Introduction
Grain sorghum [Sorghum bicolor (L.) Moench] is the fifth most important crop grown in arid and semiarid regions worldwide (Visarada and Aruna Reference Visarada, Aruna, Aruna, Visarada, Bhat and Tonapi2019). It is a drought-tolerant crop that is productive in moisture-limited environments and is mainly used for food, feed, and fuel (Mundia et al. Reference Mundia, Secchi, Akamani and Wang2019). Grain sorghum is the fourth-largest crop after corn (Zea mays L.), soybean [Glycine max (L.) Merr.], and wheat (Triticum aestivum L.) in the United States (USDA-NASS 2023). In 2021, it was harvested from 2.63 million ha in the U.S., yielding 11,375 million kg of grain, with Kansas contributing 59% of this production (USDA-NASS 2023). Weeds are a serious production challenge in grain sorghum, and if weeds are not controlled in grain sorghum, U.S. farmers would lose approximately 5,700 million kg of grain sorghum annually valued at approximately US$953 million (Dille et al. Reference Dille, Stahlman, Thompson, Bean, Soltani and Sikkema2020). Moore et al. (Reference Moore, Murray and Westerman2004) reported that grain sorghum yield was reduced by 1.8% to 3.5% with one Palmer amaranth (Amaranthus palmeri S. Watson) plant in 15 m of sorghum row.
Amaranthus palmeri is the most troublesome broadleaf weed in grain sorghum in the United States (Van Wychen Reference Van Wychen2020). It is a summer annual C4 dioecious plant belonging to the Amaranthaceae family. It is native to the southwestern United States and northwestern Mexico and is widely distributed in several countries across Africa, Asia, Europe, North America, and South America (Roberts and Florentine Reference Roberts and Florentine2022; Sauer Reference Sauer1957). Amaranthus palmeri exhibits an extended emergence period, high photosynthetic rate, prolific seed production (up to 600,000 seeds per female plant), high genetic diversity within and among field populations, and ability to evolve herbicide resistance (Chahal et al. Reference Chahal, Aulakh, Jugulam and Jhala2015; Horak and Loughin Reference Horak and Loughin2000; Keeley et al. Reference Keeley, Carter and Thullen1987; Ward et al. Reference Ward, Webster and Steckel2013). Resistance to 10 different herbicide sites of action has been identified in populations of A. palmeri (WSSA Groups 2, 3, 4, 5, 6, 9, 10, 14, 15, and 27) (Heap Reference Heap2023). Multiple herbicide–resistant A. palmeri populations have been reported in the Central Great Plains (CGP) region, including Kansas (Chahal et al. Reference Chahal, Aulakh, Jugulam and Jhala2015; Heap Reference Heap2023; Jhala et al. Reference Jhala, Sandell, Rana, Kruger and Knezevic2014; Kumar et al. Reference Kumar, Liu, Boyer and Stahlman2019b, 2020a).
Several studies have demonstrated the importance of different management strategies that can impact A. palmeri emergence. For instance, Chahal et al. (Reference Chahal, Barnes and Jhala2021) reported that an early-season shallow tillage (10-cm depth) in the first week of June increased cumulative emergence of A. palmeri (1,674 plants m−2) in the fallow field as compared with mid-season (mid-June) and late-season (first week of July) tillage operations (533 to 869 plants m−2) in south-central Nebraska. DeVore et al. (Reference DeVore, Norsworthy and Brye2013) reported a 94% reduction of glyphosate-resistant (GR) A. palmeri emergence when cereal rye (Secale cereale L.) cover crop (CC) was used in combination with deep tillage (30-cm depth) before soybean compared with no deep tillage or cereal rye CC in Arkansas. Palhano et al. (Reference Palhano, Norsworthy and Barber2018) reported that cereal rye CC reduced A. palmeri emergence by 83% in cotton (Gossypium hirsutum L.) as compared with no CC in Arkansas. In a greenhouse study, Teasdale et al. (Reference Teasdale, Pillai and Collins2005) found that hairy vetch (Vicia villosa Roth.) residue (500 g m−2) and metolachlor (10 g ha−1) had a synergistic effect and reduced smooth pigweed (Amaranthus hybridus L.) emergence by 86% compared with no metolachlor or no hairy vetch residue. Perkins et al. (Reference Perkins, Gage, Norsworthy, Young, Bradley, Bish, Hager and Steckel2021) reported that a CC mixture of cereal rye and hairy vetch in combination with pyroxasulfone + flumioxazin, flumioxazin, pyroxasulfone, or acetochlor delayed A. palmeri emergence by 27 to 34 d in soybean compared with CC with no residual herbicides. Similarly, Liu et al. (Reference Liu, Kumar, Jha and Stahlman2022) documented a differential emergence pattern and periodicity among A. palmeri populations from Kansas, Nebraska, Colorado, Oklahoma, and Texas in a no-tillage (NT) field in western Kansas.
Winter wheat–sorghum–fallow (WSF) is a dominant crop rotation in the semiarid CGP region, where producers generally rely on postemergence herbicides for weed control during the fallow period (Holman et al. Reference Holman, Obour and Assefa2022; Kumar et al. Reference Kumar, Jha, Jugulam, Yadav and Stahlman2019a, Reference Kumar, Liu, Boyer and Stahlman2019b). In this 3-yr crop rotation, a fallow period of about 10 mo occurs after the winter wheat harvest and before grain sorghum planting in the following year (Kumar et al. Reference Kumar, Obour, Jha, Liu, Manuchehri, Dille, Holman and Stahlman2020b). During this fallow period, late-season infestation of A. palmeri in postharvest wheat stubble can produce a significant number of seeds (58,300 seeds m−2 if left uncontrolled), contributing to the soil seedbank for infestations in the subsequent summer crops, including grain sorghum (Kumar et al. Reference Kumar, Liu, Jhala, Jha and Manuchehri2021). In these NT-based cropping systems, the majority of A. palmeri seeds are concentrated on the soil surface and can emerge easily (Buhler Reference Buhler1992; Buhler et al. Reference Buhler, Mester and Kohler1996; Cardina et al. Reference Cardina, Regnier and Harrison1991; Oryokot et al. Reference Oryokot, Murphy and Swanton1997). Replacing a portion of this fallow period with a CC may provide effective weed suppression in NT cropping systems of the CGP region (Kumar et al. Reference Kumar, Obour, Jha, Liu, Manuchehri, Dille, Holman and Stahlman2020b). However, limited information exists on the combined effect of CC residue and residual herbicides on emergence of A. palmeri in NT grain sorghum in this dryland region. The main objective of this study was to determine the effect of fall-planted CC after wheat harvest and terminated in the following spring with glyphosate alone or glyphosate with acetochlor/atrazine on (1) emergence periodicity and (2) emergence pattern of GR A. palmeri in NT dryland grain sorghum.
Materials and Methods
Field Experiment
A field study was conducted at Kansas State University Agricultural Research Center near Hays (KSU-ARCH), KS (38.85196°N, 99.34279°W) from fall 2020 through fall 2023. The field site was under a NT dryland WSF rotation with a history of natural seedbank of GR A. palmeri. The 30-yr average annual precipitation at the study site was 596 mm, of which most occurred from May through September, and the average temperature ranged from −2 C in January to 26 C in July with a mean of 12 C (https://mesonet.k-state.edu). All three phases of the crop rotation (WSF) were present in each experimental year. The soil type at the experimental site was Roxbury silt loam (Taxonomic class: fine-silty, mixed, superactive, mesic Cumulic Haplustolls) with a pH of 6.9 and organic matter of 1.6%. A separate greenhouse study confirmed that an A. palmeri population from the study site survived 5,040 g ha−1 (4× the field use rate) of glyphosate (data not shown). Each year, a CC mixture of winter triticale (×Triticosecale Wittm. ex A. Camus [Secale × Triticum]) (60%)/winter peas (Pisum sativum L.) (30%)/rapeseed (Brassica napus L.) (5%)/radish (Raphanus sativus L.) (5%) was drilled at a seeding rate of 67 kg ha−1 in wheat stubble during fall (September/October) and terminated in the following spring at the triticale heading stage (Table 1). During each spring, four treatments were established: (1) nontreated control (where no CC was planted and no herbicides were applied to control weeds); (2) chemical fallow (where no CC was planted but the plot area was treated with glyphosate [Roundup PowerMax®, Bayer Crop Science, St. Louis, MO, USA] at 1,260 g ae ha−1 plus a premix of acetochlor/atrazine [Degree Xtra®, Bayer Crop Science, St. Louis, MO, USA] at 1,665/826 g ai ha−1 plus dicamba [Clarity®, BASF Corporation, Research Triangle Park, NC, USA] at 560 g ae ha−1 at the same time as CC termination); (3) CC terminated with glyphosate at 1,260 g ae ha−1; and (4) CC terminated with glyphosate at 1,260 g ae ha−1 plus a premix of acetochlor/atrazine at 1,665/826 g ai ha−1. Despite the presence of GR A. palmeri at the study site, glyphosate was used in chemical fallow to control winter annuals and some grass weeds such as tumble windmill grass (Chloris verticillata Nutt.) that growers typically apply in the region. In addition, glyphosate is a cost-effective and commonly used herbicide for CC termination in the region. Each year, treatments were arranged in a randomized complete block design with four replications. During the 2020 to 2021 experimental year, the nontreated control was not present, and there were only three treatments. The individual plot size was 45-m long and 13-m wide each year. During 2021 to 2022 and 2022 to 2023, the original chemical fallow plot was further divided into two to have both nontreated control and chemical fallow treatments (each plot 45-m long and 6.5-m wide). The CC was left standing after termination and no additional management practices were performed after termination. A grain sorghum hybrid ‘DKS 38-16’ was planted at a seeding rate of 114,855 seeds ha−1 in rows spaced 76 cm apart within 3 to 4 wk of CC termination each year. The details for planting and termination dates of CC as well as grain sorghum planting and harvesting dates for each experimental year are presented in Table 1. All local agronomic practices for grain sorghum production as recommended by Kansas State University were followed (Ciampitti et al. Reference Ciampitti, Diaz, Onofre, Lancaster, Whitworth and Aguilar2022).
Table 1. Planting and termination dates for cover crops and planting and harvesting dates for grain sorghum over three growing seasons at Kansas State University Agricultural Research Center near Hays, KS

Data Collection
Each year, the aboveground shoot biomass of CC was manually harvested from two 1-m2 quadrats from each plot just before CC termination and oven-dried at 72 C for 4 d to obtain dry biomass. Two permanent 1-m2 quadrats were established in each plot immediately after the termination of CC for GR A. palmeri emergence counts. Newly emerged GR A. palmeri seedlings from each permanent quadrat were counted when cotyledons were fully expanded and removed manually every week starting from termination of CC throughout the sorghum growing season (Hartzler et al. Reference Hartzler, Buhler and Stoltenberg1999). Kochia [Bassia scoparia (L.) A.J. Scott] and puncturevine (Tribulus terrestris L.) were also present each year and were removed manually along with GR A. palmeri every week. The end date for the emergence count of GR A. palmeri was chosen when no new emergence occurred over 21-d period each year. The average number of A. palmeri seedlings from the two permanent quadrats in each plot at each count timing was used in the data analysis. Data on daily minimum and maximum air temperature and precipitation during each growing season were obtained from the Kansas State University Mesonet weather station (https://mesonet.k-state.edu) located approximately 400 m away from the study site (38.8495°N, 99.3446°W). Growing degree days (GDD) and cumulative GDD (cGDD) were calculated (started at the day of CC termination) from the daily minimum and maximum air temperatures using Equations 1 and 2, respectively (McMaster and Wilhelm Reference McMaster and Wilhelm1997).


where GDDdaily is the daily accumulated GDD (it is a nonnegative value, therefore negative GDDdaily was replaced by 0), T max is the daily maximum air temperature (C), T min is the daily minimum air temperature (C), T base is the base temperature below which plant growth ceases and was considered as 10 C for A. palmeri (Norsworthy et al. Reference Norsworthy, Oliveira, Jha, Malik, Buckelew, Jennings and Monks2008), and n is the number of days in each growing season for which the emergence counts were recorded.
Statistical Analyses
Data on weekly GR A. palmeri emergence were subjected to ANOVA using the PROC MIXED procedure and were tested for homogeneity of variance and normality of the residuals using the PROC UNIVARIATE procedure in SAS v. 9.3 (SAS Institute, SAS Campus Drive, Cary, NC). Data were log-transformed to improve the normality of the residuals and homogeneity of variance; however, back-transformed data were presented with mean separation based on the transformed data. The fixed effects in the ANOVA model included treatment, year, weekly emergence timings, and their interactions. The random effects in the ANOVA model included replication and all interactions involving replication. Due to significant year by treatment interaction (P = 0.0321), data were analyzed separately for each year. The interaction between treatment and weekly emergence timing was significant (P < 0.001); therefore, data were sorted by weekly emergence timings using PROC SORT. Total emergence counts were calculated by adding all the weekly emergence counts from each treatment. Treatment means were separated using Fisher’s protected LSD test (P < 0.05) for each emergence timing.
The percent cumulative emergence of GR A. palmeri in each treatment from each replication was calculated as the sum of emergence on a sample date and all previous sample dates and expressed as a percentage of total emergence in each growing season (Jha and Norsworthy Reference Jha and Norsworthy2009). Data on percent cumulative emergence of GR A. palmeri from all four treatments were regressed against cGDD with a three-parameter log-logistic model using the drc package in R software (Knezevic et al. Reference Knezevic, Streibig and Ritz2007; Ritz and Streibig Reference Ritz and Streibig2005; Seefeldt et al. Reference Seefeldt, Jensen and Fuerst1995).

where Y is the percent cumulative emergence of GR A. palmeri; X is the cGDD; parameter d in the model is the maximum cumulative emergence, which was fixed to 100, because the percent cumulative emergence was based on the total emergence; E 50 is the cGDD required to reach 50% cumulative emergence; and the parameter b is the slope at the inflection point. The slope (b) represents the emergence rate of GR A. palmeri over cGDD, and the negative value of the slope indicates rapid emergence (Kumar et al. Reference Kumar, Jha, Dille and Stahlman2018). This model was selected based on Akaike’s criteria as described in Ritz and Spiess (Reference Ritz and Spiess2008). The P-value > 0.05 for the lack-of-fit test indicated that the model (Equation 3) adequately explained the percent cumulative emergence of GR A. palmeri (Ritz and Streibig Reference Ritz and Streibig2005). Parameter estimates, standard errors, and E 50 values for each treatment were determined. The coefficient of determination (R2), cGDD required for 10% (E 10) and 90% (E 90) cumulative emergence of GR A. palmeri and duration (cGDDs for 10% to 90% emergence, i.e., E 90 − E 10) for each treatment were predicted from the fitted model. E 10, E 50, and E 90 values were compared among treatments using the approximate t-test with the compParm and EDcomp functions in the drc package in R software (Knezevic et al. Reference Knezevic, Streibig and Ritz2007; Ritz et al. Reference Ritz, Baty, Streibig and Gerhard2015).
Results and Discussion
Variable amount and frequency of precipitation were observed at KSU-ARCH during the experimental periods from 2020 to 2023 (Figure 1). The total amount of precipitation received during the CC growing season (September to May) in 2020 to 2021, 2021 to 2022, and 2022 to 2023 was 217, 99, and 130 mm, respectively (Figure 1). The dry CC biomass at the time of termination was 1,520, 1,130, and 1,470 kg ha−1 in 2021, 2022, and 2023, respectively. Total precipitation amount during the grain sorghum growing seasons (June to October) in 2021, 2022, and 2023, was 256, 171, and 237 mm, respectively (Figure 1).

Figure 1. Daily minimum and maximum air temperature (C) and precipitation (mm) during the growing seasons of 2021 (A), 2022 (B), and 2023 (C).
Emergence Periodicity of GR Amaranthus palmeri
Amaranthus palmeri seedlings emerged from May 12 through September 15 in 2021, May 12 through July 14 in 2022, and June 2 through July 14 in 2023 (Figures 2 to 4). The prolonged emergence in 2021 might be due to relatively higher precipitation events (17) each of >10 mm from May to September as compared with the 2022 (9 events) and 2023 (10 events) growing seasons (Figure 1). Across 3 yr, the emergence initiation of GR A. palmeri was delayed by 0 to 21 d and 7 to 28 d under the CC terminated with glyphosate only and CC terminated with glyphosate plus acetochlor/atrazine, respectively, compared with chemical fallow (Figures 2 to 4). The CC terminated with glyphosate plus acetochlor/atrazine reduced the total emergence counts of GR A. palmeri by 42% to 56% compared with the chemical fallow and by 82% to 94% compared with the nontreated control across 3 yr (Figures 2 to 4).

Figure 2. Weekly emergence counts of glyphosate-resistant (GR) Amaranthus palmeri under different treatments in 2021. Chemical fallow had no cover crop (CC) and was treated with glyphosate + acetochlor/atrazine + dicamba at the same time as CC termination. CC+Gly indicates cover crop terminated with glyphosate; CC+Gly+(ACR+ATZ) indicates cover crop terminated with glyphosate plus acetochlor/atrazine. The same letter above columns within each timing are not significantly different according to Fisher’s protected LSD test at P < 0.05. Error bars represent standard error of the mean.
The 2021 Growing Season
The total emergence counts of GR A. palmeri at the end of the grain sorghum growing season were 22 (SE = 4), 23 (SE = 4), and 11 (SE = 3) seedlings m−2 under chemical fallow, CC terminated with glyphosate, and CC terminated with glyphosate plus acetochlor/atrazine, respectively (Figure 2). GR A. palmeri in chemical fallow started to emerge on May 12 with a major emergence observed from the end of May through the end of June (Figure 2). In contrast, both CC treatments had a GR A. palmeri emergence delayed by 21 to 28 d, with the first seedlings emerging between June 2 and June 9 and a major emergence occurring from early June to mid-July. Out of the total rainfall (358 mm), 64% occurred from mid-May to mid-July with 10 rainfall events of >10 mm that coincided with the major emergence period of GR A. palmeri (Figures 1 and 2). These results indicate that CC residue suppressed GR A. palmeri emergence and provided less initial weed competition to grain sorghum in 2021.
The 2022 Growing Season
The total emergence counts of GR A. palmeri at the end of the grain sorghum growing season were 33 (SE = 6), 12 (SE = 4), 31 (SE = 6), and 7 (SE = 2) seedlings m−2 under nontreated, chemical fallow, CC terminated with glyphosate, and CC terminated with glyphosate plus acetochlor/atrazine, respectively (Figure 3). Amaranthus palmeri seedlings started to emerge at nearly the same time (May 12 through May 19) under all treatments except CC terminated with glyphosate plus acetochlor/atrazine (delayed by 7 d) in the 2022 growing season (Figure 3). The CC dry biomass was relatively low (1,130 kg ha−1) at termination because of relatively low precipitation (99 mm) during the CC growing season; thus only CC without any residual herbicide was possibly not sufficient to suppress/delay the emergence of A. palmeri (Figures 1 and 3). Although GR A. palmeri seedlings emerged at nearly the same time, the total seasonal emergence counts of GR A. palmeri were reduced by 68% under chemical fallow, by 14% under CC terminated with glyphosate, and by 82% under CC terminated with glyphosate plus acetochlor/atrazine as compared with the nontreated control. Interestingly, both CC treatments significantly reduced early-season (May 12 through June 2) emergence of GR A. palmeri by 66% to 92% as compared with the nontreated control. Our results are consistent with those of Cornelius and Bradley (Reference Cornelius and Bradley2017), who also reported that cereal rye CC reduced early-season emergence of common waterhemp (Amaranthus rudis Sauer) by 35% compared with the nontreated control in soybean. The majority of the GR A. palmeri seedlings emerged from mid-May through early June under nontreated control and chemical fallow treatments. In contrast, the emergence of GR A. palmeri was mostly observed from late May through the end of June under CC terminated with glyphosate alone and from late May through early June under CC terminated with glyphosate plus acetochlor/atrazine (Figure 3). The CC residue on the soil surface could reduce the soil temperature, altering the quantity and quality of light reaching the soil surface (Teasdale Reference Teasdale and Zimdahl2018; Weisberger et al. Reference Weisberger, Leon, Gruner, Levi, Gaur, Morgan and Basinger2024), which might have delayed the emergence of GR A. palmeri under CC treatments. Furthermore, all the major emergence periods of GR A. palmeri in May and June coincided with the precipitation events, with 60% of the total seasonal rainfall occurring during these 2 mo (Figure 1). Similar observations were also reported by Liu et al. (Reference Liu, Kumar, Jha and Stahlman2022) and Jha and Norsworthy (Reference Jha and Norsworthy2009), who found that peak A. palmeri emergence coincided with precipitation events. These findings are consistent with Wiggins et al. (Reference Wiggins, McClure, Hayes and Steckel2015), who concluded that CC alone was not sufficient for season-long control of GR A. palmeri and suggested integrating residual herbicides to complement the suppressive effect of CC.
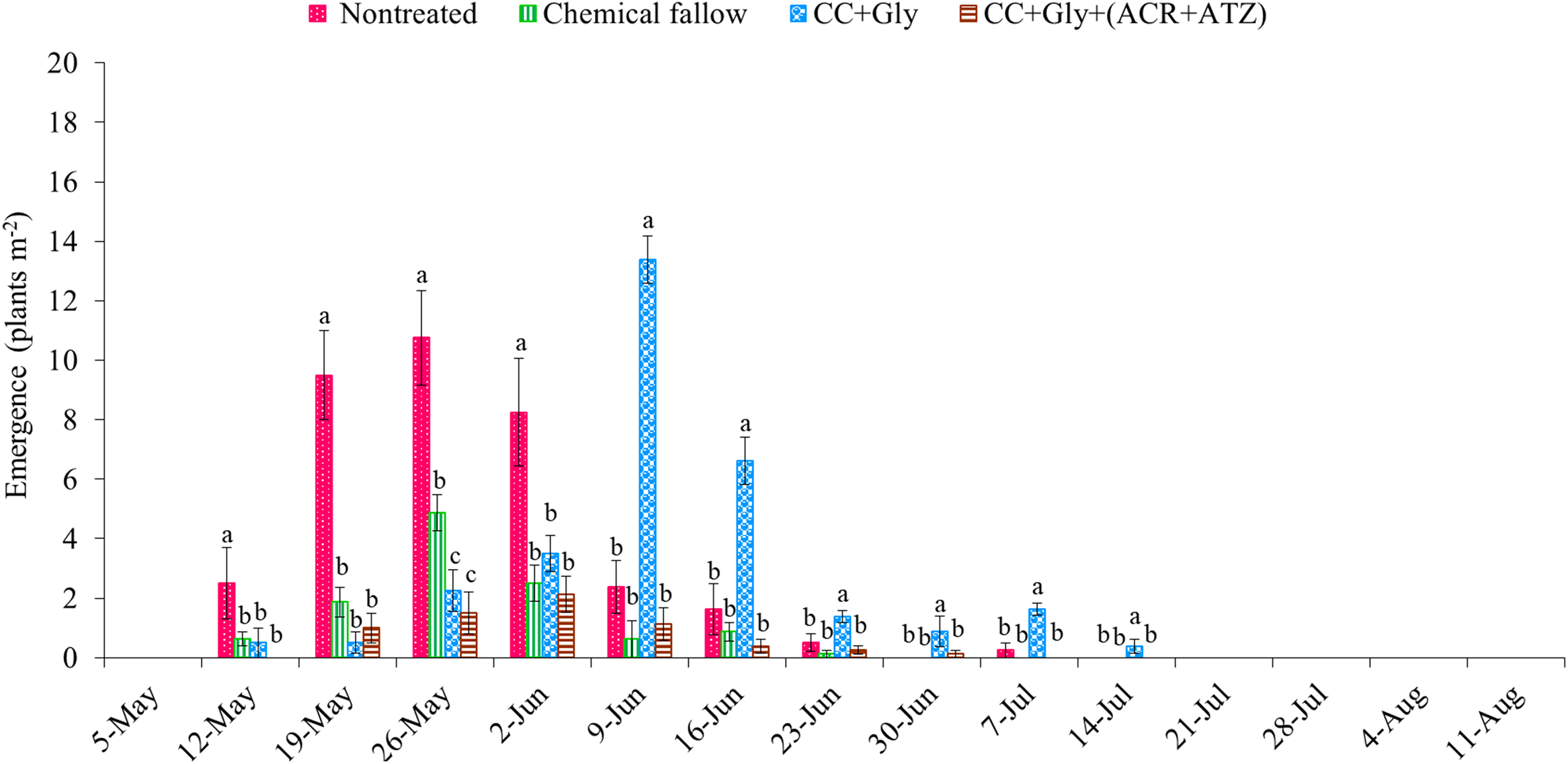
Figure 3. Weekly emergence counts of glyphosate-resistant (GR) Amaranthus palmeri under different treatments in 2022. Chemical fallow had no cover crop (CC) and was treated with glyphosate + acetochlor/atrazine + dicamba at the same time as CC termination. CC+Gly indicates cover crop terminated with glyphosate; CC+Gly+(ACR+ATZ) indicates cover crop terminated with glyphosate plus acetochlor/atrazine. The same letter above columns within each timing are not significantly different according to Fisher’s protected LSD test at P < 0.05. Error bars represent standard error of the mean.
The 2023 Growing Season
The total emergence counts of GR A. palmeri at the end of the grain sorghum growing season were 60 (SE = 8), 9 (SE = 2), 29 (SE = 6), and 4 (SE = 1) seedlings m−2 under nontreated, chemical fallow, CC terminated with glyphosate, and CC terminated with glyphosate plus acetochlor/atrazine, respectively (Figure 4). The GR A. palmeri seedlings started to emerge on June 2 in the nontreated control and on June 9 in chemical fallow and CC terminated with glyphosate alone (Figure 4). In contrast, the emergence of GR A. palmeri in CC terminated with glyphosate plus acetochlor/atrazine was delayed and was first observed on June 30, indicating the emergence was delayed by 28 d as compared with the nontreated control and by 21 d compared with chemical fallow (Figure 4). This might be due to the synergistic effect of CC and residual herbicide, as also reported by previous researchers (Perkins et al. Reference Perkins, Gage, Norsworthy, Young, Bradley, Bish, Hager and Steckel2021; Teasdale et al. Reference Teasdale, Pillai and Collins2005). Perkins et al. (Reference Perkins, Gage, Norsworthy, Young, Bradley, Bish, Hager and Steckel2021) reported that a CC mixture of cereal rye and hairy vetch in combination with pyroxasulfone + flumioxazin, flumioxazin, pyroxasulfone, or acetochlor delayed A. palmeri emergence by 27 to 34 d in soybean compared with CC with no residual herbicides. The majority of the GR A. palmeri seedlings emerged from June 2 through June 30 in the nontreated control, June 9 through July 7 in chemical fallow, June 9 through July 14 in CC terminated with glyphosate alone, and June 30 through July 7 in CC terminated with glyphosate plus acetochlor/atrazine. Most of the GR A. palmeri seedlings emerged in June and July, which coincided with eight major precipitation events (each event >5 mm) and two events of >20 mm contributing 44% of the total season rainfall (312 mm) in 2023 (Figures 1 and 4). The total seasonal emergence counts of GR A. palmeri were reduced by 85% in chemical fallow, 51% in CC terminated with glyphosate alone, and 94% in CC terminated with glyphosate plus acetochlor/atrazine as compared with the nontreated control (Figure 4). These results agree with those of DeVore et al. (Reference DeVore, Norsworthy and Brye2012), who reported that fall-planted cereal rye CC reduced the emergence of GR A. palmeri by 67% in cotton compared with no CC. Similarly, McCall (Reference McCall2018) observed a delayed emergence of GR A. palmeri under winter wheat, spring oat (Avena sativa L.), or spring oat/spring pea CC terminated with glyphosate plus flumioxazin/pyroxasulfone as compared with CC terminated with glyphosate only.

Figure 4. Weekly emergence counts of glyphosate-resistant (GR) Amaranthus palmeri under different treatments in 2023. Chemical fallow had no cover crop (CC) and was treated with glyphosate + acetochlor/atrazine + dicamba at the same time as CC termination. CC+Gly indicates cover crop terminated with glyphosate; CC+Gly+(ACR+ATZ) indicates cover crop terminated with glyphosate plus acetochlor/atrazine. The same letter above columns within each timing are not significantly different according to Fisher’s protected LSD test at P < 0.05. Error bars represent standard error of the mean.
Emergence Pattern of GR Amaranthus palmeri
Across 3 yr, 66 to 643 and 105 to 1,257 more cGDD were required to achieve 90% cumulative emergence of GR A. palmeri under the CC terminated with glyphosate alone and CC terminated with glyphosate plus acetochlor/atrazine, respectively, compared with chemical fallow (Table 2; Figure 5).
Table 2. Regression parameters estimated from the log-logistic model (Equation 3) for percent cumulative emergence of glyphosate-resistant (GR) Amaranthus palmeri, duration of A. palmeri emergence, and coefficient of determination (R2) during the 2021 to 2023 growing seasons at Kansas State University Agricultural Research Center near Hays, KS

a Chemical fallow had no cover crop (CC) and was treated with glyphosate + acetochlor/atrazine + dicamba at the same time as CC termination; CC+Gly indicates cover crop terminated with glyphosate; CC+Gly+(ACR+ATZ) indicates cover crop terminated with glyphosate plus acetochlor/atrazine.
b Regression parameters: b is the slope at the inflection point of each curve; E 10, E 50, and E 90 are cumulative GDD (cGDD) required for 10%, 50%, and 90% cumulative emergence of GR A. palmeri, respectively; SE is the standard error of mean. E 10, E 50, and E 90 estimates followed by the same letter are not different based on an approximate t-test using the CompParm and EDcomp functions in the DRC package in R software (Ritz et al. Reference Ritz, Baty, Streibig and Gerhard2015).
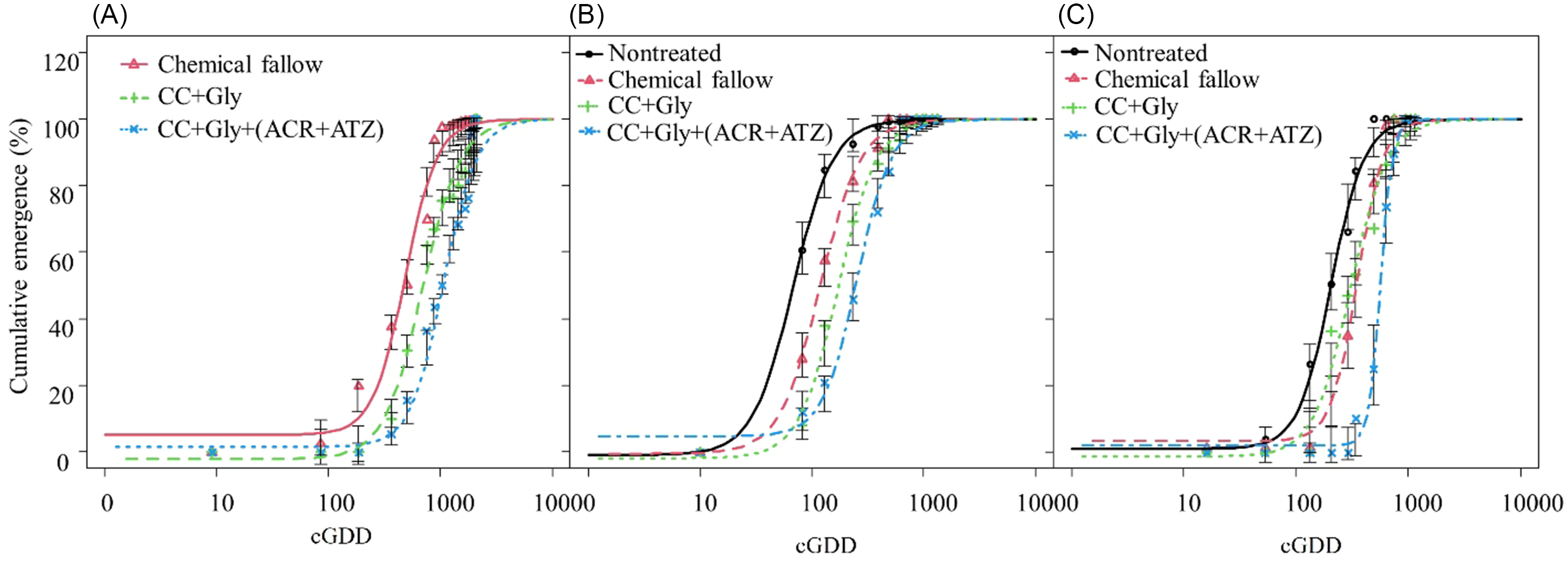
Figure 5. Cumulative emergence of glyphosate-resistant (GR) Amaranthus palmeri under different treatments during 2021 (A), 2022 (B), and 2023 (C) growing seasons in relation to cumulative growing degree days (cGDD). Chemical fallow had no cover crop (CC) and was treated with glyphosate + acetochlor/atrazine + dicamba at the same time as CC termination. CC+Gly indicates cover crop terminated with glyphosate; CC+Gly+(ACR+ATZ) indicates cover crop terminated with glyphosate plus acetochlor/atrazine. Vertical bars indicate ± standard error of the mean at each observation date. Model is based on Equation 3 with parameter estimates in Table 2.
The 2021 Growing Season
Only 230 to 286 cGDD were required for 10% cumulative emergence (E 10 value) of GR A. palmeri under chemical fallow and CC terminated with glyphosate alone, whereas about 498 cGDD were required to achieve 10% cumulative emergence of GR A. palmeri under CC terminated with glyphosate plus acetochlor/atrazine (Table 2). Similarly, about 205 and 580 more cGDD were required to achieve 50% cumulative emergence (E 50 values) of GR A. palmeri for CC terminated with glyphosate and CC terminated with glyphosate plus acetochlor/atrazine, respectively, as compared with chemical fallow. For 90% cumulative emergence (E 90 value) of GR A. palmeri, about 1,000, 1,643, and 2,257 cGDD were required for chemical fallow, CC terminated with glyphosate, and CC terminated with glyphosate plus acetochlor/atrazine, respectively (Table 2; Figure 5). The CC terminated with glyphosate or with glyphosate plus acetochlor/atrazine exhibited a prolonged emergence duration (E 90 − E 10) of GR A. palmeri and required an additional 587 and 989 cGDD, respectively, as compared with chemical fallow. The greater cGDD requirements for 10%, 50%, and 90% cumulative emergence of GR A. palmeri under CC treatments might be due to alteration in the soil microenvironment with decreasing light penetration and soil temperature as compared with no CC treatments (Teasdale and Mohler Reference Teasdale and Mohler1993). Furthermore, the CC residue on the soil surface could reduce evaporation, thereby contributing to increased soil water storage (Holman et al. Reference Holman, Assefa and Obour2020), which could possibly benefit A. palmeri for its longer emergence period. Our results are consistent with those of Perkins et al. (Reference Perkins, Gage, Norsworthy, Young, Bradley, Bish, Hager and Steckel2021), who previously reported that applications of pyroxasulfone + flumioxazin, flumioxazin, pyroxasulfone, or acetochlor at 3 wk after fall-planted cereal rye + hairy vetch CC termination delayed A. palmeri emergence by 27 to 34 d compared with CC termination with glyphosate + dicamba in soybean.
The 2022 Growing Season
GR A. palmeri seedlings emerged quickly in the nontreated control and required only 27 cGDD to achieve 10% cumulative emergence in the absence of CC and residual herbicide (Table 2; Figure 5). In contrast, GR A. palmeri under the chemical fallow treatment required 47 cGDD for 10% cumulative emergence followed by CC terminated with glyphosate alone (70 cGDD), and CC terminated with glyphosate plus acetochlor/atrazine (116 cGDD) (Table 2; Figure 5). To reach 50% cumulative emergence, the CC terminated with glyphosate alone required 103 and 52 more cGDD compared with nontreated and chemical fallow, respectively. Similarly, the CC terminated with glyphosate plus acetochlor/atrazine required 187 and 136 additional cGDD to achieve 50% cumulative emergence of GR A. palmeri compared with nontreated and chemical fallow, respectively. Furthermore, to reach 90% cumulative emergence, the CC terminated with glyphosate plus acetochlor/atrazine required 557 cGDD and had a prolonged period of emergence (E 90 − E 10 = 441 cGDD). In contrast, the nontreated control required only 168 cGDD to achieve 90% cumulative emergence of GR A. palmeri and had a short period of emergence (E 90 − E 10 = 141 cGDD) (Table 2). These results are consistent with those of McCall (Reference McCall2018), who reported that an additional 80 cGDD were required to reach 50% emergence of GR A. palmeri under winter wheat CC terminated with glyphosate plus flumioxazin/pyroxasulfone as compared with CC terminated with glyphosate only in soybean.
The 2023 Growing Season
Only 97 cGDD were required to achieve 10% cumulative emergence of GR A. palmeri in the nontreated control. Compared with the 2021 and 2022 growing seasons, the CC terminated with glyphosate alone required fewer cGDD (132) than chemical fallow (192) to achieve 10% cumulative emergence of GR A. palmeri (Table 2; Figure 5). This might be because of frequent precipitation events during late May and early June. Among all treatments, CC terminated with glyphosate plus acetochlor/atrazine required the greatest cGDD (427) for 10% cumulative emergence of GR A. palmeri (Table 2; Figure 5). In the nontreated control, GR A. palmeri required 204 cGDD for 50% cumulative emergence, while CC terminated with glyphosate plus acetochlor/atrazine required significantly higher cGDD (562) (Table 2; Figure 5). Similarly, to achieve 90% cumulative emergence of GR A. palmeri, the highest cGDD (739) were required under the CC terminated with glyphosate plus acetochlor/atrazine, while only 429 cGDD were required under nontreated control. These results are consistent with those of Teasdale et al. (Reference Teasdale, Shelton, Sadeghi and Isensee2003), who previously reported that hairy vetch residue and preemergence application of metolachlor delayed weed emergence by 7 to 10 d compared with herbicide alone. The delay in emergence of GR A. palmeri would provide an initial competitive benefit to grain sorghum.
Overall, the results from this study indicate that fall-planted CC after winter wheat harvest and terminated in the following spring with glyphosate plus acetochlor/atrazine can delay and reduce the cumulative emergence of GR A. palmeri in grain sorghum. Nevertheless, these results emphasize the need for a season-long integrated A. palmeri management strategy in grain sorghum. Previous studies have also established that adequate CC biomass is highly crucial to achieve suppression and delayed emergence of A. palmeri (Devore et al. Reference DeVore, Norsworthy and Brye2013; Palhano et al. Reference Palhano, Norsworthy and Barber2018; Perkins et al. Reference Perkins, Gage, Norsworthy, Young, Bradley, Bish, Hager and Steckel2021), and infrequent rainfall patterns are a major constraint to the widescale adoption of CC in the NT dryland CGP region. As evident from this research, limited precipitation during winter months in some years can result in low CC biomass accumulation and inactivation of residual herbicides, resulting into loss of suppressive effect of CC and residual herbicide on A. palmeri emergence. Considering these challenges with CC plus residual herbicides and rapid evolution of A. palmeri with multiple resistance to postemergence herbicides, additional novel weed control strategies (such as electric weeders and harvest weed seed control [chaff lining and seed impact mill]) should also be integrated for managing GR A. palmeri seedbanks in this NT dryland region.
Different A. palmeri populations within a CGP region could exhibit a differential emergence pattern, as previously observed by Liu et al. (Reference Liu, Kumar, Jha and Stahlman2022). Therefore, the findings of the current study will help to further improve the prediction models for A. palmeri emergence under CC plus residual herbicide strategy to build decision support tools for cost-effective and sustainable management of GR A. palmeri in the region. These combined practices of CC and residual herbicides would also help growers to target smaller GR A. palmeri seedings with postemergence herbicides for effective in-season control. Fewer and late-emerging GR A. palmeri plants would have lower biomass accumulation, and ultimately lower seed production, which can further help to prevent replenishment of the soil seedbank. Although this study investigated only one residual herbicide, sorghum producers should diversify their residual herbicide programs annually to prevent the selection of A. palmeri populations with resistance to residual herbicides. Future studies should investigate weed suppression with CC plus residual herbicides by considering the soil water budget and subsequent crop yield implications in the WSF rotation. Furthermore, based on available soil moisture, the fallow phase after grain sorghum harvest in the WSF rotation could also be replaced with the spring-planted CC such as barley (Hordeum vulgare L.), oat, or pea for summer annual weed suppression (Obour et al. Reference Obour, Dille, Holman, Simon, Sancewich and Kumar2022).
Acknowledgments
We thank Taylor Lambert and Matthew Vredenburg for their assistance in conducting the field study.
Funding
The funding from the NC SARE Graduate Student Grant (GNC22-346) supported this work.
Competing Interests
The authors declare no competing interests.