Traditionally, research into addictive processes and their treatment has focused on the mesolimbic dopaminergic reward system. Recently, however, interest in the potentially important role of the prefrontal cortex has increased; specifically, the orbitofrontal cortex is frequently implicated. This region is critically involved in inhibitory decision-making processes, especially in reward-related behaviours. It processes the reward value and/or affective valence of environmental stimuli, assesses the future consequences of the individual's own actions (response selection) and inhibits appropriate behaviours (response inhibition; Bechara & Damasio, Reference Bechara and Damasio2002; Reference KrawczykKrawczyk, 2002; Reference Fan, Flombaum and McCandlissFan et al, 2003). Thus, the decision-making function of the orbitofrontal cortex is suggested to be closely related to the well-known addiction processes of craving, salience, continued drug use despite harmful consequences and relapse (Reference Goldstein and VolkowGoldstein & Volkow, 2002; Reference Lubman, Yucel and PantelisLubman et al, 2004). We explore the evidence supporting this hypothesis, integrating data from both behavioural decision-making and neuroimaging studies of participants with substance use disorders.
METHOD
We consulted the US National Library of Medicine (Medline) to identify studies conducted between January 1990 and May 2004. Only original research articles including study populations with substance use disorders were considered. Postmortem studies and studies focusing on tobacco-smoking or gambling only were excluded.
The keywords used to search the database were SUBSTANCE ABUSE or DEPENDENCE or DRUG ADDICTION, CRAVING or DRUG CRAVING, DECISION MAKING, NEUROIMAGING or FUNCTIONAL IMAGING, ORBITOFRONTAL CORTEX or VENTROMEDIAL PREFRONTAL CORTEX, GAMBLING TASK or DECISION-MAKING TASK. Combinations of these search terms yielded 43 articles. Bibliographies were examined to identify further citations. Imaging studies were included if they used conservative statistical thresholding methods (e.g. corrections for multiple comparisons). Imaging studies in which the orbitofrontal cortex was not explicitly incorporated in the study design or in which the cue-presentation design might have been confounded by habituation effects (e.g. repetitive, long-presentation stimuli) were excluded. Three studies were excluded (Reference Maas, Lukas and KaufmanMaas et al, 1998; Reference Schneider, Habel and WagnerSchneider et al, 2001; Reference Wrase, Gruússer and KleinWrase et al, 2002). Ultimately 52 studies were included in this review: 11 behavioural task studies and 41 neuroimaging studies.
RESULTS
Behavioural laboratory task studies
The main results of the studies using behavioural decision-making tasks are shown in Table 1. One study used the Rogers Cambridge Gamble Task (RCGT; Reference Rogers, Everitt and BaldacchinoRogers et al, 1999a ) and ten studies used the Iowa Gambling Task (IGT; Reference Bechara, Damasio and DamasioBechara et al, 1994). In the RCGT, participants make a simple probabilistic judgement between two mutually exclusive outcomes, and subsequently place a bet based on their confidence in that decision. The involvement of the orbitofrontal cortex – and the anterior cingulate cortex – in performance on the Risk Task (an adaptation of the RCGT for use in functional imaging) was demonstrated in a positron emission tomography (PET) study (Reference Rogers, Owen and MiddletonRogers et al, 1999b ). The only RCGT study demonstrated decision-making deficits in both individuals on amphetamines and dependent on heroin, compared with controls (Reference Rogers, Everitt and BaldacchinoRogers et al, 1999a ).
Table 1 Main results from studies using behavioural decision-making tasks

Study | Substance misuse sample | Control group | Abstinence | Method | Decision-making deficits |
---|---|---|---|---|---|
Petry et al (Reference Petry, Bickel and Arnett1998) | Heroin (out-patients: 18 men, 14 women) | 33 men, 26 women | Buprenorphine maintenance | IGT | Yes |
Rogers et al (Reference Rogers, Everitt and Baldacchino1999a ) | Amphetamines (14 men, 4 women) Opiates (13 men) | 16 men, 10 women | 12 h | RCGT | Yes |
Grant et al (Reference Grant, Contoreggi and London2000) | Polydrug misuse (27 men, 3 women) | 18 men, 4 women | 36–38 h | IGT | Yes |
Mazas et al (Reference Mazas, Finn and Steinmetz2000) | Early-onset alcoholism (15 men, 12 women) | Control group (14 men, 18 women) ASP group (6 men, 2 women) | Sober at testing | IGT | Yes |
Bechara et al (Reference Bechara, Dolan and Denberg2001) | Substance dependency (21 men, 20 women) | 20 men, 20 women | > 15 days | IGT | Yes |
Petry (Reference Petry2001) | Substance use disorder (63 men) | 21 men | 0 day | IGT | Yes |
Bechara & Damasio (Reference Bechara and Damasio2002) | Substance dependency (21 men, 25 women) | 21 men, 28 women | > 15 days | IGT+SCR | Yes |
Bechara et al (Reference Bechara, Dolan and Hindes2002) | VMPFC lesions (5 men, 5 women) | Variant version | Yes | ||
IGT+SCR | |||||
Mintzer & Stitzer (Reference Mintzer and Stitzer2002) | Opioid-dependent MMT (7 men, 11 women) | 10 men, 11 women | 24 h MMT | IGT | Yes |
Ernst et al (Reference Ernst, Grant and London2003) | Same group as in Grant et al (Reference Grant, Contoreggi and London2000) | IGT | Yes | ||
Rotheram-Fuller et al (Reference Rotheram-Fuller, Shoptaw and Berman2004) | MMT+tobacco smokers (9) | Smokers (9) | MMT | IGT | Yes |
MMT non-smokers (9) | Non-smokers (10) |
The Iowa Gambling Task was developed to assess decision-making in patients with ventromedial lesions. The task's essential feature is that it mimics real-life situations in the way that it factors uncertainty, reward and punishment (Bechara et al, Reference Bechara, Damasio and Damasio1994, Reference Bechara, Damasio and Damasio2000) and emphasises the contribution of emotional processing to decision-making. To earn ‘pretend’ money, participants need to learn the associations between reward and punishment of four card decks. In all ten IGT studies, participants with substance use disorders performed worse than the controls. The studies that also included patients with ventromedial lesions showed that, compared with the control groups, a higher proportion of the participants with substance use disorders performed within the range of the patients with lesions (Reference Rogers, Everitt and BaldacchinoRogers et al, 1999a ; Reference Bechara and DamasioBechara & Damasio, 2002; Bechara et al, Reference Bechara, Dolan and Denberg2001, Reference Bechara, Dolan and Hindes2002). Taken together, the studies consistently demonstrate impaired decision-making in patients with substance use disorders relative to controls.
Neuroimaging studies
Imaging studies during decision-making
The studies using functional imaging during decision-making are summarised in Table 2. Fundamental to this review is the (only) PET study during IGT and a neutral control task (Reference Bolla, Eldreth and LondonBolla et al, 2003). Performance on the IGT within both a group of cocaine users and a control group was positively correlated with activation in the right medial orbitofrontal cortex region. Between-group comparison showed greater metabolic activity of this region during the IGT in the cocaine user group. This may reflect an effort to compensate for the intrinsically weaker performance of the orbitofrontal cortex in the cocaine group or an effort to compensate for weaker performance of other regions involved in decision-making, such as the dorsolateral prefrontal cortex. Although the cocaine user group's performance on the IGT was inferior to that of the controls, the difference was not significant, possibly owing to the small sample size.
Table 2 Functional imaging during decision-making tasks

Study | Main substance of misuse (sample) | Treatment | Control group | Abstinence | Method | OFC activation | Other regions activated |
---|---|---|---|---|---|---|---|
Paulus et al (Reference Paulus, Hozack and Zauscher2002) | Methamphetamine (10 men)1 | In-patient | 10 men | 22.4 days | TCPT, TCRT+fMRI | Yes | Sample group showed less task-related activity in the VMPFC and right OFC region |
Bolla et al (Reference Bolla, Eldreth and London2003) | Cocaine (10 men, 3 women) | 10 men, 3 women | 25 days | PET (FDG) during IGT | Yes | Larger activation in the right OFC and less in the DLPFC in the cocaine group | |
Performance on the IGT correlated with activation of right medial OFC | |||||||
Paulus et al (Reference Paulus, Hozack and Frank2003) | Methamphetamine (14 men) | In-patient | 10 men, 4 women | 25 days | TCPT, TCRT+fMRI | Yes | Methamphetamine-dependent patients showed less task-related activation in the OFC (BA 10), DLPFC (BA 9), ACC (BA 32) and parietal cortex (BA 7) |
In a functional magnetic resonance imaging (fMRI) study, Paulus et al (Reference Paulus, Hozack and Zauscher2002, Reference Paulus, Hozack and Frank2003) used an experimental decision-making task, a two-choice prediction task, in which participation did not know a priori which action was associated with the best outcome, and a neutral control task (two-choice response task). Compared with controls, participants dependent on methamphetamine showed less task-related activation in the orbitofrontal cortex (Brodmann areas (BA) 10 and 11), the dorsolateral prefrontal cortex (BA 9) and the anterior cingulate cortex (BA 32) during the prediction task relative to the response task. This suggests a dysfunction of the orbitofrontal cortex, which is elicited primarily in choices associated with uncertain outcomes.
Imaging studies of cue reactivity
Twenty neuroimaging studies used a cue exposure or drug priming paradigm to evoke cue reactivity. The findings, ordered by substance, are presented in Table 3. The results are conflicting. Thirteen studies demonstrated activation of the orbitofrontal cortex in response to cue exposure (‘cue reactivity’), whereas six did not. However, several other areas were activated: the dorsolateral prefrontal cortex, amygdala, insular cortices, anterior cingulate cortex and cerebellum. One study showed cue reactivity of the orbitofrontal cortex in male patients addicted to cocaine but not in similarly addicted female patients (Reference Kilts, Gross and ElyKilts et al, 2004). Seventeen studies included craving measures: two of these studies reported no craving after exposure, whereas six reported an association between the intensity of drug craving or drug ‘high’ and orbitofrontal cortex activation. In the remaining nine studies craving was associated with other regions.
Table 3 Neuroimaging studies of cue reactivity
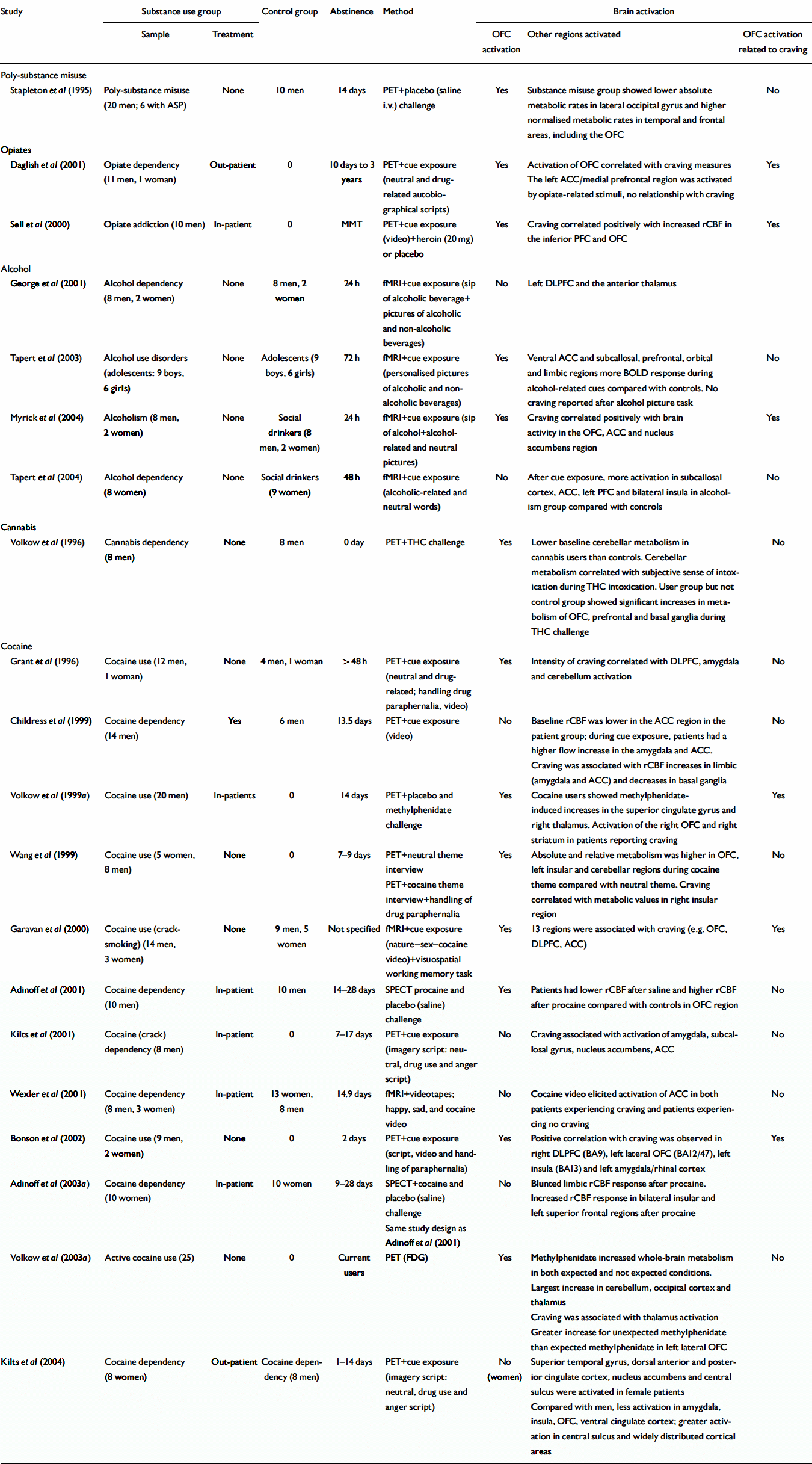
Study | Substance use group | Control group | Abstinence | Method | Brain activation | |||
---|---|---|---|---|---|---|---|---|
Sample | Treatment | OFC activation | Other regions activated | OFC activation related to craving | ||||
Poly-substance misuse | ||||||||
Stapleton et al (Reference Stapleton, Morgan and Phillips1995) | Poly-substance misuse (20 men; 6 with ASP) | None | 10 men | 14 days | PET+placebo (saline i.v.) challenge | Yes | Substance misuse group showed lower absolute metabolic rates in lateral occipital gyrus and higher normalised metabolic rates in temporal and frontal areas, including the OFC | No |
Opiates | ||||||||
Daglish et al (Reference Daglish, Weinstein and Malizia2001) | Opiate dependency (11 men, 1 woman) | Out-patient | 0 | 10 days to 3 years | PET+cue exposure (neutral and drug-related autobiographical scripts) | Yes | Activation of OFC correlated with craving measures The left ACC/medial prefrontal region was activated by opiate-related stimuli, no relationship with craving | Yes |
Sell et al (Reference Sell, Morris and Bearn2000) | Opiate addiction (10 men) | In-patient | 0 | MMT | PET+cue exposure (video)+heroin (20 mg) or placebo | Yes | Craving correlated positively with increased rCBF in the inferior PFC and OFC | Yes |
Alcohol | ||||||||
George et al (Reference George, Anton and Bloomer2001) | Alcohol dependency (8 men, 2 women) | None | 8 men, 2 women | 24 h | fMRI+cue exposure (sip of alcoholic beverage+pictures of alcoholic and non-alcoholic beverages) | No | Left DLPFC and the anterior thalamus | |
Tapert et al (Reference Tapert, Cheung and Brown2003) | Alcohol use disorders (adolescents: 9 boys, 6 girls) | None | Adolescents (9 boys, 6 girls) | 72 h | fMRI+cue exposure (personalised pictures of alcoholic and non-alcoholic beverages) | Yes | Ventral ACC and subcallosal, prefrontal, orbital and limbic regions more BOLD response during alcohol-related cues compared with controls. No craving reported after alcohol picture task | No |
Myrick et al (Reference Myrick, Anton and Li2004) | Alcoholism (8 men, 2 women) | None | Social drinkers (8 men, 2 women) | 24 h | fMRI+cue exposure (sip of alcohol+alcohol-related and neutral pictures) | Yes | Craving correlated positively with brain activity in the OFC, ACC and nucleus accumbens region | Yes |
Tapert et al (Reference Tapert, Brown and Baratta2004) | Alcohol dependency (8 women) | None | Social drinkers (9 women) | 48 h | fMRI+cue exposure (alcoholic-related and neutral words) | No | After cue exposure, more activation in subcallosal cortex, ACC, left PFC and bilateral insula in alcoholism group compared with controls | No |
Cannabis | ||||||||
Volkow et al (Reference Volkow, Gillespie and Mullani1996) | Cannabis dependency (8 men) | None | 8 men | 0 day | PET+THC challenge | Yes | Lower baseline cerebellar metabolism in cannabis users than controls. Cerebellar metabolism correlated with subjective sense of intoxication during THC intoxication. User group but not control group showed significant increases in metabolism of OFC, prefrontal and basal ganglia during THC challenge | No |
Cocaine | ||||||||
Grant et al (Reference Grant, London and Newlin1996) | Cocaine use (12 men, 1 woman) | None | 4 men, 1 woman | >48 h | PET+cue exposure (neutral and drug-related; handling drug paraphernalia, video) | Yes | Intensity of craving correlated with DLPFC, amygdala and cerebellum activation | No |
Childress et al (Reference Childress, Mozley and McElgin1999) | Cocaine dependency (14 men) | Yes | 6 men | 13.5 days | PET+cue exposure (video) | No | Baseline rCBF was lower in the ACC region in the patient group; during cue exposure, patients had a higher flow increase in the amygdala and ACC. Craving was associated with rCBF increases in limbic (amygdala and ACC) and decreases in basal ganglia | No |
Volkow et al (Reference Volkow, Wang and Fowler1999a ) | Cocaine use (20 men) | In-patients | 0 | 14 days | PET+placebo and methylphenidate challenge | Yes | Cocaine users showed methylphenidate-induced increases in the superior cingulate gyrus and right thalamus. Activation of the right OFC and right striatum in patients reporting craving | Yes |
Wang et al (Reference Wang, Volkow and Fowler1999) | Cocaine use (5 women, 8 men) | None | 0 | 7–9 days | PET+neutral theme interview PET+cocaine theme interview+handling of drug paraphernalia | Yes | Absolute and relative metabolism was higher in OFC, left insular and cerebellar regions during cocaine theme compared with neutral theme. Craving correlated with metabolic values in right insular region | No |
Garavan et al (Reference Garavan, Pankiewicz and Bloom2000) | Cocaine use (crack-smoking) (14 men, 3 women) | None | 9 men, 5 women | Not specified | fMRI+cue exposure (nature – sex – cocaine video)+visuospatial working memory task | Yes | 13 regions were associated with craving (e.g. OFC, DLPFC, ACC) | Yes |
Adinoff et al (Reference Adinoff, Devous and Best2001) | Cocaine dependency (10 men) | In-patient | 10 men | 14–28 days | SPECT procaine and placebo (saline) challenge | Yes | Patients had lower rCBF after saline and higher rCBF after procaine compared with controls in OFC region | No |
Kilts et al (Reference Kilts, Schweitzer and Quinn2001) | Cocaine (crack) dependency (8 men) | In-patient | 0 | 7–17 days | PET+cue exposure (imagery script: neutral, drug use and anger script) | No | Craving associated with activation of amygdala, subcallosal gyrus, nucleus accumbens, ACC | No |
Wexler et al (Reference Wexler, Gottschalk and Fulbright2001) | Cocaine dependency (8 men, 3 women) | In-patient | 13 women, 8 men | 14.9 days | fMRI+videotapes; happy, sad, and cocaine video | No | Cocaine video elicited activation of ACC in both patients experiencing craving and patients experiencing no craving | No |
Bonson et al (Reference Bonson, Grant and Contoreggi2002) | Cocaine use (9 men, 2 women) | None | 0 | 2 days | PET+cue exposure (script, video and handling of paraphernalia) | Yes | Positive correlation with craving was observed in right DLPFC (BA9), left lateral OFC (BA12/47), left insula (BA13) and left amygdala/rhinal cortex | Yes |
Adinoff et al (Reference Adinoff, Devous and Best2003a ) | Cocaine dependency (10 women) | In-patient | 10 women | 9–28 days | SPECT+cocaine and placebo (saline) challenge Same study design as Adinoff et al (Reference Adinoff, Devous and Best2001) | No | Blunted limbic rCBF response after procaine. Increased rCBF response in bilateral insular and left superior frontal regions after procaine | |
Volkow et al (Reference Volkow, Wang and Ma2003a ) | Active cocaine use (25) | None | 0 | Current users | PET (FDG) | Yes | Methylphenidate increased whole-brain metabolism in both expected and not expected conditions. Largest increase in cerebellum, occipital cortex and thalamus | No |
Craving was associated with thalamus activation Greater increase for unexpected methylphenidate than expected methylphenidate in left lateral OFC | ||||||||
Kilts et al (Reference Kilts, Gross and Ely2004) | Cocaine dependency (8 women) | Out-patient | Cocaine dependency (8 men) | 1–14 days | PET+cue exposure (imagery script: neutral, drug use and anger script) | No (women) | Superior temporal gyrus, dorsal anterior and posterior cingulate cortex, nucleus accumbens and central sulcus were activated in female patients | |
Compared with men, less activation in amygdala, insula, OFC, ventral cingulate cortex; greater activation in central sulcus and widely distributed cortical areas |
Priming dose effects (‘drug probing’). Six studies measured the effect of placebo and a single dose of the drug of preference (or a closely related drug; Reference Stapleton, Morgan and PhillipsStapleton et al, 1995; Volkow et al, Reference Volkow, Gillespie and Mullani1996, Reference Volkow, Wang and Fowler1999a , Reference Volkow, Wang and Ma2003a ; Reference Sell, Morris and BearnSell et al, 2000; Reference Adinoff, Devous and BestAdinoff et al, 2001). All but one (Reference Adinoff, Devous and BestAdinoff et al, 2003a ) demonstrated orbitofrontal cortex activation in response to drug administration. In addition, all but two (Volkow et al, Reference Volkow, Gillespie and Mullani1996, Reference Volkow, Wang and Ma2003a ) showed orbitofrontal cortex activation to be related to the subjective experience of craving.
Cue exposure during early abstinence. Fourteen studies used a cue exposure paradigm during early abstinence (1–28 days), with the exception of the study by Daglish et al (Reference Daglish, Weinstein and Malizia2001) in which abstinence varied strongly.
Of the seven PET studies, four demonstrated activation of the orbitofrontal cortex in response to cue exposure (Reference Grant, London and NewlinGrant et al, 1996; Reference Wang, Volkow and FowlerWang et al, 1999; Reference Daglish, Weinstein and MaliziaDaglish et al, 2001; Reference Bonson, Grant and ContoreggiBonson et al, 2002), whereas two did not (Reference Childress, Mozley and McElginChildress et al, 1999; Reference Kilts, Schweitzer and QuinnKilts et al, 2001). One PET study demonstrated less activation of this region in female compared with male cocaine-addicted patients (Reference Kilts, Gross and ElyKilts et al, 2004). Remarkably, all studies focusing on female patients with substance use disorders failed to demonstrate orbitofrontal cortex activation in response to cue exposure (Reference Kilts, Gross and ElyKilts et al, 2004; Reference Tapert, Brown and BarattaTapert et al, 2004) or drug administration (Reference Adinoff, Devous and BestAdinoff et al, 2003a ). This suggests gender differences in the functionality of this region and in the degree of its involvement in addictive processes.
Of the seven fMRI studies, three demonstrated involvement of the orbitofrontal cortex in cue reactivity (Reference Garavan, Pankiewicz and BloomGaravan et al, 2000; Reference Tapert, Cheung and BrownTapert et al, 2003; Reference Myrick, Anton and LiMyrick et al, 2004). The between-study inconsistencies may be due to technical limitations. In fMRI designs the investigation of the orbitofrontal cortex can be complicated by susceptibility to artefacts induced by the air – tissue interface (Reference London, Ernst and GrantLondon et al, 2000). Alternatively, differences in cue exposure paradigms may influence results. Using cocaine-related videotapes as cues, Wexler et al (Reference Wexler, Gottschalk and Fulbright2001) found higher activity in the anterior cingulate cortex of cocaine-dependent patients, both before these patients experienced any craving and in the absence of craving. George et al (Reference George, Anton and Bloomer2001), using a sip of alcohol and images of alcoholic beverages, demonstrated thalamic involvement. In another study using videotapes, cocaine craving was associated with the activation of 13 brain areas including the orbitofrontal and anterior cingulate cortices (Reference Garavan, Pankiewicz and BloomGaravan et al, 2000). In a PET study using videotaped cues, Kilts et al (Reference Kilts, Schweitzer and Quinn2001) demonstrated cue reactivity in the anterior cingulate cortex, right inferior parietal cortex and caudate/lateral dorsal nucleus. Although the activation of these multiple brain areas may imply that craving relates to several neuroanatomical circuits, one might – in line with Kilts et al (Reference Kilts, Schweitzer and Quinn2001) – argue that inductive cues such as videotapes are too crude to allow a distinction between activations due to conditioned drug craving and activations associated with accompanying features of psychophysiological arousal, anticipation, memory retrieval, attention and behavioural planning.
Finally, treatment status might influence results. In all but one of the cue exposure studies (Reference Kilts, Gross and ElyKilts et al 2004) comparing gender differences in patients treated for cocaine addiction) in-patients systematically failed to demonstrate orbitofrontal cortex activation in response to cue exposure, whereas substance misusers responded with activation. The only exception was the study by George et al (Reference George, Anton and Bloomer2001); however, only ‘mildly severe’ cases of alcoholism were included, which might account for the lack of orbitofrontal cortex involvement in this study.
In summary, these imaging studies evidence activation of multiple brain regions during cue exposure. The type of drug did not differentially affect orbitofrontal cortex activity. Neither cue reactivity nor craving was exclusively or reliably linked to activation of this brain region. Other regions most frequently associated with cue exposure in patients with substance use disorders are the anterior cingulate cortex, dorsolateral prefrontal cortex and amygdala.
‘Brain at rest’ imaging studies
Of the 18 available studies testing the orbitofrontal cortex in drug-dependent patients with the brain ‘at rest’, only 3 failed to demonstrate involvement of this region. The studies can be divided into 13 metabolic and 5 structural studies (Table 4).
Table 4 Main results of ‘brain at rest’ imaging studies

Study | Substance use group | Control group | Abstinence | Method | OFC abnormality | Involvement of other regions | |
---|---|---|---|---|---|---|---|
Sample | Treatment | ||||||
Alcohol | |||||||
Volkow et al (Reference Volkow, Wang and Hitzemann1993) | Alcoholism, early onset (10 men) | In-patient | 12 men | 16 days | PET+placebo (i.v. saline: baseline PET+lorazepam (i.v. 30 μg/kg) | Yes | Lorazepam decreases regional brain metabolism. The alcoholism group showed a blunted response on lorazepam administration in OFC, thalamus and basal ganglia |
Volkow et al (Reference Volkow, Wang and Overall1997) | Alcoholism, early onset, FH+ (10 men) | In-patient | 16 men | First trial at 2–3 weeks’ abstinence Second trial at 6–8 weeks’ abstinence | PET, same design as in Volkow et al (Reference Volkow, Wang and Hitzemann1993) | Yes | Baseline regional brain metabolism alcoholics < controls during first trial (early withdrawal). Second trial (late withdrawal) brain metabolism alcoholics < controls in cingulate gyrus and OFC. Trend towards blunted lorazepam response in the OFC in alcoholism group during second trial |
Dao-Castellana et al (Reference Dao-Castellana, Samson and Legault1998) | Alcoholism (11 men, 6 women) | In-patient | 6 men, 3 women | 1 week to 1 month | MRI+PET | Yes | Hypometabolism predominantly in the mediofrontal cortex |
Catafau et al (Reference Catafau, Etcheberrigaray and Perez de los Cobos1999) | Alcohol dependency, type 2, Cloninger (16 men) | In-patient | 8 men, 5 women | First SPECT: 10 days | SPECT | Yes | Baseline perfusion patterns showed frontal rCBF impairment in alcoholism group in left OFC and prefrontal cortex. A single naltrexone dose induced decreases in basal ganglia and left mesial temporal rCBF |
Second SPECT: 12 days | SPECT+naltrexone | ||||||
Fein et al (Reference Fein, DiSclafani and Cardenas2002) | Alcohol dependency (24 men) | None | Light drinking, 17 men | Sober at MRI | MRI | No | Reduced global grey-matter volume in PFC, largest difference posterior PFC and DLPFC |
Goldstein et al (Reference Goldstein, Volkow and Wang2002a ) | Early onset alcoholism (17 men), cocaine addiction (17 men) | In-patient | 17 men | Sober/clean at urine test before scanning | PET (FDG) | Yes | Higher OFC activation was associated with poorer performance in control group and better performance on the Stroop task in substance misuse group |
Stroop interference task | |||||||
Laakso et al (Reference Laakso, Gunning-Dixon and Vaurio2002) | ASP+type 2 alcoholism (24 men) | In-patient | 33 men | Several months | MRI, ROI analysis | Yes | Alcoholism group had smaller volume in left DLPFC, OFC and MFC |
Cocaine | |||||||
Volkow et al (Reference Volkow, Fowler and Wolf1991) | Cocaine use+other drug use (15 men) | Out-patient | 17 men | 12 h to 1 week (n=10) PET 2–4 weeks (n=5) | PET | Yes | Patients studied within 1 week of cocaine withdrawal (but not those with 2–4 weeks of withdrawal) had higher levels of global brain metabolism and higher metabolism in the OFC and basal ganglia than controls |
Correlation found between number of days of abstinence and metabolism in the OFC and basal ganglia | |||||||
Correlation found between craving and metabolic activity in PFC and OFC | |||||||
Volkow et al (Reference Volkow, Wang and Fowler1998) | Active cocaine use (9 men, 4 women) | None | 6 men, 8 women | 5 days | PET+placebo (i.v. saline) PET+lorazepam (i.v. 30 μg/kg) | No | Lorazepam decreased global and regional brain glucose metabolism, more in the cocaine group than in the controls. Differences greatest in striatum, thalamus and parietal cortex. At baseline whole-brain metabolism in cocaine group > controls (specifically temporal cortex, thalamus and striatum) |
Franklin et al (Reference Franklin, Acton and Maldjian2002) | Cocaine dependency (13 men) | Yes | 16 men | 5 days | VBM | Yes | Decreased grey-matter density in OFC (+insular, temporal and anterior cingulate cortices) |
Lim et al (Reference Lim, Choi and Pomara2002) | Cocaine dependency (12 men) | Yes | 10 men, 3 women | < 6 months | DTI | Yes | Disrupted white-matter integrity of inferior frontal brain regions, including OFC region |
Adinoff et al (Reference Adinoff, Devous and Cooper2003b ) | Cocaine dependency (12 men, 1 woman) | 7 men, 8 women | 21–55 days | SPECT+IGT | Yes | Lower left DLPFC and right OFC resting rCBF | |
Matochik et al (Reference Matochik, London and Eldreth2003) | Cocaine use (11 men, 3 women) | None | 7 men, 4 women | 20 days (in-patient monitoring) | MRI (VBM) VOI analyses | Yes | Lower grey-matter density in medial and lateral aspects of OFC (+cingulate gyrus, lateral PFC) |
Methamphetamine | |||||||
Volkow et al (Reference Volkow, Chang and Wang2001) | Methamphetamine dependency (6 men, 9 women) | Out-patient | 14 men, 6 women | 2 weeks to 35 months | PET ([11C]raclopride and FDG) | Yes | Methamphetamine group had lower level of D2 receptor availability than control group (16% in caudate and 10% in putamen); D2 receptor availability was associated with metabolic rate in the OFC in both groups |
Goldstein et al (Reference Goldstein, Volkow and Chang2002b ) | Methamphetamine dependency (3 men, 11 women) | Drug rehabilitation centre | 17 men, 5 women | > 2 weeks | PET (FDG) MPQ harm avoidance scale | Yes | Higher MPQ scores were associated with higher relative OFC metabolism in methamphetamine group |
Chang et al (Reference Chang, Ernst and Speck2002) | Methamphetamine dependency (10 men, 10 women) | Out-patient | 10 men, 10 women | 8 months | MRI+perfusion MRI | No | Decreased relative rCBF basal ganglia and right parietal brain region, increased relative rCBF temporoparietal and occipital regions |
Sekine et al (Reference Sekine, Minabe and Ouchi2003) | Methamphetamine use (11 men) | ? | 9 men | 5, 6 months | PET ([11C]WIN 35,428) | Yes | Dopamine transporter density lower in metahamphetamine group in OFC, DLPFC and amygdala. Reduction in OFC and DLPFC correlated negatively with score of psychiatric symptoms and duration of methamphetamine use |
London etal (Reference London, Simon and Berman2004) | Methamphetamine dependency (11 men, 6 women) | In-patient | 10 men, 8 women | 4–7 days | PET (FDG) | Yes | Methamphetamine group had higher relative rCGM in lateral OFC, lower in ACC and insula. In this group trait anxiety covaried negatively with OFC activity |
Metabolic studies. During early withdrawal (less than 7 days of abstinence), the orbitofrontal cortex metabolism in participants with substance use disorders was either comparable with controls (Reference Volkow, Wang and FowlerVolkow et al, 1998) or higher than in the control group (Reference Volkow, Fowler and WolfVolkow et al, 1991; Reference London, Simon and BermanLondon et al, 2004). Studies during late withdrawal (>7 days) or prolonged abstinence demonstrated systematically low activity in the orbitofrontal cortex region (Reference Dao-Castellana, Samson and LegaultDao-Castellana et al, 1998; Reference Catafau, Etcheberrigaray and Perez de los CobosCatafau et al, 1999; Reference Volkow, Chang and WangVolkow et al, 2001; Reference Adinoff, Devous and CooperAdinoff et al, 2003b ; Reference Sekine, Minabe and OuchiSekine et al, 2003; Reference London, Simon and BermanLondon et al, 2004). Although the studies using a lorazepam challenge do not strictly qualify as ‘brain at rest’, they all demonstrate hypofunctionality of this brain region after withdrawal (Volkow et al, Reference Volkow, Wang and Hitzemann1993, Reference Volkow, Wang and Overall1997, Reference Volkow, Wang and Fowler1998). One study administering the Iowa Gambling Task after PET recording (Reference Adinoff, Devous and CooperAdinoff et al, 2003b ) showed performance on this task to be positively correlated with dorsolateral prefrontal cortex and anterior cingulate cortex metabolism at rest. All studies of substance use disorder that included measures of psychiatric symptoms or psychological traits indicated involvement of the orbitofrontal cortex in anxiety and disordered mood (Goldstein et al, Reference Goldstein, Volkow and Wang2002a , Reference Goldstein, Volkow and Chang b ; Reference Sekine, Minabe and OuchiSekine et al, 2003; Reference London, Simon and BermanLondon et al, 2004).
Only one study, using perfusion magnetic resonance imaging, failed to demonstrate any difference in the orbitofrontal cortex in long-abstinent people with methamphetamine dependency relative to a normal control group (Reference Chang, Ernst and SpeckChang et al, 2002). This may reflect brain recovery. Recently, Wang et al (Reference Wang, Volkow and Chang2004) demonstrated partial recovery of brain function after long-lasting abstinence in methamphetamine-dependent patients.
Structural studies. Three studies revealed smaller volumes (Reference Laakso, Gunning-Dixon and VaurioLaakso et al, 2002) and decreased grey-matter density in the orbitofrontal cortex (Reference Franklin, Acton and MaldjianFranklin et al, 2002; Reference Matochik, London and EldrethMatochick et al, 2003). Another study showed disruption in white-matter integrity, predominantly in the inferior frontal brain regions, indicative of disrupted connectivity in the orbitofrontal cortex region (Reference Lim, Choi and PomaraLim et al, 2002). One study (Reference Fein, DiSclafani and CardenasFein et al, 2002) did not report orbitofrontal cortex abnormalities but did demonstrate reduced global prefrontal grey-matter volume in treatment-naïve heavy drinkers without severe behavioural consequences of their alcohol use.
Taken together, the structural and metabolic studies demonstrate involvement of the orbitofrontal cortex in people with addictions. The main findings concerning this region are its hyperactivation during early withdrawal, hypoactivation after withdrawal or during prolonged abstinence, and its involvement in mood and anxiety changes in patients with substance use disorders.
DISCUSSION
Fifty-two studies were evaluated. Behavioural decision-making tasks consistently demonstrate impairments in decision-making in patients with substance use disorders compared with controls. In contrast, the relationship between orbitofrontal cortex activity, cue reactivity and craving is not consistent across the studies reviewed. Studies during acute withdrawal reveal hyperactivation of the orbitofrontal cortex, whereas studies during abstinence demonstrate hypoactivation of this region and structural abnormalities in individuals with substance use disorders.
Decision-making and the orbitofrontal cortex
The results obtained with the behavioural decision-making tasks warrant further elucidation. First, it remains uncertain whether the different tasks all measure the same cognitive entity. An overlap in the decision-making functions tapped by the IGT and the RCGT has been demonstrated (Reference Monterosso, Ehrman and NapierMonterosso et al, 2001). However, no data are available comparing the two-choice prediction task (Paulus et al, Reference Paulus, Hozack and Zauscher2002, Reference Paulus, Hozack and Frank2003) with the IGT and the RCGT. Second, studies analysing the relationship between behavioural decision-making tasks and the orbitofrontal cortex as their anatomical correlate are limited and conflicting. The sole neuroimaging study during IGT performance showed a task-related increase in orbitofrontal cortex metabolism in the cocaine users group (Reference Bolla, Eldreth and LondonBolla et al, 2003). In contrast, studies using the two-choice prediction task found decreases in task-related activity in the right orbito-related frontal cortex and the anterior cingulate cortex of methamphetamine-dependent participants (Paulus et al, Reference Paulus, Hozack and Zauscher2002, Reference Paulus, Hozack and Frank2003). This inconsistency may reflect intrinsic differences between these decision-making tasks. Anatomically, both the orbitofrontal and the anterior cingulate cortex are involved in all three decision-making tasks. As to the IGT, which is a complex task depending on a variety of cognitive processes, other regions seem to contribute as well (i.e. the dorsolateral prefrontal cortex, amygdala and insular regions; Reference Clark, Manes and AntounClark et al, 2003).
Taken together, as discussed by Lubman et al (Reference Lubman, Yucel and Pantelis2004), the orbitofrontal and anterior cingulate cortices are critically involved in inhibitory decision-making processes, especially involving reward-related behaviours (Reference Elliott, Friston and DolanElliott et al, 2000; Reference Kiehl, Liddle and HopfingerKiehl et al, 2000; Reference Fan, Flombaum and McCandlissFan et al, 2003; Reference Rogers, Ramnani and MackayRogers et al, 2004). Specifically, these regions process the reward value and/or affective valence of environmental stimuli, assess the future consequences of the individual's own actions (response selection) and inhibit inappropriate behaviours (response inhibition; Reference Elliott, Friston and DolanElliott et al, 2000; Reference Kiehl, Liddle and HopfingerKiehl et al, 2000; Reference Bechara and DamasioBechara & Damasio, 2002; Reference KrawczykKrawczyk, 2002; Reference Fan, Flombaum and McCandlissFan et al, 2003). Dysfunctions within these regions have recently been proposed as a key neural mechanism underlying addiction (Reference Jentsch and TaylorJentsch & Taylor, 1999; Reference Goldstein and VolkowGoldstein & Volkow, 2002; Reference Volkow, Fowler and WangVolkow et al, 2003b ; Reference Lubman, Yucel and PantelisLubman et al, 2004).
The orbitofrontal cortex, cue reactivity and craving
Findings concerning the relationship between the orbitofrontal cortex, cue reactivity and craving are inconsistent across the studies. Of 20 studies, 13 demonstrated cue exposure to be associated with hyperactivity of the orbitofrontal cortex. One study demonstrated cue-induced hyperactivity in male patients with cocaine addiction but not in female patients. In addition, other brain regions are involved as well: the amygdala, dorsolateral prefrontal cortex and anterior cingulate cortex were the most commonly reported loci of activation.
The inconsistencies between the various studies may originate from differences in study design, drug use status, treatment status or gender. First, different cue exposure paradigms have been used. As yet, not enough is known about the effects elicited by the different types of non-chemical cues (videotapes, drug paraphernalia, script reading) and chemical cues (single dose administration, ethanol odour). Possibly, different cues affect different brain circuits. Second, drug use status seems important. Withdrawal is consistently linked with orbitofrontal cortex activation. In addition, single drug-dose administration (drug probing) systematically evokes hyperactivity of this region; this is of high clinical relevance, since it is known that during abstinence the use of a limited amount of the drug of choice is a powerful trigger of craving and reinstatement of drug-taking habits in people with addictions. Third, treatment status may relate to cue reactivity. Wilson et al (Reference Wilson, Sayette and Fiez2004) proposed the treatment-seeking state of the participants as a variable explaining the disparity in brain-region activation in response to cue exposure. Non-treatment-seeking people with addictions could anticipate more actual drug use shortly after testing than those who were treatment-seeking. The studies on cue exposure in the current review lend some support to this hypothesis. Finally, gender differences might mediate differences in orbitofrontal cortex cue reactivity. In the three studies of women with substance use disorders, cue exposure elicited no activity in this brain region; this suggests that processing of reward/salience may involve different neural circuits in men and women. Noteworthy in this respect is that, increasingly, research is revealing gender differences in IGT performance, with men performing better than women (Reference Reavis and OvermanReavis & Overman, 2001; Reference Bolla, Eldreth and MatochikBolla et al, 2004; Reference OvermanOverman, 2004).
The relationship between orbitofrontal cortex activity and experiences of craving remains unclear. Craving is a complex process that may involve several interacting brain regions (for a review, see Reference FrankenFranken, 2003). Craving is associated with the learned response that links the drug and its environment to an intensely pleasurable experience. Anatomically, the consolidation of this memory (trait craving) is likely to involve the amygdala, hippocampus and the nucleus accumbens shell. The actual conscious experience of craving as a result of cue reactivity has been postulated to be linked to the orbitofrontal cortex and possibly the anterior cingulate cortex (Reference Goldstein and VolkowGoldstein & Volkow, 2002). However, in our review the data did not conclusively support this hypothesis. In only 6 of the 17 studies (35%) was craving associated with orbitofrontal cortex activation. In 9 other studies other brain regions were implicated. Further research is warranted to differentiate the roles of the orbitofrontal and anterior cingulate cortices and other regions involved in cue reactivity and craving.
Orbitofrontal cortex activity after withdrawal
The structural and metabolic neuroimaging studies after drug withdrawal consistently demonstrated a decrease in orbitofrontal cortical volume, metabolism and functionality. These findings are in line with other reports revealing reductions in dopamine D2 receptor density in people with substance use disorders (Volkow et al, Reference Volkow, Wang and Fowler1999b , Reference Volkow, Chang and Wang2001). Among other regions, the areas affected are the dopaminergic projections from the striatum (nucleus accumbens) to the cingulate gyrus, prefrontal cortex and the orbitofrontal cortex. This deficit may play an important part in addictive processes, conceptualised as a process of hedonic homoeostatic dysregulation (Reference Koob and LeMoalKoob & Le Moal, 1997). Clinical correlates of a hedonic dysregulation are the dysthymic or depressive episodes that are frequently observed after detoxification. These mood changes can be persistent and difficult to treat. Such an anhedonic state can be a serious hazard in maintaining abstinence and may induce a relapse. The studies by London et al (Reference London, Simon and Berman2004), Sekine et al (Reference Sekine, Minabe and Ouchi2003), and Goldstein et al (Reference Goldstein, Volkow and Chang2002b ) suggest involvement of the orbitofrontal cortex in mood and anxiety disorders in methamphetamine addiction.
General remarks
In addition to their drug of preference, many participants in the studies we reviewed used (and misused) multiple other substances. Although poly-substance misuse is of particular clinical interest, becoming a common pattern of drug misuse, it complicates the interpretation of the results. Furthermore, although most of the participants with substance use disorders were nicotine-dependent, nicotine status was never taken into account in the various studies. This might also have biased the results reported, since nicotine use itself has been linked to changes in orbitofrontal and anterior cingulate cortical metabolism (Reference Brody, Mandelkern and LondonBrody et al, 2002). Future studies should take into account this potential confounding factor, either by excluding smokers or by statistical adjustment for smoking status.
Finally, the findings in the studies under review do not allow a distinction to be made between cause and consequence. Functional and structural deficits in decision-making cognition and orbitofrontal cortex integrity can be either a consequence of or a pre-existent vulnerability to addictive behaviour. For some drugs of misuse such as methamphetamine, evidence of their (sometimes long-lasting) neurotoxic effects is growing (Reference Wang, Volkow and ChangWang et al, 2004). However, the findings reported on in this review seem to be relatively independent of the type of substance misused. The observed abnormalities are probably not substance-specific but rather constitute a common deficit in addicted states and/or a common predisposing vulnerability (Reference Blum, Braverman and HolderBlum et al, 2000). In this context, it might be of interest to mention the evidence demonstrating involvement of the orbitofrontal cortex in non-chemical addictions such as gambling (Reference Cavedini, Riboldi and KellerCavedini et al, 2002; Reference Potenza, Steinberg and SkudlarskiPotenza et al, 2003; Reference Goudriaan, Oosterlaan and de BeursGoudriaan et al, 2004).
Collectively, both the behavioural and neuroimaging studies included in this review point to an important role of the orbitofrontal cortex in addictive processes. They lend further support for the model developed by Volkow et al (Reference Volkow, Fowler and Wang2003b , Reference Volkow, Fowler and Wang2004), who propose a network of four brain circuits involved in addiction (memory, drive, reward and control). In this model, exposure to the drug or to drug-related cues activates the memory of the expected reward, resulting in hyperactivation of the reward and motivational circuits while decreasing the activity in the cognitive control system. The deficits highlighted in our review are indicative of an important role for the orbitofrontal cortex in a brain circuit mediating goal-directed behaviour, leading to compulsive drug-seeking and relapse. In doing so, the orbitofrontal cortex contributes to the perpetuation of the addiction.
Clinical Implications and Limitations
CLINICAL IMPLICATIONS
-
▪ Pharmacological and behavioural treatment should allow for the powerful effect drug probing has on hyperactivation of the orbitofrontal cortex and the associated risk of craving and reinstatement of drug use.
-
▪ Future research should focus on the aetiological, diagnostic and therapeutic aspects of mood disorders and anhedonic states following withdrawal related to chronic hypofunctionality of this brain region.
-
▪ Extensive documentation of treatment status is warranted in future imaging studies on cue reactivity and craving.
LIMITATIONS
-
▪ The Iowa Gambling Task is not specific for orbitofrontal cortex functionality. Studies using more specific behavioural tasks should be used in research into substance misuse.
-
▪ Our review exclusively highlights the role of the orbitofrontal cortex in decision-making. Other behavioural aspects characteristic of addictive processes (e.g. impulse control) related to this region have not been considered.
-
▪ Studies specifically focusing on gambling and tobacco-smoking were excluded. Future reviews should take these populations into account.
Acknowledgements
The authors thank Eric Achten, MD, PhD, of the Workgroup fMRI Ghent, University of Ghent, Belgium, for his assistance in analysing the neuroimaging studies.
eLetters
No eLetters have been published for this article.