INTRODUCTION
During the late Pleistocene most of the megafauna, both herbivores and carnivores, became extinct on all the major continents (Martin, Reference Martin1967, Reference Martin1984; Barnosky et al., Reference Barnosky, Koch, Feranec, Wing and Shabel2004). Previous studies on the cause of this megafaunal extinction in different parts of the globe have tended to focus on two primary causes, climatic and anthropogenic (Martin, Reference Martin1973; MacPhee, 1999; Miller et al., Reference Miller, Magee, Johnson, Fogel, Spooner, Mcculloch and Ayliffe1999; Grayson and Meltzer, Reference Grayson and Meltzer2002). The current rate of population reduction and potential extinction of herbivores and carnivores in the wild is a major global ecological issue. Currently about 60% of the large herbivorous animals are now threatened with possible extinction (Ripple et al., 2015). Southeast Asia contains the world’s highest number of threatened mammals (Schipper et al., Reference Schipper, Chanson, Chiozza, Cox, Hoffmann, Vineet and Lamoreux2008), with regional faunas experiencing ongoing range reductions and extinctions driven by human activities (Brook et al., Reference Brook, Dudley, Mahood, Polet, Williams, Duckworth, Van Ngoc and Long2014). In India, a preliminary report on the status of the mega-herbivores, including the greater one-horned rhinoceros (Rhinoceros unicornis, Linnaeus, 1758; also known as the Indian rhino) describes the high probability of their local extinction (Karanth et al., 2016).
The study of the dung of individual species is an important source of information on food preferences, habitat utilized, and ecology in general. Studies of fungal remains preserved in peat and lake sediments can complement palynodata in interpreting the paleovegetation and past climate in the region (van Geel, Reference van Geel1978, 1986, 2001; van Geel et al., Reference van Geel, Bohncke and Dee1981, 1989; Gill et al., Reference Gill, Williams, Jackson, Lininger and Robinson2009; Cugny et al., Reference Cugny, Mazier and Galop2010; Feeser and O’Connell, 2010; Kramer et al., 2010; Montoya et al., 2010; Mudie et al., 2010) and in archaeological sites (van Geel et al., Reference van Geel, Buurman, Brinkkemper, Schelvis, Aptroot, van Reenen and Hakbijl2003; Zong et al., Reference Zong, Chen, Innes, Chen, Wang and Wang2007; Gauthier et al., 2010; McAndrews and Turton, Reference McAndrews and Turton2010; Rattighieri et al., Reference Rattighieri, Rinaldi, Mercuri and Bowes2013; Revelles et al., Reference Revelles, Burjachs and van Geel2016). Studies have been carried out on coprophilous fungi in surface and sedimentary soil profiles to document or infer the former presence, and subsequent decline of, herbivorous animals in a region (Davis, 1987; Burney et al., Reference Burney, Robinson and Burney2003; Barnosky et al., Reference Barnosky, Koch, Feranec, Wing and Shabel2004; Robinson et al., Reference Robinson, Pigott Burney and Burney2005; Raper and Bush, Reference Raper and Bush2009). Feranec et al. (Reference Feranec, Miller, Lothrop and Graham2011) noted the need for more studies to better understand Sporormiella as a proxy and to identify whether particular taxa are only present on the dung of specific herbivores. Here we document the presence, types, and abundance of the coprophilous fungi and associated spores present in the dung of Rhinoceros unicornis in Kaziranga National Park, India. The generated dataset can serve as a critical proxy to document the former existence of rhinoceroses in a region through samples collected from the surface and sediment/soil sediments that preserve coprophilous fungi.
Rhinoceros unicornis is one of the largest living megaherbivores in the world and is now a critically endangered species (Poudyal et al., Reference Poudyal, Rothley and Knowler2009). One of the unique behaviors of rhinoceroses, including Rhinoceros unicornis is to consistently use the same location for their daily excretion and multiple individuals may deposit dung at this site or midden over several years. The historical distribution of Rhinoceros unicornis includes habitats in northern and central India, and Pakistan (Rao, Reference Rao1947; Banerjee and Chakraborty, 1973; Mathpal, Reference Mathpal1978), but Rhinoceros unicornis is absent in these regions today. The current distribution of Rhinoceros unicornis, now restricted to a few areas in the Assam region of India and Nepal, is considerably smaller than the historical distribution of the species.
STUDY SITE, FLORA, AND FAUNA
Kaziranga National Park is an ideal place for the investigation of Rhinoceros unicornis in its natural habitat and to understand the ecology of the species. The park has the highest population of Rhinoceros unicornis in the world and the population has been increasing at a positive rate from 366 individuals in 1966 to 2048 individuals in 2009 (Medhi and Saha, Reference Medhi and Saha2014). In 2015 the rhino population was 2401 (Sharma, 2016). The park lies between 26°32′ and 26°47′N, and 93°07′ E to 93°38′ E, at an elevation between 45–90 m above sea level (Fig. 1). The vegetation is mainly tropical, semi-evergreen, deciduous, savannah, and grassland (Champion and Seth, Reference Champion and Seth1968). A list of flora in the park is provided in Table 1.

Figure 1 (color online) (a) Location of the study area. (b) Land cover map of the Kaziranga National Park, India (modified after Das et al., Reference Das, Kumar, Bora, Verma, Gogoi, Gogoi and Vasu2014)
Table 1 Plant taxa present in the Kaziranga National Park, India.

The Kaziranga National Park has rich and diverse vertebrate fauna. Among the herbivores, along with the Rhinoceros unicornis (one-horned rhino; Fig. 2a), the other common mammalian herbivores are Bubalus arnee, Elephas maximus, Rusa unicolor, and Rucervus duvaucelii.

Figure 2 (a) Rhinoceros unicornis in its natural habit in Kaziranga National Park (b) A view of a field photograph during midden dung observation by Basumatary. (c) Sampling locations (red numbers) on Rhinoceros unicornis midden in forest area. (d) Sampling locations (red numbers) on Rhinoceros unicornis midden in grassland area. (For interpretations of the references to color in this figure legend, the reader is referred to the web version of this article.)
CLIMATE AND SOIL
The climate of the region is controlled by the southwest and northeast monsoons:, it is hot and humid during the summer, and cold and dry during winter. The maximum temperature ranges from a minimum of 4°C during winter up to 37°C in summer. The relative humidity is very high and ranges from 75 to 86%. The annual rainfall ranges from 1800 to 2600 mm, and annual flooding is very common in the Kaziranga National Park. The soil composition varies from site to site and includes sandy loam soil in forests, sandy soil in grassland, and clayey soil in the swamp and water bodies (Das et al., Reference Das, Kumar, Bora, Verma, Gogoi, Gogoi and Vasu2014).
MATERIAL AND METHOD
A total of 10 Rhinoceros unicornis dung samples (G1–G10), each consisting of ~100 g, were collected from the Rhinoceros unicornis dung midden close to the road within the grassland area in the western part of the Kaziranga National Park. Samples were collected from the center to periphery of the dung midden. Another 10 samples (F1–F10) of similar size were collected from a dung midden in the forested area close to the road in the central part of the park. The accumulations of rhino dung in the sites sampled are the result of consistent use by multiple individuals of Rhinoceros unicornis for at least several years. The middens were about 27.9–32.5 m2 in area and approximately 0.6 m in thickness. The dung samples were collected from above the ground level and below the surface of the dung to avoid contamination by the surface soil and atmospheric particles (Fig. 2b–d).
The dung samples were processed using the standard acetolysis method (Erdtman, Reference Erdtman1943). Samples were successively treated with 10% aqueous potassium hydroxide (KOH) solution to deflocculate the sediments, 40% hydrofluoric acid (HF) to dissolve silica, and acetolysis (9:1 anhydrous acetic acid to concentrated sulphuric acid, [H2SO4]). Thereafter, the samples were treated twice with glacial acetic acid (GAA), and washed 3 or 4 times with distilled water. The samples were then transferred to a 50% glycerol solution with a few drops of phenol to protect against microbial decomposition. Excluding pollen grain and fern spores, 421 to 470 fungal spores were counted from each sample to produce the fungal spore spectra. Observation of the fungal spores and microphotographs was performed using an Olympus BX-61 microscope with DP-25 digital camera under 40x magnification (Fig. 3). The identified fungal spores were categorized as coprophilous and non-coprophilous fungi. We consulted the literature as well as published papers to aid in identification of fungal spores (van Geel, 2003; Cugny et al., Reference Cugny, Mazier and Galop2010; Gross, 2011; Mungai et al., Reference Mungai, Hyde, Cai, Njogu and Chukeatirote2011; Basumatary et al., Reference Basumatary, Bera, Sangma and Marak2014; van Asperen et al., Reference van Asperen, Kirby and Hunt2016).
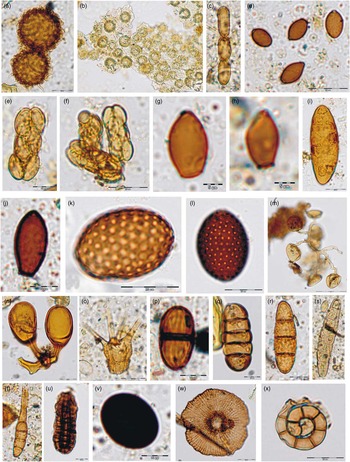
Figure 3 (color online) Fungal remains recovered from Rhinoceros unicornis dung midden samples in Kaziranga National Park, India. (a and b) Clumping of Ascodesmis. (c) Clumping of Sporormiella. (d) Group of Sporormiella. (e and f) Clumping of Saccolobus. (g and h) Cercophora. (i) Podospora. (j) Sordaria. (k and l) Gelasinospora. (m and n) Glomus with Hyphae. (o) Tetraploa. (p) Cookeina. (q and r) Meliola. (s) Helminthosporium. (t) Alternaria. (u) Dictyosporium. (v) Nigrospora. (w) Microthyriaceae. (x) Helicoon.
RESULTS
Fungal spore spectra
The fungal spectra in Rhinoceros unicornis dung samples from the forested and grassland area are listed in Tables 2 and 3. In the forested area, 10 dung samples (F1-F10) collected from the rhino dung midden located in the forested area of Kaziranga National Park (Fig. 1) were characterized by the predominance of coprophilous fungi (70.7%) over non-coprophilous fungi (29.3%). Among coprophilous fungi, Sporormiella (18.4%) was the most common, followed by Ascodesmis (17.4%) and Saccobolus (17.2%). Cercophora, Gelasinospora, Podospora, and Sordaria had values of 2.3% to 6.4%. The non-coprophilous fungi, chiefly the Microthyriaceae, Glomus, Tetraploa, Meliola, and Helminthosporium, were recorded within the range of 1.5% to 4.6% (Fig. 4).

Figure 4 Comparison of fungal spectra of Rhinoceros unicornis dung midden samples collected from the forest and grassland area in Kaziranga National Park, India.
Table 2 The fungal spore frequency data recovered from the rhino dung midden from the forest area. Numbers are given as percentages.

Table 3 The fungal spore frequency data recovered from the rhino dung midden from the grassland.

The 10 dung samples (G1-G10) collected in the grassland area from the Rhinoceros unicornis dung midden located in the forested area of Kaziranga National Park (Fig. 1) were also characterized by the dominance of coprophilous fungi (77.9%) over non-coprophilous fungi (22.1%). Among coprophilous fungi, the Sporormiella (20.3%), Ascodesmis (18.6%), and Saccobolus (18.3%) are the most common taxa, while Cercophora, Coniochaete, and Sordaria varied from 2.3 to 7.7%. The non-coprophilous fungi are chiefly Helminthosporium, Cookeina, Tetraploa, and Alternaria, which ranged from 1.0 to 4.9% (Fig. 4).
DISCUSSION
A total of 18 fungal spore types were identified in Rhinoceros unicornis dung midden samples collected both from grassland and forest area samples in Kaziranga National Park. The coprophilous fungi were predominant over non-coprophilous fungi in grassland and forested areas. The study reveals that Sporormiella, Saccobolus, and Ascodesmis were frequent and dominant in all of the studied samples. Baker et al. (Reference Baker, Bhagwat and Willis2013) listed spore types associated with megaherbivore dung based on empirical evidence and, while they included Sporormiella, neither Saccobolus nor Ascodesmis were included in their study. Richardson (Reference Richardson2001) documented that some taxa of cophrophilous fungi may have a preference for specific dung types and further research is needed to confirm whether these later two taxa are specific to Rhinoceros unicornis dung. However, other coprophilous fungi such as Cercophora, Sordaria, Podospora, and Gelasinospora listed by Baker et al. (Reference Baker, Bhagwat and Willis2013) were also consistently present in the assemblage from the Rhinoceros unicornis dung. It is, however, the presence of Sporormiella in the surface and lake soil sediments that currently is considered to serve as a powerful proxy for the present and past existence of herbivores and birds as a part of the paleoecological reconstruction of a region (van Geel et al., Reference van Geel, Buurman, Brinkkemper, Schelvis, Aptroot, van Reenen and Hakbijl2003; Graf and Chmura, Reference Graf and Chmura2006; Raper and Bush, Reference Raper and Bush2009; Parker and Williams, 2012; Etienne et al., Reference Etienne, Wilhelm, Sabatier, Reyss and Arnaud2013). The presence of the spores of Sporormiella greater than 2% in a pollen sample is considered a strong indication of the presence of megafauna in the region (Davis, Reference Davis1987; Raczka et al., Reference Raczka, Bush, Folcik and McMichael2016). Feranec et al. (Reference Feranec, Miller, Lothrop and Graham2011) noted that more taphonomic study is needed on how spores of coprophilous fungi enter the stratigraphic record and to identify the preservation potential of Sporormiella spores in different habitat and sediment types. They cite Nyberg and Persson (Reference Nyberg and Persson2002), who show that fungal diversity in moose (Alcesalces) dung was promoted in pine forest but suppressed in spruce forest. Our study partially addresses the issue of differential spore preservation. There does not appear to be any difference in the relative abundance of fungal spores in the dung of Rhinoceros unicornis recovered from two distinct habitats, forest and grassland. Similarly, the presence of Sporormiella in Pleistocene samples have been used as a direct indicator for the presence of extinct megaherbivores based on the study of mammoth (Mammuthus columbi) dung recovered in Bechan Cave (southern Utah) (Davis, Reference Davis1987). The clumping of coprophilous fungi spores, especially Sporormiella, Saccobolus, and Ascodesmis, in the Rhinoceros unicornis dung midden samples was very common and indicative of their local origin. In coprophilous fungi, especially Sporormiella, the spores have low dispersal capacity and produce localized concentrations rather than being dispersed across the region and generally are transported less than 100 m from the source area (Davis and Shafer, Reference Davis and Shafer2006; Raper and Bush, Reference Raper and Bush2009; Parker and Williams, 2012; Gill et al., Reference Gill, McLauchlan, Skibbe, Goring, Zirbel and Williams2013). The fungal spores are therefore strictly local in origin and become preserved close to the source where sporulation occurred (van Geel and Aptroot, Reference van Geel and Aptroot2006). In our study of Rhinoceros unicornis dung midden samples, the Sporormiella–Saccobolus–Ascodesmis assemblage was a strong indicator of rhinoceros, as indicated by their regular presence and high abundance in all the samples.
The high amount of coprophilous fungi along with other associated fungal spores is indicative of the warm and humid condition in the region because water availability is the important factor for the germination and sporulation of Sporormiella (Austin, 1958; Ingold and Marshall, 1962; Kuthubutheen and Webster, Reference Kuthubutheen and Webster1986a, Reference Kuthubutheen and Webster1986b). The presence of Cercophora in the assemblage has also been observed in dung and is also an indicator of woodland and grassland environments (Blackford and Innes, Reference Blackford and Innes2006; Graf and Chmura, Reference Graf and Chmura2006). The Sporormiella–Saccobolus–Ascodesmis assemblage is considered specifically characteristic for Rhinoceros unicornis dung based on their abundance in the dung midden samples. In our dataset, it is observed that, in addition to the Sporormiella, the other two taxa, Saccobolus, and Ascodesmis, were most frequently present with values >15%. The Sporormiella–Saccobolus–Ascodesmis assemblage is characteristic of rhino dung, as indicated by their consistent high frequency (16–21%) and clustering spores in all the examined samples. In contrast, non-coprophilous fungi such as Tetraploa, Cookeina, Meliola, and Dictyosporium have a relatively low presence in the assemblage and the consistency of their presence in the dung needs more investigation. The high body temperature (~37°C) and acidic environment in the gut of herbivorous animals may be the main reason that non-coprophilous fungal spores are destroyed if they are consumed during feeding.
The continuous and comparatively high presence of Microthyriaceae (epiphyllous fungi) and Helicoon in the dung samples collected from the forest area was very significant (1–2%). The presence of such fungi in the assemblage is indicative of the dense forest vegetation under warm and humid conditions in response to the high rainfall in the region (Cookson, Reference Cookson1947; Selkirk, 1975; Reddy et al., 1982; Johnson and Sutton, 2000; Limaye et al., Reference Limaye, Kumaran, Nair and Padmalal2007; Hofmann, 2010; Medeanic and Silva, Reference Medeanic and Silva2010). The comparatively high value of Helminthosporium (3.7%) in the assemblage of grassland dung samples is of interest, as it is a common pathogen in grasses. The presence of Tetraploa and Glomus in the assemblage of both area samples suggests water-logged conditions with rich plant diversity that might be incorporated through the ingestion of plants, water, and soil, as these fungi are commonly found on leaf bases, roots, and stems of Poaceae and Cyperaceae (Ellis, 1971; Tanaka et al., Reference Tanaka, Hirayama, Yonezawa, Hatakeyama, Harada, Sano, Shirouzu and Hosoya2009).
CONCLUSIONS
This study demonstrates that the Sporormiella–Saccobolus–Ascodesmis assemblage is distinctive and characteristic of Rhinoceros unicornis dung. The documentation of the coprophilous fungi present in surface soil sediments in the region can complement the data provided by the analysis of the Rhinoceros unicornis dung midden samples in Kaziranga National Park and its vicinity. The resulting fungal dataset on Rhinoceros unicornis dung can provide a baseline that can help us to document the past presence of Rhinoceros unicornis based on the study of sedimentary soil profile in Kaziranga National Park and neighboring regions. The dataset also can serve as a powerful tool to determine the past distribution and ecology of Rhinoceros unicornis in India and neighboring areas when other evidence such as bones are not available. Combined with a study of the pollen and fungal spores preserved in the dung of other herbivores animals in the region, this approach provides a way of recognizing the distribution of other animals that are found in the same habitat as Rhinoceros unicornis prior to their extirpation. Further research is needed that includes surface and sedimentary soil samples beyond the perimeter of the Rhinoceros unicornis dung midden to determine if they preserve a different or similar fungal spore assemblage than that seen in the Rhinoceros unicornis dung midden samples.
ACKNOWLEDGMENTS
We thank Prof. Sunil Bajpai, Director, Birbal Sahni Institute of Palaeosciences (BSIP), Lucknow, India for laboratory facilities to carry out this research. First author is very much thankful to Department of Science and Technology, New Delhi for funding (DST No: SB/EMEQ-225/2014 (SERB) the research work. First author acknowledge the forest department, Goverment of Assam for necessary help during field observation. Authors are also thankful to Mr. Sandeep K. Kohri, Project Assistant, for assistance in the samples maceration and technical help during the preparation of the manuscript.