INTRODUCTION
Worldwide, people have developed different canal and irrigation systems to manage their water supply in response to environmental or climatic changes or increased agricultural activities (Jacobsen Reference Jacobsen1960; Gibson and Downing Reference Gibson and Downing1974; Hole Reference Hole, Stein and Rothman1994; Cremaschi et al. Reference Cremaschi, Mercuri, Torri, Florenzano, Pizzi, Marchesini and Zerboni2016; Nissen Reference Nissen2016; Lang and Stump Reference Lang and Stump2017; Cremaschi et al. Reference Cremaschi, Degli Esposti, Fleitmann, Perego, Sibilia and Zerboni2018; Cajigas et al. Reference Cajigas, Quade and Rittenour2020). Water management is considered an important technological step in human history and is viewed as one of the “prime movers” behind the emergence of the earliest urban societies in Mesopotamia (Crawford Reference Crawford2004; Rost Reference Rost2017) which brought about many inventions we recognize as part of our own societies today (e.g., stratified society, a complex administrative system, writing, armies, laws) (Postgate Reference Postgate1992; Algaze Reference Algaze1993; Crawford Reference Crawford2004; Algaze Reference Algaze2008a). Understanding the timing of the development of canal and irrigation systems (water management practices) in ancient Mesopotamia is therefore a fundamental research objective.
Archaeological and textual records from Mesopotamia have been exerted to address this topic (Jacobsen Reference Jacobsen1960; Algaze Reference Algaze2008b; Rost Reference Rost2017; Schrakamp Reference Schrakamp2018; Borrelli Reference Borrelli2020). The earliest textual evidence for fully developed irrigation networks and their cuneiform terminology dates from the Early Dynastic IIIb/Presargonic period and stems from the Sumerian city-state of Lagash (ca. 2475–2315 BC) (Schrakamp Reference Schrakamp2018) (Table 1 for chronology). Earlier textual indications of irrigation systems are probably masked by the ambiguity of early cuneiform writing (Nissen Reference Nissen2016; Schrakamp Reference Schrakamp2018). The EDIIIb and later texts show that at least by ∼2400 BC sophisticated “hydraulic landscapes” (cf. Wilkinson et al. Reference Wilkinson, Rayne and Jotheri2015:398) formed an integral and key part of the Mesopotamian world (as irrigation system, waterways between cities, (symbolic) delineation of space, as element in warfare and the confirmation of power) (Jacobsen Reference Jacobsen1960; Algaze Reference Algaze2008b; Rost Reference Rost2017; Schrakamp Reference Schrakamp2018; Borrelli Reference Borrelli2020).
Table 1 Chronology of ancient southern Mesopotamia. This table is based on extensive bibliography, the main works are referred to here. Dating for the 6th to the 4th millennium (Ubaid and Uruk phases) is essentially based on the workshop “Delineating the End of a World: Reassessing the Ubaid/post-Ubaid Transition in Greater Mesopotamia” (Baldi, Abu Jayyab Reference Baldi and Abu Jayyab2022) and on new data from Uruk (Van Ess, Heußner Reference Van Ess and Heußner2015). Both base the chronology on new data obtained from 14C samples. The 3rd–1st millennium BC is largely based on the Middle Chronology II proposed by W. Sallaberger and I. Schrakamp for the ARCANE project (Sallaberger and Schrakamp Reference Sallaberger, Schrakamp, Sallaberger and Schrakamp2015).

With limited access on the ground for research projects due to decades of war in Iraq, much of the Mesopotamian landscape has been studied based on remote sensing data (Hritz and Wilkinson Reference Hritz and Wilkinson2006; Hritz Reference Hritz2010; Wilkinson et al. Reference Wilkinson, Rayne and Jotheri2015; Jotheri Reference Jotheri2016). This, combined with results from earlier archaeological surveys (Adams Reference Adams1965; Adams and Nissen Reference Adams and Nissen1972; Adams Reference Adams1981) has provided a fascinating dataset for Mesopotamia, which presents a complex picture of landscape evolution and human-environment interactions over millennia, spanning the rise of urbanism and the development of the earliest water engineering efforts. In search for a chronology, archaeological survey data has been used as a proxy for estimating the age of Mesopotamian landscape features. For example, the alignment of contemporaneous sites, dated based on cultural/typological chronologies of surface finds, revealed shifting settlement patterns which were inferred to reflect shifting watercourses (Adams Reference Adams1965; Adams and Nissen Reference Adams and Nissen1972; Adams Reference Adams1981). However, without direct dating, integrating palaeo-environmental, geological/geomorphological and archaeological records and distilling interactions, relationships and causality, whilst avoiding circular reasoning, is challenging.
Now that international fieldwork projects are returning to Iraq, direct dating ancient (hydraulic) landscapes and palaeo-environmental records, as well as building an up-to-date 14C database for archaeological evidence, are research priorities to provide a chronology for human-environment interactions and the pivotal processes that led to the emergence of urbanism and the earliest civilizations in Mesopotamia and offer an up-to-date dataset against which pervious theories can be tested.
We present here our efforts of dating the canal system of Girsu, one of the four main cities of the Lagash state (ca. 2475–2315 BC) (Table 1 presents a cultural chronology of southern Mesopotamia), using archaeological and 14C methods, and discuss the versatility of both methods for this context.
BACKGROUND
The site of Tello/ancient Girsu is situated about 55 km north of the modern city of Nasiriyah, in the ThiQar Province in southern Iraq, between the modern Euphrates and the Tigris (Figure 1). The floodplains of southern Mesopotamia consist of 15–20 m of Holocene fluvial deposits (Yacoub Reference Yacoub2011). Relics of anastomosing rivers, forming a micro-topography of floodbasins and levees, characterize the landscape. Girsu and many other famous sites in Mesopotamia are found along such relic levees (Adams Reference Adams1965; Adams and Nissen Reference Adams and Nissen1972; Adams Reference Adams1981) and are clearly visible in the landscape (the tell of Girsu rises ∼15 m above the floodplain). Unfortunately, the marsh settlements that must have existed in the floodbasins are deeply buried and mostly unknown (Oates Reference Oates1960; Jotheri et al. Reference Jotheri, Feadha, Al-janabi and Alabdan2022).

Figure 1 Map of Mesopotamia (modern Iraq), showing the location of Girsu in relation to the modern Euphrates and Tigris Rivers. Detailed map shows Girsu and the main archaeological sites in the region (illustration prepared by the author. Sources: High Resolution Shaded Relief (naturalearthdata.com), waterways (diva-gis.org), JAXA DSM).
French diplomat and archaeologist Ernest de Sarzec rediscovered Girsu in the 1870s and started excavations at the site in 1877. He continued until 1900, after which Gaston Cros took over (1903–1909). Cros was followed-up by Henry de Genouillac (1929–1931) and André Parrot (1931–1933). These early excavations provided the first tangible evidence of the Sumerian civilization as well as large quantities of finds from the Ubaid 4 to the Parthian periods (Cros et al. Reference Cros, Thureau-Dangin and Heuzey1910; Sarzec and Heuzey Reference Sarzec and Heuzey1912; Genouillac Reference Genouillac1936; Parrot 1948), now on display in many of the world’s largest museums. The British Museum has resumed excavations since 2016, focusing on archaeological research (including re-contextualizing previously excavated material), training and collaboration, heritage management and site conservation.
The oldest evidence of human presence at Girsu dates to the Ubaid 4 period (4900–4500 BC) (Genouillac Reference Genouillac1936; Parrot 1948). Recent discoveries suggest a complex socio-cultural settlement, which further increased during the following Uruk period (4200–3100 BC) (Genouillac Reference Genouillac1936; Parrot 1948; Rey Reference Rey2019). There seems to be a gap between the Uruk occupation and the Early Dynastic I (ED I) (2900–2600 BC) (Postgate Reference Postgate1986; Algaze Reference Algaze1993). The Early Dynastic period appears to be the most significant one at Girsu; an urban center and vast religious complex developed during this phase. Girsu became the capital of the Lagash Kingdom during the second dynasty of Lagash (2200–2100 BC) and remained the religious center of the state after political power shifted to the city Lagash. Girsu declined with the fall of Ur (ca. 2000 BC) and appears abandoned at the beginning of the Old Babylonian period (1800–1700 BC) with a short reuse of the site during the 3rd century BC.
A key landmark at Girsu is the “bridge,” which once crossed canal 3 (Figure 2, inset). Its construction is dated to the Early Dynastic and it stayed in use until the abandonment of the site after the Isin-Larsa period. It was partly excavated for the first time between 1929 and 1930 by De Genouillac (Genouillac Reference Genouillac1936). Later excavations under Parrot exposed the full structure (Parrot 1948). He provisionally called it a water regulator, but later identified it as a pseudo-tomb for a priest-king (Parrot 1948). Later scholars reinterpreted the structure i.a. as a weir (Jacobsen Reference Jacobsen1960), or canal regulator (Wilkinson Reference Wilkinson and Crawford2013), as a bridge (Margueron Reference Margueron2005; Rey and Lecompte Reference Rey and Lecompte2020), and most recently as a “throated flume” (Parshall flume) avant la lettre (E. Torun, pers. comm. 2021) in which the hydraulic feature also functioned as a bridge. Based on cuneiform textual evidence the bridge has also been interpreted as the head gate or reservoir/bridge of the well-known íd-NINA-šè-DU canal (“the canal going to Nina”), built by Urukagina in his 2nd regnal year (see Rey [Reference Rey2016] and Rost [Reference Rost2011] for a further discussion on the location of the Nina canal and its head gate).
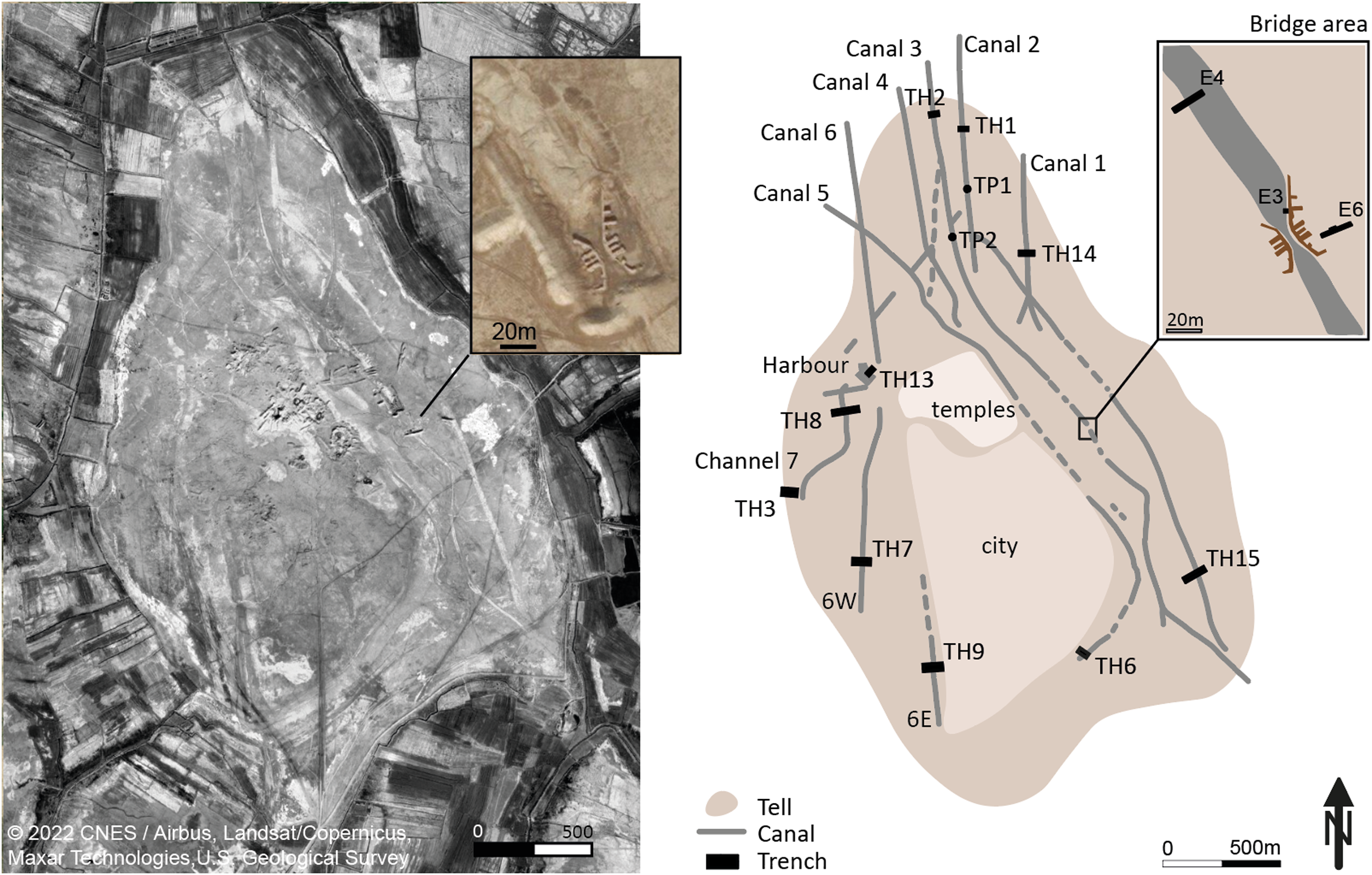
Figure 2 Google satellite image (2022) (gray scale by lightness and enhanced contrast) of Girsu with an inset close-up of the bridge area (left). Illustration of the main canals identified at Girsu, and the location of the excavation trenches (right) (illustration prepared by the author) (See for a more detailed discussion Jotheri et al. submitted).
SAMPLE COLLECTION
Radiocarbon dating of shell and charcoal samples collected from fluvial palaeo-canals around Girsu is part of an on-going multidisciplinary research program investigating the palaeo-environment of the ancient city (Egberts et al. submitted). Remote sensing analysis and field walking has provided a detailed palimpsest map of the cities’ waterscape, as well as further insights in the layout of the urban area (Jotheri et al. submitted).
We selected trench locations based on our remote sensing data and field observations. Geoarchaeological trenches were excavated across sedimentary sequences to provide stratigraphic cross sections of old canal sediments, lake, and floodplain deposits. All sections were recorded using traditional sedimentological logging techniques and photography. The locations were recorded with a Leica differential global positioning system (DGPS). Environmental, geochemical and 14C samples were obtained from suitable deposits using conventional sampling methods (Campbell et al. Reference Campbell, Moffett and Straker2011; Jotheri et al. Reference Jotheri, Allen and Wilkinson2016). J. Jotheri identified the shell samples. All 14C samples were wrapped in aluminum foil, labeled and sealed in plastic bags.
A total of 15 14C samples were collected from 7 different palaeo-canals around Girsu (Figure 2). Samples were collected during excavation and selected from key stratigraphic units. Two samples were collected from ancient lacustrine sediments discovered in the western part of the tell (Figure 2), interpreted as harbor.
Three excavations (E3, E4, E6) were conducted around the ancient bridge (Figure 2, inset). Area E3 consisted of a 2×2 m stratigraphic excavation, placed at the base of one of the bridge walls. The aim was to establish the depth of the bridge foundations and the nature of the underlying deposits. Undisturbed archaeological deposits were found below 180 cm of backfill and slope wash, which accumulated after the French excavations ceased in the bridge area in the 1930s. A dark silt layer including pottery was found directly below the bridge wall (context 13002). Below this, 15–20 cm pale, compact, clean silt was found (context 13003) covering a mudbrick wall (context 13006). The wall enclosed an internal space, which was filled with collapse, covering in situ pottery, charcoal, burnt animal bone, shell fragments, burnt mudbrick and a ceramic sickle fragment. Artifacts and pottery from this context date to Ubaid 4 (4900–4500 BC). From this context charcoal and shell 14C samples were collected.
Trench E4 was excavated to the northwest of the bridge, across the westbank of canal 3. The canal fill consisted of 300 cm normally graded, moderately compact sand and silt, with increasing anthropogenic inclusions and ceramics dating to the Late Akkadian/Isin-Larsa period (2200–1800 BC) and Old Babylonian Period (1800–1600 BC). Deposits cut by canal 3 contained in situ oven structures (tannurs) and ceramics dated to Ubaid 4.
E6 was excavated as a stepped trench against the oblique west-facing slope of the French excavation cut. Ubaid 4 deposits were exposed at the base of the trench, ∼3.3 m below ground surface (8.28 m asl) (context 12156). Overlying Ubaid deposits appear cut during the initial bridge construction. The fill of this cut yielded late third millennium BC pottery and occasional residual Uruk material. This was covered with 230 cm of deposits dated to the Isin-Larsa period (2000–1800 BC).
METHODOLOGY
All samples were analyzed by Beta Analytic by accelerator mass spectrometry (AMS). Pretreatment and analysis were conducted following standard protocols (Beta Analytic 2022). Shell samples were first washed with deionized water. The material selected for 14C dating was then crushed/dispersed, and repeatedly subjected to HCl etching. The pretreatment of the charred material consisted of an acid/alkali/acid or acidic washes (Dunbar et al. Reference Dunbar, Cook, Naysmith, Tripney and Xu2016) (Table 2). δ13C and δ18O were obtained by Beta Analytic using isotope ratio mass spectrometry (IRMS) (Beta Analytic 2022). All dates were calibrated with OxCal v 4.4.4 (Bronk Ramsey Reference Bronk Ramsey2021), using the IntCal2020 Northern Hemisphere calibration curve (Reimer et al. Reference Reimer, Austin, Bard, Bayliss, Blackwell, Ramsey, Butzin, Cheng, Edwards, Friedrich and Grootes2020). Sigmas and conventional radiocarbon ages are rounded to the nearest 10 years. When counting statistics produced sigmas lower than ± 30 years, a conservative ± 30 BP is cited for the result.
Table 2 AMS 14C dates, presented per canal and in stratigraphic order per trench; heights are in meters above sea level. The calculation of the used FRE is discussed in the text.

Chronological Framework
The chronological framework proposed here is based on geomorphological and archaeological interpretations and is a working hypothesis. The model includes the stratigraphic relationship between samples in case it could be directly observed in section, and dates BC in case the samples could be directly associated with datable archaeological evidence. In addition, a relative chronology for channel activity was reconstructed based on analysis of remote sensing data, the high-resolution DEM of the site (see Egberts et al. submitted), and field observations. Phases, sequences, and boundaries are used to represent these chronological constraints, as defined in Bronk Ramsey (Reference Bronk Ramsey2009). Bayesian modeling has been performed using OxCal v 4.4.4 (Bronk Ramsey Reference Bronk Ramsey2021) and the IntCal20 calibration curve (Reimer et al. Reference Reimer, Austin, Bard, Bayliss, Blackwell, Ramsey, Butzin, Cheng, Edwards, Friedrich and Grootes2020). Figure 3 shows a schematic representation of the Bayesian model.
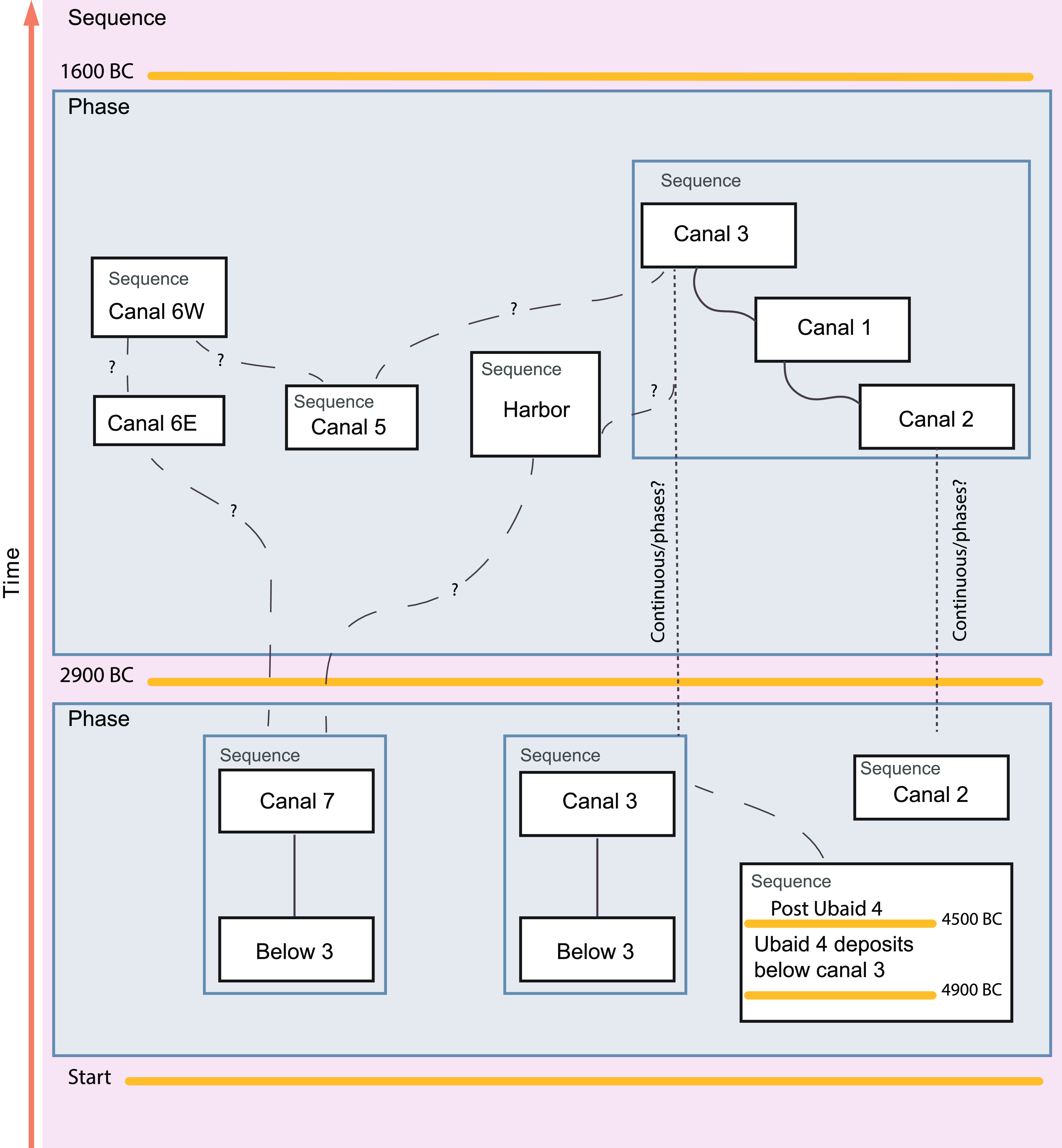
Figure 3 Schematic representation of the OxCal Bayesian model used in this study. Orange lines represent dated boundaries. Solid lines represent direct and stratigraphic relationships within channels, dashed lines represent relative chronological relationships based on remote sensing and field observations.
A two-phase sequential model is applied. In this framework no hiatus is assumed between the two main phases. Within the main phases some of the dates could be ordered according to stratigraphic or archaeological constraints, or according to the reconstructed relative chronology (e.g., the final activity of canal 2, 1 and 3). Samples in the first phase are from below the channel belts and from the earliest phases of channel activity. The second main phase contains all the samples from the canal and lacustrine sediments that were associated with archaeological evidence.
RESULTS
Results are summarized in Table 2. The date list below presents unmodeled calibrated 14C results.
Canal 1

Fossil Corbicula fluminea shell obtained at 60 cm below ground surface (6.7 m asl). The shell was collected from a thin, finely bedded silt and clay layer within a ∼120 cm thick, cross-laminated sand and silt unit filling canal 1. Preceding canal deposits and reddish brown floodplain deposits underlie and are cut by canal 1. Collected 2019 by E. Egberts and J. Jotheri; submitted by E. Egberts and J. Jotheri.
Comment: The date overlaps with a 14C date (carbonized seed [MC-2386]) at Oueili (Huot et al. Reference Huot, Calvet, Chevalier and Forest1981) from layer Level 2, related to the Ubaid 4 (4900–4500 BC). All pottery recovered from trench TH14 was found below the canal fill and dates to the Ur III/Isin Larsa period (2100–1800 BC). The 14C sample is older than the archaeological evidence obtained from below the canal fill. Considering the freshwater reservoir effect, this sample provides a terminus post quem for canal 1 infilling at Girsu.
Canal 2

Fossil Corbicula fluminea shell obtained at 40 cm below ground surface (7.9 m asl) collected from a 70 cm thick, horizontally bedded fine sand unit filling canal 2. Collected 2018 by E. Egberts and J. Jotheri; submitted by E. Egberts and J. Jotheri.
Comments: The date overlaps with a 14C date at Basra (organic rich sediments, sample AA94339) (Hritz et al. Reference Hritz, Pournelle, Smith, Albadran, Issa and Al-Handal2012), A 14C date at Oueili, from Z28 Level 1 (charcoal, sample MC-2382) (Huot et al. Reference Huot, Calvet, Chevalier and Forest1981) and a 14C date at Uruk, from remains of reed from the deepest strata in Eanna, lying on natural soil (reed, sample H138-123) (Münnich Reference Münnich1957). No cultural material was recovered from this trench. This sample provides a terminus post quem for canal 2 infilling at Girsu.

Fossil Melanoides tuberculata shell obtained at 80 cm below ground surface (7.6 m asl) and collected from a homogeneous, bedded sand unit filling canal 2. The base of the canal fill was not reached and no cultural material was recovered from this trench. Collected 2018 by J. Jotheri; submitted by E. Egberts and J. Jotheri.
Comment: This date is stratigraphically consistent with 508712/TH1-SH4-40 and 545369/TH15-SH2-35, also obtained from canal 2 fill. This date overlaps with charcoal at Oueili, Z28 Level 1 (sample MC-2382) (Huot et al. Reference Huot, Calvet, Chevalier and Forest1981) and with reed from the deepest strata in Eanna at Uruk (H138-123) (Münnich Reference Münnich1957). Considering the freshwater reservoir effect, this sample provides a terminus post quem for canal 2 infilling at Girsu.

Fossil Melanoides tuberculata shell collected at 35cm below ground surface (8.05 m asl) from a 200 cm thick well sorted, horizontally and cross-bedded sand unit filling canal 2. A cultural layer below the canal fill (∼6.4 m asl) consisted of compact reddish brown clay and yielded ceramic fragments. Collected 2019 by E. Egberts and J. Jotheri; submitted by E. Egberts and J. Jotheri.
Comment: This date is stratigraphically consistent with 515278/TH10-SH1-80 and 508712/TH1-SH4-40, also obtained from canal 2. The 14C date overlaps with a 14C date at Nippur from Level VIIB, related to the Early Dynastic II (charcoal, sample P.806) (Stuckenrath and Ralph Reference Stuckenrath and Ralph1965). Archaeological material obtained from a cultural layer below the canal dates to th UrIII/Isin-Larsa period (2100–1800 BC). This 14C age pre-dates the archaeological evidence obtained from below the canal fill. Considering the freshwater reservoir effect, this sample provides a terminus post quem for canal 2 infilling at Girsu. Compare with shell 14C sample from TH6 (sample 508716/TH6-SH5-120, see below), showing an overlapping age range.
Below the bridge and Canal 3

Two charcoal samples (553725/13007_TG33 and 555135/13009_TG35) and a fossil Corbicula fluminea shell (553724/13012_TG38) collected from context 13005 (at 6.1 m asl). Collected 2017 by E. Egberts; submitted by E. Egberts.
Comments: the charcoal samples are in good agreement and overlap with the age of the archaeological evidence. The ceramic sickle is typical for the Ubaid period and all pottery from context 13005 dates to the Ubaid 4 (4900–4500 BC). They are also stratigraphically in good agreement with charcoal samples from E4 and E6 (see 545371/TG-E6 and 515281/16006). The shell 14C date also overlaps with the age of the archaeological evidence but dates older than the charcoal samples. 55372513007_TG33 and 555135/13009_TG35 overlap with a 14C date at Oueili (carbonized seed, sample MC-2384) from Y28 black ashy layer Level 2, associated with Ubaid 4 (4900–4500 BC) (Huot et al. Reference Huot, Calvet, Chevalier and Forest1981). 553724/13012_TG38 overlaps with a 14C date from reed at Uruk (H138-123) (Münnich Reference Münnich1957) and a 14C date from charcoal at Oueili (sample MC-2382), related to Z28 Level 1 (Huot et al. Reference Huot, Calvet, Chevalier and Forest1981).
The charcoal samples provide a 14C date for Ubaid 4 occupation at Girsu. It is certain that Ubaid material discovered in the 1930s (Genouillac Reference Genouillac1936; Parrot 1948) comes from the same or closely associated occupation deposits.

Charcoal collected at ∼3.3m below ground surface (8.28 m asl) from an occupation deposit (context 12156) which yielded Ubaid 4 pottery. Collected 2019 by E. Egberts and T. Baxter; submitted by E. Egberts and J. Jotheri.
Comment: this date provides a 14C date for intact Ubaid 4 occupation deposits. The date overlaps with a 14C date from Ubaid 4 deposits at Oueili (carbonized seed sample MC-2384) (Huot et al. Reference Huot, Calvet, Chevalier and Forest1981). It is highly likely that Ubaid 4 material discovered in the 1930s (Genouillac Reference Genouillac1936; Parrot 1948), comes from similar deposits. Although no lateral relationship could be established between Ubaid 4 deposits in E3 and E4 due to previous French excavation cuts, in terms of absolute height (m asl) the dates are generally in stratigraphic agreement.

Fossil shell Melanoides tuberculata collected from 320 cm below the surface (10.26 m asl) from bedded sandy silt. Collected 2018 by E. Egberts and T. Baxter; submitted by E. Egberts and J. Jotheri.
Comments: This date overlaps with sample p.798 from Level IXB low, at Nippur, ED I, and with sample p.810 from Level V, ED II/III (Stuckenrath and Ralph Reference Stuckenrath and Ralph1965). It also overlaps with charcoal at Girsu UGAMS-8185 (G-1) from the Maison de Fruits (Hritz et al. Reference Hritz, Pournelle, Smith, Albadran, Issa and Al-Handal2012).
Considering the freshwater reservoir effect, 515280/16014-SH2-320 provides a terminus post quem for canal 3 infilling at Girsu. Archaeological material from the overlying sediments dates to the Isin/Larsa period (2000–1800 BC).

Charcoal collected from the oven structure exposed in the bank of canal 3, at 10.36 m asl. Collected 2018 by T. Baxter; submitted by E. Egberts and J. Jotheri.
Comments: This date overlaps with a 14C date for Ubaid 4 at Oueili (carbonized seed, sample MC-2384 (Huot et al. Reference Huot, Calvet, Chevalier and Forest1981). Sample 515281/16006 provides a 14C date for intact Ubaid 4 deposits at Girsu. Although no lateral relationship could be established between Ubaid 4 deposits in E3 and E4 due to previous French excavation cuts, in terms of absolute height (m asl) the dates are generally in stratigraphic agreement.
Canal 3

Fossil Corbicula fluminea shell collected at 145 cm below ground surface (6.75 m asl) from a reddish brown clay unit below a stratified cross-bedded sand unit and normally graded silt and clay units filling canal 3. No cultural material was recovered from this trench. Collected 2018 by E. Egberts and J. Jotheri; submitted by E. Egberts and J. Jotheri.
Comment: This date is stratigraphically consistent with sample 515279/TH12-SH1-40, also from canal 3 infill. The date overlaps with Melanoides tuberculata shell (sample EP3-4) at Eridu (Hritz et al. Reference Hritz, Pournelle, Smith, Albadran, Issa and Al-Handal2012) where the shell is associated with possible Ubaid 2/3 pottery. The sample also overlaps with a shell 14C date at Tell al-Oueili (Valladas et al. Reference Valladas, Evin and Arnold1996) (sample Ly-4550), thought to be associated with Ubaid I deposits. Considering the freshwater reservoir effect, this sample provides a terminus post quem for canal 3 infilling at Girsu.

Fossil Melanoides tuberculata shell collected at 40 cm below ground surface (8.8 m asl) from a homogeneous, bedded sand unit filling canal 3. The base of the canal fill was not reached and no cultural material was recovered from this trench. Collected 2018 by J. Jotheri; submitted by E. Egberts and J. Jotheri.
Comment: This date is stratigraphically consistent with sample 508713/TH2-SH1-145, from the same canal. The date overlaps with a 14C date at Eridu (shell, sample EP3-1) (Hritz et al. Reference Hritz, Pournelle, Smith, Albadran, Issa and Al-Handal2012), and with 14C dated reed remains at Uruk, obtained from “the deepest strata in Eanna lying on natural soil” (Münnich Reference Münnich1957) and with a 14C date at Oueili (charcoal, sample MC-2385) (Huot et al. Reference Huot, Calvet, Chevalier and Forest1981). Considering the freshwater reservoir effect, this sample provides a terminus post quem for canal 3 infilling at Girsu.
Canal 5

Two fossil Corbicula fluminea shells; sample 508715/TH6-SH4-100 was collected at 100 cm below ground surface (8.7 m asl) from a bedded red clay unit interbedded with laterally graded sand lenses filling canal 5. Canal sediments were found overlying compact gray clay, which yielded ceramics, charcoal, shells and bones. Sample 508716/TH6-SH5-120 was collected at 120 cm below ground surface (8.5 m asl) from this cultural layer. Collected 2018 by E. Egberts and J. Jotheri; submitted by E. Egberts and J. Jotheri.
Comment: sample 508715/TH6-SH4-100 overlaps with charcoal at Oueili (samples MC-2383 and MC-2385) (Huot et al. Reference Huot, Calvet, Chevalier and Forest1981), with shell EP3-1 at Eridu (Hritz et al. Reference Hritz, Pournelle, Smith, Albadran, Issa and Al-Handal2012), and with 14C dated reed at Uruk (sample H-138-123) (Münnich Reference Münnich1957). Pottery obtained from the cultural layer below the fill of palaeocanal 5 (from which 508716/TH6-SH5-120 was also obtained), dates to the Ur III/Isin-Larsa period (2100–1800 BC). Both shell samples date older than the archaeological context. The stratigraphic inconsistency between the samples may indicate reworking or variations in the freshwater reservoir effect.
Canal 6E

Charcoal collected at 70 cm below ground surface (8.4 m asl) from reddish brown and gray clay deposits interpreted as levee deposits and irrigation soils. This unit was found stratigraphically above and to the east of well sorted, bedded, medium sand, filling palaeocanal 6E. Stratified clay, silt and sand below and alongside the west bank of paleocanal 6E represent preceding canal and levee deposits. Collected 2018 by E. Egberts and J. Jotheri; submitted by E. Egberts and J. Jotheri.
Comment: This date overlaps with a 14C date (charcoal, sample P.810) at Nippur associated with the EDII/EDIII period (2600–2350 BC) (Stuckenrath and Ralph Reference Stuckenrath and Ralph1965). Ceramics obtained from below the earlier canal deposits date to the Early Dynastic I (2900–2600 BC). Ceramics found adjacent to the second canal belt date to the Isin-Larsa (2000–1800 BC) period.
The 14C date is consistent with the stratigraphic position of the sample, above EDI deposits.
Canal 6W

Fossil Melanoides tuberculata shell (sample 508718/TH7-SH3-100) collected at 100 cm below ground surface (8.05 m asl) from gray clay deposits yielding pottery, charcoal, bone and shell. This cultural layer was stratigraphically below 100 cm of bedded sand filling palaeocanal 6W. A second fossil Melanoides tuberculata shell (sample 508717/TH7-SH2-90) was collected at 90 cm below the ground surface (8.15 m asl), near the base of a stratified, laminated sandy fill of palaeocanal 6W. A cultural layer cut by this palaeocanal yielded ceramics dating to Ur III and the Old Babylonian period (2100–1600 BC). Collected 2018 by E. Egberts and J. Jotheri; submitted by E. Egberts and J. Jotheri.
Comment: the date of sample 508718/TH7-SH3-100 overlaps with a 14C date (charcoal, sample P.806) from Level VIIB, related to EDII (Stuckenrath and Ralph Reference Stuckenrath and Ralph1965). Sample 508717/TH7-SH2-90 overlaps with a 14C date (charcoal, sample P.810) at Nippur from sounding P, Level V, related to the ED II/III (Stuckenrath and Ralph Reference Stuckenrath and Ralph1965). The 14C dates of the shells are stratigraphically consistent. Both samples are older than the archaeological evidence obtained from below the canal fill, which dates to 2100–1600 BC. Considering the freshwater reservoir effect, these samples provide a terminus post quem for canal 6W infilling at Girsu.
Canal 7

Fossil Melanoides tuberculata shell (508720/TH8-SH2-75) collected at 75 cm below ground surface (8.43 m asl), from a sand lens interbedded in reddish brown clay and silt, fill of palaeocanal 7. A second fossil Corbicula fluminea shell (508719/TH8-SH1-60) was collected at 65 cm below ground surface (8.6 m asl), from the same stratified sand unit filling in this palaeocanal. No cultural material was recovered from this trench. Collected 2018 by E. Egberts and J. Jotheri; submitted by E. Egberts and J. Jotheri.
Comment: Sample 508720/TH8-SH2-75 overlaps with a 14C date at Oueili (charcoal, sample MC-2383) (Huot et al. Reference Huot, Calvet, Chevalier and Forest1981). Sample 508719/TH8-SH1-60 compares with a 14C date at Uruk, obtained from the Eanna Temple C-I, associated with the Early Middle Uruk (3900–3500 BC) (Boehmer Reference Boehmer1991). The 14C age difference between the shells is considerable, given their stratigraphic provenance from the same bed. This could indicate general variations in the freshwater reservoir effect, or inter-species variations in FRE. Considering the FRE, these samples provide a terminus post quem for canal 7 infilling at Girsu.

Fossil Corbicula fluminea shell collected at 400 cm below ground surface (4.6 m asl) near the base of a massive reddish brown clay unit. Stratified gray clay and silt was found below this unit. No cultural material was recovered from this trench. Collected 2018 by J. Jotheri; submitted by E. Egberts and J. Jotheri.
Comment: this overlaps with 14C dates at Shatra (sample GX- BB1) B – (11–11.5) m (Aqrawi Reference Aqrawi1995) and with a date at H3 (charcoal, sample AA-42171(GU-9301)) associated with Ubaid 2/3 material (Carter and Crawford Reference Carter and Crawford2002). It also overlaps with sample P1498 at Tell Uqair, probably from House A (Lawn Reference Lawn1973). Considering the FRE, this date indicates that floodplain deposits just outside the tell of Girsu post-date the start of Ubaid 2 and span the period of occupation of the site.
Lacustrine deposits

Fossil Melanoides tuberculata shell collected from stratified gray clay with shell inclusions, at 76 cm (sample 545376/TH13-SH1-76) and 130 cm (sample 545372/TH13-SH6-130) below ground surface (9.54 m and 8.9 m asl, respectively). Green and reddish brown clay were found stratigraphically below the gray clay unit. Collected 2019 by E. Egberts and J. Jotheri; submitted by E. Egberts and J. Jotheri.
Comment: the 14C dates of these samples are stratigraphically consistent but pre-date the archaeological material found in the reddish clay at the base of the trench. This consisted of complete conical bowls and fragmented ceramics dated to the Late Akkadian period (2200–2100 BC) until the end of the Isin-Larsa period (2000–1800 BC), with the majority of the pottery being characteristic of the Ur III period (2100–2000 BC).
Sample 545372/TH13-SH6-130 overlaps with a date at Nippur from ED II deposits (sample P.806) (Stuckenrath and Ralph Reference Stuckenrath and Ralph1965). Sample 545376/TH13-SH1-76 overlaps with a 14C date from reed remains from the ziggurat of Urnammu at Uruk (Münnich Reference Münnich1957). The mat derives from construction work under Urnammu, the first king of the 3rd dynasty, or under his son Schulgi. A period dated by W.F. Albright to between 2070–2000 BC (Münnich Reference Münnich1957).
DISCUSSION
Comparing Radiocarbon Dates and Archaeological Evidence
Table 3 summarizes the results of our radiocarbon dating and archaeological dating evidence. Most 14C dates are stratigraphically coherent, within the individual trenches as well as according to absolute heights across the site.
Table 3 Comparison of 14C dates (modeled and calibrated BC dates (95.4%) and modeled and reservoir corrected calibrated BC dates (95.4%) and archaeological evidence, discussed in this research. Results are presented per association (e.g., below the canal system, associated with canal 3, etc.), per trench, and according to stratigraphic and geomorphological interpretation and increasing height above the floodplain. The diagram on the right shows the age range of shell 14C (white lines), charcoal 14C (dark lines), the reservoir corrected 14C shell dates (gray lines), and the archaeological evidence (orange).

All 14C dates fall between the beginning of the Ubaid 1 and the end of the Early Akkadian period (Table 1 and 2). The earliest 14C dates were obtained from floodplain deposits below the canal system, and pre-date the thus far known earliest occupation at Girsu (from Ubaid 4 [Genouillac Reference Genouillac1936; Parrot 1948]).
The oldest cultural layers excavated are Ubaid 4 deposits found below the bridge and canal 3, at ∼6 m asl to ∼10 m asl. The thickness and variation in elevation of these deposits suggest an Ubaid tell existed at this location. Excavations indicate that the Ubaid deposits were cut during the building of the bridge and the digging of the canal, demonstrating that these hydraulic features obviously post-date the Ubaid 4.
The four charcoal 14C dates obtained from these deposits range from 4691–4502 cal BC (E4) to 4491–4352 cal BC (E6), overlapping with the Ubaid 4/5, consistent with the archaeological evidence. The shell from context 13005 returned a 4999–4803 cal BC 14C date (overlapping with Ubaid 3/4).
The oldest 14C date from the fluvial system was obtained from palaeocanal 7, 5324–5132 cal BC (Ubaid 3). The 14C dates from the canal system range from 5297–5050 cal BC (canal 5) (outlier, see below) and 5206–4853 cal BC (canal 3) to 2871–2581 cal BC (canal 6W), spanning the Ubaid 3 to ED IIIA periods. The youngest 14C date, 2456–2204 cal BC, was obtained from the lacustrine deposits, and overlaps with the Early Akkadian period.
The oldest archaeological evidence found in association with the canal system is ED I (2900–2600 BC) pottery from palaeocanal 6E. The initial construction of the bridge, associated with canal 3, is also thought to date to this period. All other archaeological evidence associated with the canal system dates from the Late Akkadian to the Ur III and Isin-Larsa periods (2200–1800 BC). All shells returned older 14C dates than the age ranges of the associated cultural layers based on pottery chronology (Table 3). The only 14C date (515277/TH9-CH1-70) in broadly stratigraphic agreement with the archaeological evidence and connected with the canal system is from charcoal. Our results show a clear discrepancy between 14C shell dates and the age of the archaeological evidence.
A Relative Chronological Model
Bayesian modeling of the new 14C data from Girsu shows a generally good internal agreement (Table 2 and Supplementary materials). There are two clearly outlying calibrated results (508715/TH6-SH4-100 and 545370/TH14-SH1-60). Already based on stratigraphic, archaeological and geomorphological considerations these results were regarded as problematic. 508715/TH6-SH4-100 was found stratigraphically above the younger sample 508716/TH6-SH5-120. 545370/TH14-SH1-60 was expected to post-date samples from CH2 based on geomorphological observations and was found in association with Isin-Larsa pottery. These samples were therefore marked as outliers in the model.
Archaeological evidence found in association with the 14C samples found in Ubaid levels was used to define a dated upper and lower boundary for this sequence. Sample 545371/TG-E6 from trench E6 is placed after the Ubaid 4 end boundary, based on the pottery from this layer, which included Ubaid 4/5 material. The addition of these boundaries caused sample 1555135/3009_TG35 to fall just below the 60% threshold (A= 59.8%), potentially highlighting some refinements could be made to the cultural chronology of the Ubaid.
The integration of archaeological information was also tested by adding a dated chronological boundary between the two main phases. The date of this boundary was based on the archaeological evidence found in association with the channel fills linked to Phase 2. The pottery from these channel fills mainly dates to 2100–1800 BC, with some material dating to 2900–2600 BC. However, even with a conservative boundary of 2900 BC between Phase 1 and 2 the agreement indices of four samples in Phase 2 (545372/TH13-SH6-130, 508715/TH6-SH4-100, 508718/TH7-SH3-100, TH15-SH2-35) fell below the 60% threshold (0.9%, 0.0%, 2.0%, and 0.1% respectively). Integration of the archaeological evidence in the model again suggests an offset of the shell 14C dates. However, the general internal agreement may indicate that the offset is relatively constant.
Freshwater Reservoir Effect
Several explanations can be put forward for the offset in the 14C shell dates, such as reworking or bad preservation of the used shells. Reworking is not consistent with the generally coherent, though old, 14C dates across the site (Table 3), neither is bad preservation as the selected shells were in good condition. The variations in calibration curves, its wiggles and radiocarbon offsets, though important for correlations with historical events, are of a different order of magnitude (Manning et al. Reference Manning, Barjamovic and Lorentzen2017, Reference Manning, Wacker, Büntgen, Bronk Ramsey, Dee, Kromer, Lorentzen and Tegel2020). The most evident explanation is that the shells are affected by the freshwater reservoir effect (FRE) (Philippsen Reference Philippsen2013; Philippsen and Heinemeier Reference Philippsen and Heinemeier2013). The otherwise good match between the charcoal 14C dates and the archaeological evidence supports this explanation.
Although the FRE is a widely recognized phenomenon (Philippsen Reference Philippsen2013; Svyatko et al. Reference Svyatko, Reimer, Schulting, Shevnina, Logvin, Voyakin, Stobbe, Merts, Varfolomeev and Soenov2022), also for river systems (Philippsen and Heinemeier Reference Philippsen and Heinemeier2013; Yu et al. Reference Yu, Chen, Cheng, Chen and Hou2018), its influence has been mostly dismissed in 14C shell dating in geological and archaeological contexts in Mesopotamia (Hritz et al. Reference Hritz, Pournelle, Smith, Albadran, Issa and Al-Handal2012; Jotheri Reference Jotheri2016; Wilkinson and Jotheri Reference Wilkinson and Jotheri2021) also when clear age differences between shell 14C and cultural evidence existed (Hritz et al. Reference Hritz, Pournelle, Smith, Albadran, Issa and Al-Handal2012). Others recognized the FRE in shell samples and refrained from publishing the 14C data (Zaina Reference Zaina2015).
In general, the number of 14C dates for Mesopotamia is limited (Wencel Reference Wencel2017) and due to poor preservation of organics, and financial considerations, shell radiocarbon dating has remained the go-to method (e.g., Jotheri Reference Jotheri2016; Al Ameri and Briant Reference Al Ameri and Briant2018; Wilkinson and Jotheri Reference Wilkinson and Jotheri2021). Arguments generally put forward in favor of using shells in Mesopotamia diminish the potential impact of FRE for the following reasons (e.g., by Hritz et al. (Reference Hritz, Pournelle, Smith, Albadran, Issa and Al-Handal2012): there are no carbon outcrops in the vicinity of the site (Hritz et al. Reference Hritz, Pournelle, Smith, Albadran, Issa and Al-Handal2012) or are deeply buried (Al Ameri and Briant Reference Al Ameri and Briant2018); correlations or corrections are not possible (no pre-bomb specimens or living specimens available) (Hritz et al. Reference Hritz, Pournelle, Smith, Albadran, Issa and Al-Handal2012:75; Jotheri Reference Jotheri2016:185–186); the marine reservoir effect from the Gulf is considered limited (Hritz et al. Reference Hritz, Pournelle, Smith, Albadran, Issa and Al-Handal2012:75); the environmental context (not a lake or sea) is assumed not to cause a FRE (Hritz et al. Reference Hritz, Pournelle, Smith, Albadran, Issa and Al-Handal2012:75). These are inadequate reasons and dismiss the FRE in river systems. The Tigris and Euphrates Rivers are to our knowledge not discussed with regard to the FRE on radiocarbon ages in the region, even though their importance for life on the floodplain, human history, as well as landscape formation in Mesopotamia is rarely understated. Both rivers cut through several limestone formations before entering the Mesopotamian floodplain and the water of both rivers is classified as hard to very hard (Al-Mallah et al. Reference Al-Mallah, Al-Bayati and Al-Marsoumi2006).
The best option for assessing the impact of FRE at Girsu, is provided by the two 14C charcoal dates and one shell from an Ubaid 4 close context (excavation area E3). The difference between the 14C age of the aquatic reservoir under study and the 14C age of the contemporaneous atmosphere is the reservoir age R and can be calculated by subtracting the atmospheric 14C age from the aquatic 14C age, provided that the 14C age of the atmosphere can be estimated from a charcoal sample and that the charcoal sample and aquatic sample are contemporaneous (Philippsen Reference Philippsen2013). The charcoal dates are in good agreement with the associated archaeological evidence and found in clear association with the aquatic shell sample.
Thus:



The uncertainty is:


(see Table 2 for standard deviations)


This suggests a FRE of between 310 ± 42 and 380 ± 42 14C years.
We ran the model again (Supplementary materials), applying this reservoir R ((R1+R2)/2 ± √422+422= 345 ± 60 14C years) to the Intcal20 calibration curve for all the shell samples. The FRE in hard-water rivers can be variable and vary over time and between species (Philippsen and Heinemeier Reference Philippsen and Heinemeier2013; Kulkova et al. Reference Kulkova, Mazurkevich, Dolbunova, Regert, Mazuy, Nesterov and Sinai2015; Schulting et al. Reference Schulting, Bronk-Ramsey, Scharlotta, Richards, Bazaliiskii and Weber2022; Svyatko et al. Reference Svyatko, Reimer, Schulting, Shevnina, Logvin, Voyakin, Stobbe, Merts, Varfolomeev and Soenov2022) so this is an over simplistic representation of the FRE around Girsu, but offers an initial correction (Table 2).
The reservoir correction of the shell samples brings all the 14C dates from the fluvial system within the period of known occupation at Girsu. The samples assigned to channel activity in the first phase of the model now overlap with the Ubaid 4 period, the samples assigned to the second phase of the model now all date after 2900 BC and agree with the archeological age boundary. The youngest date, from the lacustrine deposits, now overlaps with the age range from the associated archaeological evidence (2100–1800 BC). This exercise demonstrates the validity of the model and the initial FRE correction. However, in most instances the calibrated and corrected shell 14C dates still pre-date the associated archaeological material (Table 3) showing that the FRE at Girsu is indeed more variable and complex and should be further characterized with additional analysis.
CONCLUSIONS
Dating the canal system at Girsu using 14C and archaeological evidence has shown that a large difference exists between the two dating methods which likely reflects the FRE from the Tigris and the Euphrates. The shell 14C dates therefore only provide a terminus post quem for final canal activity. The archaeological evidence suggests that the canals were in use from at least the EDI period, which agrees with the textual evidence. The youngest archaeological evidence found below several canal fills dates to the Isin-Larsa period (2000–1800 BC). This indicates that the canals fell out of use at or shortly after this time, when Girsu becomes largely abandoned (at the beginning of the Old Babylonian period).
The use of cultural chronologies remains a widely used dating method in Mesopotamia. It has proven informative in the case of Girsu and obviously provides a clear link with the cultural history. However, it does not provide absolute dates and due to the wide age ranges for cultural periods does not offer a high enough chronological resolution for short-term changes.
Shell 14C dating remains useful as shell is the most widely available organic material in geological/environmental and geoarchaeological contexts in Mesopotamia and offers the possibility to obtain high resolution absolute dates. However, as our results indicate, FREs are a major drawback, and need to be better assessed for the region.
The fact that FREs in hard-water rivers can be large and variable (Philippsen and Heinemeier Reference Philippsen and Heinemeier2013), vary geographically and over time, between and within species (Kulkova et al. Reference Kulkova, Mazurkevich, Dolbunova, Regert, Mazuy, Nesterov and Sinai2015; Schulting et al. Reference Schulting, Bronk-Ramsey, Scharlotta, Richards, Bazaliiskii and Weber2022; Svyatko et al. Reference Svyatko, Reimer, Schulting, Shevnina, Logvin, Voyakin, Stobbe, Merts, Varfolomeev and Soenov2022) and affects shells, water plants, fish, and animals (and humans) whose subsistence was based on freshwater fish or water plants (Philippsen Reference Philippsen2013; Philippsen and Heinemeier Reference Philippsen and Heinemeier2013; Svyatko et al. Reference Svyatko, Reimer, Schulting, Shevnina, Logvin, Voyakin, Stobbe, Merts, Varfolomeev and Soenov2022), together with the scarceness of other radiocarbon dating material in Mesopotamia, can be disheartening when seeking to improve absolute chronologies in the region. As the Euphrates and Tigris are the source of life in the Mesopotamian floodplain, FREs from these rivers will never be far away. With an increasing interest in geoarchaeological approaches to studying past waterscapes in Mesopotamia (e.g., the “Sumer and the Sea” ARWA International Research Workshop, D’Agostino and Romano Reference D’Agostino and Romano2018; Fassbinder et al. Reference Fassbinder, Ostner, Scheiblecker, Parsi and Van Ess2019; Hammer Reference Hammer2022), understanding the FREs in the region becomes even more relevant. Sufficiently characterizing FREs in river systems requires a comprehensive research program (cf. Philippsen and Heinemeier Reference Philippsen and Heinemeier2013), which as yet has to be established in Mesopotamia. This is exactly the argument for publishing this paper: to highlight the problem, and re-initiate collaboration to create a database for paired terrestrial and freshwater 14C dates and archaeological evidence.
SUPPLEMENTARY MATERIAL
To view supplementary material for this article, please visit https://doi.org/10.1017/RDC.2023.40
ACKNOWLEDGMENTS
The Girsu Project is funded by the J. Paul Getty Trust. Our thanks go to the Iraq Ministry of Culture’s State Board of Antiquities and Heritage and the Iraqi Ministry of Higher Education and Scientific Research for their support, as well as to colleagues from Iraq, the British Museum Middle East department, and the Tello/Girsu team. The model was discussed with Maarten Blaauw, Queen’s University Belfast, to whom we are indebted for his guidance. We also thank our good friend and colleague Chikako Watanabe for her continuous support.
COMPETING INTERESTS
The authors declare that they have no known competing financial interests or personal relationships that could have appeared to influence the work reported in this paper.