INTRODUCTION
The giant Cape zebra (Equus capensis) is one of the extinct Quaternary large mammal species of southern Africa. The type specimen was described by Broom (Reference Broom1909) based on part of a mandible with four teeth that was found in 1907 embedded in a calcarenite layer on the coast near Cape Town. Broom (Reference Broom1913) noted that E. capensis was more powerfully built than a modern horse. Eisenmann (Reference Eisenmann2000) estimated a body mass for E. capensis of as much as 450 kg, and a height of 144–156 cm at the withers. This weight estimate is almost double that of its extant relatives, E. quagga (the plains zebra) and E. zebra (the mountain zebra), which respectively have recorded weights of 235 kg (Venter et al., Reference Venter, Prins, Balfour and Slotow2014) and 234 kg (Skinner and Chimimba, Reference Skinner and Chimimba2005). As noted by Faith (Reference Faith2014), E. capensis is the largest equid from the Quaternary of Africa. We use the term ‘giant Cape zebra’ rather than the formerly used ‘giant Cape horse’ because E. capensis has been shown to fall within the zebra clade rather than the horse clade (Orlando et al., Reference Orlando, Metcalf, Alberdi, Telles-Antunes, Bonjean, Otte and Martin2009).
Through the Cape south coast ichnology project, 26 Pleistocene equid (Order Perissodactyla; Family Equidae) tracksites have been identified, more than half of which are attributed to E. capensis. The aim of this paper is to review the equid tracksites, in particular those of E. capensis, and to consider their implications.
BACKGROUND
Body fossils of E. capensis have been reported from numerous localities. Many were initially assigned to separate species, based on variations in dental configuration and wear patterns. It was later recognized that these did not represent significant taxonomic differences, and 18 separate species were synonymized within E. capensis (Churcher and Richardson, Reference Churcher, Richardson, Maglio and Cooke1978; Churcher, Reference Churcher2006). Churcher (Reference Churcher2006) considered E. capensis to be conspecific with Grevy's zebra (E. grevyi), and that it represented an extant species, sensu strictu. However, based on skeletal material, Eisenmann (Reference Eisenmann2000) reported that E. capensis was substantially different from E. grevyi, and subsequent DNA analysis has demonstrated that E. capensis was more closely related to the extant plains zebra (E. quagga burchelli) than to E. grevyi (Orlando et al., Reference Orlando, Metcalf, Alberdi, Telles-Antunes, Bonjean, Otte and Martin2009). Furthermore, E. capensis has been shown to have a broader muzzle relative to its length than E. grevyi, as well as a simpler occlusal morphology and larger teeth (O'Brien et al., Reference O'Brien, Tryon, Blegen, Kimeu, Rowan and Faith2021). The status of E. capensis as a separate, extinct species therefore appears valid (Faith, Reference Faith2014).
Churcher (Reference Churcher2006) summarized the distribution of E. capensis in southern Africa to include the coastal areas of the western Cape, the Karoo, the Highveld, a single region in Namibia, and the Zimbabwe highlands. There are no Pliocene records of Equus in Africa (Bernor et al., Reference Bernor, Armour-Chelu, Gilbert, Kaiser, Schulz, Werdelin and Sanders2010). The earliest specimens, from Baard's Quarry in the Western Cape Province (Tankard, Reference Tankard1975; Hooijer, Reference Hooijer1976), date to the Early Pleistocene and from sites such as Kromdraai, Sterkfontein, and Swartkrans in Gauteng Province (Avery, Reference Avery2019). Most E. capensis fossils from southern Africa span a range from 90–11 ka (Faith, Reference Faith2014), and Klein (Reference Klein, Deacon, Hendey and Lambrechts1983) noted that E. capensis went extinct at ca. 12–10 ka. The last records (radiocarbon dates recalibrated here following Hogg et al., Reference Hogg, Heaton, Hua, Palmer, Turney, Southon and Bayliss2020) are from Elands Bay Cave in the southwestern Cape (Klein and Cruz-Uribe, Reference Klein, Cruz-Uribe, Parkington and Hall1987, Reference Klein and Cruz-Uribe2016), where the last occurrence of E. capensis (layer 12) dates to 10,650–11,180 cal yr BP (Pta-0686; 9600 ± 90 14C yr BP), and from Wonderwerk Cave in the Northern Cape Province (Thackeray et al., Reference Thackeray, Thackeray, Beaumont and Vogel1981; Thackeray, Reference Thackeray2015). In the latter case, a single specimen was obtained from unit 4d, for which the range of calibrated radiocarbon dates (from charcoal fragments; Thackeray Reference Thackeray, Thackeray, Beaumont and Vogel1981) spans 10,120–10,510 cal yr BP (Pta-2786; 9130 ± 90 14C yr BP) to 11,310–12,100 cal yr BP (Pta-2786; 10,200 ± 90 14C yr BP). The temporal range of E. capensis is thus from the Early Pleistocene to the terminal Pleistocene/early Holocene.
Klein (Reference Klein1980) noted that E. capensis fossils were more numerous in what are currently more arid regions. This is borne out by the synopsis of fossil and archaeological sites from the Cape coast by Marean et al. (Reference Marean, Cawthra, Cowling, Esler, Fisher, Milewski, Potts, Singels, De Vynck, Allsopp, Colville and Verboom2014), who noted that E. capensis formed the dominant herbivore taxon at the Early or Middle Pleistocene Elandsfontein site on the currently more arid west coast. For example, among a large suite of herbivore species at Elandsfontein, Klein et al. (Reference Klein, Avery, Cruz-Uribe and Steele2007) reported E. capensis specimens representing a minimum number of individuals (MNI) of 118. By contrast, the E. quagga MNI was 7. Other west coast sites (Duinefontein, Sea Harvest, Spreeuwal) also have yielded E. capensis remains (Marean et al., Reference Marean, Cawthra, Cowling, Esler, Fisher, Milewski, Potts, Singels, De Vynck, Allsopp, Colville and Verboom2014). In contrast, from the currently wetter Cape south coast, E. capensis fossils have been documented only from two sites: at Blombos Cave four specimens (MNI of 1) were identified in 100 ka layers (Henshilwood et al., Reference Henshilwood, Sealy, Yates, Cruz-Uribe, Goldberg, Grine, Klein, Poggenpoel, van Niekerk and Watts2001; Badenhorst et al., Reference Badenhorst, van Niekerk and Henshilwood2016), and at Pinnacle Point 30 two specimens (MNI of 1) were identified in ca. 151 ka layers (Rector and Reed, Reference Rector and Reed2010). Equus capensis has been reported from Boomplaas Cave (Faith, Reference Faith2013), ~75 km inland from the current Cape south coast. Two sites west of our study area (see below) in the southwestern Cape also have yielded single E. capensis specimens: Die Kelders Cave 1 (Klein and Cruz-Uribe, Reference Klein and Cruz-Uribe2000) and Byneskranskop 1 (Schweitzer and Wilson, Reference Schweitzer and Wilson1982).
Despite this wealth of skeletal material for E. capensis from southern Africa, there was only a single reference to Pleistocene equid tracks from the subcontinent prior to the initiation of the Cape south coast ichnology project. Roberts et al. (Reference Roberts, Bateman, Murray-Wallace, Carr and Holmes2008) noted that aeolianites in cliffs east of Still Bay on the Cape south coast contained a rich archive of mammal trackways, including equids, but no details were provided. Brief synopses of equid tracksites were provided by Helm et al. (Reference Helm, McCrea, Cawthra, Thesen and Mwankunda2018a, Reference Helm, Cawthra, De Vynck, Lockley, McCrea and Venter2019b, Reference Helm, Cawthra, Cowling, De Vynck, Lockley, Marean, Thesen, Venter, Cleghorn, Potts and Cawthra2020a) as part of ichnological summaries of the Cape south coast. Subsequent exploration has led to the identification of additional equid tracksites, as described and dated here.
GEOLOGICAL CONTEXT
Pleistocene aeolianites of the Waenhuiskrans Formation, which contain the tracksites described herein, are exposed along the Cape south coast of South Africa (Malan, Reference Malan1989). Pleistocene shoreface, foreshore, and lagoonal deposits of the Klein Brak Formation are also exposed (Malan, Reference Malan1991). The two formations comprise part of the Bredasdorp Group.
Through the Cape south coast ichnology project, >300 tracksites have been identified since 2007 between Arniston in the west and the Robberg Peninsula in the east, a distance of 350 km (Fig. 1). Optically stimulated luminescence (OSL) dating has revealed that most near-coastal aeolianites date to Marine Isotope Stage (MIS) 5 (Roberts et al., Reference Roberts, Bateman, Murray-Wallace, Carr and Holmes2008; Carr et al., Reference Carr, Bateman, Roberts, Murray-Wallace, Jacobs and Holmes2010a; Bateman et al., Reference Bateman, Carr, Dunajko, Holmes, Roberts, McLaren, Bryant, Marker and Murray-Wallace2011; Cawthra et al., Reference Cawthra, Jacobs, Compton, Fisher, Karkanas and Marean2018). MIS 11 aeolianite deposits at Dana Bay (Roberts et al., Reference Roberts, Karkanas, Jacobs, Marean and Roberts2012) and MIS 3 aeolianite deposits at Robberg (Carr et al., Reference Carr, Bateman, Cawthra and Sealy2019) also have been reported.
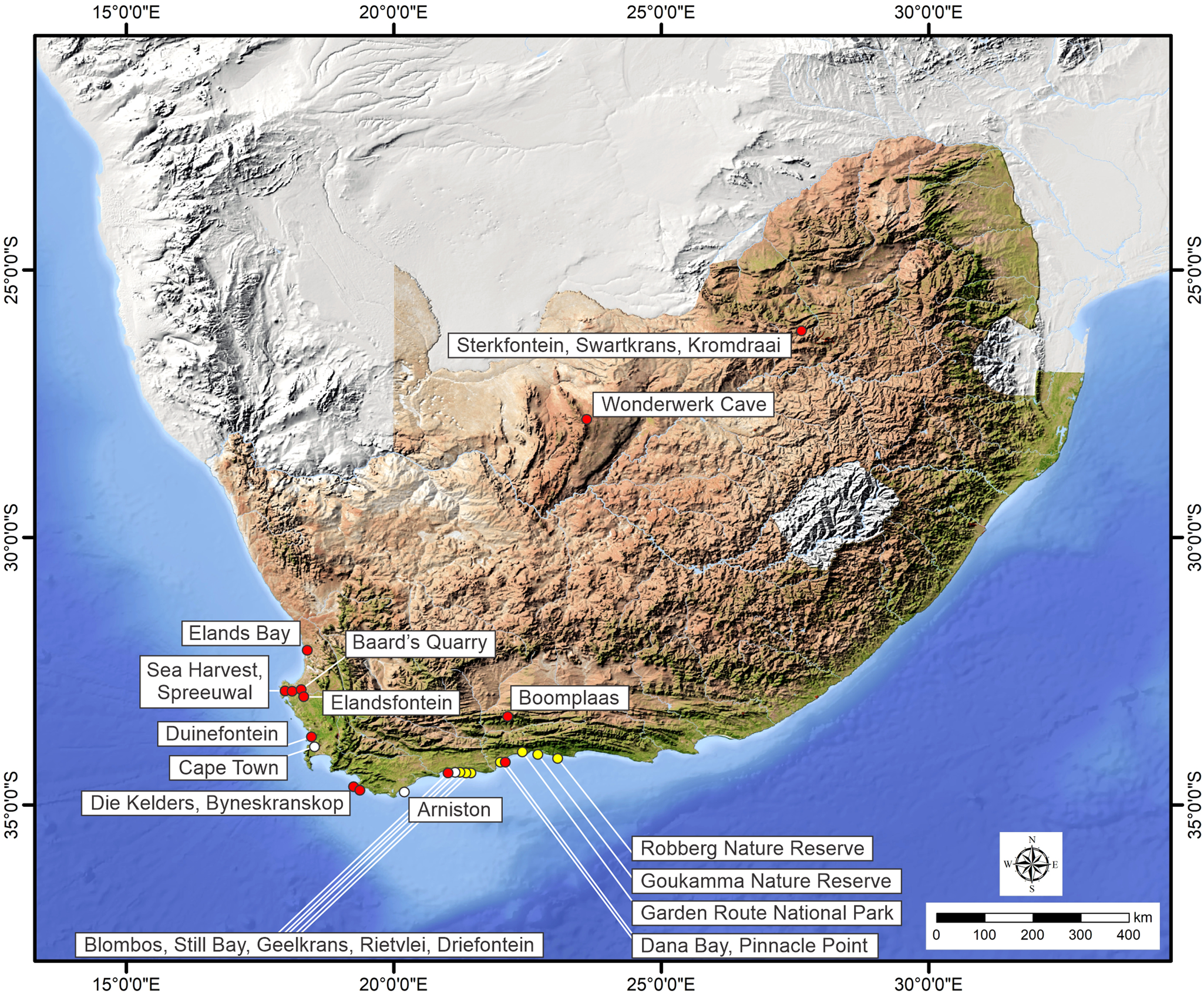
Figure 1. South Africa and the Cape south coast, showing sites mentioned in the text. Selected E. capensis body fossil sites are indicated by red circles; areas in which E. capensis ichnosites (totaling 13) have been identified are indicated by yellow circles; white circles indicate other place names.
Roberts et al. (Reference Roberts, Bateman, Murray-Wallace, Carr and Holmes2008) noted that planar cross-bedded units form the dominant primary sedimentary structure in coastal aeolianites east of Sill Bay, with foreset orientation indicating a dominance of westerly winds. Similar facies occur elsewhere on the coastline in areas from which the tracksites described herein have been recorded, including the Goukamma Nature Reserve (Bateman et al., Reference Bateman, Carr, Dunajko, Holmes, Roberts, McLaren, Bryant, Marker and Murray-Wallace2011).
Tracks may be preserved in epirelief as natural molds, in hyporelief as natural casts, or in profile in cliffs. While many tracksites occur in situ, storm surges and high tides may cause cliff collapse. Dislodged slabs may tumble down to the base of the coastal cliffs and be subjected to wave and wind erosion or may slump into the sea. Therefore, an approximate equilibrium exists between exposure of new sites and loss of known sites.
Belvedere and Farlow (Reference Belvedere, Farlow, Falkingham, Marty and Richter2016) developed a four-point (0-1-2-3) numerical scale in order to quantify the level of track preservation and quality, in which level 3 indicates a perfectly registered and preserved track. Tracks in Cape south coast aeolianites seldom rise above level 2 on this scale, largely due to the relatively coarse nature of the dune sands and the absence of effective cohesion of dry sand. Well-preserved tracks with a high level of morphological detail, such as those registered in muddy substrates, are therefore relatively rarely encountered in this region.
There is a potential bias in the trace fossil record towards preservation of tracks of larger, heavier animals, which leave larger, deeper tracks that may be easier to recognize and interpret when cemented surfaces are re-exposed. This is a different bias compared with the body fossil record, which often represents animal remains that have been selectively transported to scavenger dens or archaeological sites. In contrast, the trace fossil record provides a census of the creatures that moved upon Pleistocene dune surfaces. Therefore, the trace fossil and body fossil records have the potential to complement each other.
REVIEW OF EQUID TRACK MORPHOLOGY
The characteristics of well-preserved equid tracks include a curved, unbroken anterior hoof wall, and a distinctive ‘frog’ outline (Fig. 2). The frog is a structure of dense tissue that extends from the posterior margin of the foot (where it is broad) to an apex in the center of the hoof. The resulting triangular feature is seen in well-preserved tracks but is often not registered. The absence of evidence of an interdigital sulcus (a characteristic of bovid tracks) is a useful diagnostic feature. The manus track tends to be rounder than that of the slightly longer and narrower pes (Liebenberg, Reference Liebenberg2000; van den Heever et al., Reference van den Heever, Mhlongo and Benadie2017; Stuart and Stuart, Reference Stuart and Stuart2019).

Figure 2. Track morphology of Equus quagga burchelli, reproduced with permission from Alex van den Heever: a left manus track is on the left, and a left pes track is on the right.
On firm substrates only the hoof wall tends to be registered (Stuart and Stuart, Reference Stuart and Stuart2019). In soft, unconsolidated sand all that may be evident is a crater of appropriate size and relative dimensions, but without morphological detail. In between these extremes there is a substrate (usually damp) of ideal cohesiveness, in which the characteristic equid features may be preserved.
Equid and bovid tracks may be of similar size and relative dimensions. Where track preservation is poor and morphological detail is lacking, they may be indistinguishable. Confident identification is therefore limited to relatively well-preserved tracks. Equids tend to ‘overstep’ more frequently than bovids, rather than employing a direct register (van den Heever et al., Reference van den Heever, Mhlongo and Benadie2017). Thus, the manus is often placed immediately ahead of the pes in a walking gait. If the level of preservation is poor and the two tracks coalesce, the outline and dimensions may potentially cause confusion with hominin tracks (Helm et al., Reference Helm, Lockley, Cole, Noakes and McCrea2019a).
Equids may exhibit a walking, trotting, or galloping gait, each with a distinctive pattern. As speed increases from a slow walk to a trot, the distance between the manus track and the pes track ahead of it increases, and the straddle decreases (van den Heever et al., Reference van den Heever, Mhlongo and Benadie2017). Because equids are largely gregarious, it may be difficult to distinguish individual trackways within a large collection of tracks.
Track length for the extant plains (Burchell's) zebra (Equus quagga burchelli) has been reported as 8.5–11 cm (van den Heever et al., Reference van den Heever, Mhlongo and Benadie2017), with track width less for the pes than the manus. Liebenberg (Reference Liebenberg2000) reported a length of 9.5 cm for the manus and 10 cm for the pes. Stuart and Stuart (Reference Stuart and Stuart2019) reported average track length for this species of 7.7 cm for the manus and 8.3 cm for the pes. For the Cape mountain zebra (Equus zebra zebra) van den Heever et al. (Reference van den Heever, Mhlongo and Benadie2017) reported track lengths of 7.5 cm (manus) and 8 cm (pes). Liebenberg (Reference Liebenberg2000) reported track lengths of 9 cm (manus) and 10 cm (pes), and noted that tracks were smaller than those of the plains zebra. Stuart and Stuart (Reference Stuart and Stuart2019) reported track lengths of 10 cm and 11 cm for both the manus and the pes of this species, and noted that tracks were noticeably narrower than those of the plains zebra.
METHODS
Global Positioning System readings were taken for tracksites using a hand-held device. Locality data are reposited with the African Centre for Coastal Palaeoscience at Nelson Mandela University, South Africa, to be made available to researchers upon request. Geological outcrops were investigated in the field and their field relations were compared to dated deposits from the area of investigation. Standard field techniques were applied for understanding the context of the ichnofossils to determine the strata from which they were derived. These included measurement of the thickness of aeolian foresets, observation of color, texture and degree of carbonate cementation, and sedimentary characteristics of the clasts. Dip and strike measurements were taken on in situ bedding.
Measurements and photographs were taken of track-bearing surfaces and better-preserved tracks. Measurements included track length, width, and depth. Where feasible, stride length was measured. Results were recorded in centimeters. In some cases, tracings were made on clear acetate film. In one case, a DJI Mine 2 drone with an inbuilt DJI camera/video was used to obtain photographs from directly above a trackway.
Photogrammetry (Matthews et al., Reference Matthews, Noble, Breithaupt, Falkingham, Marty and Richter2016) was performed on well-preserved tracks, and 3D models were generated with Agisoft Metashape Professional (v. 1.0.4) using an Olympus Tough model TG-6 camera (focal length 4.5 mm; resolution 4000 × 3000; pixel size 1.56 × 1.56 μm). The final images were rendered using CloudCompare (v. 2.10-beta).
In this study, new samples for OSL dating were obtained from one track-bearing layer, and other samples were obtained close to equid tracksites. These were obtained as block samples and were shipped to the University of Leicester (United Kingdom), where they were broken up under red light conditions to obtain unexposed sand-sized sediment (and assigned lab codes beginning with ‘Leic’ followed by a number). The coarse-grained (usually in the range 150–250 μm) quartz fraction was isolated (e.g., Wintle, Reference Wintle1997, and supplementary information) and equivalent doses were determined using the single aliquot regeneration method (Murray and Wintle, Reference Murray and Wintle2000, Reference Murray and Wintle2003). Environmental dose rates were determined using elemental concentrations obtained via inductively coupled plasma-mass spectrometry (ICP-MS) for U and Th, and ICP-optical emission spectroscopy (ICP-OES) for K. Details of the equivalent dose measurement conditions and quality control procedures are provided as supplementary information, along with the dose rate calculations.
RESULTS AND INTERPRETATION
Twenty-six sites containing tracks attributable to equid trackmakers were identified. Of these, three that were inaccessible had to be assessed through binoculars without the benefit of a scale, one could not be reliably measured, and one was equivocal but of interest because it occurred in MIS 11 aeolianite deposits at Dana Bay. The sites are listed in Table 1, from west to east.
Table 1. Equid tracksites on the Cape south coast, from west to east. L = length; W = width; GRNP = Garden Route National Park.

For the remaining 21 sites it was apparent that no track length between 10–12 cm was recorded at any site. Given the dimensions of tracks of extant regional equid species (Liebenberg, Reference Liebenberg2000; van den Heever et al., Reference van den Heever, Mhlongo and Benadie2017; Stuart and Stuart, 2020), for which no tracks longer than 11 cm have been reported, we chose to separate the sites into two groups: the first contained tracks ≥12 cm in length, and the second contained tracks ≤10 cm in length. Some sites exhibited multiple tracks, mostly of the same size. Each site was assigned a single measurement in cm for track length, representing the dominant track length from that site. Table 1 indicates that 13 sites contained tracks that were at least 12 cm in length, with a range from 12–17 cm (mean 13.6 cm), whereas eight sites contained tracks that were 10 cm or less in length, with a range from 7–10 cm (mean 8.9 cm).
Sites with distinctive features are presented here from west to east, first of tracks with length >11 cm, then <11 cm. All are inferred to occur in Waenhuiskrans Formation aeolianites.
Large-equid tracks
Geelkrans Nature Reserve
The reserve, situated east of Still Bay, contains two large-equid tracksites. The western site contains four in situ tracks in profile in a low aeolianite cliff (Fig. 3a). The hyporelief surface of one track is visible and has equid features (length ~15 cm, width ~12 cm). The remaining tracks are evident in profile only, with considerable deformation of underlying layers. On an in situ surface at the base of these cliffs, a single large equid track containing infill (Fig. 3b) is evident in epirelief (length ~14.5 cm, width ~12 cm).

Figure 3. (A, B) The western Geelkrans site. (A) Track in profile in a low cliff indicated by arrow; scale bars = 10 cm; (B) large-equid track in epirelief at the base of the low cliff in (A); scale bar= 10 cm. (C, D) The eastern Geelkrans site. (C) Tracks in hyporelief are indicated with arrows; (D) detailed view of the middle track in (C); scale bar = 15 cm.
At the eastern site a well-preserved composite track is preserved in hyporelief on the underside of an in situ overhang in aeolianite cliffs (Fig. 3c, d). The overall track dimensions are 20 × 15 cm; both manus and pes tracks (with prominent frog and hoof wall impressions) are discernable. The pes track, partly superimposed on the manus track, measures 15 cm in length and 13.5 cm in width. Transmitted tracks with similar dimensions occur on the surface, and additional tracks are evident in profile several cm higher in the stratigraphic section, suggesting repeated use of the site. The nearest sampled site (Leic21008), 3.3 km to the east, yielded an OSL age of 134 ± 9 ka (Helm et al., Reference Helm, Carr, Cawthra, De Vynck, Dixon, Lockley, Stear and Venter2022a).
East of Geelkrans
This area of coastline contains two large-equid tracksites. At the western site, on a loose slab, two shallow equid tracks (~15 cm long and 12 cm wide) illustrate an overstepping or understepping gait and invite comparison with a hominin footprint if the substrate had been less firm (Fig. 4a). A sample (Table S1, Leic21005) for OSL dating from a site 80 m to the west, produced an age of 126 ± 9 ka.

Figure 4. (A) Large-equid tracks east of Geelkrans, with an overstepping or understepping gait, illustrate a possible resemblance to a hominin track; scale bar = 10 cm. (B) Four large tracks and one smaller track east of Geelkrans (outlined in chalk); scale bar = 10 cm.
At the eastern site, two loose slabs lay at the base of cliffs near the high-tide mark. A probable layer of origin, ~1 m above the slabs, was determined. Nearby, in this layer, a ‘traceway’ was present, inferred to have been made by a sand-swimming golden mole such as Eremitalpa, and which formed a paratype for the ichnospecies Natatorichnus sulcatus Lockley et al., Reference Lockley, Helm, Cawthra, De Vynck and Perrin2021. One of the slabs, which was portable and was accessioned in the Blombos Museum of Archaeology in Still Bay (museum number ICH011AN), contained three tracks (~15 cm long, 13 cm wide). The second slab (non-portable) contained four large tracks (13 cm long, 12 cm wide), and one smaller track beside them, possibly representing a juvenile (Fig. 4b). An OSL age (Table S1, Leic20033) of 161 ± 12 ka was obtained from the track-bearing surface.
Driefontein
The most important large-equid tracksite thus far identified is situated ~14 km east of Still Bay in the Driefontein area. A large loose slab that lies near the low tide mark (Fig. 5; Figs. S2, S3 in supplementary information) contains a long, narrow-straddle trackway (total length 320 cm) of 12 tracks. The distal two tracks are transmitted tracks on an underlying layer. Track length and width of ~12–14 cm were recorded. Stride lengths of 142 cm, 146 cm, 150 cm, and 150 cm (mean = 147 cm) were measured. A repeating short-long pattern was noted. The mean minimum distance between consecutive tracks was 11 cm in the short segments and 23 cm in the long segments. While the layer of origin was not confidently determined, two nearby sites were sampled for dating. Leic20033, 1.2 km to the west, was dated to 161 ± 12 ka (Table S1) while Leic20024, 0.8 km to the east, was dated to 109 ± 9 ka (Helm et al., Reference Helm, Carr, Cawthra, De Vynck, Dixon, Lockley, Stear and Venter2022a).

Figure 5. (A) 3D photogrammetry image of long large-equid trackway on a loose slab in the Driefontein area; horizontal and vertical scales are in meters. (B) Long large-equid trackway on a loose slab on the Driefontein coast; all scale bars = 10 cm. (C) 3D photogrammetry image of two of the tracks, illustrating prominent hoof wall impressions; horizontal and vertical scales are in meters.
Goukamma Nature Reserve
Two sites previously have been reported from this reserve (Helm et al., Reference Helm, McCrea, Cawthra, Thesen and Mwankunda2018a). At the coastal site, three large equid tracks (length = 15 cm; width = 13 cm) with similar orientation form a short trackway segment on a loose slab (Fig. 6a). One may be a composite track. An OSL sample (Leic20003) that was obtained from 40 m to the east produced an age of 70 ± 4 ka (Helm et al., Reference Helm, Carr, Cawthra, De Vynck, Dixon, Lockley, Stear and Venter2022a).

Figure 6. (A) Large-equid tracks (indicated by arrows) form a short trackway on the Goukamma Nature Reserve coastline. (B) Arrows indicate large-equid tracks at an inland site in the Goukamma Nature Reserve.
At the inland site, above the Goukamma River, three large tracks were exposed on a loose slab (Fig. 6b). The unbroken hoof wall was a prominent feature. The surface became eroded following initial identification, and the tracks are no longer identifiable.
Robberg Nature Reserve
An in situ surface (Fig. 7a) on The Island (an aeolianite stack at the southern end of a tombolo) contained several large equid tracks, most of which were shallow, exhibiting just hoof wall impressions. However, one track displayed better morphological detail, with a discernible frog (Fig. 7b). Aeolianites on the Robberg Peninsula were dated previously to late MIS 3 (Carr et al., Reference Carr, Bateman, Cawthra and Sealy2019). The new ages obtained here from basal layers (stratigraphically below the ages reported in Carr et al., Reference Carr, Bateman, Cawthra and Sealy2019) confirm the MIS 3 age of The Island's aeolianites (Table S1, Leic21003: 56 ± 5 ka, Leic20028: 44 ± 4 ka, and Leic21004: 43 ± 4 ka).

Figure 7. (A) Aeolianite surface in the Robberg Nature Reserve, containing large-equid tracks. (B) 3D photogrammetry image of a large-equid track on the surface shown in (A); horizontal and vertical scales are in meters.
Small-equid tracks
A site in the Driefontein area contains two trackways that intersect at a right angle on a loose slab (Fig. 8a). The longer trackway contains at least ten equid tracks (9 cm long, 7 cm wide), some of which appear composite, and in one case two tracks are situated beside each other. The shorter trackway contains six smaller tracks, possibly registered by a juvenile equid. An OSL sample (Leic20030) from 1 km to the west, produced an age of 131 ± 9 ka (Helm et al., Reference Helm, Bamford, Carr, Cawthra, De Vynck, Dixon, Quick and Stear2022b). A sequence 1.8 km to the west was reported by Roberts et al. (Reference Roberts, Bateman, Murray-Wallace, Carr and Holmes2008) as 140 ± 8 ka to 91 ± 5 ka.
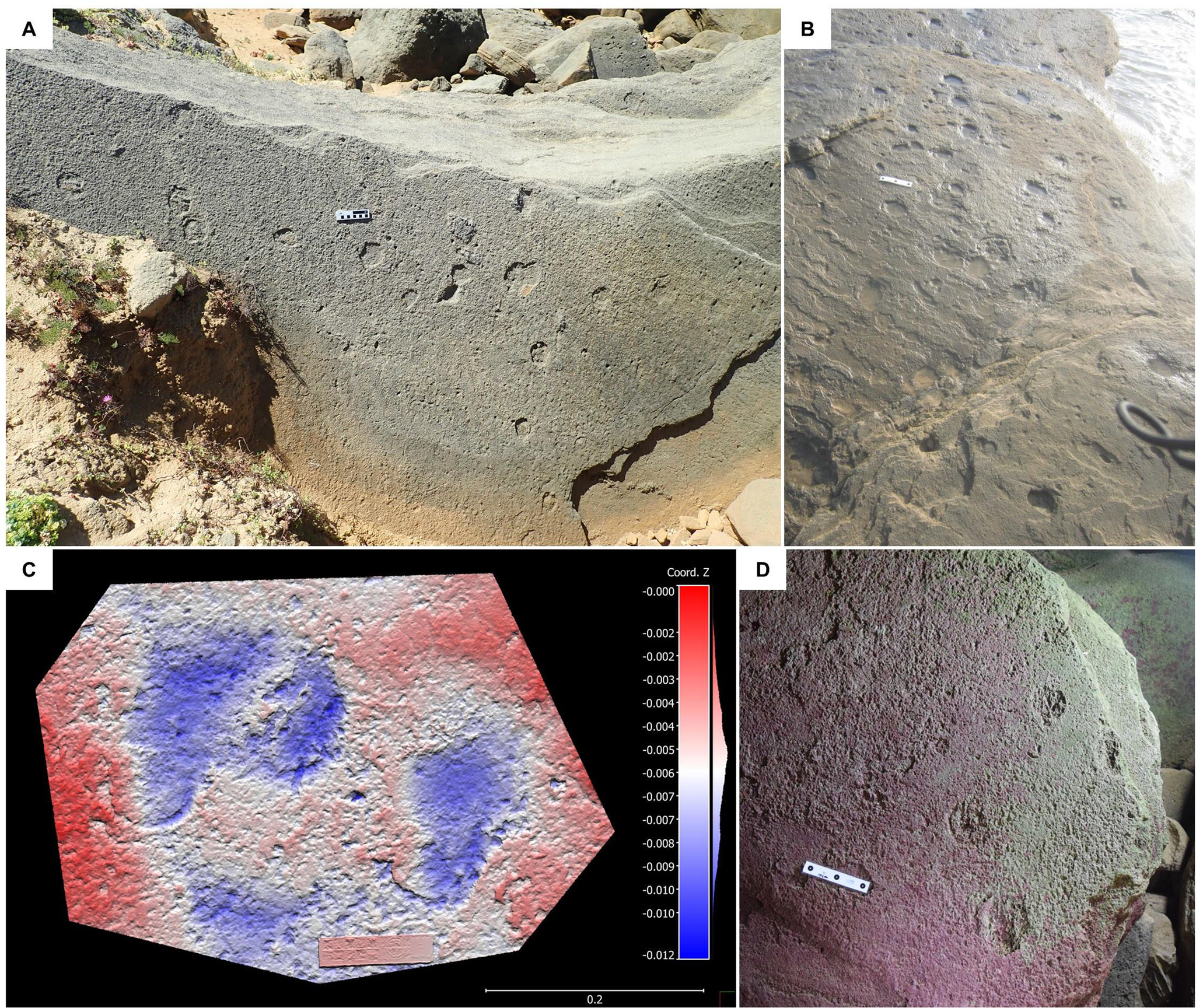
Figure 8. (A) Intersecting trackways on the Driefontein coastline; scale bar = 10 cm. (B) The western Garden Route National Park site, showing multiple small-equid tracks without identifiable trackways; the distance between the outer black dots on the scale bar = 10 cm. (C) 3D photogrammetry image of shallow small-equid tracks at the eastern Garden Route National Park site; horizontal and vertical scales are in meters. (D) Small-equid tracks on the surface of a loose slab in a cave in the Robberg Nature Reserve; the distance between the outer black dots on the scale bar = 10 cm.
Two sites in the Garden Route National Park (GRNP) are instructive. The western site comprises an assemblage of >20 tracks, without clearly discernible trackways (Fig. 8b). The tracks occur on a series of in situ layers that are exposed at low tide. Track length is ~9 cm. Previous OSL dating of a locale 3.5 km to the east produced ages of 133 ± 7 ka and 131 ± 7 ka (Bateman et al., Reference Bateman, Carr, Dunajko, Holmes, Roberts, McLaren, Bryant, Marker and Murray-Wallace2011). At the eastern GRNP site an occasionally exposed loose block exhibits three shallow tracks (8 cm long), illustrating how sometimes on a firm substrate only the hoof wall impression is preserved (Fig. 8c).
Sea caves on The Island at Robberg contain tracks (Helm et al., Reference Helm, Cawthra, Hattingh, Hattingh, McCrea and Thesen2019c). A loose slab on the floor in one cave contained four equid tracks (8 cm long and 8 cm wide) in epirelief (Fig. 8d). The site's proximity to the aforementioned OSL samples from The Island provides some confidence in assigning an MIS 3 age.
DISCUSSION
The origin of all horses can be traced back to the Eocene in North America (Flannery, Reference Flannery2001), following which the genus Equus radiated to Eurasia (and to Africa) via the Bering land bridge in the Pliocene. It can therefore be noted that equids on South Africa's Cape south coast have over time made the longest possible land journey from their region of origin.
Pleistocene equid tracks have been reported from a number of locations, as summarized here in order of decreasing age. Equid tracks dated to 1.5 Ma were reported in a trackmaker assemblage at Ileret in Kenya (Roach et al., Reference Roach, Du, Hatala, Ostrofsky, Reeves, Braun, Harris, Behrensmeyer and Richmond2018). Possible equid tracks, dated to ca. 0.7 Ma, were reported in a trackmaker assemblage at Melka Kunture in Ethiopia (Altamura et al., Reference Altamura, Bennett, D'Août, Gaudzinski-Windheuser, Melis, Reynolds and Mussi2018). A short trackway comprising three well-defined equid tracks that cross a hominin trackway, dated to ca. 350 ka, was reported from the Roccamonfina Volcano site in Italy (Pillola et al., Reference Pillola, Palombo, Panarello and Zoboli2020). A single small equid track, possibly registered by a wild ass, was reported from a trackmaker assemblage at the Alathar site on the Arabian Peninsula dated to 121–112 ka (Stewart et al., Reference Stewart, Clark-Wilson, Breeze, Janulis, Candy, Armitage and Ryves2020). An equid trackway comprising 28 tracks formed part of a large trackmaker assemblage identified in ca. 50 ka deposits in the 1880s at Carson City, Nevada, USA, the tracks subsequently were covered during construction in the 1930s (Lockley and Hunt, Reference Lockley and Hunt1995, p. 280). Probable small equid tracks, dated to 19–15 ka, were reported from the Tamanduá de Cima site in Brazil (Oliveira et al., Reference Oliveira, Bélo, Fambrini, Sial, Silva and Barreto2019). Two large well-preserved equid tracks and two undertracks, dated to ca. 12 ka, were reported from the Pehuen Co site in Argentina (Aramayo et al., Reference Aramayo, de Bianco, Bastianelli and Melchor2015). McNeil et al. (Reference McNeil, Mills, Tolman, Kooyman, Lucas, Spielmann and Lockley2007) reported a trackway comprising five tracks, 10.5 cm in length, and dated to ca. 11 ka, from Wally's Beach, Alberta, Canada, which was attributed to Equus conversidens.
It is evident that the majority of these examples represent isolated tracks or short trackway segments, and that the Driefontein sites described herein, containing 10 and 12 tracks, are unusual. Furthermore, the global record of long horse trackways appears to be rather sparse. Vincelette (Reference Vincelette2021, and references therein) reported Miocene, Pliocene, and Pleistocene examples. We are aware of only one other record of a long African equid trackway: Pliocene trackways containing 8–13 tracks were reported from Laetoli, Tanzania (Renders, Reference Renders1984; Renders and Sondaar, Reference Renders, Sondaar, Leakey and Harris1987).
Given that no tracks of extant equids longer than 11 cm have been reported, the large equid tracks (12 cm or longer) on the Cape south coast are inferred to represent the first documented ichnological reports of E. capensis. The smaller equid tracks are more consistent with rounder tracks of plains zebra (E. quagga), rather than the more elongate tracks of Cape mountain zebra (E. zebra), which is also a less plausible trackmaker on dunes given its habitat preferences (e.g., Skinner and Chimimba, Reference Skinner and Chimimba2005). The quagga (E. quagga quagga, the southern race of the plains zebra that became extinct in the nineteenth century) appears to be a likely trackmaker, based on historical distribution range and habitat preference (e.g., Skinner and Chimimba, Reference Skinner and Chimimba2005). However, juvenile E. capensis cannot be excluded.
The current data indicate a possible higher prevalence of tracks attributable to E. capensis (mean length 13.6 cm) than E. quagga quagga (mean length 8.9 cm) on the Cape south coast. However, there may be a bias towards identification of larger, deeper tracks. Reliable identification of more equid tracksites, with a larger sample size, may allow for a more accurate determination of the relative prevalence of large and small Pleistocene equid trackmakers in the region.
Stachurska et al. (Reference Stachurska, Kolstrung, Pieta and Silmanowicz2011) obtained a ratio range of 12.83–13.16 between the height at the withers and manus length in the modern horse (E. caballus). Applying this ratio to the mean length of the large tracks (13.6 cm) yields an estimated height range at the withers of 174–179 cm. This is probably an overestimate because the mean track length does not distinguish between manus tracks and possibly longer pes tracks. The estimated height at the withers of E. capensis, based on track length and acknowledged to be a probable overestimate, thus appears to be a reasonable match for the height range of 144–156 cm obtained by Eisenmann (Reference Eisenmann2000) based on skeletal material.
Trackway patterns corresponding to equid gaits have been described (Vincelette, Reference Vincelette2021, and references therein), and patterns pertaining to southern African equids have been summarized (van den Heever et al., Reference van den Heever, Mhlongo and Benadie2017). To determine the gait that an equid trackmaker employed, a reasonably long trackway is required, but bedding-plane exposures in Cape south coast aeolianites are seldom large enough to exhibit sufficiently long trackways, and track morphological detail may not be sufficient to allow distinction between manus tracks and pes tracks. Vincelette (Reference Vincelette2021) acknowledged the challenges inherent in determining the gait patterns of horses from fossil tracks.
The Driefontein large-equid tracksite, which contains a trackway (320 cm) of 12 tracks, is notable for its length and for representing a single trackmaker. The repeated short-long sequence of tracks in six pairs, with a short stride length of 147 cm, is most consistent with a walking gait pattern. Vincelette (Reference Vincelette2021) described the walking footfall pattern as comprising ipsilateral couplets that alternate between the left and the right side of the midline, with a short stride length (147.1 cm for a slow walk and 177.0 cm for a fast walk). We cannot reliably distinguish manus from pes tracks in this trackway, although the seventh track (proximal track in Fig. 5C) appears relatively long and narrow. If this interpretation is correct, it would imply a very slow walking gait with substantial understepping. However, this pattern cannot be discerned in the other couplets, and we prefer to simply infer a walking gait pattern.
The Driefontein small-equid tracksite, exhibiting more regularly spaced tracks, may also be amenable to gait pattern interpretation. The apparent composite nature of some of the tracks suggests predominantly a direct register, and perhaps also a walking gait.
The OSL ages reported in this study, combined with those published previously (Roberts et al., Reference Roberts, Bateman, Murray-Wallace, Carr and Holmes2008; Bateman et al., Reference Bateman, Carr, Dunajko, Holmes, Roberts, McLaren, Bryant, Marker and Murray-Wallace2011; Carr et al., Reference Carr, Bateman, Cawthra and Sealy2019; Helm et al., Reference Helm, Carr, Cawthra, De Vynck, Dixon, Lockley, Stear and Venter2022a, Reference Helm, Bamford, Carr, Cawthra, De Vynck, Dixon, Quick and Stearb), provide an indication of the time period during which equids such as E. capensis and E. quagga quagga left their tracks on regional dunes. The range extends from MIS 6 (161 ± 12 ka) through MIS 5 to MIS 3 (43 ± 4 ka). Within this time frame, the climate, temperature, and distance from the dunes to the coastline would have varied substantially. The possible large-equid tracks at Dana Bay in deposits identified as dating to MIS 11 (Roberts et al., Reference Roberts, Karkanas, Jacobs, Marean and Roberts2012) inspire exploration of these deposits with a view to extending the temporal range of E. capensis tracks.
The tracksites would have been situated at the margin of the Palaeo-Agulhas Plain, the currently mostly submerged 85,000 km2 region extending south from the modern Cape coastline (Cleghorn et al., Reference Cleghorn, Potts and Cawthra2020), which was alternately inundated and exposed during Pleistocene sea-level oscillations. The Palaeo-Agulhas Plain was characterized by fertile soils, C3 and/or C4 grasslands, rivers, floodplains, and wetlands (Marean et al., Reference Marean, Cowling, Franklin, Cleghorn, Potts and Cawthra2020). The hypsodont teeth of E. capensis imply that it was a bulk grazer of dry grasses (Faith, Reference Faith2011). Marean et al. (Reference Marean, Cowling, Franklin, Cleghorn, Potts and Cawthra2020) noted that the northern portion of the Palaeo-Agulhas Plain would have contained floodplain and shale grasslands, providing suitable habitat for grazing species such as E. capensis. Likewise, the renosterveld vegetation in the northwestern portion of the Palaeo-Agulhas Plain would have included a grassy component, likely attractive to E. capensis. Equids are non-ruminants that require a high rate of intake (Okello et al., Reference Okello, Wishitemi and Muhoro2002). When the well-watered Palaeo-Agulhas Plain was exposed during the Pleistocene, its C4 grasslands were probably a key resource for E. capensis (Marean et al., Reference Marean, Cowling, Franklin, Cleghorn, Potts and Cawthra2020).
Most grazers associate with water sources (Smit et al., Reference Smit, Grant and Devereux2007). Extant equids, being water dependent, usually stay within 12 km of the nearest source of water (Skinner and Chimimba, Reference Skinner and Chimimba2005). The 14 Geelkrans, Rietvlei, and Driefontein sites all occur within 12 km of the elongate interdune freshwater wetland, Rietvlei, which is situated just inland (north) of the first row of cliffs and dunes. A core sample from Rietvlei indicates that it dates back to at least MIS 3 (Carr et al., Reference Carr, Boom, Chase, Roberts and Roberts2010b; Quick et al., Reference Quick, Carr, Meadows, Boom, Bateman, Roberts, Reimer and Chase2015), and older sediments have been cored (ASC, personal observation, 2009) from below the basal radiocarbon date (ca. 32 ka) reported in Quick et al. (Reference Quick, Carr, Meadows, Boom, Bateman, Roberts, Reimer and Chase2015). Roberts et al. (Reference Roberts, Bateman, Murray-Wallace, Carr and Holmes2008, fig. 9) also noted evidence for high water tables at this locale preserved within aeolianite cliff exposures, potentially indicating the presence of an “ancestral” Rietvlei. Proximity to such a water source may account for the profusion of equid tracksites in this area.
Grassland habitats would have been almost completely absent from the region at the Pleistocene-Holocene transition. Faith (Reference Faith2014) noted that at least 24 large mammal species disappeared from continental Africa during the Late Pleistocene or Holocene, mostly between 13 ka and 6 ka, with grazing species predominantly affected. Three of these extinctions (E. capensis, the long-horned buffalo Syncerus antiquus, and the giant wildebeest Megalotragus priscus) represent the largest members of their lineages. All three were adapted to consume large amounts of low-quality forage, necessitating larger home ranges, which made them susceptible to habitat loss (Faith, Reference Faith2011). Environmental change can be implicated in these extinction events, and in the case of E. capensis the loss of suitable habitat provided by the Palaeo-Agulhas Plain may have been a contributing factor (Venter et al., Reference Venter, Brooke, Marean, Fritz, Helm, Cleghorn, Potts and Cawthra2020). While Faith (Reference Faith2014) did not contend that humans played an important role in these extinctions, the timing of the extinction of E. capensis coincides with a probable increased presence of Homo sapiens (Marean et al., Reference Marean, Cawthra, Cowling, Esler, Fisher, Milewski, Potts, Singels, De Vynck, Allsopp, Colville and Verboom2014, Reference Marean, Cowling, Franklin, Cleghorn, Potts and Cawthra2020). Increased predation by humans conceivably may have played a contributory role.
The abundance of E. capensis tracksites on the Cape south coast contrasts with the relative paucity of body fossils of the species from this region and challenges the impression obtained from the body fossil record that E. capensis may have been a predominantly west coast species. The situation is analogous to the profusion of tracksites of the African elephant (Loxodonta africana), which pointed to a more substantial presence than was suggested by the body fossil record (Helm et al., Reference Helm, Lockley, Moolman, Cawthra, De Vynck, Dixon, Stear and Thesen2021). Examples of species from the region known only through their tracks include giraffe (Helm et al., Reference Helm, Cawthra, Cowling, De Vynck, Marean, McCrea and Rust2018b), large reptiles (Helm et al., Reference Helm, Cawthra, Combrink, Helm, Rust, Steer and van den Heever2020b), large avians (Helm et al., Reference Helm, Lockley, Cawthra, De Vynck, Helm and Thesen2020c), sea turtles (Lockley et al., Reference Lockley, Cawthra, De Vynck, Helm, McCrea and Nel2019), and ‘sand-swimming’ golden moles (Lockley et al., Reference Lockley, Helm, Cawthra, De Vynck and Perrin2021). The presence of tracks of E. capensis on the same horizon as the sand-swimming traces (resembling those of Eremitalpa) is significant because Eremitalpa is characteristic of arid environments (Lockley et al., Reference Lockley, Helm, Cawthra, De Vynck and Perrin2021).
CONCLUSIONS
This study indicates that E. capensis was present on the Cape south coast through the late Middle and Late Pleistocene (based on the OSL dating range of 161 ± 12 ka to 43 ± 4 ka). These findings demonstrate the power of the trace fossil record to complement the body fossil record, and to provide new understandings of the distribution and regional prevalence of the species during the Pleistocene. The findings further illustrate the utility of integrating ichnological studies with archaeological and paleontological investigations. Equid tracks on the Cape south coast are readily separated into large and small categories. While current data indicate that large-equid tracks are identified more frequently, a larger sample size is needed to establish this with more certainty. The equid tracksites at Driefontein contribute to a sparse global record of long fossil horse trackways.
Supplementary Material
The supplementary material for this article can be found at https://doi.org/10.1017/qua.2023.1
Acknowledgements
We thank Jack Carrigan and Carina Helm, Johan Huisamen, Elbie Pama, Peter Todd, and Alex van den Heever for their assistance and support. Adam Cox is thanked for performing the ICP-MS and ICP-OES analyses. We thank Associate Editor Tyler Faith, reviewer Carlos Neto De Carvalho, and an anonymous reviewer for their insights and constructive suggestions, which significantly enhanced the manuscript.