreading. Furthermore, seeds will be removed at harvest through the cleaning of mechanical harvesters. Using this integrated system, control efficacy for multi-resistant E. crus-galli is often greater than 85% (Ma et al. Reference Ma, Bai, Liu, Liu, Lu and Yu2012; Zhang et al. Reference Zhang, Ma, Zhou, Tang, YU and Lu2018b).
Similarly, another integrated management system can be established to manage tribenuron-resistant G. aparine and D. sophia, which are prevalent in wheat. Such a system could involve replacement of herbicides at high resistance risk with different MOA herbicides, mixtures with no cross-resistant herbicides, rotation with highly effective herbicides, and ancillary measures involving cultural practices. This means that different effective commercial herbicide products, including fluroxypyr, pyraflufen-ethyl, florasulam, carfentrazone-ethyl, and mixtures of florasulam with fluroxypy/carfentrazone-ethyl/clodinafop-propargyl, which do not share cross-resistance with tribenuron, are applied to control tribenuron-resistant G. aparine and D. sophia. These chemical practices, combined with rice–wheat crop rotation and 30- to 35-cm-deep tillage before wheat planting, can effectively control tribenuron-resistant weeds.
Summary and Perspectives
Herbicides alone cannot create resistant weeds. Resistance to herbicides is an outcome of natural selection in the presence of selection pressure exerted by herbicides. Resistance is bound to happen if an herbicide is in continuous use without proper levels of risk assessment in place, as shown in Table 4. In China, ALS inhibitors, ACCase inhibitors, synthetic auxin herbicides, EPSPS inhibitors, PPO inhibitors, PSI electron diverters, PSII inhibitors, and LCFA inhibitors are the eight herbicide groups most prone to developing resistance. Based on the underlying resistance mechanisms (reviewed here), China has established diverse strategies, including chemical, cultural, biological, physical, and most recently, integrated tactics to manage HR weeds. To develop more effective integrated management systems against herbicide resistance, future work should focus on (1) understanding the biology and ecology of crop–weed interactions; (2) elucidating herbicide-resistance mechanisms, especially for the grossly understudied NTSR; (3) strengthening research on preventive measures; and (4) developing new resistance management strategies, specifically for non-chemical approaches, through continued and collaborative efforts among scientists, farmers, manufacturers, and policy makers both in China and worldwide.
Acknowledgments
The authors are grateful to the anonymous reviewers for their constructive comments and suggestions. This work was supported by a grant from the National Natural Science Foundation of China (award no. 31672043), the Natural Science Funds of Hunan Province (award no. 2018JJ2165), a China Postdoctoral Science Foundation–funded project (award no. 2015M582329), and the China Scholarship Council (file no. 201608430210). The authors have declared that no conflict of interest exists.
Author Contributions
XZ and LYB designed the contents of the text; XYL and XZ analyzed the data; XYL drafted the article; XZ and AM revised the article; SHX and TZ assisted with the literature search. All authors read and approved the final version of the article.