Introduction
Chimeric antigen receptor (CAR) T-cell therapy is a novel type of cellular immunotherapy in which a patient’s T cells are engineered in vitro to target and eliminate cancer cells in vivo. In CAR-T treatment, the T cells from a patient’s blood are extracted by apheresis. The gene for a specific receptor (CAR) which binds to a certain tumor target is delivered to the T cells by viral vector or nonviral transposon methods (Srivastava and Riddell, Reference Srivastava and Riddell2015; Jain and Davila, Reference Jain and Davila2018; Ittershagen et al., Reference Ittershagen, Ericson, Eldjerou, Shojaee, Bleickardt, Patel, Taran, Anak, Hall, Leung, Roccoberton, Salmon, Fuchs, Romanov and Lebwohl2019). At present, two anti-CD19 CAR-T products have been approved by the U.S. FDA for the treatment of B-cell acute lymphoblastic leukemia and non-Hodgkin lymphoma; CAR-T therapy for other cancer types are undergoing vigorous clinical studies. CAR-T therapy holds great promise for treating hematologic malignancies, and recent clinical evidence has indicated that similar approaches can also be used to treat solid tumors (Baybutt et al., Reference Baybutt, Flickinger, Caparosa and Snook2019).
However, there are several potentially fatal side effects during CAR-T treatment including: cytokine release syndrome (CRS), neurologic events, neutropenia and anemia (Xu and Tang, Reference Xu and Tang2014). Among all the side effects, CRS is significant and can be life-threatening. Cytokines are essential immune mediators. Yet, a large and rapid release of cytokines into the blood from immune cells can induce a ‘cytokine storm’, or CRS, which is associated with systemic symptoms of various severity. Most patients with CRS develop a mild flu-like reaction such as fever, fatigue, headache and rash. However, the reaction may progress to an uncontrolled, systemic inflammatory response with extreme pyrexia and become life-threatening (Shimabukuro-Vornhagen et al., Reference Shimabukuro-Vornhagen, Godel, Subklewe, Stemmler, Schlosser, Schlaak, Kochanek, Boll and Von Bergwelt-Baildon MS2018).
CRS is not manifested only as a side effect of cellular immunotherapy. It can also be triggered by viral infections such as influenza and hepatitis virus (de Jong et al., Reference De Jong, Simmons, Thanh, Hien, Smith, Chau, Hoang, Chau, Khanh, Dong, Qui, Van Cam, Ha, Guan, Peiris, Chinh, Hien and Farrar2006; Tisoncik et al., Reference Tisoncik, Korth, Simmons, Farrar, Martin and Katze2012; Savarin and Bergmann, Reference Savarin and Bergmann2018). The current Coronavirus Disease 2019 (COVID-19) triggers CRS in many stages of its pathological course that causes lung fibrosis, acute respiratory distress syndrome, and eventually leads to multi-organ failure (Huang et al., Reference Huang, Wang and Li2020; Xu et al., Reference Xu, Shi, Wang, Zhang, Huang, Zhang, Liu, Zhao, Liu and Zhu2020). Other conditions, including graft-versus-host disease, sepsis, Ebola, avian influenza, smallpox and systemic inflammatory response syndrome, also involve extensive release of undesired cytokines (Drazen et al., Reference Drazen, Goldman and Bennett2000). To alleviate the symptoms and treat the disease, it is important to remove the excessive cytokines efficiently and rapidly.
The chemokine receptor CCR9, together with its ligand CCL25, contribute to intestinal homing of T cells. This signaling pathway promotes invasion, metastasis, anti-apoptosis and drug-resistance in many types of cancer (Hu et al., Reference Hu, Zhang, Wu, Han, Jia, Jiang, Cheng, Gan, Tao and Zhang2011; Tu et al., Reference Tu, Xiao, Xiong, Tembo, Deng, Xiong, Liu, Wang and Zhang2016). Specifically, CCR9 is aberrantly expressed in acute and chronic T-cell leukemias that contribute to the aggressiveness of the diseases (Somovilla-Crespo et al., Reference Somovilla-Crespo, Monzon, Vela, Corraliza-Gorjon, Santamaria, Garcia-Sanz and Kremer2018). Targeting CCR9 (such as anti-CCR9 monoclonal antibodies with high specificity, affinity and stability) is a rational therapeutic strategy for these diseases.
We have previously devised a novel tool called ‘QTY code’ which regulates the water solubility of redesigned membrane proteins through pairwise substitution of hydrophobic amino acids by hydrophilic ones (Zhang et al., Reference Zhang, Tao, Qing, Tang, Skuhersky, Corin, Tegler, Wassie, Wassie, Kwon, Suter, Entzian, Schubert, Yang, Labahn, Kubicek and Maertens2018). Hydrophobic amino acids Leu, Val, Ile and Phe are exchanged for hydrophilic Gln, Thr and Tyr in the transmembrane regions of a receptor, based on the structural and electron density map similarity in their side chains. It has been shown that this protein design approach enables the solubilization of many types of chemokine receptors with tunable functionality (Qing et al., Reference Qing, Han, Skuhersky, Chung, Badr, Schubert and Zhang2019). The QTY code also provides flexibility in studying the physiological and functional properties of transmembrane receptors as well as promoting their utilization, without the requirements of time consuming and expensive detergent screening or use of nanodisks.
We, here, report the QTY code design of six types of cytokine receptors including two variants of chemokine receptors CCR9 and CXCR2, two variants of interleukin receptors, IL4Rα and IL10Rα, as well as two variants of interferon receptors, IFNγR1 and IFNλR1. These QTY code-designed receptors show ligand-binding properties similar to their native receptor counterparts without the presence of hydrophobic patches. The receptors were fused with Fc domain of mouse IgG2a protein to form an antibody-like structure. These Fc-fusion receptors can be expressed and purified in an Escherichia coli system with sufficient yield (~mg/l) in lysogeny broth (LB) media. We also showed that these QTY receptors are capable of binding to their respective ligands with affinity close to isolated native receptors on solution-based assays. These QTY code designs of functional, water-soluble Fc-fusion cytokine receptors can potentially be used clinically as decoy therapy to rapidly remove excessive cytokines in the setting of hyperactive immune reactions during CRS including current COVID-19 severely infected patients.
Results
Design of Fc-fusion QTY variant cytokine receptors
Six types of cytokine receptors were selected and redesigned by QTY code. Two variants of chemokine receptors, belonging to the 7-transmembrane (7-TM) G protein-coupled receptor (GPCR) family, and four types single-transmembrane interleukin and interferon receptors were chosen. The L, I, V and F amino acid residues in the transmembrane region of the corresponding receptors were replaced by Q, T and Y accordingly.
Similar to our previous reports for chemokine receptors, sequences of QTY code-designed CCR9 and CXCR2 were aligned with native receptors to compare substitutions of amino acids (Fig. 1a,b). QTY substitutions were applied to all corresponding residues but only to the 7-TM region. Amino acid changes were highlighted in red color denoting an exchange. Residues in extracellular domain (EC, colored black) or intracellular domain (IC, colored yellow) were untouched. Molecular weight of the QTY variant receptors are slightly increased due to the higher molecular mass of Q, T and Y as compared to L, I, V and F. Despite a total difference of 26.0% (46.4% in 7-TM) in the primary sequence for CCR9QTY, and 25.5% (58.9% in 7-TM) for CXCR2QTY, the changes in the isoelectric point (pI) of the redesigned proteins were only 0.05 and 0.06 units, respectively. This is attributed to the nonionic nature of Q, T and Y as these amino acids do not introduce significant changes in the net charge of a protein. Rather, the substituted amino acids form numerous intra-helical and inter-helical hydrogen bonds that contribute to the structural integrity as well as those to the surrounding water molecules that enhance the overall solubility of a protein (Qing et al., Reference Qing, Han, Skuhersky, Chung, Badr, Schubert and Zhang2019).
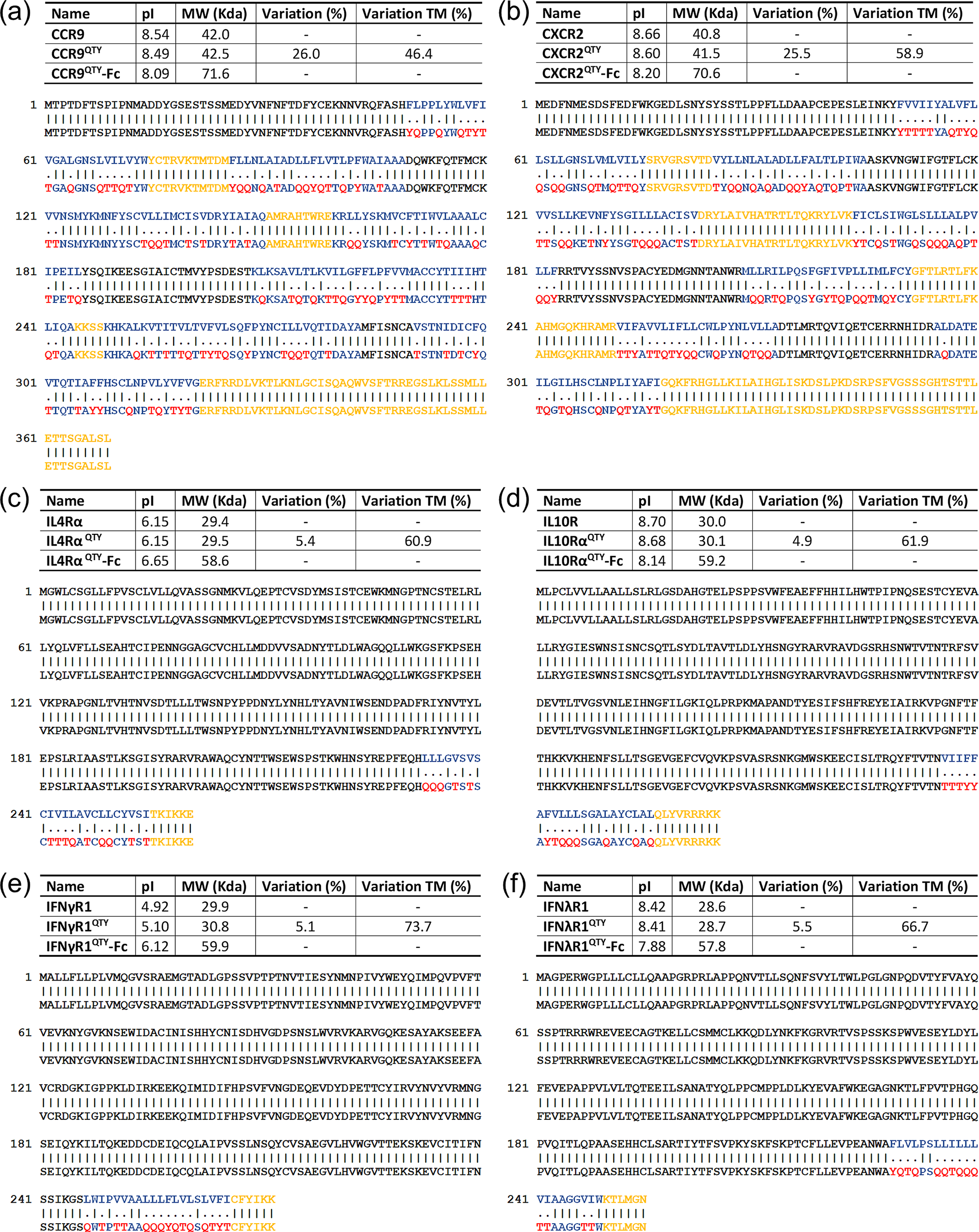
Fig 1. Protein sequence alignment between natural (Top) and QTY redesigned cytokine receptors. (a) CCR9 versus CCR9QTY; (b) CXCR2 versus CXCR2QTY; (c) IL4Rα versus IL4RαQTY; (d) IL10Rα versus IL10RαQTY; (e) IFNγR1 versus IFNγR1QTY and (f) IFNλR1 versus IFNλR1QTY. The substitutions of Q, T, and Y are denoted with ‘.’, while ‘|’ indicates no change in residues between the two sequences. The Q, T and Y amino acid substitutions are colored in red. The N-terminus, extracellular loops are black, transmembrane is blue and intracellular components of the receptors are yellow. Characteristics of native, QTY variant–Fc-fusion receptor proteins’ pI, molecular weight, and overall variation rate and that % changes only transmembrane segments are presented.
Both interferon receptors and interleukin receptors have a single-pass transmembrane domain. The ligand binding domain is typically comprised of multiple stranded β-sheets that form two connected anti-parallel β-barrels. The β-barrels are connected to the transmembrane α-helix which is responsible for signal transduction, presumably playing a role in ligand interaction (Richter et al., Reference Richter, Moraga, Winkelmann, Birkholz, Wilmes, Schulte, Kraich, Kenneweg, Beutel, Selenschik, Paterok, Gavutis, Schmidt, Garcia, Muller and Piehler2017). In order to best mimic a native receptor, we included the transmembrane domain in our design with a few amino acids in the cytoplasmic region to serve as a short linker so as to optimize the binding and structure of the QTY code modified receptors.
Similar to chemokine receptors, the QTY code was only applied to the transmembrane domains of these receptors, as shown in Fig. 1c–f. Amino acid exchanges colored in red are selected to eliminate the hydrophobic patches in the designed receptors. Both extracellular domains and intracellular linkers are untouched. Due to the relative weight of TM region, the changes in molecular weight of interferon and interleukin receptors were minimal. pI changes are 0.00, 0.02, 0.18 and 0.01 for IL4RαQTY, IL10RαQTY, IFNγR1QTY and IFNλR1QTY, respectively. The larger pI change in IFNγR1QTY was probably due to its larger deviation towards a charge neutral point as compared to other receptors as shown in Fig. 1 (5.10 for IFNγR1QTY compared to 6.15 for IL4RαQTY, 8.68 for IL10RαQTY and 8.41 for IFNλR1QTY).
We specifically designed the QTY receptor variants to fuse with the Fc region of IgG protein in order to acquire an antibody-like structure. The primary benefit of Fc-fusion is to significantly enhance the half-life of the fused protein in human plasma. It can also improve the safety profile of the fused proteins due to reduced immunogenicity whereas synergistic therapeutic effects from both fusion parts is achievable (Levin et al., Reference Levin, Golding, Strome and Sauna2015). On the other hand, the Fc-fusion is naturally a homodimer through covalent bond. They can be easily tuned to form higher order multimeric states with enhanced stability and efficacy (Czajkowsky et al., Reference Czajkowsky, J, Shao and Pleass2012). Additionally, Fc-fusion further enhances the solubility of QTY-designed cytokine receptors, and serves as an affinity tag for protein purification, as well as for utilization in affinity-based vehicles (beads) for drug delivery. A spacer was introduced to optimize the conformation of QTY-designed receptors in the heavy chain. We used the Fc region of mouse IgG2a in the specific design as it is the functional equivalent of human IgG1. Mouse IgG is chosen over human IgG due to the consideration of implementing mouse cytokine storm model in subsequent experiments, beyond the scope of the current study. The Fc region can be easily exchanged in future designs. Fig. 2 shows a schematic illustration of these cytokine receptor–Fc complex for the six QTY receptor variants. The structural illustrations of corresponding cytokine receptors were obtained through PDB (Protein Data Bank) where applicable (Thiel et al., Reference Thiel, Le Du, Walter, D’arcy, Chene, Fountoulakis, Garotta, Winkler and Ealick2000; Yoon et al., Reference Yoon, Jones, Logsdon and Walter2005; Miknis et al., Reference Miknis, Magracheva, Li, Zdanov, Kotenko and Wlodawer2010; Moraga et al., Reference Moraga, Richter, Wilmes, Winkelmann, Jude, Thomas, Suhoski, Engleman, Piehler and Garcia2015; Oswald et al., Reference Oswald, Rappas, Kean, Dore, Errey, Bennett, Deflorian, Christopher, Jazayeri, Mason, Congreve, Cooke and Marshall2016) or from a homology model (CXCR2) (Kwon, Reference Kwon2010). The molecular weight and pI for each of the cytokine receptor–Fc proteins were also denoted in Fig. 1.

Fig. 2. Schematic illustration for Fc-fused QTY variant cytokine receptors with antibody-like structure. (a) CCR9QTY–Fc; (b) CXCR2QTY–Fc; (c) IL4RαQTY–Fc; (d) IL10RαQTY–Fc; (e) IFNγR1QTY–Fc; (f) IFNλR1QTY–Fc. These illustrations are not to scale and the receptors parts are significantly emphasized for clarity.
Bioinformatics analysis
QTY variant protein sequences were analysed using a web-based tool TMHMM Server v2.0 to predict the existence of hydrophobic transmembrane segments. The server is based on a Hidden Markov Model that takes into account actual biological architectures of a transmembrane helix whereas likelihood of presence is calculated (Sonnhammer et al., Reference Sonnhammer, Von Heijne and Krogh1998).
In Fig. 3, the hydrophobicity of a protein is plotted versus the protein sequences. The X-axis shows the number of amino acids in sequences from N-terminus to C-terminus. Both native sequences (top row) and QTY variant receptor sequences (bottom row) were analysed and aligned. It is apparent that native CCR9 (Fig. 3a) and CXCR2 (Fig. 3b) exhibits seven distinctive high-probability hydrophobic segments, corresponding to the 7-TM domains. The segments disappear in QTY designed counterparts. In interleukin and interferon receptors, there is only a single high probability hydrophobic segment near the C-terminus end of each receptor which is also eliminated though QTY modification. The hydrophobicity of both extracellular and intracellular components is unchanged.

Fig. 3. Bioinformatic predictions of cytokine receptors with hydrophobic segment of native (top) and QTY variant (bottom). The hydrophobicity probability of a protein is plotted vs the sequence. (a) CCR9QTY–Fc; (b) CXCR2QTY–Fc; (c) IL4RαQTY–Fc; (d) IL10RαQTY–Fc; (e) IFNγR1QTY–Fc and (f) IFNλR1QTY–Fc. Color code: pink line, extracellular regions; red line, transmembrane regions and blue line, intracellular region.
E. coli expression and gel-electrophoresis of QTY variant receptors
The corresponding genes with E. coli specific codons were synthesized and expressed in sufficient quantities. The throughput for each receptor differed but was all in the mg/l range in LB media. All Fc-fusion receptors were expressed into inclusion bodies. They were purified by (a) affinity purification and (b) gel filtration in denatured state and then folded into functional state for subsequent analysis. Both arginine and dithiothreitol (DTT) were beneficial for solubilizing the proteins so either or both of them were included in the storage buffer or for ligand binding tests.
The gel-electrophoresis results for purified Fc-fusion QTY variant receptors are shown in Fig. 4. All interleukin and interferon receptors exhibited monomer bands that corresponded well with their respective molecular weight. For the two chemokine receptors, there are several bands above the monomer bands. It is plausible that these bands can be attributed to a dimeric or higher order of multimeric receptors.

Fig. 4. Gel-electrophoresis of purified QTY code designed Fc-fusion receptors. (a) CXCR2QTY–Fc; (b) CCR9QTY–Fc; (c) IL4RαQTY–Fc; (d) IL10RαQTY–Fc; (e) IFNγR1QTY–Fc and (f) IFNλR1QTY–Fc. The molecular weight of the ladder is labelled on the left in KDa. It is plausible that these bands can be attributed to dimeric or higher order of multimeric receptors. For panels d and f, the bands are likely to be impurities that are too close to the target band which we were not able to separate with either His-tag or gel-filtration purification. These bands might be eliminated with further Protein A/G purification in future experiments
Ligand-binding measurement in buffer
The affinity of QTY modified cytokine receptors fused with Fc of IgG for their respective native ligands was measured using microscale thermophoresis (MST). Changes in thermophoretic movement for labelled proteins upon ligand-binding were recorded and plotted as a function of ligand concentration. Both QTY code-designed Fc-fusion interleukin and interferon receptors showed no nonspecific adhesion or aggregation during the measurement. The binding data were obtained in early T-Jump period, where rapid changes in fluorophore properties induced by fast temperature change was recorded (Jerabek-Willemsen et al., Reference Jerabek-Willemsen, Andre, Wanner, Roth, Duhr, Baaske and Breitsprecher2014) because two 7-TM chemokine receptors exhibited minor aggregation during prolonged incubation. For better visualization, the data were replotted as bound fraction versus concentration with a scale of 0–1, as shown in Fig. 5. The plot was then used to calculate the dissociation constant (K d) value for receptor–ligand interaction using the K d model, as presented in the Materials and Methods section. The fitted curves for K d calculation are also presented in graphs for illustration.

Fig. 5. Microscale thermophoresis (MST) ligand-binding measurements. The receptors were labelled with fluorescent dye. The ligands were purchased commercially from and dissolved in deionized water. (a) CCR9QTY–Fc with CCL25; (b) CXCR2QTY–Fc with IL8; (c) IL4RαQTY–Fc with IL4; (d) IL10RαQTY–Fc with IL10; (e) IFNγR1QTY–Fc with IFNγ and (f) IFNλR1QTY–Fc with IL29. The K d values calculated from the graphs are listed in Table 1.
These QTY Fc-fusion receptors exhibit affinity for their respective ligands typically in a range of up to tens of nM (Table 1). The affinities are lower compared to the native receptors without Fc-fusion. The binding affinity of CXCR2QTY–Fc with IL8 (60.3 ± 21.0 nM) is much lower compared to cell-based assay, for example, monomeric IL8 binding with native CXCR2 (0.5 ± 0.3 nM) but closer to IL8 in dimeric state (8.5 ± 2.0 nM) (Rajarathnam et al., Reference Rajarathnam, Prado, Fernando, Clark-Lewis and Navarro2006). Caccuri et al. (Reference Caccuri, Giagulli, Bugatti, Benetti, Alessandri, Ribatti, Marsico, Apostoli, Slevin, Rusnati, Guzman, Fiorentini and Caruso2012) also reported a dissociation constant (70 nM) that is similar to our affinity measurement of CXCR2QTY–Fc with IL8. For affinities of interleukin and interferon receptors, previously-reported studies primarily used human neutrophil cell-based assays with isotope 125I-labelled ligand that is significantly more sensitive than using the purified receptors measured by biophysical instrument; thus, they may not be directly comparable. The affinity K d derived from MST displays similar values compared to previous SPR measurement on purified proteins with the exception of IL4RαQTY–Fc. The method that was used to determine the K d in literature is also included in Table 1.
Table 1. Ligand-binding affinity of Fc-fused QTY cytokine receptors

The methods used for are included after the reported values. The references for reported K d values are also cited.
Different types of cytokines are aberrantly expressed during CRS in various pathological conditions. Together with many other types of cytokines, levels of the interleukins, IL-8 and IL-10 are elevated over 10 times and 50 times to ~101.7 and ~ 100.8 pg/ml, respectively, in the peripheral blood in a nonfatal infection of influenza A (H5N1) (de Jong et al., Reference De Jong, Simmons, Thanh, Hien, Smith, Chau, Hoang, Chau, Khanh, Dong, Qui, Van Cam, Ha, Guan, Peiris, Chinh, Hien and Farrar2006). Infection with Francisella tularensis can lead to an accumulation of excessive IFN-γ, IL-10 and IL-8 to respective levels of ~700, ~1 and ~4 ng/ml in the lungs (Sharma et al., Reference Sharma, Mares, Li, Morris and Teale2011). A more recent study on COVID-19 indicates a high-level expression of IL-6 in the blood with an average of 7 and 12 ng/ml detected in discharged and expired patients, respectively (Ruan et al., Reference Ruan, Yang, Wang, Jiang and Song2020). By carefully choosing the vehicle and delivery mechanism such levels of cytokines are surely treatable by our designed Fc-fused QTY cytokine receptors. Further data and information from ongoing research with in vivo and in vitro cytokine release assays as well as mouse animal model studies will be presented in separate reports.
Discussion
In this study, the successful design of QTY code-modified variant chemokine receptors CCR9QTY and CXCR2QTY further expands the plausible applicability of such a protein design algorithm on 7-TM GPCRs. Combined with our prior work (Zhang et al., Reference Zhang, Tao, Qing, Tang, Skuhersky, Corin, Tegler, Wassie, Wassie, Kwon, Suter, Entzian, Schubert, Yang, Labahn, Kubicek and Maertens2018, Qing et al., Reference Qing, Han, Skuhersky, Chung, Badr, Schubert and Zhang2019), our laboratory has successfully designed and engineered eight variants of soluble GPCRs while retaining their physiological and functional properties, including seven variants of chemokine receptors and one variant of olfactory receptor. The general applicability of the QTY code for GPCRs may further promote the study of these previously difficult targets in a functionally equivalent form. It is likely that the QTY code may be generally applied to other types of multi-pass membrane proteins and difficult-to-express proteins. Those studies are ongoing.
Although truncated soluble interleukin and interferon receptors also exist in vivo, primarily by cleavage between the extracellular and transmembrane segments, the QTY code is still meaningful for designing these single transmembrane receptors for additional studies. While for some receptors the transmembrane α-helix appears to play a role in ligand interaction (Richter et al., Reference Richter, Moraga, Winkelmann, Birkholz, Wilmes, Schulte, Kraich, Kenneweg, Beutel, Selenschik, Paterok, Gavutis, Schmidt, Garcia, Muller and Piehler2017), inclusion of the QTY-designed water-soluble transmembrane helical segment in the entire receptors may contribute to the overall understanding of receptor functional mechanism and signal transduction. On the other hand, not all native-form soluble interleukin and interferon receptors can be readily synthesized and purified in a high-throughput low cost E. coli system. In addition, integral multi-transmembrane cytokine receptors such as CCR9 and CXCR2 do not have correspondingly truncated soluble segments.
Our Fc-fusion water-soluble receptor may be able to rapidly soak up excessive cytokines from a cytokine storm unleashed during CAR-T treatment and COVID-19. When the QTY-designed water-soluble Fc-receptors bind to excessive cytokines, they may inhibit excessive cytokine interaction with target cells, thereby reducing the organ damage and toxicity. There are over 20 Fc-fusion proteins commercially available and several of these have been developed as therapeutics (Czajkowsky et al., Reference Czajkowsky, J, Shao and Pleass2012). Although there have been many Fc-fusion proteins developed for various applications, they are water-soluble proteins in the native state (Mekhaiel et al., Reference Mekhaiel, Czajkowsky, Andersen, Shi, El-Faham, Doenhoff, McIntosh, Sandlie, He, Hu, Shao and Pleass2011; Czajkowsky et al., Reference Czajkowsky, J, Shao and Pleass2012). Our QTY code designed Fc-fusion receptors, especially chemokine receptors CCR9 and CXCR2, provide a novel platform for further design of other types of fusion membrane receptors for therapeutic and diagnostic applications.
The QTY code design and synthesis of functionally equivalent transmembrane receptor proteins have implications beyond biological and clinical use. Highly specific membrane receptors towards their respective ligands, QTY code modified transmembrane proteins can also serve as ideal candidates for molecular sensing. Complex electrical arrays functionalized with a variety types of water-soluble membrane proteins can potentially mimic cell response in vitro and be fabricated into a pseudo cell with electrical readouts.
Materials and methods
Genes identification and QTY modification
Sequences of the selected proteins were obtained from Uniprot: https://www.uniprot.org/. The respective extracellular, transmembrane and cytoplasmic domains were identified. The QTY code was only applied to the transmembrane helical domain to solubilize the proteins.
Bioinformatics analysis
Protein properties were calculated based on their primary sequences via the open access web-based tool ExPASy: https://web.expasy.org/protparam/. The existence of hydrophobic segments within the transmembrane region in native and QTY variant protein sequences was determined via the open access web-based tool TMHMM Server v.2.0: http://www.cbs.dtu.dk/services/TMHMM-2.0/.
E. coli expression system and protein purification
Genes of QTY modified cytokine receptor proteins were cloned into the Fc region of mouse IgG2a as the functional equivalent of human IgG1. The full sequences were codon-optimized for E. coli expression and obtained from Genscript. The genes were cloned into pET20b expression vector with Carbenicillin resistance. The plasmids were reconstituted and transformed into E. coli BL21(DE3) strain. Transformants were selected on LB medium plates with 100 μg/ml Carbenicillin. E. coli cultures were grown at 37°C until the OD600 reached 0.4–0.8, after which IPTG (isopropyl-d-thiogalactoside) was added to a final concentration of 1 mM followed by 4-h expression. Cells were lysed by sonication in B-PER protein extraction agent (Thermos-Fisher) and centrifuged (23,000g, 40 min, 4°C) to collect the inclusion body. The biomass was then subsequently washed twice in buffer 1 (50 mM Tris-HCl pH 7.4, 50 mM NaCl, 10 mM CaCl2, 0.1%v/v Trition X100, 2 M Urea, 0.2 μm filtered), once in buffer 2 (50 mM Tris-HCl pH 7.4, 1 M NaCl, 10 mM CaCl2, 0.1%v/v Trition X100, 2 M Urea, 0.2 μm filtered) and again in buffer 1. Pellets from each washing step were collected by centrifugation (23,000g, 25 min, 4°C).
Washed inclusion bodies were fully solubilized in denaturation buffer [6 M guanidine hydrochloride, 1× phosphate buffered saline (PBS), 10 mM DTT, 0.2 μm filtered] at room temperature for 1.5 h with magnetic stirring. The solution was centrifuged at 23,000g for 40 min at 4°C. The supernatant with proteins was then purified by Qiagen Ni-NTA beads (His-tag) followed by size exclusion chromatography using an ÄKTA Purifier system and a GE healthcare Superdex 200 gel-filtration column. Purified protein was collected and dialysed twice against renaturation buffer (50 mM Tris-HCl pH 9.0, 3 mM reduced glutathione, 1 mM oxidized glutathione, 5 mM ethylenediaminetetraacetic acid and 0.5 M l-arginine). Following an overnight refolding process, the re-natured protein solution was dialysed into storage buffer of 50 mM Tris-HCl pH 9.0 with various arginine content.
Microscale thermophoresis
MST is an optical method detecting changes in thermophoretic movement and temperature related intensity change of the protein-attached fluorophore upon ligand binding. Active labelled proteins contribute to the thermophoresis signal upon ligand binding. Inactive proteins influence the data as background but not the signals and only data from binding proteins are used to derive the K d value. Herein ligand binding experiments were carried out with 5 nM NT647-labelled protein in 1× PBS, 10 mM DTT buffer with different concentration of arginine, against a gradient of respective ligands on a Monolith NT.115 pico instrument at 25°C. Synthesized receptors were labelled with Monolith NT second generation protein labelling kit RED – NHS (NanoTemper Technologies) so as to obtain unique fluorescent signals. MST time traces were recorded and analysed to obtain the highest possible signal-to-noise levels and amplitudes, >5 Fnorm units. Multiple rounds of buffer optimization were conducted for CXCR2QTY–Fc and CCR9QTY–Fc receptors. The data in optimized buffer was reported. The buffer condition was then adopted directly by Fc-fused QTY interleukin and interferon variants. The recorded fluorescence was plotted against the concentration of ligand, and curve fitting was performed using the K d fit formula derived from the law of mass action. For clarity, binding graphs of each independent experiment were normalized to the fraction bound (0 = unbound, 1 = bound). MST experiments were performed in the Centre for Macromolecular Interactions at Harvard Medical School.
Kdfitting model
K d model is the standard fitting model based on law of mass action.
Curve fit formula:


where F u is the fluorescence in unbound state; F b the fluorescence in bound state; K d the dissociation constant, to be determined; c AT the concentration of formed complex; c A the constant concentration of molecule A (fluorescent), known and c T the concentration of molecule T in serial dilution.
Open Peer Review
To view the open peer review materials for this article, please visit https://doi.org/10.1017/qrd.2020.4.
Acknowledgements
This work was primarily funded by Avalon GloboCare Corp. Shilei Hao gratefully acknowledges the fellowship of China Scholarship Council No. 201808505038 and Chongqing University, China. We also thank Dorrie Langsley for her quick turn-around of English editing of this paper.
Comments
Comments to Author: This manuscript is a very interesting follow-up on previous reports from Zhang and coworkers on the QTY code and its application to solubilize membrane protein receptors. Here, QTY variants of cytokine receptors were further fused to the Fc domain of (a mouse) IgG. The authors highlight the potential use of these fusion constructs for treatment of various cytokine disorders, which is of great medical relevance. In essence, the authors suggest that the receptor-Fc fusion proteins could serve as antibody-like decoys to reduce cytokine levels. Naturally, one would like to know if this really works, but in the spirit of the mission of QRB Discovery, I believe that this research merits publication at the present stage provided that the underlying data are sufficiently convincing. In this regard, the present version the manuscript is somewhat lacking.
The most central result in this manuscript is the binding assay aimed to prove that the Fc-fusion QTY variants are functional and bind their targets. Thus, it is critical that these experiments are reproducible and provide accurate estimates of the binding affinities. Compared to previous results reported in Fig. 3 of Zhang et al. (2018) PNAS 115, E8652, the MST data in the present paper (Fig. 5) are of much lower quality. Please describe how many replicates were performed to generate the data in Fig. 5 and please add error bars to the individual data points. Describe how you estimated the uncertainties of the Kd values reported in Table 1.
Further, please report whether negative control experiments were performed, and if so, what type of protein was used as control.
Please describe the rationale behind the receptor-Fc fusion design. At present, you merely state that this was done to “form an antibody-like structure”. Are there any immunological considerations behind this choice? Do you expect the Fc region to bind to the corresponding Fc receptor? (What purpose would this serve?)
It would strengthen the manuscript if you could provide calculations to back up the expectations that the receptor-Fc fusion proteins might be used in the clinic to effectively reduce aberrantly high cytokine levels. How high levels of cytokines are present during a “cytokine storm”? Is it feasible to administer sufficiently high levels of the fusion proteins to the patient in order for the treatment to have a significant effect?
Minor points:
p. 4, 3rd paragraph: I cannot see how both of the following 2 statements can be true: “QTY code was only applied to the transmembrane domains” and (two lines down) “Both extracellular domains (colored black) and intracellular linkers (colored yellow) are redesigned according to the QTY code algorithm”. Am I missing something?
Fig. 4: There are extra bands in panels D and F as well as in A and B. Please comment on these.
Fig. 5 is not mentioned in the paragraph that presents these data (p. 5).