Introduction
Would a grown building be comfortable to live in? And how would it sound?
If we could grow a building, the built environment would not only become a product of our living environment but – at least during the growth stage – be part of the natural cycle and the underlying biochemical processes. During the biofabrication of the structure, living agents would transform water, nutrients and energy into materials. Such a circular bioconstruction process would not only be waste free as all natural residues of one process become nutrients for another, but it would also potentially generate an ecological gain, such as capturing anthropogenic carbon from the atmosphere, or absorbing toxicants from contaminated soils. In this understanding, the ability to grow buildings would be a key enabler to restore and regenerate the health of the living environment of our planet following the principles of the emerging paradigm of regenerative design (Armstrong Reference Armstrong2023). This article investigates in what ways such biofabricated enclosures could also tangibly contribute to healthy living environments focusing on the acoustic comfort of a grown living space, based on the case study of the Myx Sail installation at the Danish Design Museum (DDM) in Copenhagen.
Mycelium based composites (MBC)
MBC are one of the most extensively researched biofabricated building materials today. MBC are produced by inoculating a substrate of lignocellulose, often consisting of plant biomass and residues, with fungal spores. In a controlled humid and warm environment, the hyphae, the vegetative part of the fungi, spread across the substrate, branching in all three dimensions, binding the plant fibres of the substrate together. A heat treatment stabilises the biocomposite material and stops growth before fruiting takes place. Replicable growth protocols, composition and processing of substrates and moulding techniques have been established to produce MBC performative building materials (Vanden Elsacker Reference Vanden Elsacker2021) with generally a very limited environmental impact (Livne et al. Reference Livne, Wösten, Pearlmutter and Gal2022). Due to their porous fibrous structure and low density, MBC have been successfully introduced in the market as acoustic absorbers by different companies such as mogu (MOGU 2023). Composition strategies have been developed and tested to improve the limited mechanical performance of MBC and widen potential application fields (Rigobello et al. Reference Rigobello, Colmo and Ayres2022).
Mycelium Textiles and the Myx Sail at DDM
In contrast to fabricating discrete MBC components such as bricks or panels designer Jonas Edvard has developed a technique of “Mycelium Textiles” that does not work with discrete MBC components but employs fibre mats as a growth substrate (Jonas Edvard Studio 2023).
Currently larger scale mycelium structures incorporating textiles are being developed and investigated, such as the BioKnit installation incorporating textiles for permanent formwork (Kaiser et al., Reference Kaiser, Bridgens, Elsacker and Scott2023). The Myx Sail is one of the biggest structures of Mycelium Textiles to date, designed in collaboration with Arup and displayed at the DDM in Copenhagen as part of the exhibition “The Future is present” curated by Pernille Stockmarr. It is 3 m wide, 4 m long and has a bow of approx. 0,15 m to 0,25 m. It is composed of 24 mycelium panels of 1 m × 0,5 m. The panels are suspended off the ceiling approx. 2,5 m to 3 m from the floor shaping a continuous concave absorbing surface (see Figure 1).
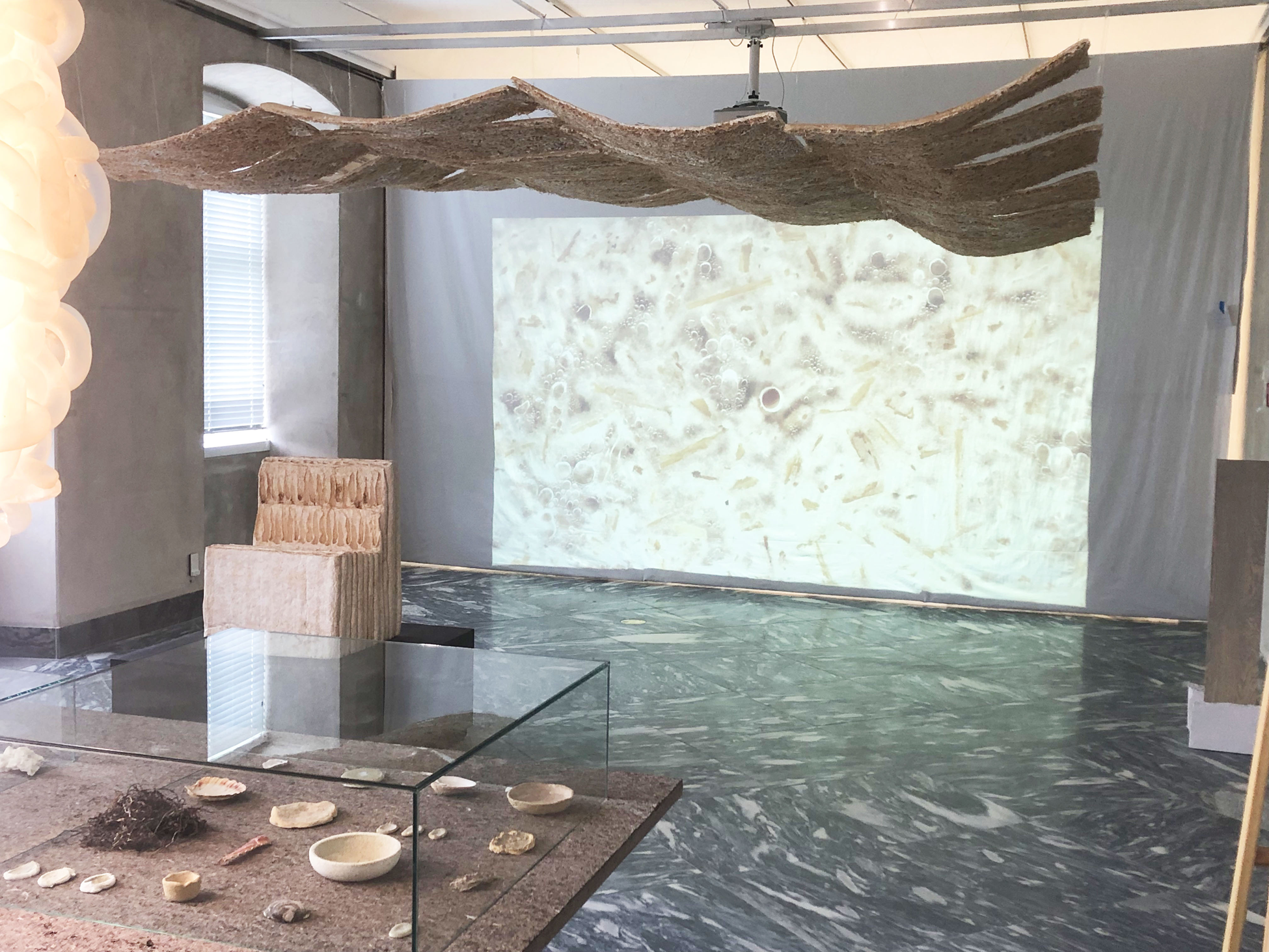
Figure 1. Setup of the Myx Sail at Danish Design Museum in May 2020, Source: Jonas Edvard.
The production of the panels started early spring 2021. The exhibition was opened in June 2022 and is scheduled to last until end of 2024. To date, the installation has been moved once resulting in two different configurations. The modular and flexible structure can enable different configurations in the future to adopt to the specific architectural settings on site.
The installation was designed to showcase the acoustic properties of a grown mycelium composite surface. The selection of materials for the Myx Sail is informed by an initial research on the acoustic properties of MBC, which was carried out by Jonas Edvard and Arup in 2020–21, funded by Fritz und Trude Fortmann Stiftung (Edvard Reference Edvard2023). Based on an extensive research of more than 250 samples of fibrous plant materials, differing in size, composition and principal acoustic absorbance, a selection of 20 MBC combinations was tested at the TU Berlin to characterise the acoustic absorption through an impedance tube test based on ISO 10534-2.
This article investigates in what ways the installation of the composite sail contributes to the acoustic comfort of the exhibition space. Based on taken acoustic measurements on site the absorption factors of the installation are quantified. In addition the subjective perception of the visitors is captured through an online survey. Both presented data sets are interpreted to assess the acoustic qualities and comfort of a grown enclosure made of MBC.
Methods
Introduction
The installation of the Myx Sail display at the DDM offered a unique opportunity to study acoustic interactions between a large absorber surface of MBC and a public environment, through a diversity of investigative methods. The positioning of the sail at one end of an exhibition hall allowed for a physical investigation of its acoustic properties, such as the degree to which it dampens reverberation or reduces noise levels in the room (see Figure 7). Additionally, data on the exhibition visitors’ thoughts and impressions of the mycelium sail could be gathered through a public survey. These two sets of data – the physical effects of the sail and the subjective perceptions of the public – provided insights into the potential uses and future developments of mycelium composite materials.
To investigate the acoustic properties of the Myx Sail, two independent series of acoustic tests were carried out. The test sequences were designed to gather technical information about the following aspects of the mycelium composite sail:
-
The degree to which the sail fundamentally absorbs acoustic energy (test sequence 1)
-
The effectiveness/limitations of the sail as a room acoustic treatment, considering various spatial arrangements between sound sources and receivers (test sequence 2)
Composition, making and installation of Myx Sail
The final substrate chosen for the Myx Sail is a combination of wood wool from pine trees (Pinus) and a nonwoven hemp fibre mat with a supporting Hessian textile of loose woven jute fibre. The wood wool was chosen for its lightweight and flexible structure, keeping its inherent strength and position during the handling, sterilisation and moulding process. The hemp fibre mat was selected as a cultivation substrate. Hemp fibres are naturally rich in lignocellulose providing nutrients supporting the growth of the hyphae. Finally the woven jute textile was integrated to provide stability and strength and facilitate suspension of the individual panels. The textile controls the position of each panel in two dimensions while enhancing the flexibility and ability of the dehydrated panels to move slightly in the third dimension. In the build-up the textile was positioned on top to facilitate the structural suspension from the ceiling while the acoustically more absorbing wood wool on the bottom is facing the museum space (see Figure 2).
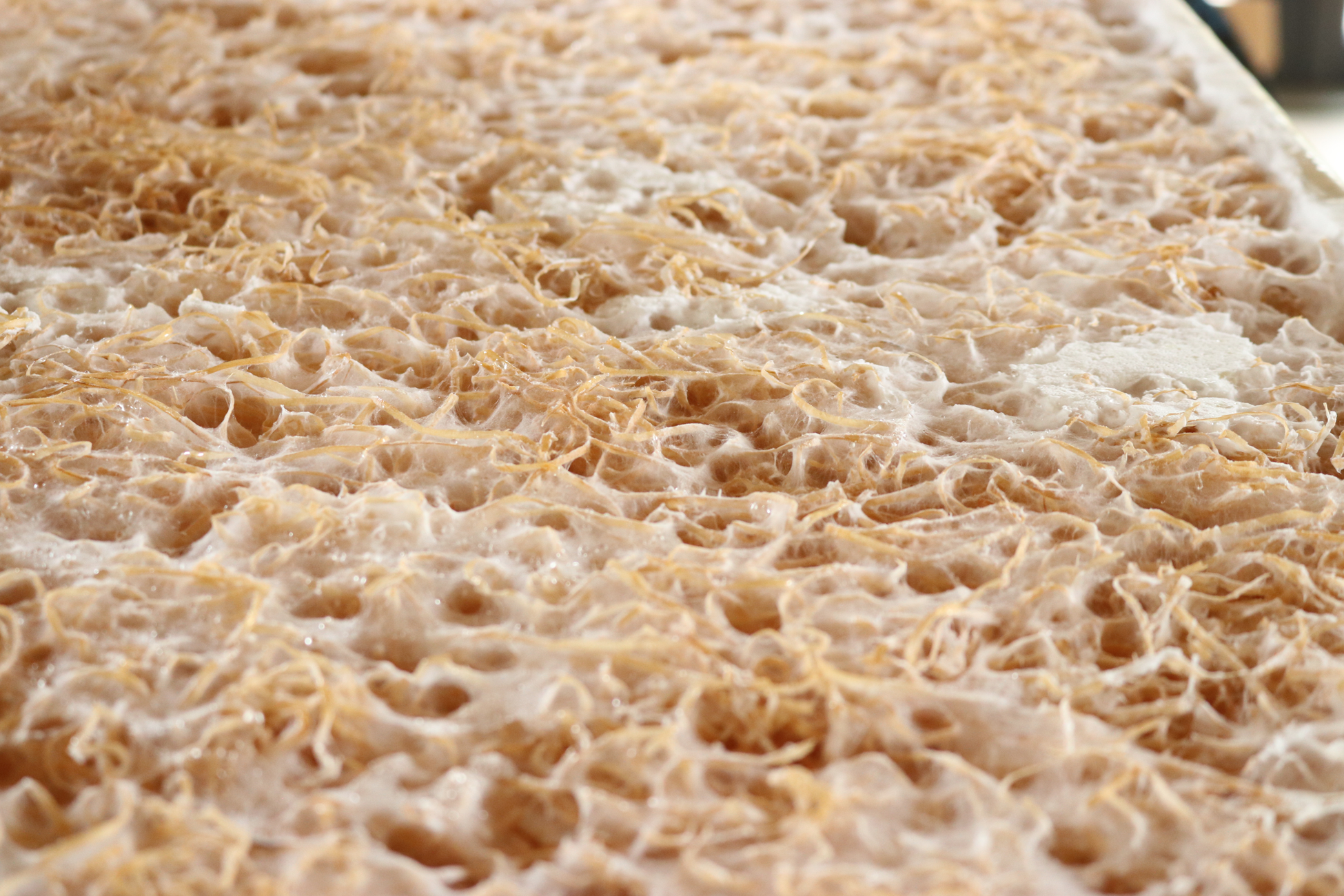
Figure 2. Close-up detail of underside of a Myx Sail panel, Source: Jonas Edvard.
The inoculation of the pasteurised material followed the build-up of the different layers of materials. The added mycelium was in the shape of grain (millet) inoculated with oyster mushroom (Pleurotus ostreatus) spores and the grains were added in between the different layers. As a relatively fast growing species, Pleurotus ostreatus reduces a risk of contamination during the colonisation of the substrate of the individual panels.
The materials were colonised at room temperature (20–22 °C) in moulds of timber crates (see Figure 3). The first layer inside the mould was the nonwoven hemp fibre mat, second the jute textile with the wood wool being the top layer inside the mould (see Figure 4). The positioning of the wood wool on top of the open mould allowed to enhance the airflow across the surface and to control colonisation rates to avoid the forming of a continuous mycelium skin and instead to encourage growth of a more porous and sound-absorbing structure.
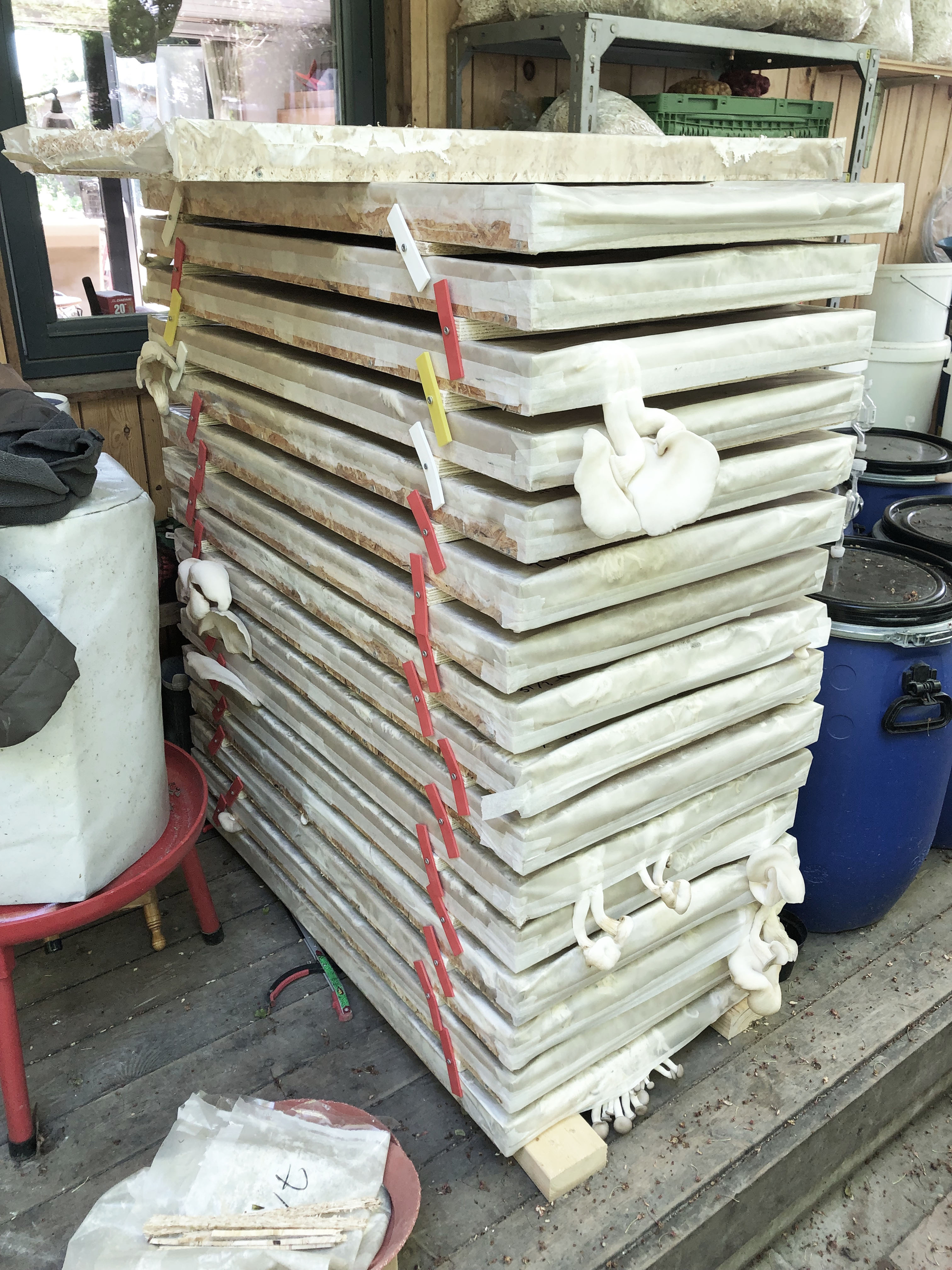
Figure 3. Picture of stacked moulds in the studio, Source: Jonas Edvard.
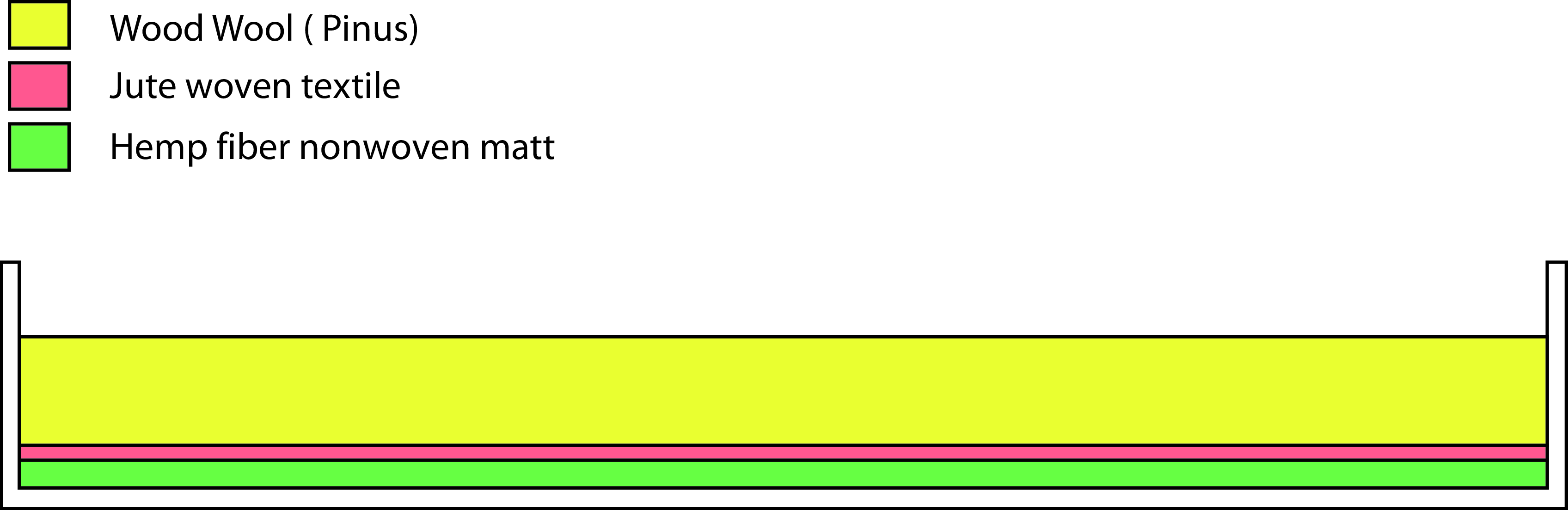
Figure 4. Diagrammatical section through mould showing build-up of layers of different substrate materials combined for the inoculation phase, Source: Jonas Edvard.
The inoculation of the materials occurs during a 10 day growth period with the mycelium spreading through the fibres of the different materials until the substrate is fully colonised. The panels were stabilised in a final hydration with a temperature of 60 °C in order to stop the microbial activity of the material.
The arrangement of the panels and the installation of the sail was developed by testing the flexibility of the mycelium panels by means of their suspension. Each panel is connected with its neighbouring panels at the centre points in both directions when spread out on a flat surface. The deadload of the panels leads to a gravity pull downwards, generating a curved surface. Each panel deflects also by its own weight creating an undulating, curvy structure enhancing the acoustic responsiveness of the sail. The bend lines of the individual panels describe different curvatures which control the orientation of the acoustic absorbers (see Figure 5).

Figure 5. Adjustment of Myx Sail suspension model, Source: Jonas Edvard/Benedikt Trojer.
Basis for the acoustic test strategy
The importance of the chosen test sequences may not be obvious at first glance but can be easily explained. When there is a sound inside of a room – such as a person speaking, or music playing from a loudspeaker – that sound will bounce off the interior surfaces of the room, becoming quieter with each reflection, until it finally is no longer audible. If the room only has hard surfaces (such as stone, concrete, or glass), the sound energy will stay in the room for a longer time – or in technical terms, the room will have a longer reverberation (DIN EN ISO 3382, 2009).
Long reverberation times are not always beneficial. While it creates a nice ambience for instrumental music, longer reverberation times also decrease speech intelligibility. Reverberation times longer than one or two seconds produce a condition where any words which were spoken in the past few seconds will still be partially audible – and will interfere with the word currently being spoken, making them less understandable. Materials which absorb acoustic energy can reduce the length of reverberation in a room, making it better for speech communication and also reducing overall noise levels (DIN 18041, 2016).
A standard method for determining the sound-absorbing properties of an object is to measure the length of the reverberation in a room, with and without the object. The difference in the length of the room’s reverberation can be used to determine how much acoustic energy the object absorbed (ISO 354, 2003).
However, the position of the sound-absorbing material in a room is also important. Sound absorption is a localised phenomenon. If a room is very large compared to the sound-absorbing object, the result will be a room with different “acoustic zones” – one zone could be more reverberant and louder, while the other zone is more dampened and quieter. This is sometimes the case in restaurants, where the open area is quite noisy, but areas near a curtain are quieter (DIN 18041, 2016).
Acoustic testing strategy: parallel investigations into material properties and spatial effects of the sail
In order to gain a detailed understanding of how the Myx Sail influences the room acoustics of the exhibition hall, two complementary acoustic tests were conducted. One focused on the material properties of the sail itself, and the other focused on the effect it had on the exhibition hall. With this combined approach, a strong technical understanding of how visitors should experience the acoustic influence of the sail was established.
For the first acoustic test sequence, the reverberation time in an unoccupied and unfurnished room of the DDM was measured, with and without the Myx Sail. This provided a view into the fundamental properties of the sail itself. For the second test sequence, the sound levels and speech intelligibility in the official Myx Sail exhibition room were measured across a grid of 12 locations (as marked on Figure 7), to show how sound levels and intelligibility changed in relationship to distance from the sail and position in the room. This gave insight into the degree to which the benefits of the sail are spatially dependent and/or localised.
All acoustic measurements were carried out using calibrated equipment including an omnidirectional loudspeaker and a laboratory-grade sound level metre (IEC 61672, 2013). The test procedures were designed using the specifications of international standards which have been referenced previously, with particular focus on the methods provided in ISO 3382-2 (2009) and ISO 354 (2003).
Survey
The subjective data about visitor’s experiences and impressions of the sail was gathered using an online survey format. As museum visitors approached the Myx Sail exhibit, they could read information about the material on a display poster – which included a scannable QR Code that functioned as a link to the survey. At date of analysis (30.08.2023) total of 134 museum visitors participated in the online survey, providing a statistically robust data set.
The online survey was designed to allow visitors to describe their experiences in real time. Participants were asked a total of seven questions – the first six of which involved rating various aspects of their experience on a 1–5 numeric scale, and the seventh question allowed them to write a short comment or text describing their experience in their own words.
The questions with numeric responses were arranged in a specific order, so that the simpler questions were at the beginning, and the more detailed observations were at the end. All seven questions in the survey are listed below:
-
1. How many people are in the room with you right now?
-
2. How do you feel right now? Are you comfortable and relaxed, or do you feel uncomfortable and stressed?
-
3. How much of a change in your experience do you notice, when you stand under the sail?
-
4. Which of your five senses are most effected by the sail?
-
5. How does the sail change the acoustics of the room?
-
a. Is it more loud or quiet?
-
b. Is there more or less echo?
-
c. Are sounds more clear or less clear?
-
-
6. How do you find the sail itself?
-
a. Do you find it pleasant or unpleasant?
-
b. Do you find it to be more artificial, or more natural?
-
c. Do you find it to be very familiar, or very new?
-
-
7. How would you describe the way the Sail influenced your experience? (short text response)
The design of the survey enabled an investigation into whether the visitors perceived the same acoustic effects of the sail which were documented in the physical tests. It was expected that not all visitors would experience the sail the same way, and the goal of the study was to identify non-acoustic factors which influenced their answers.
To investigate the survey responses, a statistical analysis method was used, which helps to demonstrate correlation between various responses. The goal was to understand to what degree a positive or negative response to a particular question (such as “how many people are in the room with you right now?”) is correlated to positive or negative responses in all of the other questions. These types of correlations can reveal useful trends in the data set that help with an understanding of how visitors experienced the sail in its context in the museum.
There are many diverse types of statistical analysis. Picking the most appropriate one of them will depend on the aspects of the data that are being investigated. In the case of this investigation, a Pearson correlation analysis was used.
The Pearson’s correlation coefficient is one of the most widely used methods for measuring the dependence between two quantities (Kirch Reference Kirch2008). It can be mathematically defined as follows:

Where:
-
ρ xy : Correlation coefficient.
-
cov(X,Y): Covariance of X and Y data variables.
-
σ x , σ y : Standard deviation of X and Y variables respectively.
The correlation coefficient ρ can vary between “−1” and “1”, where “−1” means that the two sets of data are fully inverse correlated and the second that they are fully correlated. A value of “0” determines that the samples are not correlated at all. The other values show the degree of correlation between the two samples, which increases as shown in the following Table 1.
This covariance-correlation analysis was done using the Python programming language. Numerical data was drawn from the text responses (question 7) using a novel object-based syntactic linguistic analysis method developed by Arup. This linguistic analysis allows for individual objects in the text responses (such as the sail, the museum, or the visitor’s feelings) to be numerically rated on various axes such as “pleasantness” or “familiarity”, giving more detailed insights into the visitor’s logic behind their numerical responses in the first six questions. These numerical assessments of the text responses were calculated in such a way as to match the 1–5 rating scales used in the other six questions, so that numerical data extracted from the text responses could be analysed side-by-side with the responses to the first six questions.
Table 1. Degree of correlation for a given correlation coefficient value

Table 2. Measured reverberation times in acoustic test sequence 1, with and without mycelium sail

Results
The acoustic measurements were carried out at the beginning of the exhibition period, on a day when the museum was closed, to ensure that no visitors were present. This allowed for an investigation into the physical-acoustic properties of the material, while avoiding the risk of data inaccuracy due to noise levels produced by visitors and other exhibits.
Mycelium enhancement of acoustic absorbance
The specific layering of the substrate and the subsequent cultivation process proofed to be key to achieve a foam like sound-absorbing mycelium material (see Figure 8). The layer of entangled wood wool acts as a supporting structure and scaffold for the mycelium to spread, nurtured by the hemp fibre mat below.
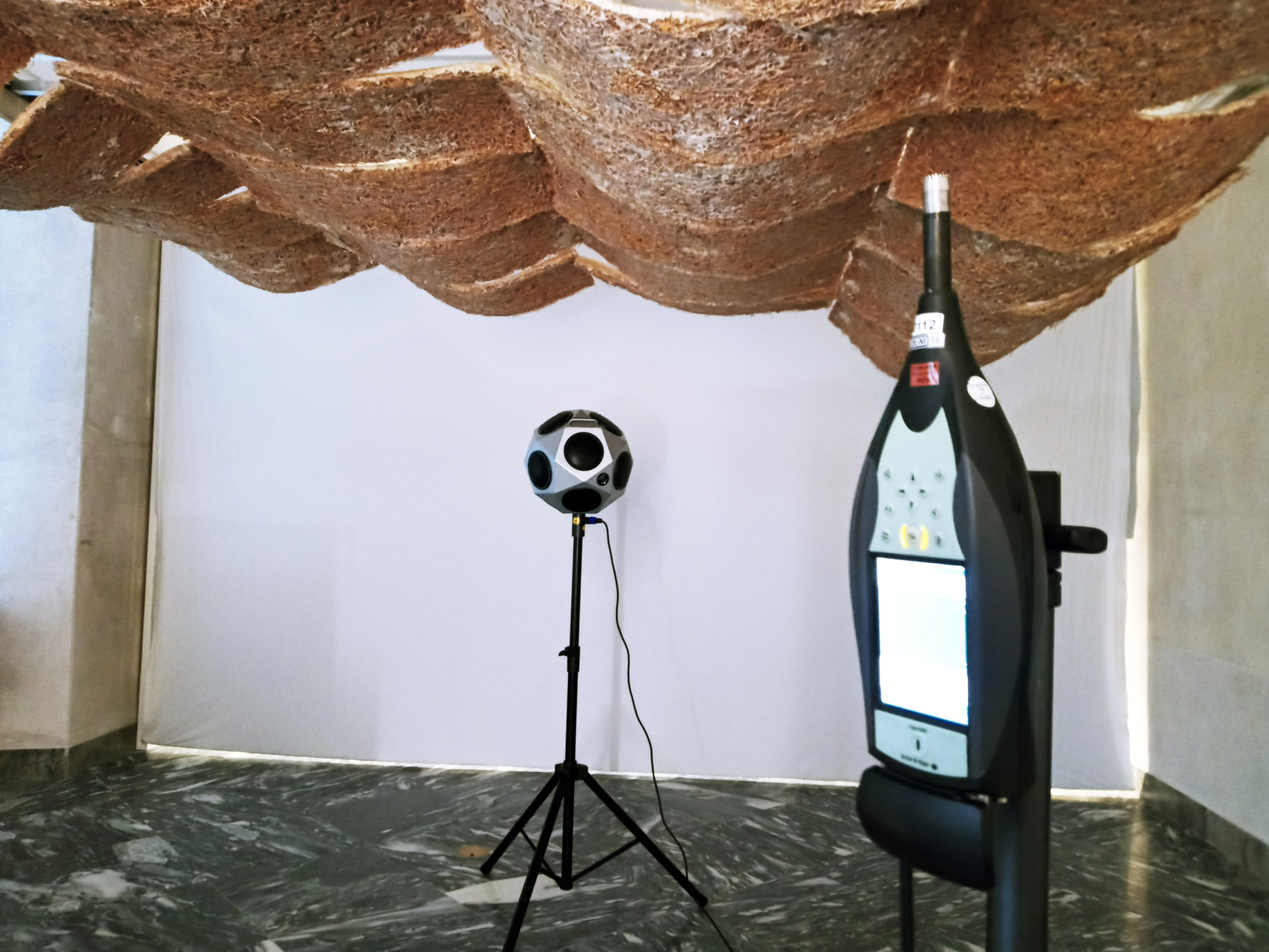
Figure 6. Acoustic test signals being played from an omnidirectional loudspeaker under the sail, and measured using a Class 1 (IEC 61672-1) laboratory-grade sound level meter, Source: Albert Dwan (Arup).
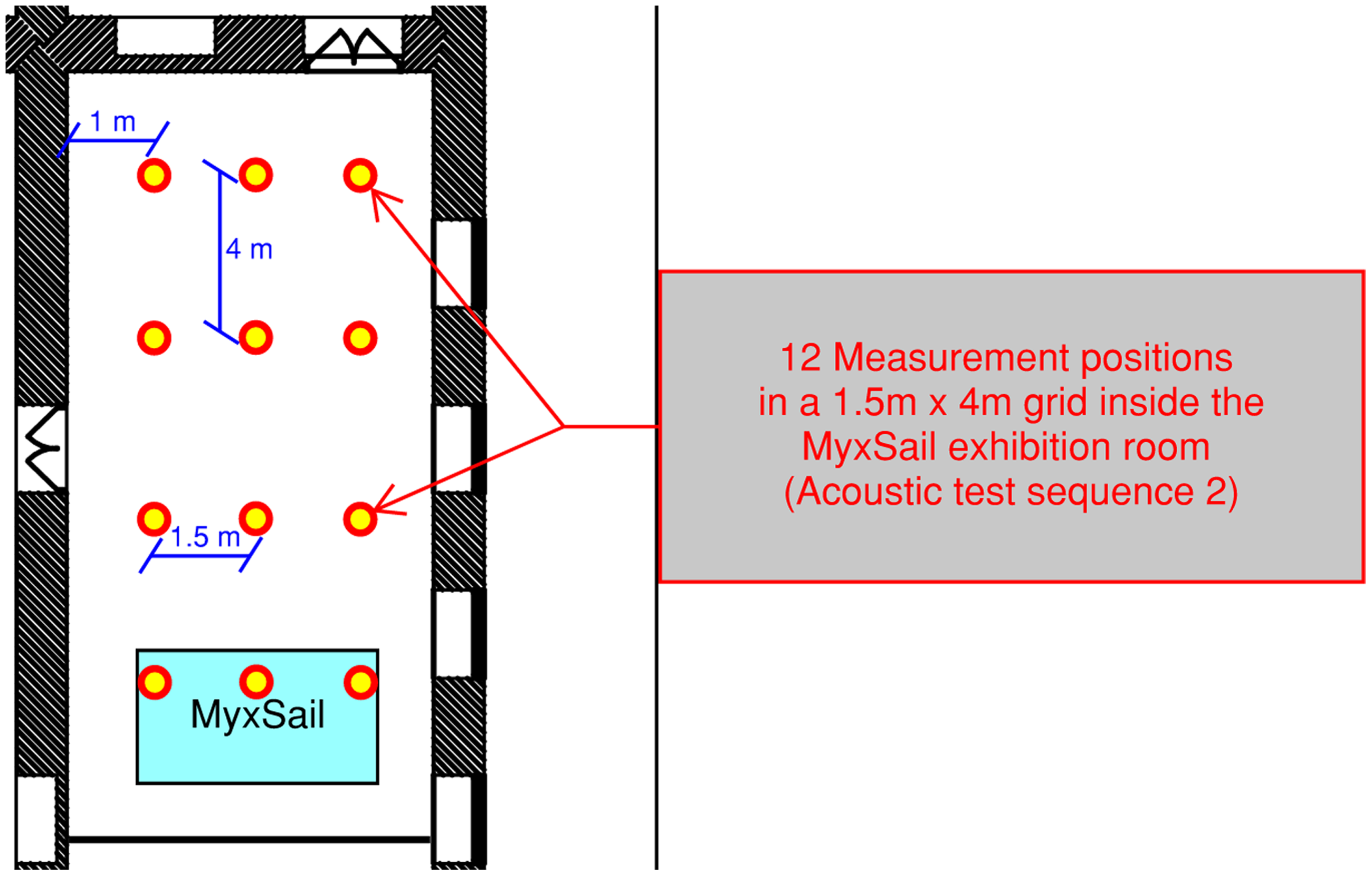
Figure 7. Positioning of the Myx Sail and grid of measurement positions in the exhibition hall under acoustic test sequence 2, Source: Albert Dwan (Arup).
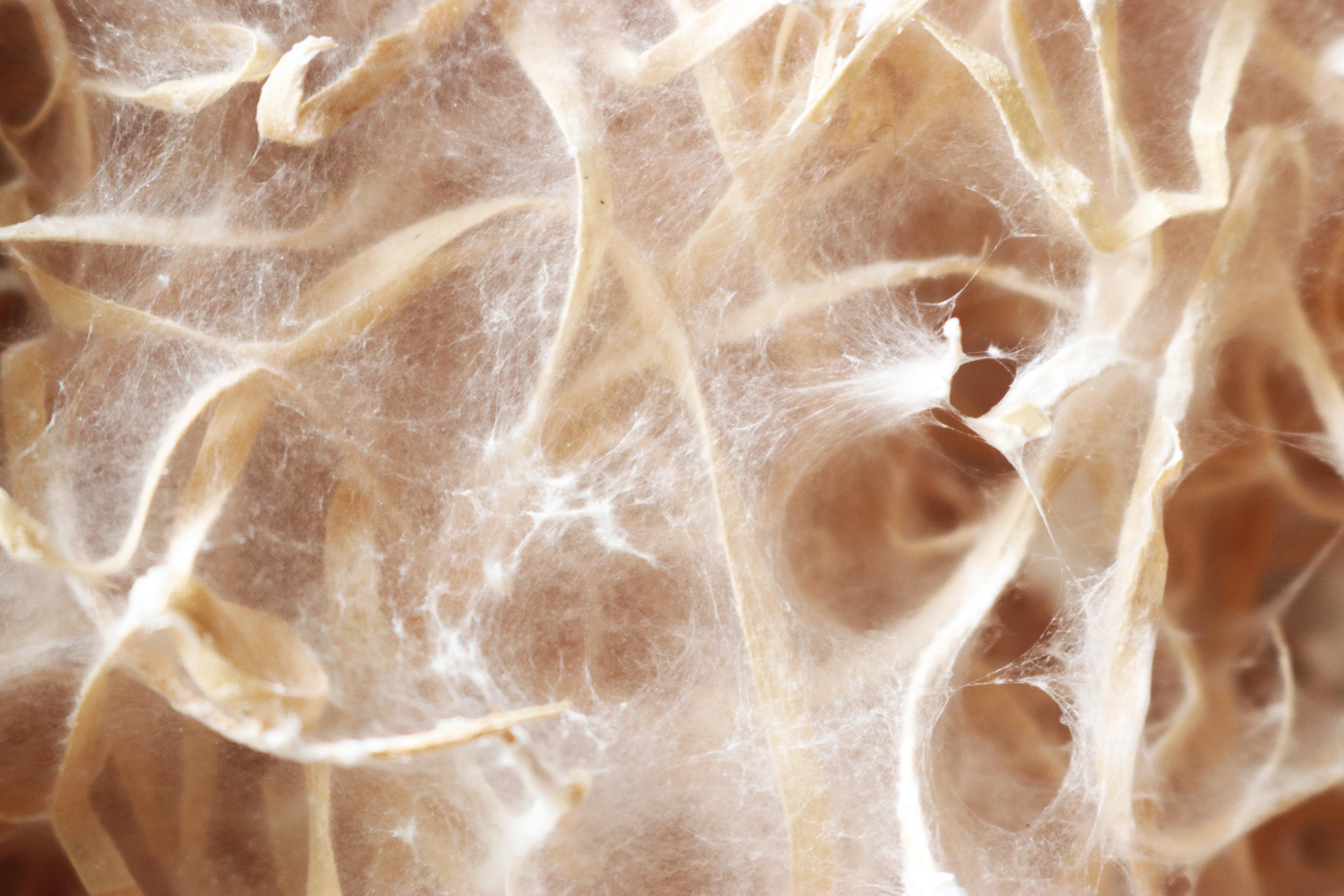
Figure 8. Detail of the composite material and the fine mycelium web after hydration, Source: Jonas Edvard.
The porous and open cell spatial structure of the MBC is characterised by the hyphae forming between the wood wool shavings that effectively function as a growth support for the propagation of the mycelium.
The emergent material effects of the wood wool, characterised by its natural curling, stiffness and length of wood wool fibres plays therefore a key role in the forming of the mycelium and its sound-absorbing properties. Further research needs to be carried out to understand the interrelation between the geometric structures, availability of embedded nutrients and environmental conditions to maximise the sound absorbance of such a layered MBC.
Acoustic test sequence 1: Material properties of the sail
For the first series of acoustic tests, the reverberation times in an unused room of the museum were measured with and without the Myx Sail installed. The measured reverberation times (shown in Table 2 and Figure 9) show a clear difference between the two cases – indicating that the Myx Sail does fundamentally absorb acoustic energy and can be used to reduce reverberation in rooms. The conditions of the room do not perfectly match a laboratory environment, so some components of the measurement data may be influenced by the room geometry and positioning of the sail with respect to other interior surfaces (particularly in the low-frequency range).
Table 3. Calculated absorption coefficient of the Myx Sail, in third-octave frequency bands

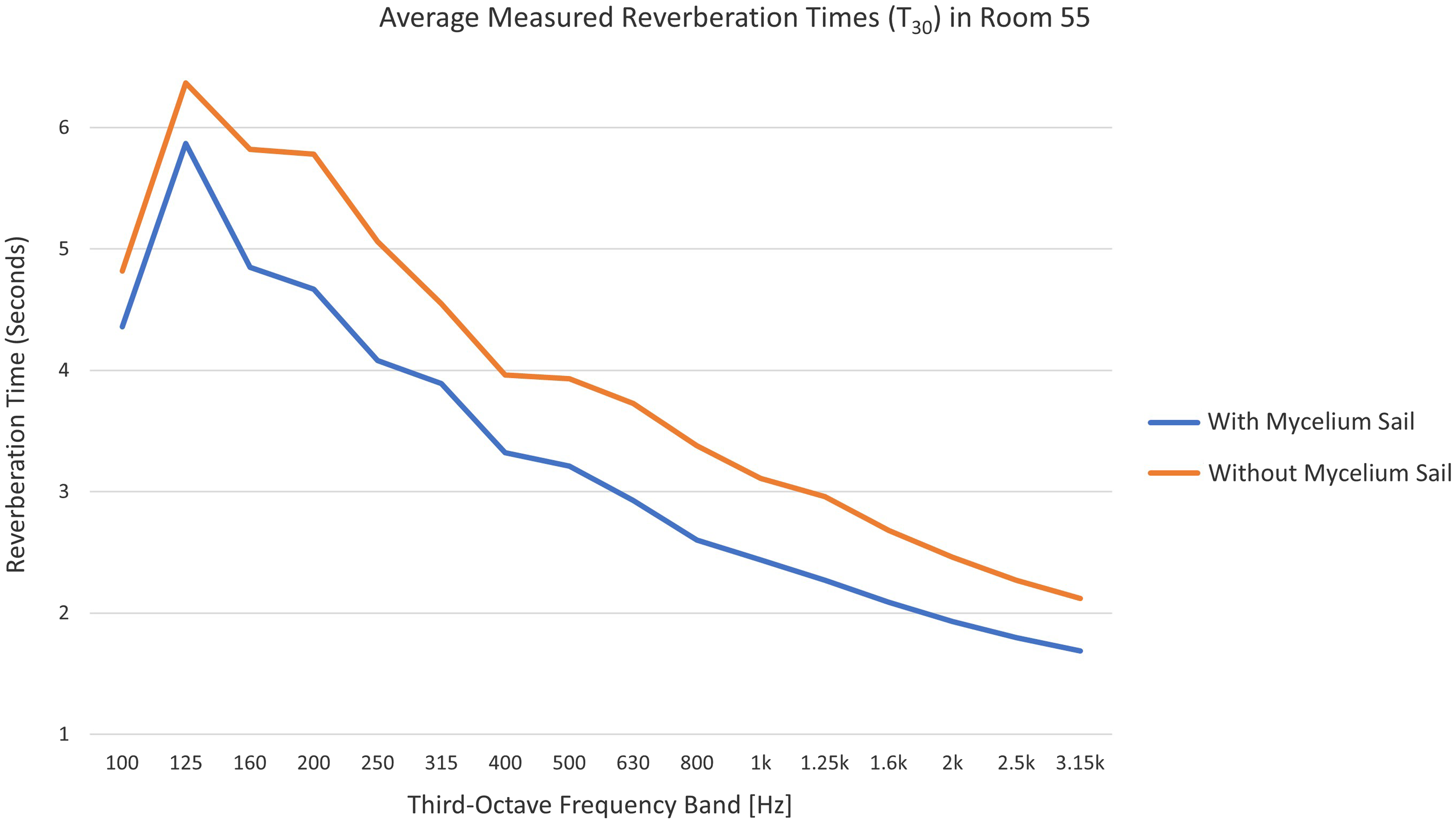
Figure 9. Measured reverberation times in Acoustic Test Sequence 1, with and without mycelium sail, Source: Albert Dwan (Arup).
The change in reverberation time demonstrates that the Myx Sail can be used as an effective sound-absorber, but do not show the precise degree of efficacy, as a property of the material itself.
In this case, the material property in question is called the absorption coefficient (represented by the symbol α), which is a number between 0 and 1 indicating the percentage of acoustic energy which gets absorbed by the material. If a material has an acoustic coefficient of 1, this indicates that there is perfect absorption, and no sound will be reflected from that material at all.
By applying the methods provided in ISO standard 354, an estimate of the absorption coefficient of the mycelium composite material was made (Table 3 and Figure 10). It should be noted that these results are not laboratory values. However, these absorption coefficient estimates do approximately represent the absorption characteristics of the mycelium composite material.
Table 4. Summary of statistically significant Pearson correlations in the survey response data
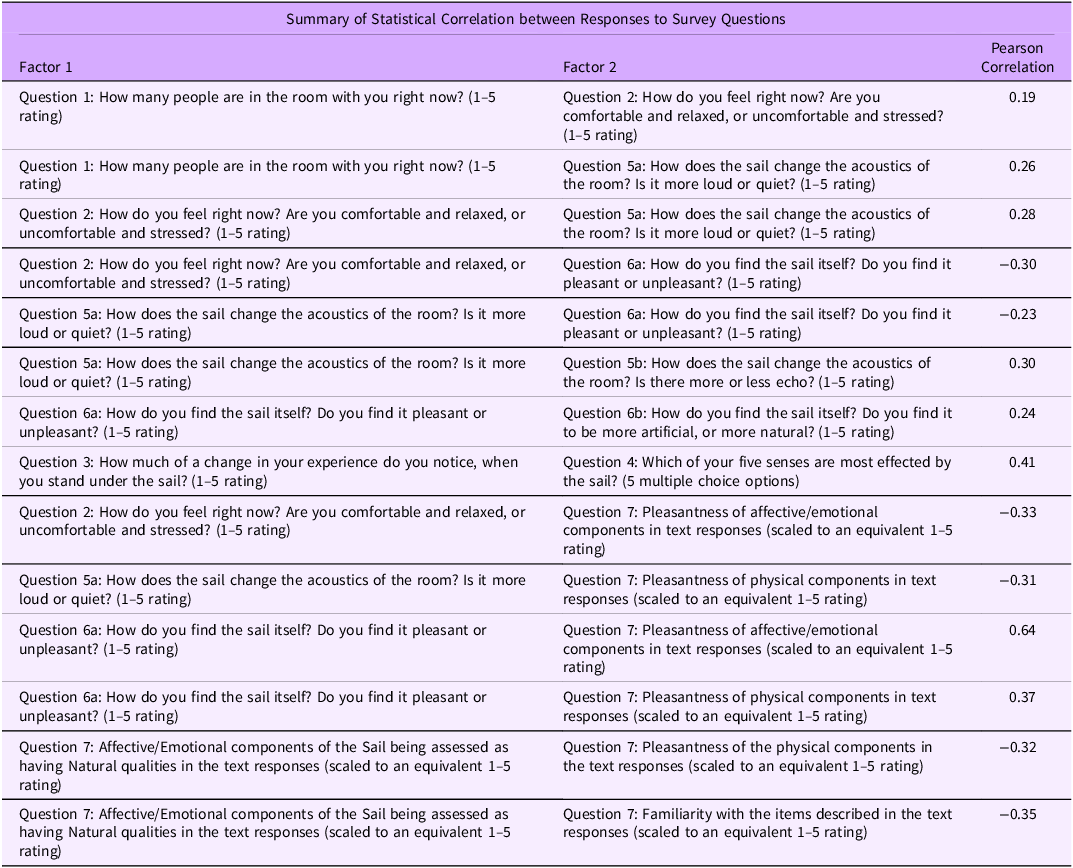
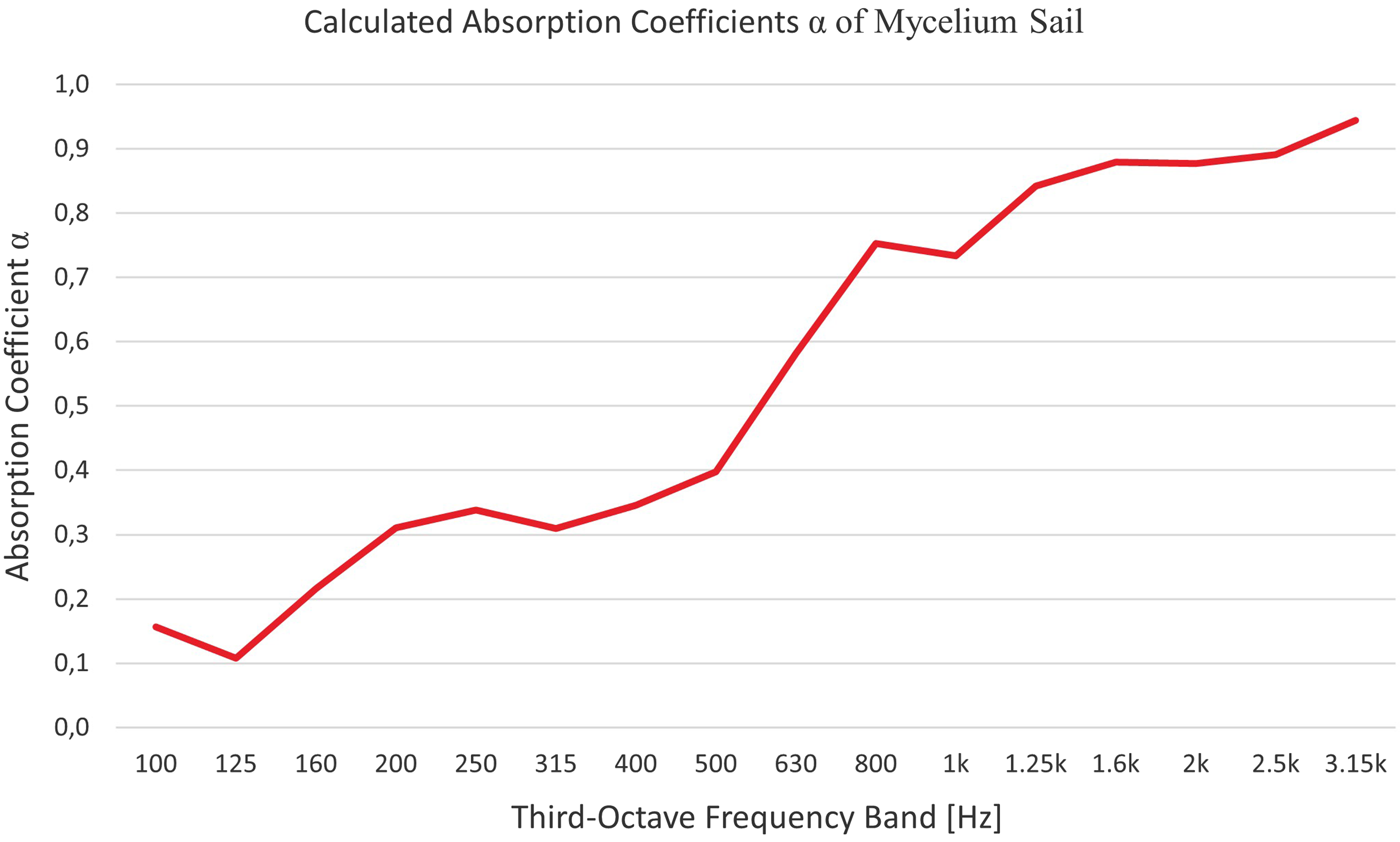
Figure 10. Calculated Absorption Coefficient of the Myx Sail, in third-octave frequency bands, Source: Albert Dwan (Arup).
To maximise the accuracy of the measurements, the “Precision-Method” according to the measurement standard DIN EN ISO 3382-2:2008-09 was used for gathering the reverberation time data. According to this “Precision-Method” the measurement of reverberation times must be repeated at least 12 times using at least two loudspeaker positions. In the case of this measurement, two loudspeaker locations and eight microphone positions for each source position were used, resulting in 16 repetitions of the measurement. This was important for the measurement precision, because the geometric distribution of measurement points in a room will strongly influence the measured sound levels and reverberation times, creating a range of results that must be averaged to reach a statistically representative value. Standard deviation was most significant for low-frequency ranges (below 800 Hz), which is to be expected due to the spatial distribution of room modes and standing waves corresponding to unfavourable geometric relations between acoustic wavelengths and specific room dimensions (i.e. distance between parallel walls). The strong spatial dependency of room acoustic measurements is normally managed with a high degree of measurement repetition, and standard deviation is often not reported as high variation is an expected result of the physical phenomenon being investigated. However, it is worth noting that in the higher frequency (above 1 kHz) ranges, where the interaction of the sound field with the room geometry plays less of a role, the standard deviation of the measured reverberation times of 4.2% achieved compliance with the recommended maximum value of 5% established by the measurement standard 3382-2. This indicates that the diffuse sound field in the room was relatively even in its spatial distribution in these higher frequency ranges, implying that the room featured relatively good conditions for this experiment.
The Myx Sail does absorb sound across a broad range of frequencies, but is more effective in the higher range of frequencies. This is true of many commonly available products, and the absorption coefficients shown here are not very different from some currently available sound-absorbers.
These results mean that the sail is more effective at absorbing sound associated with consonants in speech. The letters T, P, S and sounds such as SH or CH are critical for the intelligibility of speech. Without a clear delivery of these sounds, speech begins to sound “muddy”, consisting of a blurry mix of lower-frequency vowel sounds such as O, U and A (DIN 18041, 2016).
By absorbing sound in the “consonant range” of frequencies, this sail can improve acoustic conditions for speech intelligibility. It should be noted that all measurement results pertain to the performance of the composite material consisting of wood fibres bound together by a mycelial root structure. It was not possible in the context of this study to differentiate between the sound-absorbing properties of the mycelium itself as opposed to a loose matrix of wood fibres, as either of these materials by themselves would not have been able to be suspended in the form of a hanging acoustic sail.
Acoustic test sequence 2: Spatial relationships between sound sources and receivers
While it is technically useful to understand the material properties of the sail, the absorption coefficients are only one piece of the puzzle. Another important aspect is the effectiveness of the sail in real-world conditions.
Under this sequence, measurements were carried out in the exhibition hall to show the influence of the sail on sound sources at various positions in the room. The two conditions were (as shown on Figures 11–14):
-
With the sound source located directly under the Myx Sail (Pos L1)
-
With the sound source positioned in the middle of the exhibition hall, further away from the Myx Sail (Pos L2)
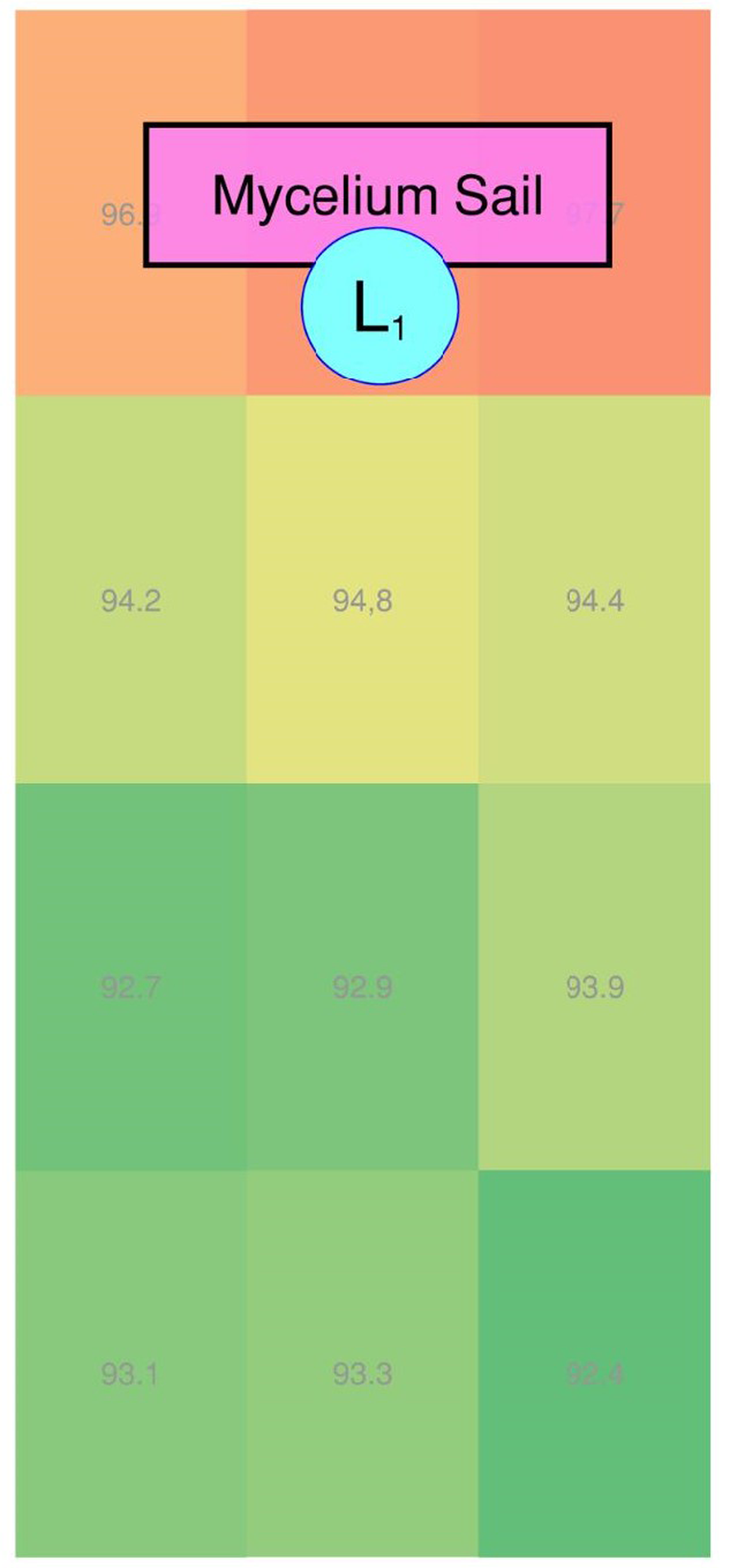
Figure 11. Measured dB (A), source under the sail (L1), Source: Albert Dwan (Arup).
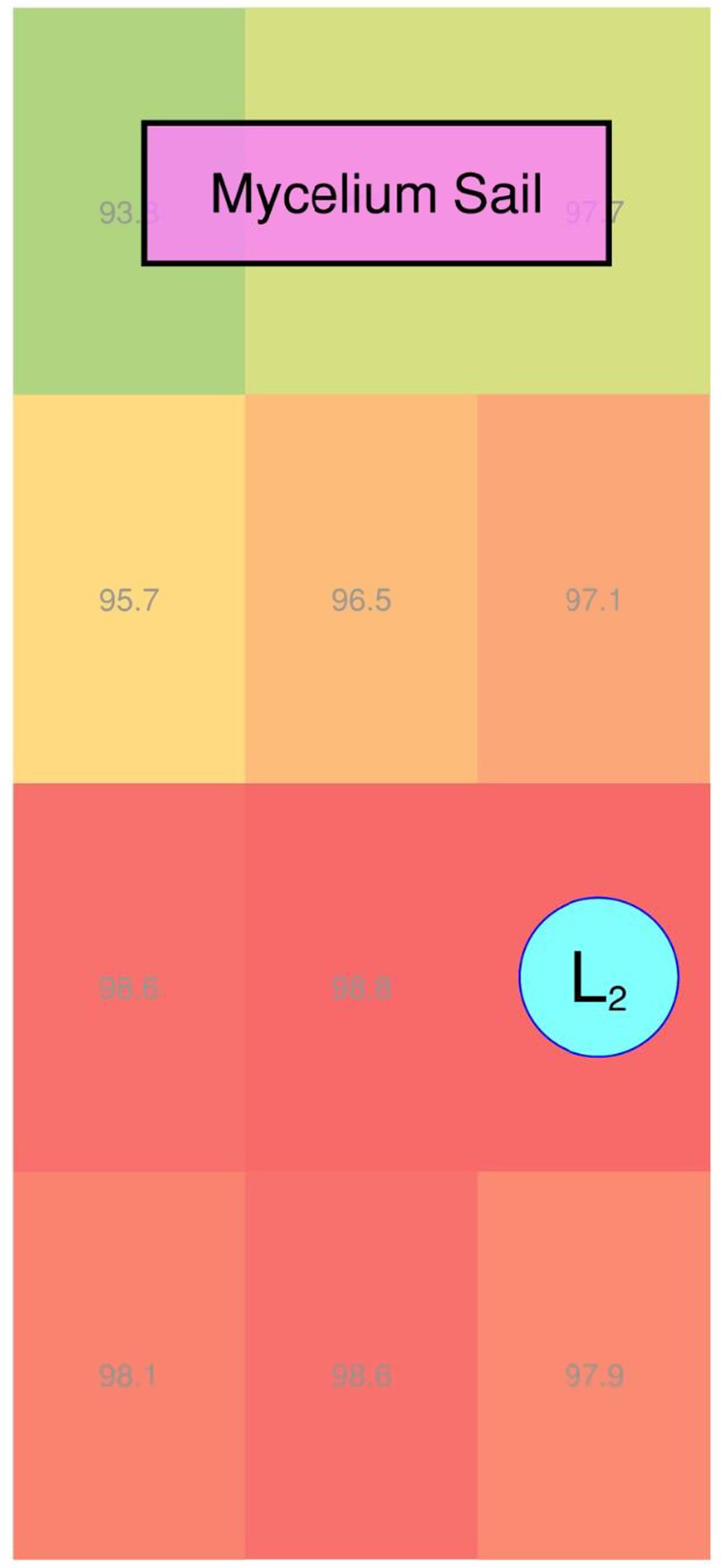
Figure 12. Measured dB (A), Source further away from sail (L2), Source: Albert Dwan (Arup).
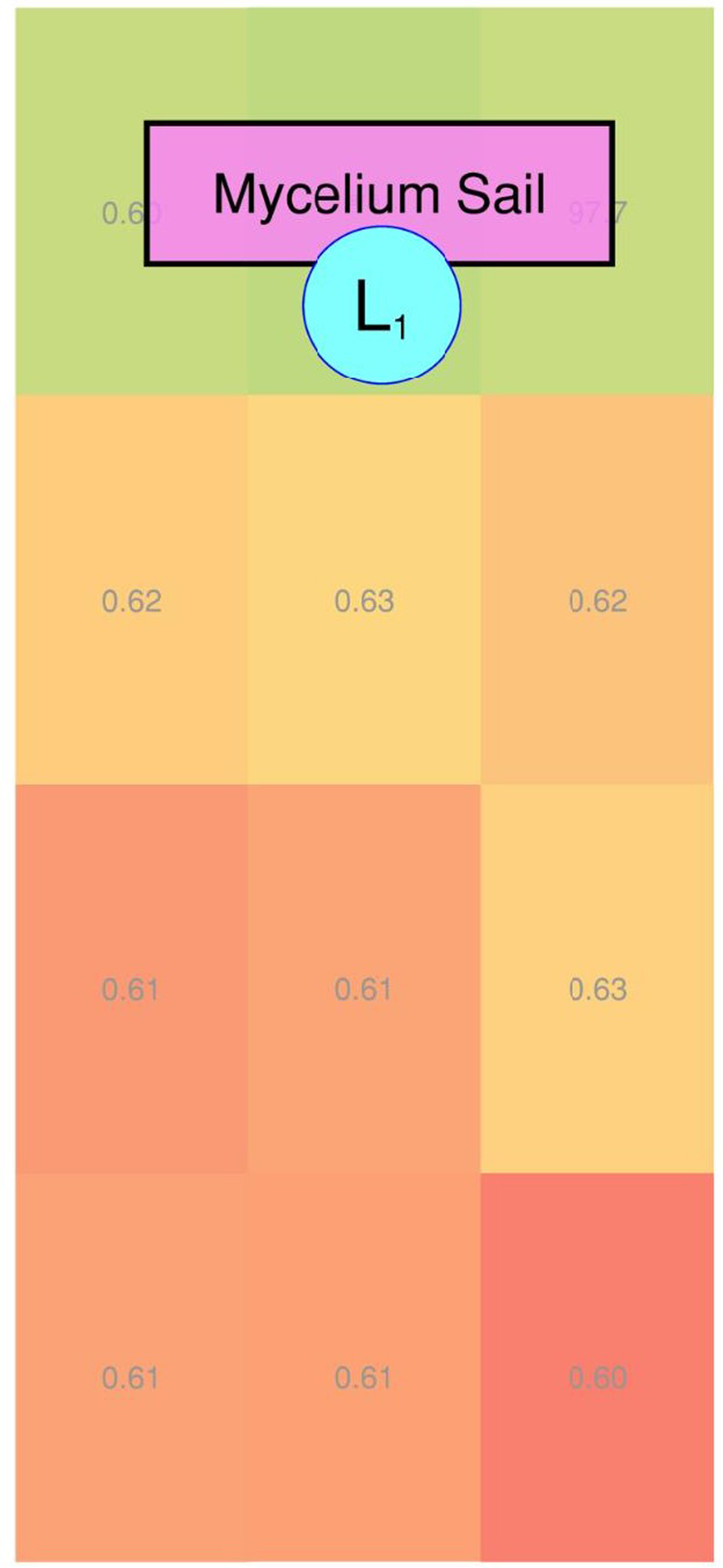
Figure 13. Calculated STI, Source under the Sail L1, Source: Albert Dwan (Arup).
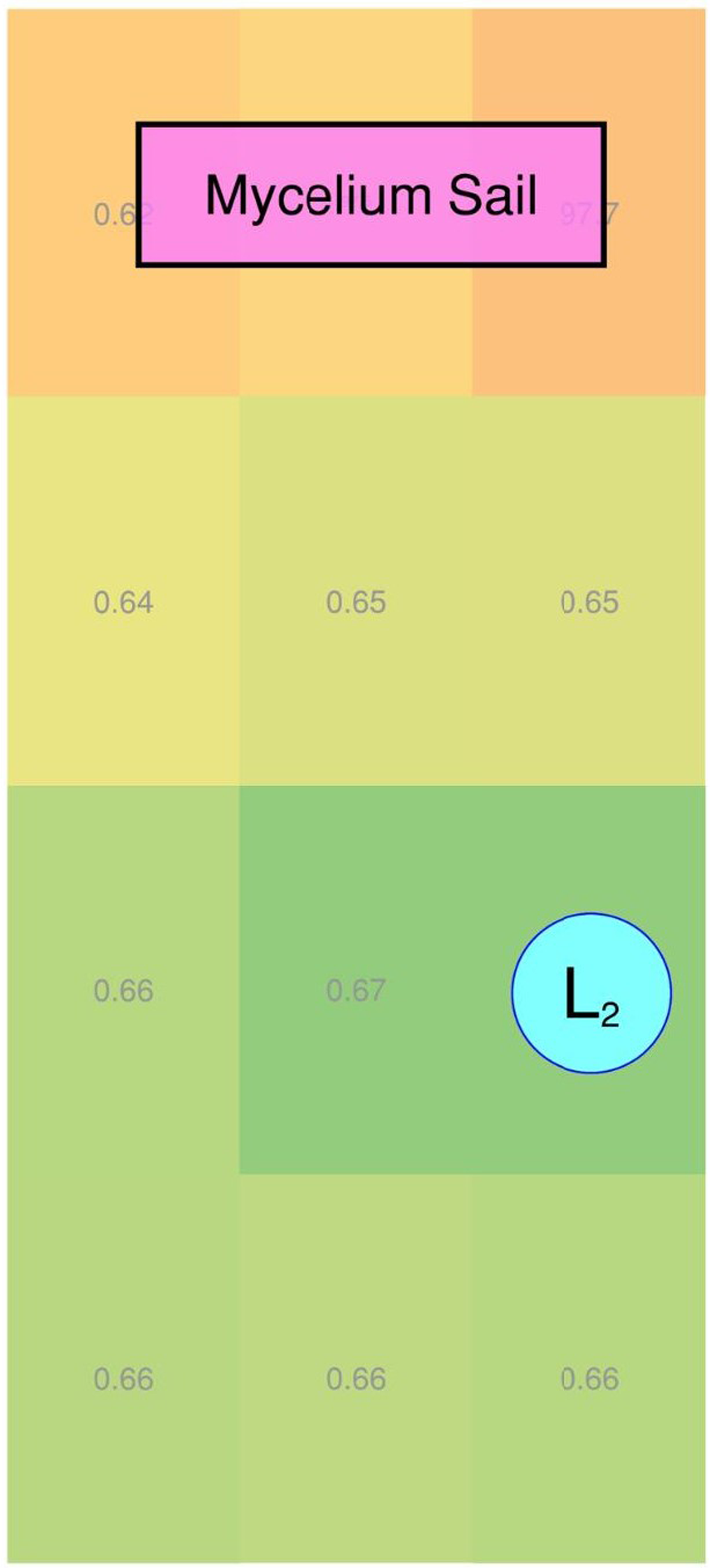
Figure 14. Calculated STI, Source further from Sail L2, Source: Albert Dwan (Arup).
Taking measurements at a grid of 12 positions throughout the exhibition room allowed for a visualisation of the sound propagation inside the room, by overlaying the measurement results on a diagram with the grid of measurement positions.
In order to maximise the accuracy of the results, each measurement (consisting of each combination of the two loudspeaker positions and the twelve microphone positions) was repeated three times, to achieve compliance with the “Precision-Method” for room acoustic measurements as defined by the measurement standard DIN EN ISO 3382-2:2008-09 §4.3. This differs from the precision criteria applied to the first Acoustic Test Sequence, in which the determination of the properties of the sail itself required the reduction of error emerging from spatial distribution of variances in the acoustic field. In this second acoustic test sequence, it is precisely the variances in the acoustic field in the room which were the object of study, requiring a repetition of measurements for each loudspeaker-microphone combination. Here, the standard deviation between measurements was smaller than in the first acoustic test sequence, which is to be expected, because the spatial relationships were only marginally changed (slight variation in microphone position, within a margin of 15 cm), resulting in an average standard deviation of 3.8%, which falls below the maximum permissible value for the Precision-Method of 5%.
The main result of this second investigation was that the overall sound level in the exhibition room was significantly reduced when the sound source was positioned directly under the Myx Sail. This is because more acoustic energy is captured from the source before radiating out into the rest of the room.
When the sound source was positioned further away from the Mycelium Sail (Pos L2), the overall sound levels in the room increased on average by 2.5 dB(A), which represents almost a doubling of acoustic energy in the room.
In the following figures, the measurement results are overlayed on a diagram of the room. The position of the Myx Sail is shown with the blue-grey rectangle, and the sound source position is shown with the blue circle labelled “L1” and “L2” for Loudspeaker at its first and second position, respectively. At each of the twelve measurement positions, the relative sound level is shown using a green-to-red colour scale, with green indicating quieter sound levels, and red indicating louder sound levels.
As can be seen in these visualisations of sound level in the exhibition hall, the Myx Sail can significantly reduce sound levels in a room, but only when the sound source is positioned close to the sail. A useful application would be to hang this sail above a conference table or group meeting area, so that conversations in that area do not radiate out and produce loud sound levels in the rest of the room.
It was also possible to determine speech intelligibility using the Speech Transmission Index (STI) Scale (DIN EN ISO 3382, 2012). STI is similar to the absorption coefficient in that it is a scale from 0 to 1, but STI represents the percent of speech which is understood as the acoustic signal travels from the loudspeaker to the measurement location. An STI rating of 0.0 corresponds to 0% of the speech being understood, and 1.0 corresponds to all speech being perfectly understood.
Opposite to the findings about overall sound level, we found that the Mycelium Sail is useful in creating a localised zone with better conditions for listening and understanding speech coming from other parts of the room. This is even more true for sounds originating from under the sail itself.
These results show that locating the sail over an audience (whether the presenter is also under the sail or not) can have the positive effect of “balancing” speech intelligibility across the whole room. This is particularly useful for improving intelligibility for audience members who are furthest away from the presenter. This can be used, for example, to improve the acoustics in classrooms and seminar rooms, helping even the students in the furthest seats to understand the words of the presenter.
Survey results
The results of the online visitor survey showed some clear trends directly in the numeric responses, without the need for further analysis. Two clear examples are shown in the distribution of responses to the questions “which of your five senses are most effected by the sail?” (see Figure 15) and “do you find the sail to be more artificial or natural?” (see Figure 16).
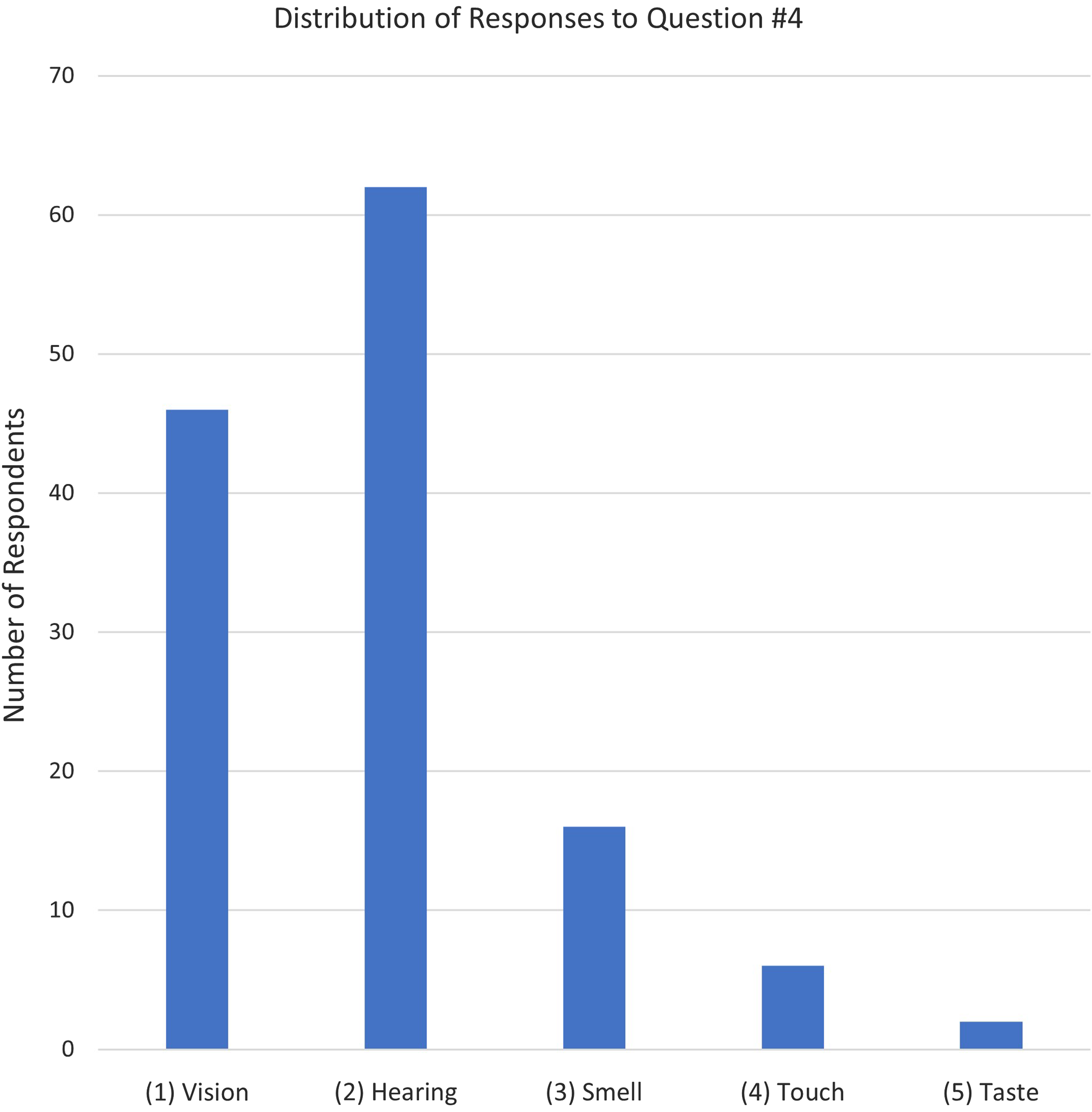
Figure 15. Distribution of survey responses, “which of your five senses are most effected by the sail?”.
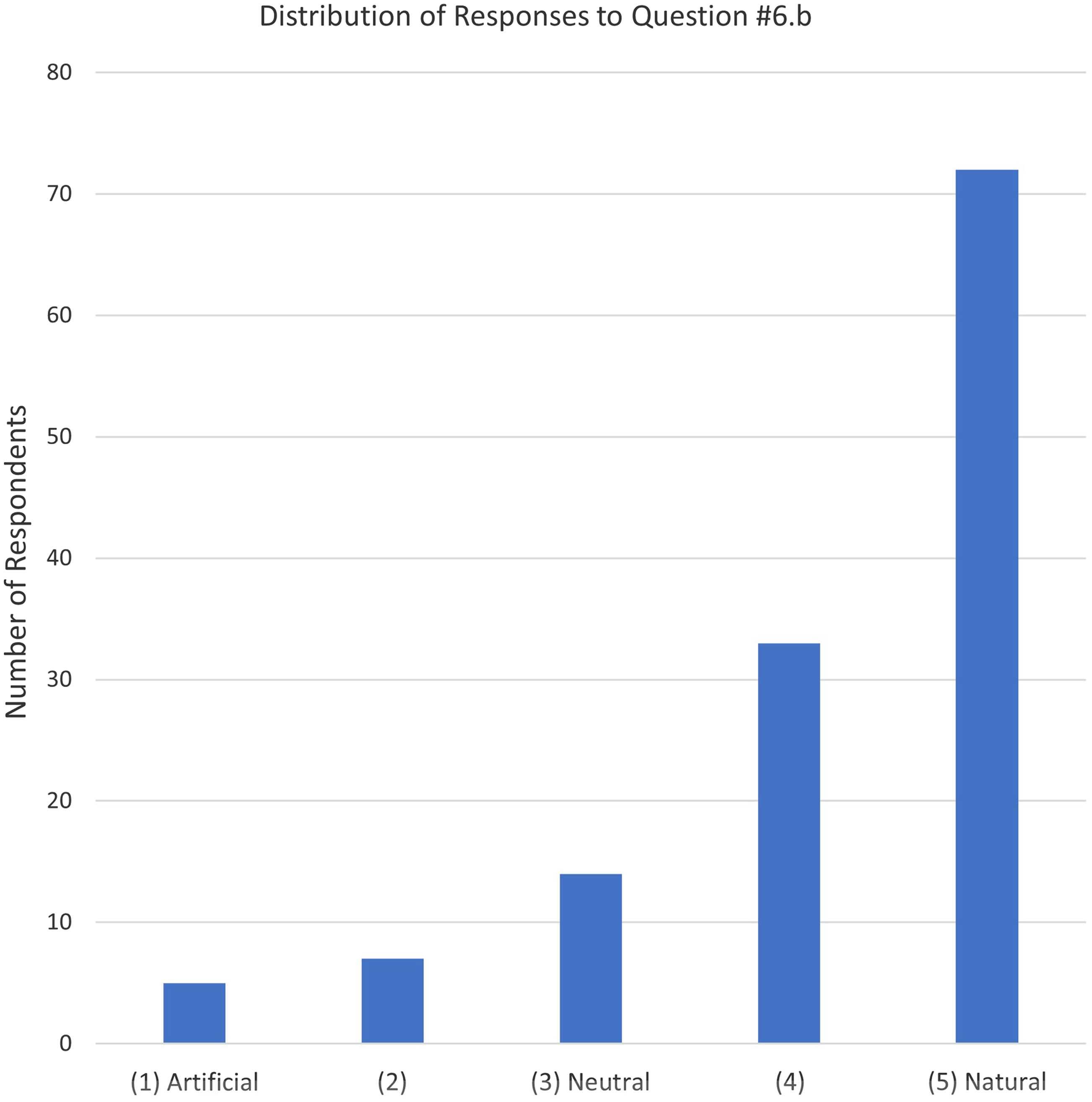
Figure 16. Distribution of survey responses, “do you find the sail to be more artificial or natural?”.
The majority of respondents felt that the sail primarily effected their hearing or vision, and the majority of respondents also felt that the sail was more natural than artificial.
However, other questions do not show such clear distribution of responses. The distribution of responses to question 6a “do you find the sail pleasant” (see Figure 17) and question 6b “is it more familiar or new” (see Figure 18) do not show any clear trend and were difficult to interpret without deeper analysis.
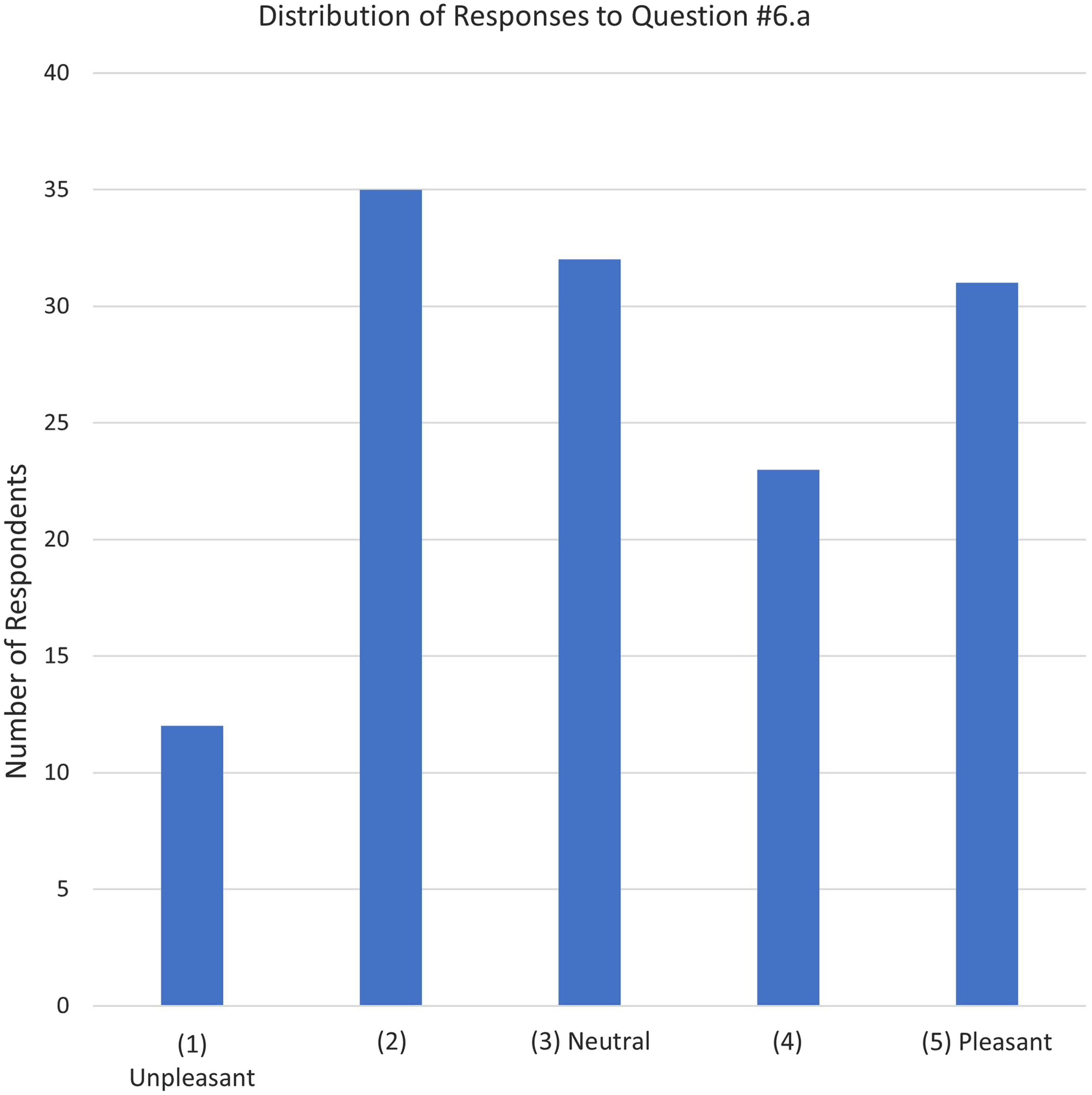
Figure 17. Distribution of survey responses, question 6a “do you find the sail to be pleasant or unpleasant?”.
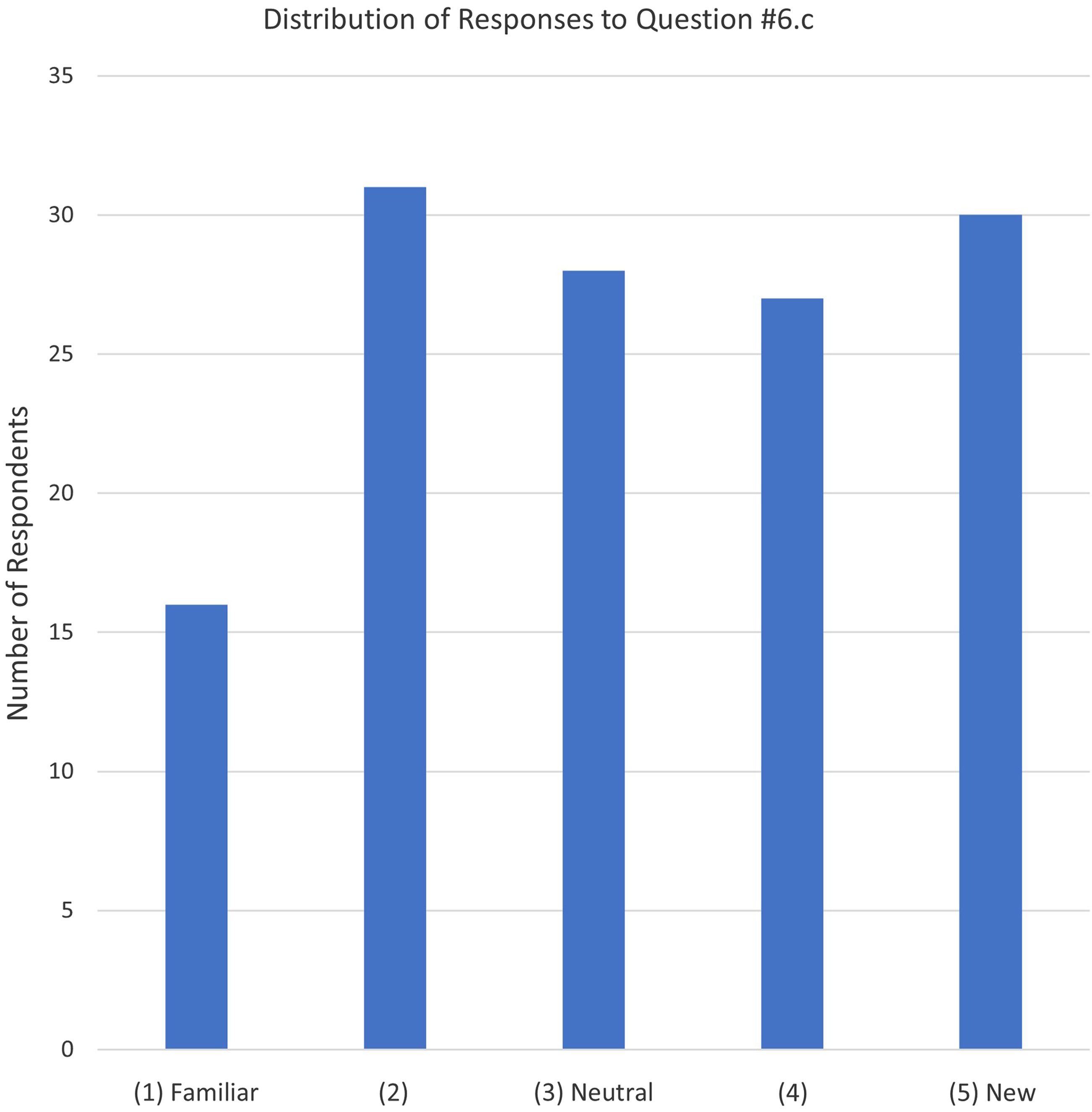
Figure 18. Distribution of survey responses, question 6b “do you find the sail to be more familiar or new?”.
The Pearson correlation analysis (Kirch Reference Kirch2008), in combination with the syntactic linguistic analysis of the text responses, identified some factors which clarified the trends driving responses to these two questions. The most significant correlations are summarised in Table 4.
First, there was a moderately high correlation between the results of the syntactic linguistic analysis of the text responses along the axis “pleasant to unpleasant” and the self-reported numeric responses about pleasantness of the sail (p = 0.64), indicating that the text responses have a reliable explaining power for the odd distribution of numeric responses to the “pleasantness” question.
The aspects of the text responses with the highest “pleasantness” ratings in the linguistic analysis involved learning, fascination, the unexpected improvements that the sail had on visitor experience, as well as personal interest in sustainability. The negative dimension of “unpleasantness” was driven by affective evaluations relating to personal health – in short, that the visitor was suspicious of the material, and felt unsafe around it, because of the possible side effects of mould exposure.
There was also a low-to-moderate correlation between participants who reported being stressed or uncomfortable at the beginning of the survey, those who gave a low numeric score for “pleasantness of the sail”, and those who reported high noise levels in the room. This indicates that the presence of distracting conversations, as well as pre-existing feelings of stress or discomfort, have a direct impact on whether the visitor found the sail pleasant or not.
In all cases, negative evaluations of the sail oriented around personal safety and were moderately correlated with the senses Touch, Taste and Smell (p = 0.41). Positive evaluations oriented around positive emotional effects (feeling calmed, peaceful) as well as positive social outcomes (better sustainability, learning about alternatives) and were more strongly correlated with the visual and auditory senses.
Interestingly, the “familiarity vs. novelty” dimension also showed correlation with both the “natural” and “pleasantness” dimensions, such that a stronger familiarity with physical aspects of nature were viewed negatively (examples including rot, mould and disease). Unfamiliar natural aspects of the sail were more affective, including feelings of peace and calm, shelter, as well as ability to focus or reduce stress. This demonstrates that “natural” objects are not always seen positively – and health hazards in nature can be very familiar preconceptions.
These survey results suggest that the acceptance of mycelium composite materials depends on a combination of contextual factors. First, the underlying experience of the visitor (showing up stressed vs. calm) plays a role in whether they will view the material positively or negatively. Similarly, the presence of distracting sounds or too many people can reduce acceptance. A peaceful, calm environment can help people feel open to the positive benefits of the material for sustainability and society – and helps them overcome anxiety about personal health or feelings of disgust.
There were also clear examples in the text responses of the acoustic properties being accurately perceived. Many respondents commented that the room was quieter under the sail, and they were more able to clearly understand speech within their group. This was not reported by all respondents, however, as the conditions for being affectively and cognitively open to such observations were not available in all cases (namely, when the room was too crowded, or the visitor had an underlying feeling of stress).
Considering mycelium composite materials as an enclosure for a human habitat should take these results into account. The acoustic effects of the sail can be perceived by members of the public, but only under relaxed conditions, and most strongly when their attention is oriented towards affective dimensions of sustainability – including peace, calm, shelter and learning.
Conclusions
The Myx Sail demonstrates the technical feasibility to construct architectural enclosures and spaces with “Mycelium Textiles”, composed of different layers of plant fibres to achieve a light-weight composition.
Mycelium Textiles can be flexible and strong building materials with a high acoustic absorbance. The modular structure of the Myx Sail is to date one of the largest hanging objects made from MBC materials, showcasing the structural integrity of the specific build-up of the inoculated mycelium sheets.
While many material combinations are possible to compose the substrate of Mycelium Textiles, fibre strength, nutrient content and stability during the wet growth stage are key parameters for material selection to achieve a performing MBC. The final material selection of hemp, jute and wood wool for the Myx Sail proved to successfully combine strength, flexibility and a good nutrient base for the cultivation of MBC.
The control over air and CO2 exposure inside the mould during final growth stage is a critical element for avoiding fruiting and achieving a desired outcome and material performance, requiring further understanding and research. Further improvements in the acoustic properties of the dehydrated material are likely to be related to the inoculation, composition, sizing and placement of woodwool fibres that as a scaffold organise the growth of the acoustically absorbing mycelium foam structure. Future research on growing Mycelian Textiles on site would allow to overcome the size retractions of the described biofabrication process and the need for a modular construction potentially leading to a new architectural expression and technical performance of grown building structures.
How would it be to live in such a grown building? The results of the acoustic investigation indicate that, if planned properly, a grown building has the potential to create a physically comfortable and quiet environment. The acoustic conditions can be optimised for a variety of usage scenarios, offering high speech intelligibility (for classrooms or meeting places) and reduction of noise levels (for workshops or other noisy spaces).
Understanding the subjective dimension of comfort in or under a grown structure is more complex than the physical dimension. The results of this investigation indicate that the degree to which a grown surface (and by extension, a grown building) is perceived positively or negatively depends on several interconnected contextual factors. Positive perceptions of the Myx Sail were most clearly associated with fascination, learning and a personal commitment to sustainability – and this cognitive orientation allowed survey participants to evaluate their sensations more openly, leading them to perceive a calm, quiet and sheltering effect from the sail. Negative perceptions were primarily rooted in an anxiety about health risks from natural materials such as mould and fungus, which were often described as familiar or well-known risks. These preconceptions tended to overpower the visitor’s sensory observation of the acoustic benefits.
This suggests that people who have a strong personal commitment to sustainability and new ways of living may automatically find a grown house to be comfortable. This does not exclude the possibility that other people would also find it comfortable – however, the content of the negative responses indicates that there is work to be done in changing the “familiar story” about the relationship between nature and humans. Grown buildings would not pose a medical risk to human health – they could, in fact, improve the health of our environment and by extension, our bodies. Shifting the narrative about health risks from natural materials can begin with scientific investigations (such as the present study) but will need to be taken forward into the public realm through interventions which change common discourse and alleviate anxieties about our bodies coming into contact with materials fabricated by other living organisms.
Data availability statement
The authors confirm that the data supporting the findings of this study are available within the article.
Acknowledgements
The authors thank the following for their support and contributions: Pernille Stockmarr (DDM), Nicole Perez, Joachim Klein, Victor de Tejada Molera (all current or former employees of Arup), Jeanne Sarton Du Jonchay, Freja Kræmmer, Benedikt Trojer (personal assistants to Jonas Edvard during project duration in chronological order).
Author contribution
We confirm that all listed authors have contributed substantially to the related research and the drafting and reviewing of this article and have agreed on the final version for publication. We have applied accuracy, care and integrity in drafting this publication and are accountable for all aspects of the work.
Financial support
Myx Sail material development was funded by Fritz und Trude Fortmann Stiftung. The Myx Sail production and installation was funded by The Danish Art Foundation and Nationalbankens Jubilæumsfond. Acoustic Testing, Design and Analysis by Arup were funded internally by “Invest in Arup”.
Ethics statement
Ethical approval and consent are not relevant to most aspects of this research and article, except for the public survey. The survey and analysis were conducted using a secure data management platform, in compliance with GDPR (General Data Protection Regulation) requirements. All participants provided explicit consent to participate prior to providing any data, and no identifying information was recorded.
Comments
No accompanying comment.