Introduction
A volunteer has been defined as a person who offers service or undertakes a task without being paid (Simpson and Weiner Reference Simpson and Weiner1989). Similarly, a crop volunteer is a plant that grows on its own rather than being planted intentionally. Crop plants grow voluntarily in a rotation crop, often from seeds lost preharvest (seed shattering) or during harvest of a previous crop or through seed dispersal by wind, water, birds, or contaminated compost (Chahal and Jhala Reference Chahal and Jhala2015). Like weeds, crop volunteers compete with crops for nutrients, moisture, space, and light (Beckie and Owen Reference Beckie and Owen2007) and pose the same problems as weeds, such as reducing crop quality and yields (Kniss et al. Reference Kniss, Sbatella and Wilson2012), interfering with crop harvest (Chahal and Jhala Reference Chahal and Jhala2016b), serving as a host for insects/diseases (DPIRD 2016), and depending on the species, having an allelopathic effect (Walsh et al. Reference Walsh, Sanderson, Hall, Mugo and Hills2014). Volunteerism is a well-known phenomenon in agronomic crops, and in certain cases, presents a challenge for growers to find a selective herbicide for their management (Chahal and Jhala Reference Chahal and Jhala2015). Herbicides commonly used for controlling weeds in rotational crops may not completely control herbicide-resistant (HR) crop volunteers, particularly in monoculture such as corn (Zea mays L.) after corn (Striegel et al. Reference Striegel, Lawrence, Knezevic, Hein and Jhala2020) or rice (Oryza sativa L.) after rice (Sanders et al. Reference Sanders, Bond, Lawrence, Golden, Allen and Bararpour2020). Since the discovery of 2,4-D for broadleaf weed control in the early 1940s (Peterson et al. Reference Peterson, McMaster, Riechers, Skelton and Stahlman2016), several herbicides with distinct sites of action (SOAs) have been discovered and applied in a number of crops (Rüegg et al. Reference Rüegg, Quadranti and Zoschke2007); however, in recent years, the cost of discovery of a herbicide with a novel SOA that meets regulatory standards has increased significantly (Hall et al. Reference Hall, Mackie, Gendall, Perugini and da Costa2020). A successful alternative strategy for selective weed control in major agronomic crops has been to use genetic engineering or traditional breeding methods to develop crop traits resistant to existing herbicides (Duke Reference Duke2005). HR crops, such as bromoxynil-resistant cotton (Gossypium hirsutum L.) and triazine-resistant (TR) canola (Brassica napus L.) were introduced in the 1980s; however, poor agronomic performance and yield limited their adoption (Duke Reference Duke2015). In 1996, 1997, and 1998, glyphosate-resistant (GR) soybean [Glycine max (L.) Merr.], cotton, and corn, respectively, were commercially released and adopted at an unprecedented rate because of little or no yield penalty and economical weed management (Kniss Reference Kniss2018). Since then, GR crops have been grown on most of the alfalfa (Medicago sativa L.), canola, corn, cotton, soybean, and sugarbeet (Beta vulgaris L.) acreage in the United States and corn and soybean acreage in Canada (Beckie Reference Beckie2011).
The development and commercial cultivation of HR crops have revolutionized weed control options in agronomic crops (Duke Reference Duke2005). Widespread adoption of GR crops, particularly in developed countries, has reduced production costs, increased adoption of no-tillage practice, and simplified weed management (Duke Reference Duke2015); however, overreliance on glyphosate for several years resulted in 50 weeds resistant to glyphosate globally, including 17 species in the United States (Heap Reference Heap2020). In addition, GR crop volunteers are considered to be GR problem weeds in specific cropping systems, such as GR corn volunteers in the midwestern United States (Chahal and Jhala Reference Chahal and Jhala2016b) and GR canola volunteers in western Canada (Beckie and Owen Reference Beckie and Owen2007). Kniss (Reference Kniss2018) conducted an analysis to identify trends in herbicide use and evolution of HR weeds in the United States and reported that although the number of GR weeds increased, the evolution of new GR weeds as a function of the area sprayed with glyphosate has remained relatively low compared with commonly used herbicides such as acetolactate synthase (ALS) inhibitors.
Multiple HR crops have been developed and commercialized in recent years, including glyphosate/dicamba- or 2,4-D–resistant soybean and cotton, glyphosate/glufosinate-resistant corn, and 2,4-D/glufosinate/glyphosate-resistant corn primarily for control of GR weeds (Beckie et al. Reference Beckie, Ashworth and Flower2019). Shyam et al. (Reference Shyam, Chahal, Jhala and Jugulam2021) reported herbicide programs for effective control of GR Palmer amaranth (Amaranthus palmeri S. Watson) in 2,4-D/glufosinate/glyphosate-resistant soybean. A few other studies reported greater control of GR weeds in multiple HR soybean (Byker et al. Reference Byker, Soltani, Robinson, Tardif, Lawton and Sikkema2013; Vink et al. Reference Vink, Soltani, Robinson, Tardif, Lawton and Sikkema2012; Yadav et al. Reference Yadav, Kumar and Jha2020). While multiple HR crops have provided effective control of GR weeds, management of multiple HR crop volunteers is complicated for growers. For example, glufosinate/glyphosate-resistant corn cannot be controlled with selective herbicides in corn (Striegel et al. Reference Striegel, Lawrence, Knezevic, Hein and Jhala2020). Imidazolinone-resistant (IR) barley (Hordeum vulgare L.), wheat (Triticum aestivum L.), or rice volunteers need alternate herbicides for their control in rotational crops (Burgos et al. Reference Burgos, Norsworthy, Scott and Smith2008). In contrast, multiple HR crops have provided an opportunity to use a certain herbicide for control of crop volunteers. For instance, corn resistant to aryloxyphenoxypropionates (FOPs) provides an opportunity for selective control of glyphosate/glufosinate-resistant corn volunteers with quizalofop (Striegel et al. Reference Striegel, Lawrence, Knezevic, Hein and Jhala2020).
The scientific literature is limited on risk assessment of HR crop volunteers and their interference with and management in rotational crops. Therefore, the objectives of this review were (1) to summarize HR crop traits developed and available commercially in barley, canola, corn, cotton, rice, soybean, sugarbeet, and wheat, and their potential for volunteerism; and (2) to review existing literature on the interference of HR crop volunteers, yield loss, and their management in rotational crops.
Barley
Barley is a member of the Poaceae family and native to the Middle East (the Fertile Crescent) with Hordeum vulgare ssp. spontaneum (K. Koch) Asch. & Graebn. as the progenitor species (Australian Government 2020). The domesticated landraces, over the past 100 yr, have been displaced by pure line cultivars with reduced genetic diversity. The main factors used to distinguish barley cultivars are feed or malt quality; winter or spring growth habit; starch amylose/amylopectin ratio; hulled or hull-less; and six, four, or two rows (Australian Government 2020). Like wheat, barley is a highly self-pollinated crop. Barley is widely grown in both temperate and Mediterranean climates around the world. Because HR cultivars of the crop were only developed and commercially introduced in Australia, the following sections will be primarily restricted to HR trait development, grower adoption, interference of volunteers in rotational crops, and management of volunteers in barley production areas within that country. In 2020, barley was grown on 4.4 million ha across Australia (ABARES 2020).
Herbicide-Resistant Traits in Barley
The only HR trait developed in barley is imidazolinone resistance in Australia (Moody et al. Reference Moody, Materne, Pittock, Crane and Liesegang2016). The first malt barley cultivar, ‘Scope’ was introduced in 2011, with imazamox/imazapyr registered for POST control of grass and broadleaf weeds (Australian Government 2020). Imazamox/imazapyr improved in-crop control of some key weeds such as brome grass (Bromus spp.). In addition, IR barley could be safely grown in fields with imidazolinone herbicide residues in soil potentially phytotoxic to non-IR barley (Moody et al. Reference Moody, Materne, Pittock, Crane and Liesegang2016). This trait also provides growers with the opportunity to achieve higher yields and improve grain quality through earlier sowing into wheat stubble; volunteer wheat can be controlled in-crop rather than waiting for seedlings to emerge before control by glyphosate or paraquat. Adoption of cultivars with this trait has been rapid, occupying about 50% of the area sown to barley in Western Australia (DPIRD 2020).
Interference of Herbicide-Resistant Barley Volunteers
Barley is one of the most weed-competitive crops grown globally. Therefore, interference of barley volunteers can be significant in rotational crops (O’Donovan et al. Reference O’Donovan, Harker, Clayton, Hall, Cathcart, Sapsford, Holm and Hacault2007; Shinn et al. Reference Shinn, Thill and Price1999). In studies conducted across western Canada, increasing density of barley volunteers up to 120 plants m−2 reduced spring wheat grain yield by 50% or more (O’Donovan et al. Reference O’Donovan, Harker, Clayton, Hall, Cathcart, Sapsford, Holm and Hacault2007). In a study in the Pacific Northwest region of the United States, barley volunteers at a density of 65 to 69 plants m−2 reduced winter wheat grain yield up to 10% (Shinn et al. Reference Shinn, Thill and Price1999). Barley volunteers can act as a green bridge for pathogens such as barley leaf rust (Puccinia hordei Otth) (DPIRD 2016). Furthermore, barley volunteers admixed with wheat seeds at harvest can decrease the grade and price.
Management of Herbicide-Resistant Barley Volunteers
Crop rotation planning is important for managing IR barley volunteers, particularly when IR crops such as wheat or canola are grown. Like HR wheat volunteers, HR barley volunteers are less easily controlled in rotational cereal than in broadleaf crops because of fewer herbicide options (Moody et al. Reference Moody, Materne, Pittock, Crane and Liesegang2016). There are several herbicide options to control HR or non-HR barley volunteers before seeding wheat or broadleaf crops. In canola or pulses, acetyl-CoA carboxylase (ACCase) inhibitors, including, clethodim, sethoxydim, and quizalofop, are used to control HR or non-HR barley volunteers (Farmanco 2020).
Canola
Canola is a member of the Brassicaceae family; Brassica rapa L. and Brassica oleracea L. are progenitor species, which are native to Eurasia (reviewed in Gulden et al. Reference Gulden, Warwick and Thomas2008). These cultivars were named “canola” in the Americas or “oilseed rape” in Europe. Canola is commonly grown in the Northern Great Plains of North America, ranking second after wheat in cultivated area and economic importance in western Canada (8.4 million ha; Statistics Canada 2020). The most common crop rotation in the region is wheat–canola. In the United States, canola is grown on 0.8 million ha annually, with 85% of production in North Dakota (USDA-NASS 2020). Canola has an indeterminate growth habit, with pods that shatter easily at maturity.
Herbicide-Resistant Traits in Canola
The first HR trait was conventionally bred into canola from TR weedy B. rapa and introduced in 1981 in Canada (Beversdorf and Kott Reference Beversdorf and Kott1987); however, the trait conferred a 20% to 30% fitness (yield) penalty due to reduced photosynthetic efficiency. In addition, atrazine lacked broad-spectrum weed control, including for cruciferous weeds such as wild mustard (Sinapis arvensis L.). The introduction in 1990 of the POST selective canola herbicide ethametsulfuron reduced the utility of TR canola. These cultivars peaked at 4% of crop area in 1988 and decreased to less than 1% by 1996 (Downey Reference Downey1999). However, TR cultivars (open-pollinated or hybrids) dominate the crop area in Australia (DPIRD 2019). Transgenic bromoxynil-resistant cultivars in Canada occupied less than 1% of crop area in 2000 and 2001 due to the narrow weed spectrum controlled by the herbicide (Beckie Reference Beckie2006). GR (Roundup Ready®) or glufosinate- (LibertyLink®) resistant canola were introduced in the mid-1990s and now account for 95% of the crop area, with similar market share for each trait in Canada (Beckie Reference Beckie2016). Non-transgenic (mutagenesis-derived) Clearfield® cultivars, resistant to imidazolinone herbicides (ALS inhibitors), comprise the remaining crop area. HR canola facilitated conservation tillage because soil incorporation of PRE herbicides was no longer required and led to greater yields due to better genetics and water-use efficiency. In contrast to other GR crops in the Americas, the trait in canola did not result in greater selection of HR weeds. The main reasons are crop rotation interval (canola grown every 2 to 4 yr) and moderate adoption rate of this trait (Beckie Reference Beckie2016). Many growers typically plant canola with different HR traits on their farm each year. The overall impact is low to moderate selection pressure for glyphosate in dominant weed species in the region (Beckie and Owen Reference Beckie and Owen2007). The stacked HR trait cultivars (triazine + glyphosate) are only grown in Australia (DPIRD 2019).
Interference of Herbicide-Resistant Canola Volunteers
Canola volunteers are abundant in western Canada, where canola is widely grown. The relative abundance ranking of volunteer canola averaged 5th place in preharvest weed surveys conducted in the prairie provinces of Alberta, Saskatchewan, and Manitoba from 2010 to 2016, significantly higher than previous surveys (e.g., Leeson et al. Reference Leeson, Gaultier and Grenkow2017). This increased ranking reflects the yearly trends in canola area and its frequency in annual crop rotations, as well as prevailing herbicide-use and management practices. Weediness of canola volunteers is aided by high fecundity, seed loss before or during harvest, and secondary seed dormancy (Mierau et al. Reference Mierau, Johnson, Gulden, Weber, May and Willenborg2019). Although pod-shattering tolerance is being bred into commercial cultivars, potential seed loss before and during harvest is still substantial (Gulden et al. Reference Gulden, Shirtliffe and Thomas2003). Canola seedbank additions through harvest losses range from 2,500 to greater than 8,000 seeds m−2 (4% to 10% of seed yield) in western Canada (Cavalieri et al. Reference Cavalieri, Harker, Hall, Willenborg, Haile, Shirtliffe and Gulden2016; Gulden et al. Reference Gulden, Shirtliffe and Thomas2003; Haile et al. Reference Haile, Gulden and Shirtliffe2014). While canola has almost no primary seed dormancy, it possesses secondary dormancy that is influenced by environmental conditions, burial, and genotype (Gulden et al. Reference Gulden, Thomas and Shirtliffe2004). Most volunteers emerge in the first year following the canola crop, although seeds may persist, and seedlings emerge at low densities throughout a 3- or 4-yr rotation (Harker et al. Reference Harker, Clayton, Blackshaw, O’Donovan, Johnson, Gan, Holm, Sapsford, Irvine and Van Acker2006). In a study in western Canada, seedbank persistence was 44%, 14%, and 0.2% of the original seedbank over the first 3 yr, respectively, following canola (Gulden et al. Reference Gulden, Shirtliffe and Thomas2003). Seedbanks are depleted by predation, fatal germination, expiration, or pathogens. Volunteer canola can emerge early in the growing season and can strongly interfere with less-competitive broadleaf crops grown in rotation, such as field pea (Pisum sativum L.), sugarbeet (Stachler and Luecke Reference Stachler and Luecke2009), or soybean (Beckie and Owen Reference Beckie and Owen2007; Gulden et al. Reference Gulden, Warwick and Thomas2008). Rotational crop yield loss potential is high from IR canola volunteers in field pea because of reliance on ALS inhibitors for weed control and from GR canola volunteers in sugarbeet and soybean because of dominance of the GR trait. For example, GR canola volunteers at 180 plants m−2 caused near complete loss of sugarbeet plant population and root or sucrose yield (Stachler and Luecke Reference Stachler and Luecke2009).
Management of Herbicide-Resistant Canola Volunteers
Management of HR canola volunteers is most challenging in broadleaf crops. For example, limited herbicide options to control HR canola volunteers in pulse crops, sugarbeet, and soybean (Stachler and Luecke Reference Stachler and Luecke2009) increases the necessity of optimizing crop competitiveness to mitigate yield loss (Geddes and Gulden Reference Geddes and Gulden2018; Mierau et al. Reference Mierau, Johnson, Gulden, Weber, May and Willenborg2019). Pollen-mediated gene flow from adjacent GR canola fields or admixtures in planted seed lots can result in trait stacking in volunteers, even if the cultivar grown in a field is not GR (Beckie et al. Reference Beckie, Warwick, Nair and Séguin-Swartz2003; Friesen et al. Reference Friesen, Nelson and Van Acker2003; Hall et al. Reference Hall, Topinka, Huffman, Davis and Good2000; Figure 1), which the grower may not anticipate. Uncontrolled volunteers contribute to seedbank persistence. In a study in western Canada, transgenic canola volunteers persisted for 7 yr in an arable field around wet depressional areas that did not permit crop cultivation or herbicide application (Beckie and Warwick Reference Beckie and Warwick2010). Tidemann et al. (Reference Tidemann, Hall, Harker, Beckie, Johnson and Stevenson2017) conducted a study in western Canada to determine whether retention and height criteria were met to determine if harvest weed seed control is an effective non-herbicidal management tool for HR canola volunteers. Volunteer canola seed had greater than 95% retention, with the majority of seed retained >15 cm above ground level, indicating the suitability of this method. The potential persistence or invasiveness of volunteer or feral canola in non-crop disturbed (ruderal) areas is transient or low probability, as analyzed by population demography (Alexander Reference Alexander2016; Crawley et al. Reference Crawley, Halls, Rees, Kohn and Buxton1993; Knispel and McLachlan Reference Knispel and McLachlan2010). Seed dispersal from adjacent fields or spillage via grain transportation can result in abundant road- or railside populations (Busi and Powles Reference Busi and Powles2016; Knispel and McLachlan Reference Knispel and McLachlan2010; Yoshimura et al. Reference Yoshimura, Beckie and Matsuo2006). These studies have concluded that populations can persist in ruderal areas with periodic seed replenishment or application of glyphosate for nonselective weed control but are otherwise ephemeral if seed immigration is prevented. Nevertheless, future canola cultivars with abiotic stress tolerance (e.g., drought, salinity) have potential for greater weediness, persistence, and invasiveness in ruderal areas (Beckie et al. Reference Beckie, Hall, Simard, Leeson and Willenborg2010).

Figure 1. Glyphosate- and glufosinate-resistant canola volunteers in adjacent fields in Saskatchewan, Canada, due to bidirectional pollen-mediated gene flow the previous year.
Corn
Corn is an annual monoecious crop belonging to the Poaceae family. It is an open-pollinated species with a C4 pathway of photosynthesis; therefore, it uses water and carbon dioxide efficiently (Kiesselbach Reference Kiesselbach1999). Harshberger (Reference Harshberger1896) reported that corn originated in Mexico and had once been a wild plant in central Mexico, based on climatic data where corn is the most productive. Different types of corn are grown, including field corn, sweet corn, white corn, popcorn, and ornamental corn. Corn is primarily used for human consumption, livestock feed, fuel, and industrial applications (Farnham et al. Reference Farnham, Benson and Pearce2003). The United States is the largest producer of corn in the world, with the production of 364 billion kg in 2018 followed by China, Brazil, Argentina, Ukraine, India, and Mexico (USDA-FAS 2020). The area of corn planted in the United States has increased from 29.7 million ha in 1990 to 37.2 million ha in 2019 (USDA-NASS 2020).
Herbicide-Resistant Traits in Corn
Season-long weed management in corn is required to achieve optimum yield (Jhala et al. Reference Jhala, Knezevic, Ganie and Singh2014). HR corn hybrids have been developed to expand weed management options. Imidazolinone- and sethoxydim-resistant corn hybrids were introduced in 1993 and 1996, respectively, in the United States; however, they were not widely adopted (Beckie and Owen Reference Beckie and Owen2007). Similarly, GR and glufosinate-resistant corn were commercialized in 1997 and 1998, but they were not rapidly adopted by growers (Dill Reference Dill2005). The widescale adoption of GR corn occurred with the insertion of the Bacillus thuringiensis (Bt) trait to manage insects along with weed management in the same hybrid (Marquardt and Johnson Reference Marquardt and Johnson2013), thus providing multiple benefits to the farmer for pest management. Adoption of glyphosate/Bt hybrids has accelerated in the last few years. In 2014, 76% of the corn planted in the United States was stacked-resistant hybrids, and that increased to 89% in 2019 (USDA-ERS 2020a). As in the United States, GR corn was first grown in Canada in 1998 (Beckie et al. Reference Beckie, Harker, Hall, Warwick, Légère, Sikkema, Clayton, Thomas, Leeson, Séguin-Swartz and Simard2006). A corn trait resistant to 2,4-D/glufosinate/glyphosate/FOPs (Enlist E3®) has been commercially available since the 2018 growing season in the United States (Striegel et al. Reference Striegel, Lawrence, Knezevic, Hein and Jhala2020). A new corn trait resistant to five herbicides—2,4-D/dicamba/glufosinate/glyphosate/quizalofop (MON 87429 event)—has been developed, and a petition has been submitted to the U.S. Department of Agriculture−Animal and Plant Health Inspection Service for nonregulated status in 2020 (USDA-APHIS 2020).
Interference of Herbicide-Resistant Corn Volunteers
Volunteer corn results from the seeds or ears of hybrid corn lost during harvest the previous year (Chahal and Jhala Reference Chahal and Jhala2015; Figure 2) or from a failed corn stand during a corn replant situation (Steckel et al. Reference Steckel, Thompson and Hayes2009). Many other factors, including storm damage or poor stalk quality, can lead to kernel and ear losses, resulting in corn volunteers (Jhala and Rees Reference Jhala and Rees2018; Figure 3). Most volunteer corn seeds in northern latitudes overwinter and germinate the following spring, while in warmer climates, seeds germinate soon after corn harvest (Owen Reference Owen2005). Chahal and Jhala (Reference Chahal and Jhala2016a) compared factors affecting germination and emergence of GR hybrid corn and volunteers. The GR corn volunteers showed similar germination and emergence response as hybrid corn to the day/night temperature, duration of light, osmotic stress, and salt concentration.

Figure 2. Soybean after corn is a typical rotation in the midwestern United States. If not controlled, volunteer corn is a problem weed in soybean fields.
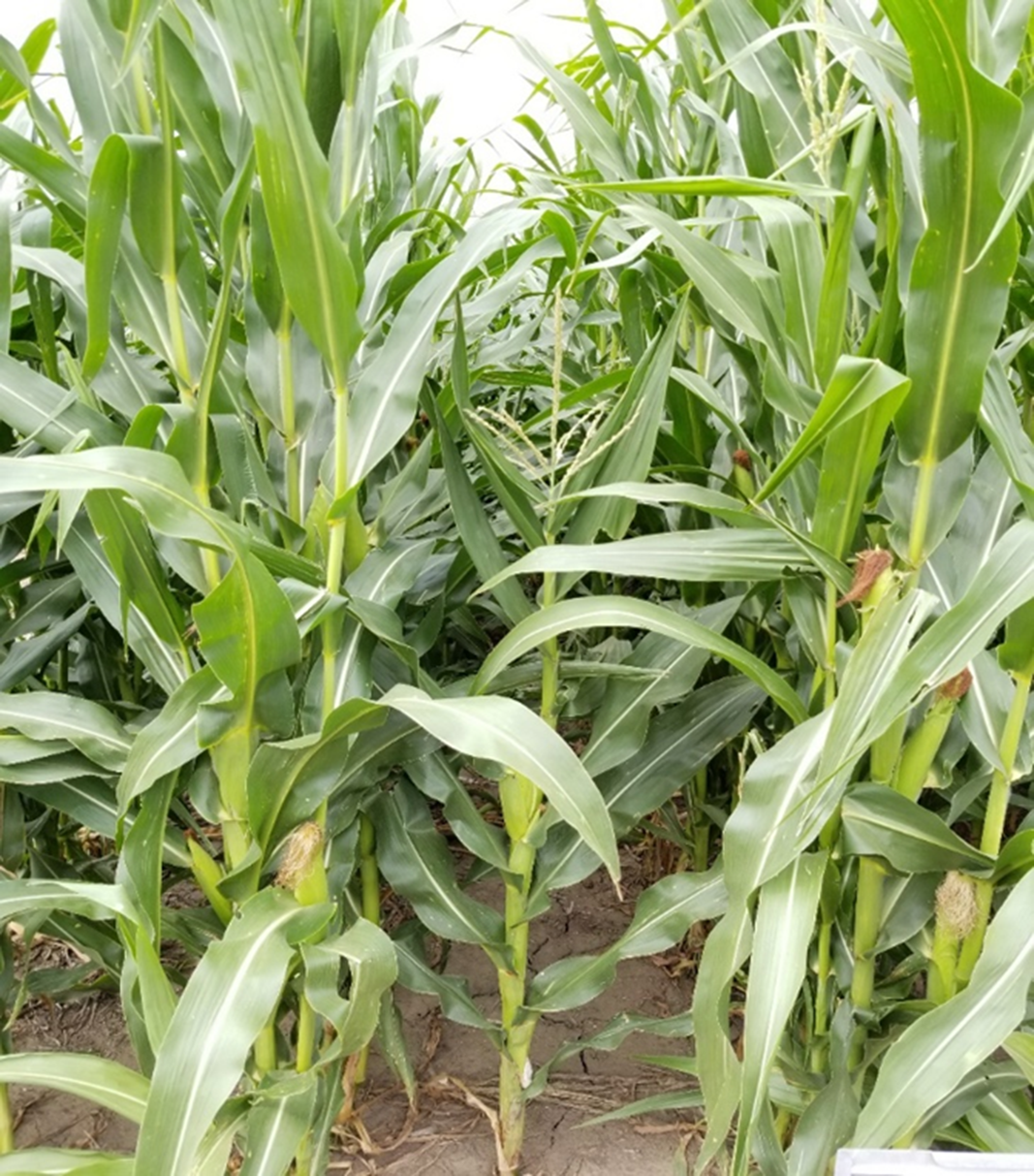
Figure 3. Volunteer corn in a cornfield in Nebraska. Highly productive soils and easy access to irrigation have encouraged growers to adopt a corn-on-corn cropping system in south-central Nebraska that results in corn volunteers.
Several studies have reported yield loss in crops grown in rotation with corn through direct competition with volunteer corn (Rees and Jhala Reference Rees and Jhala2018). Corn is grown in rotation with several crops, including cotton, sugarbeet, and soybean, with yield loss reported in the range of 4% to 83% depending on crop and density of volunteer corn (Chahal et al. Reference Chahal, Jha, Jackson-Ziems, Wright and Jhala2016; Rees and Jhala Reference Rees and Jhala2018). A monoculture of corn is a common practice among several growers in southcentral Nebraska that results in volunteer corn issue (Striegel et al. Reference Striegel, Lawrence, Knezevic, Hein and Jhala2020). Jeschke and Doerge (Reference Jeschke and Doerge2008) reported 1.5% to 13% corn grain yield losses with volunteer corn density of 0.5 to 4 plants m−2. Cotton lint yield loss in the range of 4% to 8% has been reported with a 0.5-kg increase in volunteer corn biomass per meter of crop row (Clewis et al. Reference Clewis, Thomas, Everman and Wilcut2008). Kniss et al. (Reference Kniss, Sbatella and Wilson2012) reported 19% sucrose yield loss with volunteer corn density of 1 to 1.7 plants m−2 in sugarbeet. Wilson et al. (Reference Wilson, Sandell, Klein and Bernards2010) reported 10% and 27% soybean yield loss with volunteer corn density of 8,750 and 17,500 plants ha−1, respectively.
Clumps (lost ear of corn producing several corn plants) of volunteer corn plants emerging and interfering in rotational crops cause more yield loss compared with individual plants (Chahal and Jhala Reference Chahal and Jhala2016a). Andersen et al. (Reference Andersen, Ford and Lueschen1982) reported 31% to 83% soybean yield reduction as volunteer corn clump density increased from 1 to 4 clumps spaced among every 2.4 m of soybean row. Corn volunteers can further affect crop yield by indirect interference. Under corn–fallow–winter wheat rotation in the west central Great Plains, the presence of corn volunteers in the fallow reduced available soil water by 2.5 cm for every 2,500 volunteer corn plants ha−1 and resulted in reduced wheat yield (Holman et al. Reference Holman, Schlegel, Olson and Maxwell2011).
Management of Herbicide-Resistant Corn Volunteers
Best management practices to prevent volunteer corn from becoming a problem weed include selection of hybrids with good stalk quality and proper combine settings to minimize harvest loss (Jeschke and Doerge Reference Jeschke and Doerge2008). Agronomic practices such as early fall tillage can stimulate the germination and emergence of volunteer corn, thus exposing the emerged seedlings to winter freeze (Jeschke and Doerge Reference Jeschke and Doerge2008); however, with the widespread adoption of no-tillage or minimum tillage practices, fall tillage is not a common practice in the midwestern United States (Farmer et al. Reference Farmer, Bradley, Young, Steckel, Johnson, Norsworthy, Davis and Loux2017). In a corn replant situation, control of GR corn was greater than 90% with paraquat (70 g ai ha−1) in a mixture with a photosystem II inhibitor such as atrazine, diuron, metribuzin, or linuron before replant (Steckel et al. Reference Steckel, Thompson and Hayes2009).
Soil-applied PRE herbicides labeled in soybean only suppress (<80% efficacy) corn volunteers (Beckett and Stoller Reference Beckett and Stoller1988; Chahal et al. Reference Chahal, Kruger, Blanco-Canqui and Jhala2014). Therefore, complete control of corn volunteers before emergence is not achievable in soybean due to the lack of a selective residual herbicide. A cost-effective option for controlling GR corn volunteers in GR soybean is POST application of ACCase-inhibiting herbicides such as clethodim, sethoxydim, and quizalofop applied alone or in a mixture with fluazifop or fluazifop + fenoxaprop (Beckett and Stoller Reference Beckett and Stoller1988; Beckett et al. Reference Beckett, Stoller and Bode1992; Chahal et al. Reference Chahal, Kruger, Blanco-Canqui and Jhala2014; Deen et al. Reference Deen, Hamill, Shropshire, Soltani and Sikkema2006; Marquardt and Johnson Reference Marquardt and Johnson2013; Young and Hart Reference Young and Hart1997). Glufosinate applied in a single or sequential application provided 85% to 97% control of GR corn volunteers in glufosinate-resistant soybean (Chahal and Jhala Reference Chahal and Jhala2015; Figure 4A); however, if volunteer corn is resistant to glufosinate, an ACCase-inhibiting herbicide will need to be applied (Chahal and Jhala Reference Chahal and Jhala2015; Figure 4B).
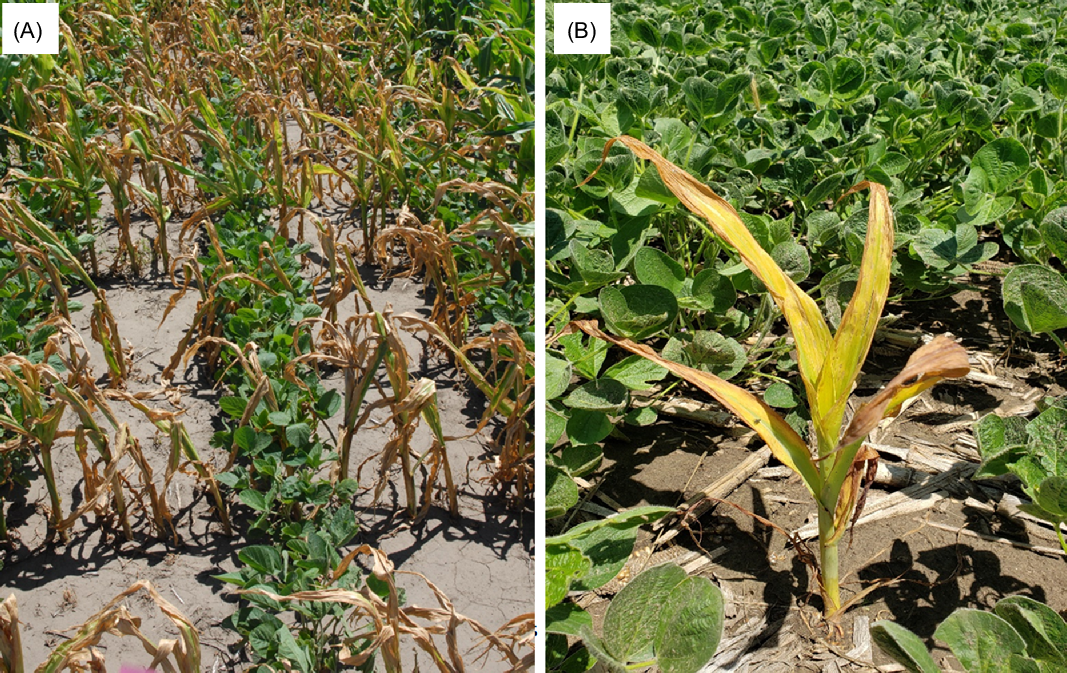
Figure 4. Symptoms of (A) glufosinate on glyphosate-resistant volunteer corn in glufosinate-resistant soybean and (B) sethoxydim on glyphosate/glufosinate-resistant volunteer corn in dicamba/glyphosate-resistant soybean.
Management of volunteer corn in continuous corn production systems is difficult, as most of the corn grown in the United States and Canada is HR and there is no selective herbicide that can provide effective control (Figure 3). In certain scenarios, glufosinate can be applied if the corn hybrid planted the previous year was GR and the corn hybrid planted in rotation is glufosinate resistant; however, a corn trait conferring resistance to glyphosate/glufosinate is commercially available; thus, control of volunteers with this stacked trait is a challenge. In a 2-yr study in Nebraska, Striegel et al. (Reference Striegel, Lawrence, Knezevic, Hein and Jhala2020) reported that quizalofop can be applied for selective control of glyphosate/glufosinate-resistant volunteer corn in corn resistant to FOPs. Multiple HR soybean traits have been developed that enable use of phenoxy herbicides such as 2,4-D or dicamba in a mixture with glyphosate (Byker et al. Reference Byker, Soltani, Robinson, Tardif, Lawton and Sikkema2013; Shyam et al. Reference Shyam, Chahal, Jhala and Jugulam2021). Growers prefer to mix 2,4-D or dicamba with ACCase inhibitors, assuming this mixture will provide broad-spectrum weed control; however, it can antagonize grass weed control (Blackshaw et al. Reference Blackshaw, Harker, Clayton and O’Donovan2006; Underwood et al. Reference Underwood, Soltani, Hooker, Robinson, Vink, Swanton and Sikkema2016). Recent greenhouse and field studies in Illinois reported that clethodim should be applied at ≥105 g ha−1 and should include crop oil concentrate and/or an adjuvant-inclusive clethodim product to avoid the antagonistic effect of mixing clethodim with 2,4-D or dicamba for controlling GR corn volunteers (Harre et al. Reference Harre, Young and Young2020).
Cotton
Cotton, a perennial often referred to as a shrub, is a self-pollinated plant with a C3 pathway of photosynthesis and an indeterminate growth habit (Mauney Reference Mauney1986; Sage and Zhu Reference Sage and Zhu2011). Mauney (Reference Mauney1986) suggested cotton had perhaps the most complex plant structure of any major field crop with sympodial fruiting branches developing a four-dimensional occupation of space and time. The United States is the third-largest producer of cotton in the world, after China and India (USDA-FAS 2020), with 16 southern states producing nearly 4.04 million ha in 2018 (USDA-NASS 2020).
Herbicide-Resistant Traits in Cotton
Season-long weed management is challenging in cotton compared with crops such as corn or soybean, because it has a slow growth habit and is typically produced in wide rows (Wilcut et al. Reference Wilcut, York and Monks1995). In addition, weeds interfere with cotton harvesting and can have a greater adverse impact on quality of the harvested product (Morgan et al. Reference Morgan, Fromme, Baumann, Grichar, Bean, Matocha and Mott2012; Smith et al. Reference Smith, Baker and Steele2000). Hence, effective weed control is an essential component of a cotton production system. Cotton traits resistant to topical applications of bromoxynil were first commercialized in 1995, and they offered control of many troublesome weeds (Culpepper and York Reference Culpepper and York1997; Jordan et al. Reference Jordan, Smith, McClellan and Frans1993). Although bromoxynil was effective, adoption of this trait was short-lived, mostly due to undesirable agronomic performance (Culpepper and York Reference Culpepper and York1997).
The first GR cotton was commercialized in 1997, but glyphosate applications could only be made beyond the 4-leaf stage of growth; later applications had to be directed to the base of the plant to avoid fruit abortion (Pline et al. Reference Pline, Edmisten, Wilcut, Wells and Thomas2003). Although resistance to glyphosate was not complete, adoption of this technology from 1997 through 2006 was greater than 80% (Beckie and Owen Reference Beckie and Owen2007). It was not until 2006 that a new generation of GR cotton was grown— expressing the resistance trait effectively in plant reproductive tissue and thus allowing higher rates of glyphosate and late-season applications (Wallace et al. Reference Wallace, Sosnoskie, Culpepper, York, Edmisten, Patterson, Jones, Crooks, Cloud and Pierson2011).
A cotton trait resistant to glufosinate was launched in 2004 with minimal adoption, as it lacked resistance to glyphosate and agronomic performance in some cotton-producing regions in the United States. Once GR A. palmeri began to dominate the Cotton Belt landscape, growers started looking for tools in addition to glyphosate for weed management—glufosinate was an ideal choice (Sosnoskie and Culpepper Reference Sosnoskie and Culpepper2014). Interestingly, the first cotton cultivars treated by farmers with both glufosinate and glyphosate were not specifically designed to be treated with glufosinate. WideStrike® cotton was developed to be resistant to lepidopteran pests (Culpepper et al. Reference Culpepper, York, Roberts and Whitaker2008; Wallace et al. Reference Wallace, Sosnoskie, Culpepper, York, Edmisten, Patterson, Jones, Crooks, Cloud and Pierson2011) by expressing two Bt insecticidal proteins, Cry1Ac and Cry1F. The molecular constructs of each of these insecticidal transgenes include a single copy of the phosphinothricin acetyltransferase (pat) gene, which confers glufosinate resistance. But in this case, the pat genes were not optimized to confer complete resistance, but rather were used as a selectable marker to aid in trait introgression. As such, glufosinate was not commercially promoted for these cultivars, as they lacked the level of resistance expected in a commercially available cultivar (Barnett et al Reference Barnett, Mueller and Steckel2013; Culpepper et al. Reference Culpepper, York, Roberts and Whitaker2008). However, once farmers became aware of this unique management opportunity in cultivars with favorable agronomic qualities, they rapidly adopted glyphosate plus glufosinate–based programs (Sosnoskie and Culpepper Reference Sosnoskie and Culpepper2014). The first glyphosate- and glufosinate- (bar gene) resistant cultivars were commercialized in 2011/2012, providing effective weed control using both herbicides without crop injury concerns (Wallace et al. Reference Wallace, Sosnoskie, Culpepper, York, Edmisten, Patterson, Jones, Crooks, Cloud and Pierson2011).
The 2017 season was the first opportunity for growers to plant triple-stacked cotton with fully registered herbicide options of glyphosate, glufosinate, and either dicamba or 2,4-D. By 2019, nearly 55% of the cotton planted in the United States was XtendFlex® cultivars, which are resistant to glyphosate/glufosinate/dicamba (USDA-AMS 2019). Multiple HR cotton traits, including auxin herbicides, have been widely planted, because they provide flexibility in weed control and an option for GR A. palmeri management (Barnett et al. Reference Barnett, Mueller and Steckel2013; Leon et al. Reference Leon, Ferrell and Sellers2016).
Interference of Herbicide-Resistant Cotton Volunteers
HR cotton volunteers can become a concern in several ways: when cotton seeds are lost from the previous harvest for a multitude of reasons or when faced with a replant situation during the same season. In addition, unique to cotton, is when the plant survives the winter and becomes “ratooned” cotton—having regrowth from the stalk (Figure 5). The latter situation is much more challenging. Although it is possible for cotton volunteers at high populations to reduce yield of a rotational crop, it would be rare. Lee et al. (Reference Lee, Miller and Blouin2009) observed a cotton density of 5.25 plants m−1 row would have to interfere with soybean for at least 6 wk following emergence before yield reduction of less than 1% would be expected. The critical need to control cotton volunteers is actually driven by boll weevil (Anthonomus grandis Boem.) and cotton diseases such as the newly identified cotton leafroll dwarf virus disease (Bag et al. Reference Bag, Tabassum, Sedhain, Whitaker, Chee, Roberts and Kemerait2019; Francischini et al. Reference Francischini, Constantin, Matte, Oliveira, Rios and Machado2019; Greenberg et al. Reference Greenberg, Sparks, Norman, Coleman, Bradford, Yang, Sappington and Showler2007).
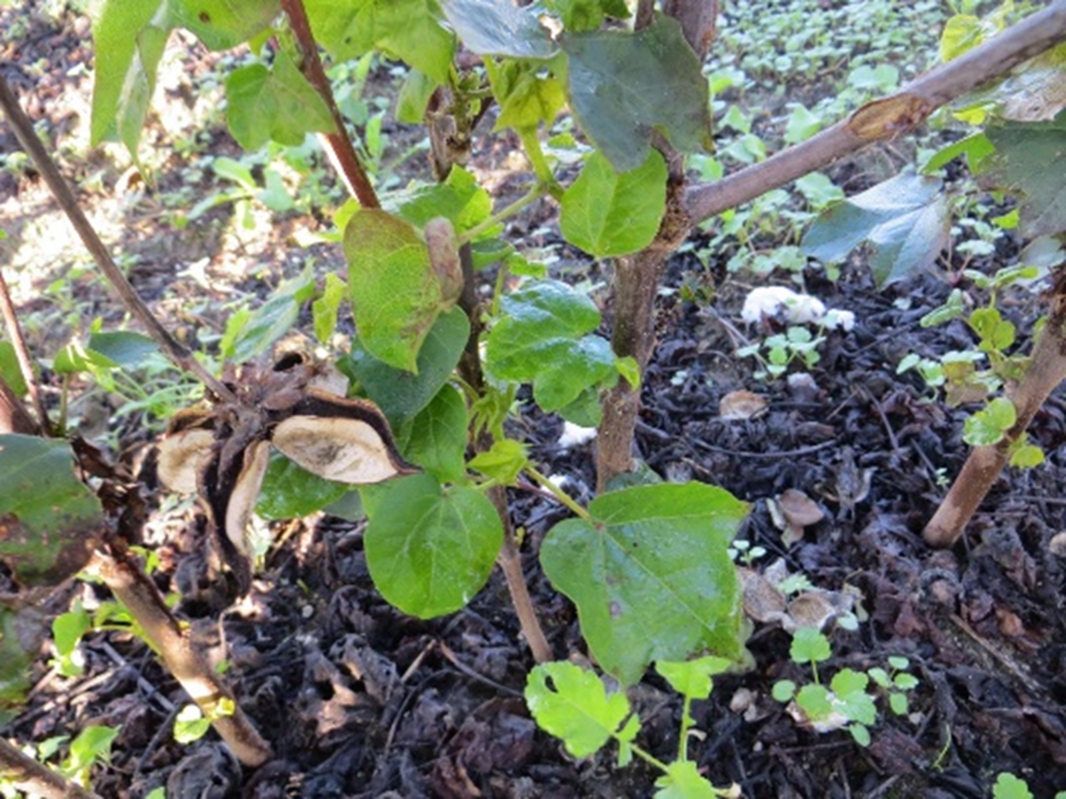
Figure 5. Cotton stalk regrowth in a field in Georgia.
Anthonomus grandis has been eradicated from most of the Cotton Belt in the United States (Barker et al. Reference Barker, Parker, King and Anderson2001; NCC 2020; USDA-APHIS 2013). After eradication, a containment program with trapping at each cotton field is necessary to detect reintroduced A. grandis, which allows for immediate mitigation plans. Crops other than cotton are not monitored for A. grandis, as it is the only host; hence fruit on cotton volunteers in other crops could provide oviposition sites for A. grandis and allow the insect to build up undetected. If cotton volunteers are detected in rotational crops, farmers are required to control the plants completely or pay to have the field trapped by the Boll Weevil Eradication Program. Cotton leafroll dwarf virus disease, transmitted by aphids, was identified in the United States during 2017. With no current leafroll dwarf virus–resistant cotton cultivars, concern of spread and impact has become relevant (Bag et al. Reference Bag, Tabassum, Sedhain, Whitaker, Chee, Roberts and Kemerait2019). Preventing cotton plants and potentially the disease from overwintering may be one critical component in a long-term disease mitigation plan protecting the cotton industry (Nichols Reference Nichols2020).
Management of Herbicide-Resistant Cotton Volunteers
Controlling Cotton Volunteers from Seed
Cotton is extremely unique when managing volunteers. Production practices implementing tillage can be effective. Deep tillage to place the seed at a depth where it cannot emerge or conducting secondary tillage if the growing point is removed from the plant have been successful (Charles et al. Reference Charles, Roberts, Kerlin and Hickman2013; Morgan et al. Reference Morgan, Fromme, Baumann, Grichar, Bean, Matocha and Mott2012). In conservation production, where tillage is not an option, volunteers emerging from seed can still be controlled. The POST applications of 2,4-D or 2,4-DB (non-resistant cultivars), atrazine, bromoxynil, carfentrazone, chlorimuron, diquat, flumiclorac, paraquat, glufosinate (non-resistant cotton), and saflufenacil have been effective when applied to young, less than four-node cotton, growing in favorable conditions (Charles et al. Reference Charles, Roberts, Kerlin and Hickman2013; Morgan et al. Reference Morgan, Fromme, Baumann, Grichar, Bean, Matocha and Mott2012; York et al. Reference York, Stewart, Vidrine and Culpepper2004). In these studies, 2,4-D, bromoxynil, carfentrazone, and paraquat were consistently effective. Residual herbicides such as diclosulam, metribuzin, and sulfentrazone have also provided effective control but are dependent upon being activated by a timely rainfall or irrigation (Kichler and Culpepper Reference Kichler and Culpepper2020; Minozzi et al. Reference Minozzi, Christoffoleti, Monquero, Zobiole, Pereira and Duck2017; York et al. Reference York, Stewart, Vidrine and Culpepper2004). The most effective approach to manage volunteer cotton emerging from seed in a rotation crop would be by eliminating any plants before planting with tillage or herbicides and then designing a PRE followed by a POST herbicide program (York et al. Reference York, Stewart, Vidrine and Culpepper2004).
Controlling Cotton Volunteers from Stalk Regrowth
Ratooned cotton volunteers are difficult to manage. Smart and Bradford (Reference Smart and Bradford1997) found that a moldboard plow and stalk puller were very effective for removing cotton stalks. These approaches would be more effective than relying on herbicides, which rarely eliminate all volunteers (Braz et al. Reference Braz, Andrade, Nicolai, Lopez Ovejero, Cavenaghi, Oliverira, Christoffoleti, Pereira, Constantin and Guimaraes2019; Ferreira et al. Reference Ferreira, Bogiani, Sofiatti and Filho2018; Greenberg et al. Reference Greenberg, Sparks, Norman, Coleman, Bradford, Yang, Sappington and Showler2007). When attempting to control ratooned plants with herbicides, it is critical to consider treatments that are most effective immediately after cotton harvest and not in the following spring (Charles et al. Reference Charles, Roberts, Kerlin and Hickman2013). In addition, sequential applications of 2,4-D (non–2,4-D-resistant cotton volunteers) are usually required for success. Applications should begin after stalk shredding (adequate regrowth, approximately 2 wk) with a second application made after new regrowth occurs, generally 3 to 6 wk later (Braz et al. Reference Braz, Andrade, Nicolai, Lopez Ovejero, Cavenaghi, Oliverira, Christoffoleti, Pereira, Constantin and Guimaraes2019; Ferreira et al. Reference Ferreira, Bogiani, Sofiatti and Filho2018; Greenberg et al. Reference Greenberg, Sparks, Norman, Coleman, Bradford, Yang, Sappington and Showler2007; Yang et al. Reference Yang, Greenberg, Everitt and Norman2006). Few studies have shown that herbicides such as carfentrazone, saflufenacil, glufosinate, and flumiclorac included with the second 2,4-D application improved control (Braz et al. Reference Braz, Andrade, Nicolai, Lopez Ovejero, Cavenaghi, Oliverira, Christoffoleti, Pereira, Constantin and Guimaraes2019; Francischini et al. Reference Francischini, Constantin, Matte, Oliveira, Rios and Machado2019). Francischini et al. (Reference Francischini, Constantin, Matte, Oliveira, Rios and Machado2019) observed glyphosate plus 2,4-D plus saflufenacil applied sequentially was the most effective for controlling non–2,4-D-resistant cotton volunteers. It cannot be emphasized enough that drought stress at application time will greatly reduce control (Braz et al. Reference Braz, Andrade, Nicolai, Lopez Ovejero, Cavenaghi, Oliverira, Christoffoleti, Pereira, Constantin and Guimaraes2019). The literature is overwhelmingly clear that sequential 2,4-D applications can be highly effective in mitigating ratooned cotton growth; however, with 2,4-D–resistant cotton, volunteer control using herbicides is far more complex. Effective tillage options remain sustainable, but effective herbicide programs have not been fully developed. The use of dicamba applied alone has been variable (McGinty et al. Reference McGinty, Morgan, Livingston and Mott2019; Yang et al. Reference Yang, Greenberg, Everitt and Norman2006). Current efforts to develop cotton traits with dicamba with other SOA herbicides (e.g., thidazuron) and duplosan-based systems (Anonymous 2020b) may be the more effective herbicide options currently available for 2,4-D–resistant cotton stalk management (Figure 6).
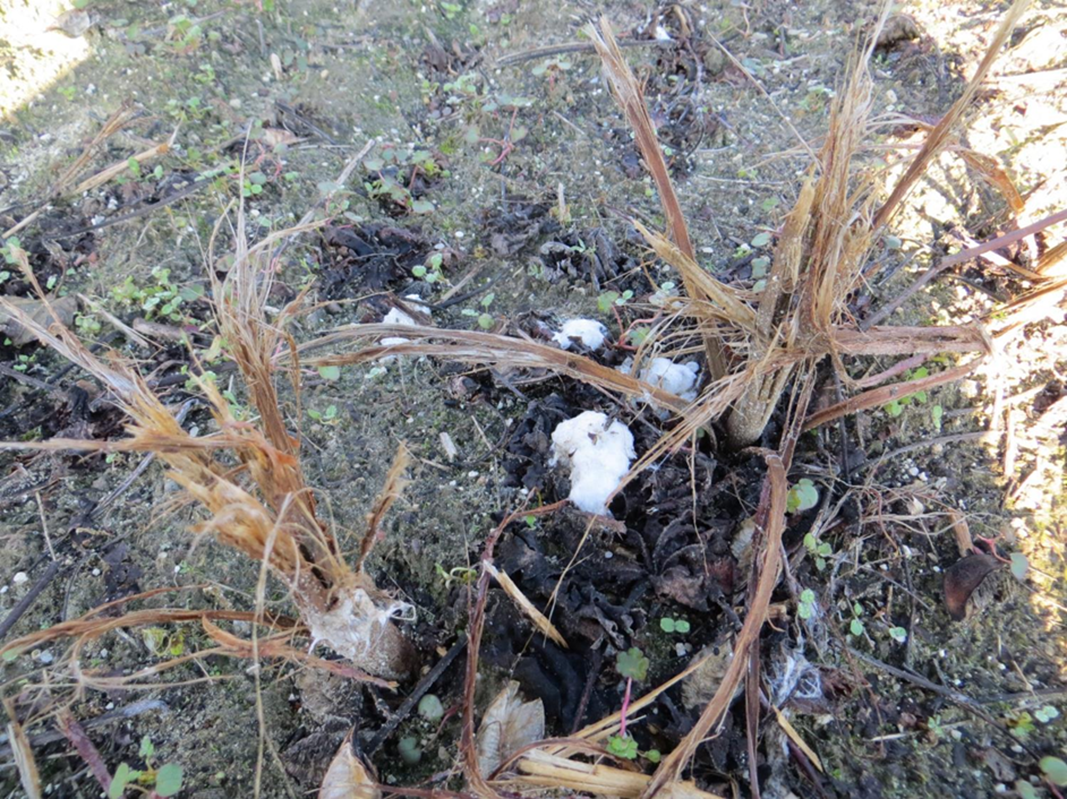
Figure 6. Stalk regrowth of 2,4-D-resistant cotton after shredding and treatment with duplosan.
Rice
Rice is a member of the Poaceae family, and evidence suggests domestication first occurred in the Yangtze River region of China (Lu et al. Reference Lu, Liu, Wu, Berné, Saito, Liu and Wang2002). Most of the world’s rice is grown in Asia, with China, India, and Indonesia being the leading rice-producing countries. Most of the Asian rice is an indica type, whereas a japonica type is common in the midsouthern United States (USDA-ERS 2020b). Rice in the United States is grown on approximately 1.1 to 1.2 million ha annually on poorly drained soils, mainly in Arkansas, California, Louisiana, Mississippi, Missouri, and Texas. Rice fields are often flooded for a prolonged period to aid weed management, especially control of grasses that are most problematic in the crop. While transplanted rice is the predominant means of establishment in the leading rice-producing countries in Asia, farmers in the United States rely solely on dry-seeded or water-seeded establishment techniques.
Red rice, a closely related weedy relative of rice, is often categorized as Oryza sativa L. although samples from across the southern United States rice-growing region have revealed tremendous diversity within and among fields based on molecular markers, even the inclusion of Oryza rufipogon Griffiths in some states (Vaughn et al. Reference Vaughn, Ottis, Prazak-Havey, Sneller, Chandler and Park2001). This diversity among populations along with similarities to cultivated rice makes management of the weed virtually impossible with herbicides alone in the absence of a herbicide resistance trait. Because of the propensity of rice and Oryza sativa f. spontanea to outcross due to synchronization of flowering, especially rice cultivars from japonica backgrounds, pollen-mediated gene flow between the crop and weed is common, resulting in offspring having characteristics of the weedy and cultivated parents (Shivrain et al. Reference Shivrain, Burgos, Rajguru, Anders, Moore and Sales2007, Reference Shivrain, Burgos, Sales, Mauromoustakos, Gealy, Smith, Black and Jia2009b, 2010). Hybrid rice makes up the majority of rice grown in the midsouthern United States, and some level of seed shattering during or before harvest is common, with subsequent generations exhibiting weedy characteristics such as dormancy, differential stature, and progression of the imidazolinone resistance trait, especially where IR rice is grown without crop rotation. With O. sativa f. spontanea, outcrosses, and volunteer hybrids all being of the same genus as cultivated rice, the term “weedy rice” will be used to encompass all unwanted types of rice in this review.
Oryza sativa f. spontanea is most problematic in fields where rice has been repeatedly grown without rotation to other crops such as soybean. In Arkansas, some landowners seldom allow farmers that rent their ground to rotate from zero-grade rice fields, because these fields are highly prone to flooding and are hence not conducive for production of a high-yielding alternative crop (Burgos et al. Reference Burgos, Norsworthy, Scott and Smith2008). Because rice is repeatedly grown in these fields, O. sativa f. spontanea populations quickly build up in the soil seedbank. Oryza sativa f. spontanea often produces more biomass and tillers, grows taller, and outcompetes cultivated rice (Burgos et al. Reference Burgos, Norsworthy, Scott and Smith2008; Estorninos et al. Reference Estorninos, Gealy, Talbert and Gbur2005). Based on densities present in fields in Arkansas in 2006, it was estimated that weedy rice caused an average of US$275 ha−1 in yield losses, without including dockage for reductions in grain quality (Burgos et al. Reference Burgos, Norsworthy, Scott and Smith2008). Studies have estimated that from 2002 to 2014, the economic loss from dockage at the mill caused by weedy rice was US$295 ha−1 in heavy infested fields (Durand-Morat and Nalley Reference Durand-Morat and Nalley2019).
Herbicide-Resistant Traits in Rice
In the mid-1990s, scientists with the Louisiana State University Agricultural Center (LSU AgCenter) successfully created glufosinate-resistant rice through insertion of the bar gene into the crop (Oard et al. Reference Oard, Linscombe, Braverman, Jodari, Blouin, Leech, Kohli, Vain, Cooley and Christou1996). Although sequential applications of glufosinate were effective for controlling O. sativa f. spontanea (Sankula et al. Reference Sankula, Braverman, Jodari, Linscombe and Oard1997), commercialization of glufosinate-resistant rice never occurred, because it did not gain import approval in many countries. Subsequently, LSU AgCenter scientists developed IR (Clearfield®) inbred and IR-hybrid rice with a non-transgenic trait; they was commercialized in 2002 and 2003, respectively (Croughan Reference Croughan2003). The IR technology allowed selective removal of emerged weedy rice from cultivated rice, which was not previously possible with any herbicide. By 2006, growers and consultants began to report O. sativa f. spontanea populations with resistance to imazethapyr and imazamox, the two imidazolinone herbicides labeled for use in IR rice (Burgos et al. Reference Burgos, Norsworthy, Scott and Smith2008). As populations of IR O. sativa f. spontanea continued to increase because of IR hybrid volunteers and outcrossing with weedy relatives, utility of the technology diminished (Hardke Reference Hardke2020; Sudianto et al. Reference Sudianto, Beng-Kah, Ting-Xiang, Saldain, Scott and Burgos2013).
To combat IR O. sativa f. spontanea populations, quizalofop-P-ethyl–resistant rice, another non-transgenic trait, was commercialized in 2019 (Sanders et al. Reference Sanders, Bond, Lawrence, Golden, Allen and Bararpour2020). The use of this technology has been limited because of the low yield potential of available cultivars (Hardke et al. Reference Hardke, Frizzell, Castaneda-Gonzalez, Frizzell, Hale, Clayton, Moldenhauer, Sha, Shakiba, Wamishe and Wisdom2020). Two additional herbicide resistance traits in rice could become available in the near future, permitting use of oxyfluorfen or 4-hdyroxyphenylpyruvate dioxygenase (HPPD)-inhibiting herbicides for selective control of weedy rice in rice fields (Hinga et al. Reference Hinga, Moon, Channarayappa, Rasmussen and Cuevas2018; McKenzie et al. Reference McKenzie, Andaya, Andaya and De Leon2020). Benzobicyclon, an HPPD-inhibiting herbicide that is anticipating registration in rice, can be safely applied to the crop in the absence of a herbicide trait for selective removal of some, but not all, weedy rice accessions (Figure 7) (Young et al. Reference Young, Norsworthy, Scott, Bond and Heiser2018).
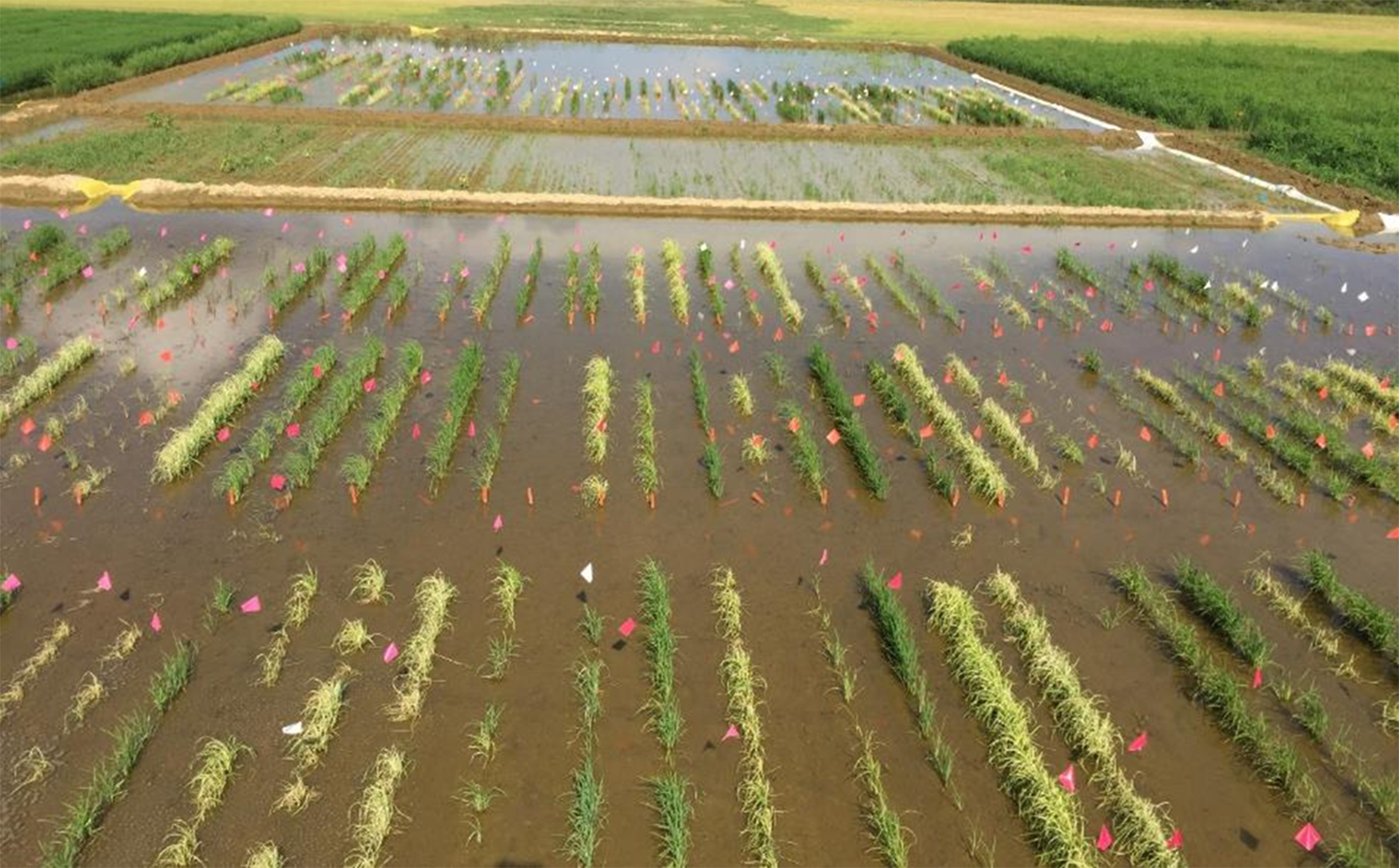
Figure 7. Individual rows of weedy rice accessions or cultivated rice cultivars 8 d following a post-flood application of benzobicyclon at 371 g ai ha−1. Healthy rows are cultivated rice or resistant weedy rice accessions, whereas chlorotic rows are benzobicyclon-sensitive weedy rice accessions.
Interference of Herbicide-Resistant Rice Volunteers and Weedy Rice
Grains from rice volunteers from a different cultivar can reduce the milling quality of rice grown in rotation if volunteers are present above threshold levels (Singh et al. Reference Singh, Burgos, Singh, Gealy, Gbur and Caicedo2017). Hybrid rice is better able to overwinter than inbred rice; therefore, hybrid rice results in more volunteers (Singh et al. Reference Singh, Burgos, Singh, Abugho, Earnest, Gbur and Scott2016). In addition, rice volunteers from hybrid rice can emerge at different times because of variability in emergence pattern and different depth of seed placement (Sudianto et al. Reference Sudianto, Beng-Kah, Ting-Xiang, Saldain, Scott and Burgos2013). Singh et al. (Reference Singh, Burgos, Singh, Gealy, Gbur and Caicedo2017) surveyed rice fields in Arkansas and reported that at 8% infestation, volunteer rice can impact head rice yield and can reduce total yield at 18% infestation. Similarities between weedy rice and cultivated rice make it challenging to differentiate and selectively hand remove O. sativa f. spontanea from a rice field during early developmental stages, and early-season interference can have a negative effect on the crop. Literature on interference of volunteer rice in the crop grown in rotation is very limited; therefore, this section will focus on interference of O. sativa f. spontanea. Oryza sativa f. spontanea interference at a density of 20 plants m−2 for as few as 40 d after rice emergence can reduce rough rice grain yield as much as 6% (Kwon et al. Reference Kwon, Smith and Talbert1991). Yield losses can approach 85% at a density of 50 weedy rice plants m−2 following season-long interference, and complete loss of the crop can occur at higher densities in some instances (Smith Reference Smith1988; JKN, personal observation). In addition to crop yield loss from interference, O. sativa f. spontanea at high densities often results in lodging of the cultivated crop, which further complicates harvesting.
Oryza sativa f. spontanea density in cultivated rice is not the only factor that influences the degree of interference and lodging. Because O. sativa f. spontanea ecotypes greatly differ morphologically, lodging and interference with the cultivated crop is also dependent upon the characteristics of the weed. Some tall-statured O. sativa f. spontanea ecotypes can produce more than twice the tillers and aboveground biomass compared with short-statured ecotypes (Estorninos et al. Reference Estorninos, Gealy, Talbert and Gbur2005). When competing with the inbred, long-grain ‘Kaybonnet’ cultivar, short- and tall-statured O. sativa f. spontanea at 31 plants m−2 reduced rice grain yields approximately 14% and 68%, respectively, demonstrating strong differences in competitiveness among ecotypes (Estorninos et al. Reference Estorninos, Gealy, Talbert and Gbur2005). Similarly, for cultivated rice, semi-dwarf, inbred cultivars are less competitive with O. sativa f. spontanea than taller-statured or hybrid cultivars (Shivrain et al. Reference Shivrain, Burgos, Gealy, Smith and Scott2009a; Sudianto et al. Reference Sudianto, Beng-Kah, Ting-Xiang, Saldain, Scott and Burgos2013).
Management of Herbicide-Resistant Rice Volunteers and Weedy Rice
Rice volunteers resulting from the planting of ALS inhibitor–resistant hybrid rice cannot be selectively controlled in conventional rice or ALS inhibitor–resistant rice the subsequent year. The only effective way to control volunteer rice from cultivated rice is to use an alternative trait such as quizalofop-P-ethyl–resistant rice (Provisia®) to control IR rice volunteers. A recent study in Mississippi reported that early-POST followed by a late-POST application of quizalofop-P-ethyl provided greater than 95% control of rice volunteers and other grass weeds in ACCase inhibitor–resistant rice (Sanders et al. Reference Sanders, Bond, Lawrence, Golden, Allen and Bararpour2020). Volunteer rice is not a major issue in broadleaf crops grown in rotation because of availability of selective herbicides; therefore, this section will mainly focus on management of O. sativa f. spontanea. Before IR rice, crop rotation to soybean or water-seeded crop establishment were two strategies frequently used for O. sativa f. spontanea management (Griffin and Harger Reference Griffin and Harger1990). By rotating to soybean, a wide array of residual and POST herbicides could be used to control O. sativa f. spontanea, ultimately minimizing, or preventing weedy rice seed production. In addition to providing effective control when applied in a timely manner in soybean, fluazifop and quizalofop applied late in the season substantially reduced weedy rice seed production, aiding long-term management in a rice–soybean rotation (Salzman et al. Reference Salzman, Smith and Talbert1988). With commercialization of GR soybean in 1996, rotation to soybean became a more effective means for controlling O. sativa f. spontanea because of in-crop glyphosate use. There are no known O. sativa f. spontanea populations with resistance to glyphosate, and complete control can be achieved with sequential applications of glyphosate (Barber et al. Reference Barber, Butts, Cunningham, Selden, Norsworthy, Burgos and Bertucci2020).
Soybean
Soybean, also referred as soya or soyabean, is the most important oilseed crop in the world as well as in the United States. East Asia is believed to be the center of origin of soybean, where it was domesticated from wild soybean (Glycine soja Sieb. & Zucc.) 9,000 yr ago (Carter et al. Reference Carter, Hymowitz and Nelson2004). Soybean is primarily used for edible oil and high-quality protein for human consumption and animal feed (Hartman et al. Reference Hartman, West and Herman2011). The majority of soybean is produced in North and South America. The United States is among the top two largest soybean-producing countries in the world, with the production of 96.7 billion kg in 2019 with an average productivity of 3,190 kg ha−1 (USDA−NASS 2020). Illinois, Iowa, Minnesota, Nebraska, Indiana, Missouri, and Ohio were major soybean-producing states in the United States in 2019 (USDA−NASS 2020). Soybean production in Canada has been historically in Ontario and Quebec; however, in recent years, growers have adopted GR soybean in Manitoba and Saskatchewan (Mierau et al. Reference Mierau, Johnson, Gulden, Weber, May and Willenborg2019).
Herbicide-Resistant Traits in Soybean
GR soybean has been commercially available since 1996 in the United States and 1997 in Canada (Beckie and Owen Reference Beckie and Owen2007). Glufosinate-resistant soybean has been commercially available since 2009 in the United States. Soybean resistant to multiple herbicides (glyphosate/dicamba; Roundup Ready 2 Xtend®) came to the market in the 2017 growing season, and 2,4-D/glufosinate/glyphosate-resistant soybean (Enlist E3®) was commercially available from the 2019 growing season in the United States (Beckie et al. Reference Beckie, Ashworth and Flower2019). Soybean resistant to isoxaflutole/glufosinate/glyphosate (LibertyLink®/GT27™) was available for commercial cultivation in the United States in 2020; however, isoxaflutole (Alite 27®) is registered in selected counties in several states (Anonymous 2020a). Soybean resistant to dicamba/glufosinate/glyphosate (XtendFlex®) has been developed and recently received import approval by the European Commission and will be available commercially in the 2021 growing season in the United States.
Interference of Herbicide-Resistant Soybean Volunteers
Volunteer soybean is not generally considered a problem weed, because seeds do not overwinter well, particularly in the midwestern United States and Canada (Owen Reference Owen2005). In warmer climates, such as the southern United States, however, volunteer soybean can be a problem weed in rice, as the majority of rice is grown in rotation with soybean in Mississippi (Bond and Walker Reference Bond and Walker2009). A survey conducted in 2008 reported GR volunteer soybean as the third most troublesome weed in rice production fields in Mississippi (Webster Reference Webster2008). In Asian countries such as India and China, volunteer soybean is not a major weed because of intensive agricultural practices and prevailing manual weed control (Lu Reference Lu2005). Under unfavorable conditions such as flooded fields and wet soil conditions when soybean fields do not get harvested, volunteer soybean can be a problem (Figure 8). Additionally, some fields occasionally experience significant shattering due to windstorm or a hailstorm in the fall, with soybean seed knocked from the plants and thus not harvested, which may result in volunteer soybean the following year. Volunteer soybean is usually a weak competitor, particularly when corn is planted in rotation (Figure 8).

Figure 8. Volunteer soybean in a cornfield in Nebraska.
Management of Herbicide-Resistant Soybean Volunteers
Limited literature is available on management of volunteer soybean in rotational crops, primarily because the majority of volunteer soybean seeds do not survive, or herbicides are available that can effectively control soybean volunteers in rotational crops. Atrazine, applied alone or in a premix or tank mixture with other herbicides commonly used in corn, can provide effective control of soybean volunteers in corn (Owen Reference Owen2005). Most soybean grown in the United States is GR; therefore, glyphosate is not an option for control of GR soybean volunteers. Field experiments were conducted in Nebraska at multiple locations for control of GR volunteer soybean in GR corn with POST herbicides. When soybean volunteers were at the V2 to V3 stage, POST corn herbicides such as atrazine in a mixture with mesotrione, tembotrione, or topramezone provided 90% control; however, when soybean volunteers were at the V5 to V6 growth stage, those herbicides were not effective, and dicamba applied alone or in a mixture with diflufenzopyr provided 90% control (Jhala et al. Reference Jhala, Sandell, Kruger, Wilson and Knezevic2013). Soybean resistant to dicamba/glyphosate has been rapidly adopted by growers in the United States; therefore, dicamba/glyphosate−based herbicides will not be effective for their control. Additionally, 2,4-D/glufosinate/glyphosate-resistant volunteer soybean will make these herbicides ineffective for their control. A study in Mississippi reported paraquat can provide at least 95% control of GR soybean volunteers when applied immediately after rice planting (Bond and Walker Reference Bond and Walker2009). The same study reported POST rice herbicides such as triclopyr, bispyribac-sodium, penoxsulam, and halosulfuron provided >95% control of GR soybean volunteers in rice. York et al. (Reference York, Stewart, Vidrine and Culpepper2005) reported that GR soybean volunteers can be controlled with trifluroxysulfuron applied POST in cotton. Soltani et al. (Reference Soltani, Shropshure and Sikkema2019) reported that halosulfuron applied early POST provided 90% control of GR soybean volunteers in white bean (Phaseolus vulgaris L.) in field studies conducted in Ontario, Canada. Brighenti (Reference Brighenti2015) reported that volunteer soybean can be a problem weed in sunflower (Helianthus annuus L.) grown in rotation in Brazil, with no selective herbicide available for effective control without sunflower injury.
Sugarbeet
Sugarbeet is a biennial root crop, providing approximately 20% of the worldwide sugar supply (ISO 2019) and approximately 55% of the total sugar consumed in the United States (USDA-ERS 2020c). Sugarbeet is a member of the Betoidae subfamily within Amaranthaceae and includes approximately 2,500 species divided into 175 genera (Müller and Borsch Reference Müller and Borsch2005). Sugarbeet is largely cultivated in temperate regions in the Northern Hemisphere, including 11 states in the United States, two provinces of Canada, France, Germany, and Russia. Cultivated beets (fodder, chard, table beet, sugarbeet) are derived from wild Mediterranean Sea beet [Beta vulgaris ssp. maritima (L.) Arcang.] (OECD 2001). Sugarbeet has been a relatively important crop in the Americas since the late 19th century. One of the earliest attempts to grow sugarbeet in the United States was in 1836 (Kaufman Reference Kaufman2008), and the first sugarbeet processing facility was in Massachusetts in 1838 (Harris Reference Harris1919).
Herbicide-Resistant Traits in Sugarbeet
The GR sugarbeet was deregulated in 1998 but not sold commercially until 2005 due to concern from the sugar processors (USDA-APHIS 1999). A second event was deregulated in 2005 after completing environmental assessment demonstrating that GR sugarbeet was unlikely to pose a plant pest risk to agricultural crops or other plants or plant products, and it has been available commercially since 2008. Dexter et al. (Reference Dexter, Rothe, Luecke and Smith1999) reported glyphosate on GR sugarbeet should be applied for control of small weeds 2 to 3 wk after the cotyledon sugarbeet stage to avoid early-season weed competition and yield loss. Dexter and Luecke (Reference Dexter and Luecke2000) reported glyphosate at 0.84 kg ha−1 applied at the cotyledon, 4-leaf and 10-leaf sugarbeet stage provided 100% control of green foxtail [Setaria viridis (L.) P. Beauv.], yellow foxtail [Setaria pumila (Poir.) Roem. & Schult.], kochia [Bassia scoparia (L.) A. J. Scott], and redroot pigweed (Amaranthus retroflexus L.) with 0% visible sugarbeet stature reduction. Weed control and sugarbeet root yield were consistently greater in commercial fields utilizing GR cultivars compared with fields utilizing conventional cultivars (Glascock Reference Glascock2006). A survey of sugarbeet growers attending the 2020 weed control and sugarbeet production seminars indicated between two and three glyphosate applications per season in Minnesota and North Dakota in 2019 (Peters et al. Reference Peters, Khan, Lystad and Boetel2020). Commercial adoption of GR sugarbeet was rapid in the United States. More than 60% of harvested sugarbeet acreage was planted using GR sugarbeet seed in 2008. Adoption rates rose to 95% by 2009 (Fernandez-Cornejo et al. Reference Fernandez-Cornejo, Wechsler and Milkove2016) and 98.5% in 2013 (ISAAA 2014).
Interference of Herbicide-Resistant Sugarbeet Volunteers
Sugarbeet volunteers mostly occur from seed produced by cultivated sugarbeet, although volunteers infrequently develop from seed of weed beet or portions of root and attached crown that overwinter after harvest. Weed beet is an off-type Beta vulgaris ssp., derived by pollen-mediated gene flow from sea beet (Beta vulgaris ssp. maritima) during variety development, and carries the dominant allele ‘B’ for annual habit at high frequency. Weed beet, largely eradicated as a contaminant in sugarbeet genetics, can bolt, flower, and set seed without vernalization. Sugarbeet cultivars grown in California (overwintered or winter planted) require non-bolting genetics, whereas cultivars grown in Colorado, Minnesota, and North Dakota do not (R Lewellen, USDA, Salinas, CA, personal communication). Sugarbeet volunteers are rarely observed growing along roadsides or in ditches from sugarbeet roots lost during transportation from field to processing facilities.
Incidence of GR sugarbeet volunteerism is production-area specific. Sugarbeet volunteers have been observed in Alberta, Canada, especially when GR corn follows sugarbeet, but volunteers are not a production challenge. In 2020, there was no significant GR sugarbeet volunteer issue, even after producers left 45% of their 2019 planted GR sugarbeet in the field due to weather-related challenges (TJ Peters, personal observation). Major crops following GR sugarbeet in the Imperial Valley, California, are alfalfa, wheat, bermudagrass (Cynodon dactylon L.), sudan grass [Sorghum bicolor (L.) Moench. ssp. drummondii (Nees ex Steud.) de Wet & Harlan], winter vegetables, and melons; however, sugarbeet volunteers are not a production challenge. Volunteer sugarbeet is a rare problem in Michigan and usually not observed in high populations; however, they are occasionally observed in GR soybean fields (C Sprague, Michigan State University, personal communication). Sugarbeet volunteers are derived from either vegetative propagules or seeds in Minnesota and North Dakota, but at very low incidence rates, and do not interfere with yield of crops grown in rotation. The technology agreement between producers and the seed companies requires producers to remove bolters, thus minimizing production of volunteer seed. Sugarbeet volunteers have been observed when wheat, onion (Allium cepa L.), or dry bean (Phaseolus vulgaris L.) follow GR sugarbeet in Oregon (J Felix, Oregon State University, personal communication). Onion is direct seeded in late February and March before sugarbeet volunteers sprout, and they can occasionally become an early-season production challenge in onion in Oregon. Volunteer sugarbeet occurs only on rare occasions in Ontario, as temperature fluctuation above and below freezing between growing seasons triggers emergence.
Management of Herbicide-Resistant Sugarbeet Volunteers
Intensity of volunteerism and management approach is sugarbeet production-area specific in the United States and Canada. Sugarbeet volunteers are easily controlled with broadleaf herbicides used in cereal crops in Alberta, Canada. Volunteers are not a production challenge in the Imperial Valley, California. Bolters also are not a problem, because high temperatures tend to reverse the effects of vernalization. ALS inhibitors have been used for controlling sugarbeet volunteers in GR soybean in Michigan (C Sprague, Michigan State University, personal communication). Small grains and corn are grown in rotation with sugarbeet in Minnesota, North Dakota, and Ontario, Canada, and auxinic herbicides applied in these crops can easily control GR sugarbeet volunteers. Similarly, in Nebraska, sugarbeet volunteers are easily controlled using synthetic auxin herbicides in fields planted to corn and imazamox in dry bean (N Lawrence, University of Nebraska–Lincoln, personal communication). Most volunteer sugarbeet sprouted roots are uprooted during seedbed preparation, as soybean or dry bean are seeded in late May to early June in Oregon (J Felix, Oregon State University, personal communication). Very few sugarbeet volunteers are visible following herbicide application with no yield interference. In onion, pendimethalin, bromoxynil, oxyfluorfen, S-metolachlor, dimethenamid-P, fluroxypyr, or ethofumesate do not provide complete control of GR sugarbeet volunteers; however, growers normally use hand weeding to remove surviving volunteers. Sequential POST application of bromoxynil or oxyfluorfen followed by hand weeding to control volunteer sugarbeet and rarely cause yield loss in onion. Corn or barley typically follows sugarbeet in Wyoming and Colorado, and use of tillage between sugarbeet and those rotational crops is common to remove sugarbeet volunteers, if present (A Kniss, University of Wyoming, personal communication).
Wheat
Common or bread wheat is a member of the Poaceae family and a highly self-pollinated species. Modern wheat cultivars are either tetraploid (durum, AABB) or hexaploid (common and club-types, AABBDD), descended from a wild form of Triticum monococcum (L.) sensu lato in the Middle East (the Fertile Crescent) (biology reviewed in CFIA 2014; Willenborg and Van Acker Reference Willenborg and Van Acker2008). Common and durum wheat (Triticum durum Desf.) are often grown in the same areas globally, although common wheat is more prevalent. Volunteerism and response to herbicides are similar between both types of wheat. Wheat is widely grown globally in both temperate and Mediterranean climates. Winter or spring wheat is commonly grown across the Great Plains or Pacific Northwest region of North America. In 2020, the area planted to wheat in Canada was 10.1 million ha (Statistics Canada 2020), while the area planted in the United States was 17.9 million ha (USDA-NASS 2020). Because wheat has historically occupied the largest crop area across the Great Plains, the evolution of HR weeds has also been the greatest: 77 weed species or >140 biotypes (Heap Reference Heap2020). In particular, selection of ACCase or ALS inhibitor–resistant grass weeds such as wild oat (Avena fatua L.) threatens sustainable wheat production.
Herbicide-Resistant Traits in Wheat
Two non-transgenic HR traits have been incorporated into wheat: ACCase- and ALS- (imidazolinone) inhibitor resistance (Nakka et al. Reference Nakka, Jugulam, Peterson and Asif2019). GR wheat was developed and evaluated for cultivation in Canada and the United States but was not commercially introduced because of lack of market acceptance (Berwald et al. Reference Berwald, Carter and Gruere2006). However, its expected introduction led to several excellent research studies evaluating various aspects of the biology, ecology, and management of the crop, including volunteers. An imidazolinone-resistant (Clearfield®) spring and winter wheat system has been available since the early 2000s (Figure 9). Clearfield® Plus wheat is like Clearfield® wheat, but has two genes that endow high-level resistance to imazamox. Adoption of IR spring wheat is relatively low in Canada, whereas adoption of winter wheat cultivars with this trait is relatively high in the United States. For example, IR winter wheat cultivars in Nebraska collectively occupy the largest cultivated area of the crop (Baenziger et al. Reference Baenziger, Rose, Santra, Guttieri and Xu2016). The difference in adoption rates between the two countries is likely attributable to weed control benefits and profitability relative to non-HR (conventional) wheat. With increasing incidence of ALS-inhibitor resistance or to improve the level of control in a number of important grass weeds, such as jointed goatgrass (Aegilops cylindrica Host) and downy brome (Bromus tectorum L.), another HR trait was needed by growers. CoAXium® (two gene)/AXigen® (one gene) HR cultivars were introduced in the Great Plains of the United States in 2018. They are resistant to quizalofop, an ACCase inhibitor, and provide control of troublesome grassy weeds such as feral rye (cereal rye, Secale cereale L.), B. tectorum, and volunteer wheat or barley. Cultivars with this trait are currently grown on 243,000 ha in the Great Plains and Pacific Northwest regions of the United States (Weaver Reference Weaver2020). The trait was initially developed in hard red winter wheat cultivars but will be expanded to other wheat classes in the future.

Figure 9. Field-scale evaluation of imidazolinone-resistant (Clearfield®) wheat compared with non–herbicide resistant wheat (including volunteers the following year) in Saskatchewan, Canada, in the early 2000s (adapted from Beckie et al. Reference Beckie, Warwick, Sauder, Hall, Harker and Lozinski2011).
Interference of Herbicide-Resistant Wheat Volunteers
Wheat volunteers can be very competitive if not controlled and can cause substantial yield losses in broadleaf crops such as canola, flax (Linum usitatissimum L.), and alfalfa (Harker et al. Reference Harker, Clayton, Blackshaw, O’Donovan, Johnson, Gan, Holm, Sapsford, Irvine and Van Acker2005). In the semi-arid Central Great Plains, uncontrolled volunteer wheat can use an average of 8 cm of soil water and therefore significantly reduce the yields of rotational crops such as corn or sorghum [Sorghum bicolor (L.) Moench ssp. bicolor]. Average harvest losses for wheat have been estimated at 300 seeds m−2 (Clarke Reference Clarke1985), markedly fewer than for canola. The lack of seed dormancy means that seedbank persistence depends on factors affecting germination and mortality. The seedbank of wheat is relatively short-lived. In studies across western Canada, the maximum viability of wheat seeds at 0-, 2-, and 15-cm depth in the spring following planting the previous fall was 43%, 7%, and 2%, respectively; 99% loss of seed viability occurred from 490 to 1,110 d, 320 to 650 d, and 175 to 350 d after planting, respectively (Nielson et al. Reference Nielson, McPherson, O’Donovan, Harker, Yang and Hall2009). In another study in the region, seedbank persistence did not exceed 1 yr, and cumulative emergence from the seedbank occurred mostly in early spring, averaging only 4.3% (De Corby et al. Reference De Corby, Van Acker, Brûlé-Babel and Friesen2007). Similarly, Harker et al. (Reference Harker, Clayton, Blackshaw, O’Donovan, Johnson, Gan, Holm, Sapsford, Irvine and Van Acker2005) reported that most wheat volunteers emerged in spring before time of crop seeding. In the Central Great Plains, volunteer wheat seed generally persists in the soil for less than 1 yr, and seedlings emerge periodically over the growing season for up to 2 yr after harvest (Anderson and Soper Reference Anderson and Soper2003). Wheat volunteers can act as a green bridge or host for numerous plant pathogens and insects between cropping seasons in addition to interference in rotational crops (Cook and Veseth Reference Cook and Veseth1991). Wheat volunteers can be a host for mites that carry wheat streak mosaic and other viral diseases (e.g., barley yellow dwarf virus), which collectively can cause severe crop yield losses. Wheat volunteers can attract the Russian wheat aphid [Diuraphis noxia (Kurdjumov)] and other cereal aphids. In addition, volunteer plants can serve as a source of inoculum for rust (Puccinia sp.), Fusarium sp., or other diseases.
Management of Herbicide-Resistant Wheat Volunteers
There are several herbicide options to control HR or non-HR wheat volunteers pre-seeding, such as glyphosate or paraquat plus diuron (Beckie and Owen Reference Beckie and Owen2007; Rainbolt et al. Reference Rainbolt, Thill and Young2004). Some of the PRE herbicides have soil residual activity to control later-emerging seedlings. If wheat volunteers emerge after application of nonselective preplant herbicides, then in-crop herbicide control may be required depending upon their abundance. However, the largest cohort of wheat volunteers generally emerge before planting rotational crops and therefore can be managed by nonselective or selective PRE herbicides or tillage. Volunteers only need to be controlled in the following rotational crop, given the short seedbank persistence of wheat as described previously. Wheat volunteers, whether HR or non-HR, can be controlled easily in rotational broadleaf crops because of more herbicide options compared with cereal crops. Imidazolinone herbicides used in IR wheat will control CoAXium®/AXigen® or non-HR wheat volunteers. Conversely, quizalofop used in CoAXium®/AXigen® wheat will control IR or non-HR wheat volunteers. Successful pest (weeds, diseases, or insects) management necessitates avoiding back-to-back HR or non-HR wheat cultivation in a field. In Australia, where both IR wheat and barley are grown, careful crop rotation and herbicide planning are needed to control these cereal volunteers. Even with the introduction of two HR wheat production systems, their utility will constantly be diminished over time. Both herbicide SOAs (ACCase and ALS inhibitors) are considered high risk (≤10 applications) for the evolution of HR weeds (Beckie Reference Beckie2006). Grass weed species with multiple resistance to both herbicide SOAs (ACCase and ALS inhibitors) are becoming increasingly prevalent (Heap Reference Heap2020). Rigorous stewardship of both cultivation of HR wheat and effective management of volunteers in the following crop is required to maximize or prolong their economic benefits and minimize or reduce their economic costs.
Summary and Research Needs
HR crop volunteers can interfere with the crop grown in rotation and, depending on volunteer density, can reduce yield. Corn after soybean is a typical rotation in several states in the midwestern United States. Interference of volunteer corn in soybean is well documented and, depending on density of HR corn volunteers, can cause yield loss, if not controlled. ACCase- inhibiting herbicides are typically used for control of HR corn volunteers in soybean and other broadleaf crops grown in rotation. Corn volunteers resistant to FOPs would require cycohexanedione (DIM)-based herbicides for their control in soybean; however, Extension efforts are needed to convey this message to growers. In contrast, soybean volunteers do not generally persist, are poor competitors if overwintering, and are effectively controlled by labeled herbicides in corn. Similarly, cotton and sugarbeet volunteers are not a major issue in crops grown in rotation; however, cotton volunteers from stalk regrowth (ratooned) are difficult to control with herbicides and require a tillage operation. Barley and wheat volunteers are competitive weeds and, if not controlled, can result in significant yield loss in rotational crops. IR barley/wheat volunteers can make it more complicated for growers to find alternate herbicides for their management, particularly when an IR crop is grown in rotation. Hybrid rice has more potential for volunteerism compared with inbred rice; therefore, selection of the crop in rotation with hybrid rice is important. HR canola volunteers in broadleaf crops require a management plan by growers, because numerous seeds are returned to the soil seedbank due to high potential of pod shattering and harvest losses from harvesting equipment.
Growers are applying herbicides in mixture to expand the weed control spectrum, reduce the number of herbicide applications, and reduce selection pressure of a single SOA herbicide. Due to commercial cultivation of dicamba or 2,4-D–resistant soybean, management of HR corn volunteers is problematic for growers, because auxinic herbicides, which stimulate proton efflux, often antagonize ACCase-inhibiting herbicide (graminicide) efficacy. Therefore, there is a need to study the interaction of mixtures containing an ACCase inhibitor, glyphosate, and/or 2,4-D or dicamba for control of glyphosate/glufosinate-resistant corn volunteers in 2,4-D– or dicamba-resistant soybean and cotton. In addition, quizalofop is the only ACCase-inhibiting herbicide labeled for use in corn resistant to FOPs (i.e., Enlist™ corn) that can be used for control of corn volunteers from the previous year, assuming the hybrid corn planted the previous year was not resistant to the FOPs (i.e., Enlist™ corn). Therefore, research is needed to evaluate the interaction of quizalofop applied in a mixture with 2,4-D/glyphosate for control of volunteer corn in corn resistant to FOPs. Similarly, research is needed to investigate the interaction of DIMs with 2,4-D/glyphosate for control of FOP-resistant corn volunteers in 2,4-D–resistant soybean. It is known that herbicide rate, formulation, and inclusion of adjuvants can influence herbicide interactions; therefore, research is needed to explore these variables to overcome antagonism of mixing ACCase inhibitors and auxin herbicides for effective control of multiple HR corn volunteers.
Increased focus on developing multiple HR crops to manage HR weeds is slowing the development and adoption of nonchemical weed control methods and increases concern of interference by and management of multiple HR crop volunteers. Bringing diversity to crop and weed management strategies is an important consideration for effectively managing HR crop volunteers and minimizing their negative impact in agronomic cropping systems. Future weed management technologies, such as harvest weed seed control strategies, bioherbicides, robotic weeding, and herbicides based on RNAi technology, may augment the role of HR crops and use of herbicides for management of HR weeds and multiple HR crop volunteers. Harvest weed seed control has shown promising results for weed seed control in Australia (Walsh et al. Reference Walsh, Ouzman, Newman, Powles and Llewellyn2017) and the United States (Schwartz-Lazaro et al. Reference Schwartz-Lazaro, Norsworthy, Walsh and Bagavathinnan2017). There is a need to explore this technique for reducing seedbank input of HR crop volunteers. Machine vision technology has been developed to recognize crop row patterns and control automated devices that perform tasks such as removal of intrarow weeds (Fennimore et al. Reference Fennimore, Slaughter, Siemens, Leon and Saber2016). There is a need to explore this technology to distinguish crop from the volunteers to augment effective control of multiple HR crop volunteers.
Acknowledgments
This project was partially supported by the Nebraska Agricultural Experiment Station with funding from the Hatch Act through the U.S. Department of Agriculture (USDA) National Institute of Food and Agriculture Project No. NEB-22-396. This project was also supported by the USDA National Institute of Food and Agriculture’s Nebraska Extension Implementation Program. No conflicts of interest have been declared.