Introduction
Attention-deficit/hyperactivity disorder (ADHD) is characterised by persisting, age-inappropriate and impairing symptoms of inattention and/or impulsivity-hyperactivity (American Psychiatric Association, 2013). ADHD is also associated with deficits in executive functions (EF), most prominently in motor and interference inhibition, sustained attention and vigilance, switching, working memory and timing (Coghill, Toplak, Rhodes, & Adamo, Reference Coghill, Toplak, Rhodes, Adamo, Banaschewski, Coghill and Zuddas2018; Rubia, Reference Rubia2018). Neuroimaging studies indicate delayed structural and functional brain maturation (Shaw et al., Reference Shaw, Eckstrand, Sharp, Blumenthal, Lerch, Greenstein and Rapoport2007; Sripada, Kessler, & Angstadt, Reference Sripada, Kessler and Angstadt2014), and consistent underactivation in predominantly right inferior frontal (rIFC), dorsolateral prefrontal (dlPFC) and anterior cingulate cortices, as well as striatal, parietal and cerebellar regions during cognitive control, timing and attention tasks (Hart, Radua, Mataix-Cols, & Rubia, Reference Hart, Radua, Mataix-Cols and Rubia2012, Reference Hart, Radua, Nakao, Mataix-Cols and Rubia2013; Lukito et al., Reference Lukito, Norman, Carlisi, Radua, Hart, Simonoff and Rubia2020; Norman et al., Reference Norman, Carlisi, Lukito, Hart, Mataix-Cols, Radua and Rubia2016; Rubia, Reference Rubia2018).
Psychostimulants are the gold-standard treatment for improving ADHD symptoms, but have possible associated side effects (Cortese, Reference Cortese2020), poor adherence in adolescence (Cortese et al., Reference Cortese, Adamo, Del Giovane, Mohr-Jensen, Hayes, Carucci, Atkinson and Cipriani2018; Cunill, Castells, Tobias, & Capellà, Reference Cunill, Castells, Tobias and Capellà2016) and are not indicated for all individuals with ADHD (Faraone et al., Reference Faraone, Asherson, Banaschewski, Biederman, Buitelaar, Ramos-Quiroga and Franke2015). Evidence of longer-term efficacy is also limited (Cortese et al., Reference Cortese, Adamo, Del Giovane, Mohr-Jensen, Hayes, Carucci, Atkinson and Cipriani2018; Swanson et al., Reference Swanson, Arnold, Jensen, Hinshaw, Hechtman, Pelham and Roy2018), possibly due to brain adaptation (Fusar-Poli, Rubia, Rossi, Sartori, & Balottin, Reference Fusar-Poli, Rubia, Rossi, Sartori and Balottin2012). Meta-analyses indicate small to moderate efficacy of behavioural therapies, cognitive training (CT), neurofeedback or dietary interventions on ADHD symptoms (Catalá-López et al., Reference Catalá-López, Hutton, Núñez-Beltrán, Mayhew, Page, Ridao, Tobías and Moher2017). Non-invasive brain stimulation techniques, however, are promising given their potential to stimulate key dysfunctional brain regions associated with ADHD, with potentially longer-term neuroplastic effects that drugs cannot offer (Cinel, Valeriani, & Poli, Reference Cinel, Valeriani and Poli2019; Rubia, Reference Rubia2018; Sierawska et al., Reference Sierawska, Prehn-Kristensen, Moliadze, Krauel, Nowak, Freitag and Buyx2019; STIPED Press Release, 2017; Westwood, Radua, & Rubia, Reference Westwood, Radua and Rubia2020). Transcranial direct current stimulation (tDCS) is particularly well-suited for paediatric populations as it is user-friendly, well tolerated with minimal side effects (Bikson et al., Reference Bikson, Grossman, Thomas, Zannou, Jiang, Adnan and Woods2016), and is cheaper relative to other techniques, such as transcranial magnetic stimulation (Gilbert, Reference Gilbert, Oberman and Enticott2019).
In tDCS, a weak direct electrical current is delivered through two electrodes placed on the scalp (one anode, one cathode), generating subthreshold, polarity-dependent shifts in resting membrane potentials in underlying brain regions. The resulting net increase (predominantly under the anode) or decrease (predominantly under the cathode) in neuronal excitability leads to modulation of the neuronal network (Liu et al., Reference Liu, Vöröslakos, Kronberg, Henin, Krause, Huang and Buzsáki2018), with neurophysiological effects persisting after stimulation, presumably by potentiating mediators of practice-dependent synaptic plasticity, including GABA, glutamate (Filmer, Ehrhardt, Bollmann, Mattingley, & Dux, Reference Filmer, Ehrhardt, Bollmann, Mattingley and Dux2019; Stagg & Nitsche, Reference Stagg and Nitsche2011), dopamine and noradrenaline (Fonteneau et al., Reference Fonteneau, Redoute, Haesebaert, Le Bars, Costes, Suaud-Chagny and Brunelin2018; Kuo, Paulus, & Nitsche, Reference Kuo, Paulus and Nitsche2008; Monte-Silva, Liebetanz, Grundey, Paulus, & Nitsche, Reference Monte-Silva, Liebetanz, Grundey, Paulus and Nitsche2010).
Evidence of cognitive performance and clinical improvement following tDCS is, however, limited. Two meta-analyses of tDCS studies stimulating mainly the left dlPFC in 1–5 sessions in children and adolescents with ADHD indicate a modest improvement in inhibitory control measures (Salehinejad, Wischnewski, Nejati, Vicario, & Nitsche, Reference Salehinejad, Wischnewski, Nejati, Vicario and Nitsche2019) with the latter one showing non-significant improvement on processing speed and inhibitory measures with no effects on attention measures (Westwood et al., Reference Westwood, Radua and Rubia2020). Only two sham-controlled studies tested ADHD symptoms, reporting improvement in inattentive symptoms, but not impulsivity/hyperactivity, immediately, 1 (Cachoeira et al., Reference Cachoeira, Leffa, Mittelstadt, Mendes, Brunoni, Pinto and Schestatsky2017; Soff, Sotnikova, Christiansen, Becker, & Siniatchkin, Reference Soff, Sotnikova, Christiansen, Becker and Siniatchkin2017) and 2 weeks (Cachoeira et al., Reference Cachoeira, Leffa, Mittelstadt, Mendes, Brunoni, Pinto and Schestatsky2017) after anodal tDCS over left or right dlPFC.
There is evidence that tDCS effects can be enhanced when combined with CT by functionally priming the brain regions that mediate the cognitive function being trained (Au et al., Reference Au, Katz, Buschkuehl, Bunarjo, Senger, Zabel and Jonides2016, Reference Au, Karsten, Buschkuehl and Jaeggi2017; Katz et al., Reference Katz, Au, Buschkuehl, Abagis, Zabel, Jaeggi and Jonides2017; Kronberg, Bridi, Abel, Bikson, & Parra, Reference Kronberg, Bridi, Abel, Bikson and Parra2017). Multi-session anodal tDCS combined with CT has also been shown to elicit longer-term cognitive improvements in healthy controls for up to 1 (Jones, Stephens, Alam, Bikson, & Berryhill, Reference Jones, Stephens, Alam, Bikson and Berryhill2015; Stephens & Berryhill, Reference Stephens and Berryhill2016), nine (Ruf, Fallgatter, & Plewnia, Reference Ruf, Fallgatter and Plewnia2017) or 12 months (Katz et al., Reference Katz, Au, Buschkuehl, Abagis, Zabel, Jaeggi and Jonides2017), and clinical improvements in psychiatric disorders for at least 1 month (Kekic, Boysen, Campbell, & Schmidt, Reference Kekic, Boysen, Campbell and Schmidt2016; Moffa, Brunoni, Nikolin, & Loo, Reference Moffa, Brunoni, Nikolin and Loo2018; Tortella et al., Reference Tortella, Casati, Aparicio, Mantovani, Senço, D'Urso and Brunoni2015), suggesting longer-term neuroplastic effects.
Most studies in ADHD have targeted the left dlPFC. However, one of the most consistent findings of meta-analyses of functional magnetic resonance imaging (fMRI) studies is the underactivation of rIFC during tasks of cognitive control (Hart, Radua, Nakao, Mataix-Cols, & Rubia, Reference Hart, Radua, Nakao, Mataix-Cols and Rubia2013; Lukito et al., Reference Lukito, Norman, Carlisi, Radua, Hart, Simonoff and Rubia2020; McCarthy, Skokauskas, & Frodl, Reference McCarthy, Skokauskas and Frodl2014; Norman et al., Reference Norman, Carlisi, Lukito, Hart, Mataix-Cols, Radua and Rubia2016; Rubia, Reference Rubia2018). The rIFC is a cognitive control ‘hub’ region, playing a key role in cognitive control (Aron, Robbins, & Poldrack, Reference Aron, Robbins and Poldrack2014; Langner, Leiberg, Hoffstaedter, & Eickhoff, Reference Langner, Leiberg, Hoffstaedter and Eickhoff2018), sustained attention (Kim, Reference Kim2014; Langner & Eickhoff, Reference Langner and Eickhoff2013; Petersen & Posner, Reference Petersen and Posner2012) and timing networks (Radua, del Pozo, Gómez, Guillen-Grima, & Ortuño, Reference Radua, del Pozo, Gómez, Guillen-Grima and Ortuño2014), mediating key functions of impairment in ADHD (Coghill et al., Reference Coghill, Toplak, Rhodes, Adamo, Banaschewski, Coghill and Zuddas2018; Noreika, Falter, & Rubia, Reference Noreika, Falter and Rubia2013). The rIFC dysfunction has also been shown to be disorder-specific to ADHD compared to several neurodevelopmental disorders in comparative fMRI meta-analyses of cognitive control (Lukito et al., Reference Lukito, Norman, Carlisi, Radua, Hart, Simonoff and Rubia2020; Norman et al., Reference Norman, Carlisi, Lukito, Hart, Mataix-Cols, Radua and Rubia2016; Rubia, Reference Rubia2018). Further, its upregulation is the most consistent effect of single-dose and longer-term psychostimulant medication (Norman et al., Reference Norman, Carlisi, Lukito, Hart, Mataix-Cols, Radua and Rubia2016; Rubia et al., Reference Rubia, Alegria, Cubillo, Smith, Brammer and Radua2014).
Only three published studies applied tDCS over rIFC in ADHD, in one or five sessions. One found improvements after anodal relative to sham tDCS in Flanker task errors and intrasubject response variability in 21 adolescents with ADHD (Breitling et al., Reference Breitling, Zaehle, Dannhauer, Bonath, Tegelbeckers, Flechtner and Krauel2016), but no effect in 14 children with ADHD on a combined n-back and Stop task with high definition or conventional anodal tDCS (Breitling et al., Reference Breitling, Zaehle, Dannhauer, Tegelbeckers, Flechtner and Krauel2020). In 20 undiagnosed high-school students with ADHD symptoms, anodal compared to sham tDCS improved Go accuracy in a Go/No-Go (GNG) task but no other inhibitory or Stroop task measures (Soltaninejad, Nejati, & Ekhtiari, Reference Soltaninejad, Nejati and Ekhtiari2015b). To our knowledge, no sham-controlled study so far has measured clinical and cognitive effects of multi-session anodal tDCS over rIFC in combination with CT in patients with ADHD
In this double-blind, sham-controlled, randomised controlled trial (RCT), 50 children and adolescents with ADHD were administered 15 consecutive weekday sessions of anodal or sham tDCS over rIFC combined with CT of EF typically impaired in ADHD. The primary outcome measures were parent-rated clinical symptoms and cognitive performance in an inhibition and a sustained-attention task. Secondary outcome measures were other clinical, safety and EF measures.
Given some evidence of clinical and cognitive improvements with anodal tDCS over the dlPFC (Cachoeira et al., Reference Cachoeira, Leffa, Mittelstadt, Mendes, Brunoni, Pinto and Schestatsky2017; Soff et al., Reference Soff, Sotnikova, Christiansen, Becker and Siniatchkin2017) or rIFC (Breitling et al., Reference Breitling, Zaehle, Dannhauer, Bonath, Tegelbeckers, Flechtner and Krauel2016; Soltaninejad, Nejati, & Ekhtiari, Reference Soltaninejad, Nejati and Ekhtiari2015a) and prolonged effects when tDCS is paired with CT, we hypothesised that, compared to sham, at posttreatment, multi-session anodal tDCS of rIFC with multi-EF training would show greater improvement in ADHD symptoms and/or performance on EF tasks mediated by rIFC and targeted by the CT. We also hypothesised that clinical and EF task improvement would persist 6 months after posttreatment. Finally, we hypothesised no side or adverse effects.
Materials and methods
Trial design
This pre-registered (ISRCTN48265228), double-blind, sham-controlled, parallel RCT compared multi-session anodal v. sham tDCS over the rIFC combined with multi-EF training. Randomisation to stimulation group was stratified by age (10–14.5 and 14.5–18 years) and medication status (naïve and non-naïve) using Smith randomisation (Kaiser, Reference Kaiser2012; Smith, Reference Smith1984) conducted by Innosphere Ltd (Haifa, Israel). Experimenters, participants and parents/caregivers were blind to stimulation group. This trial received local research ethics committee approval (RECID: 17/LO/0983) and was conducted in accordance with the Declaration of Helsinki and Consolidated Standards of Reporting Trials (CONSORT) guidelines (Moher et al., Reference Moher, Hopewell, Schulz, Montori, Gotzsche, Devereaux and Altman2010).
Participants
Fifty male participants (10–18 years) were recruited from South London clinics, social media and parent support groups (February 2018–20). All participants had a clinical DSM-5 diagnosis of ADHD established by a clinician, and validated by the Schedule of Affective Disorders and Schizophrenia for School-Age Children-Present and Lifetime version (K-SADS-PL; DSM-IV) (Kaufman et al., Reference Kaufman, Birmaher, Brent, Rao, Flynn, Moreci and Ryan1997) and the Conners 3rd Edition–Parent (Conners 3-P, cut-off t score > 60) (Conners, Pitkanen, & Rzepa, Reference Conners, Pitkanen, Rzepa, Kreutzer, DeLuca and Caplan2011). Autism spectrum disorder (ASD) was excluded using a combination (both required) of both the parent-rated Social Communication Questionnaire (SCQ, cut-off > 17) (Eaves, Wingert, Ho, & Mickelson, Reference Eaves, Wingert, Ho and Mickelson2006) and the pro-social scale of the Strengths and Difficulties Questionnaire (SDQ, cut-off < 5) (Goodman, Reference Goodman1997); for participants falling outside these criteria, the absence of ASD was confirmed by their clinician. Further exclusion criteria were IQ below 80 (Wechsler Abbreviated Scale of Intelligence, WASI-II) (Wechsler, Reference Wechsler2011), history of alcohol or substance abuse, neurological illness, comorbid major psychiatric disorders as established by the K-SADS-PL or clinical diagnoses [except for conduct disorder/oppositional defiant disorder (ODD)], contraindications to tDCS [e.g. metallic implants (except dental braces), previous neurosurgical procedures, history of migraine, diseased/damaged skin on the scalp], treated with drugs that lower seizure thresholds (e.g. antipsychotics, tricyclic antidepressants), and an inability to provide consent from the legal caregiver for children under 16 years or from participants over 16 years (Fig. 1). Participants received £540 plus travel expenses for participating.

Fig. 1. CONSORT flow diagram (Moher et al., Reference Moher, Hopewell, Schulz, Montori, Gotzsche, Devereaux and Altman2010) of this RCT from enrolment, intervention allocation, follow-up and analysis.
Baseline assessment was scheduled at least 2 weeks after medication titration. Thirty-two participants received stable ADHD medication (non-psychostimulants: 2; psychostimulants: 30; between 21 weeks and 10 years). To help avoid medication effects from masking tDCS effects, 14 of the 32 participants followed our optional request to abstain from psychostimulants at least 24 h before each assessment time point (range: 24–72 h), of which eight abstained only 24 h beforehand (sham, 4; anodal tDCS, 4). A further seven participants abstained for trial duration (i.e. baseline to posttreatment). One participant stopped taking medication 3 months before follow-up.
Intervention
Over 15 consecutive weekdays, participants played four 5 min CT games during 20 min of anodal or sham tDCS. CT was composed of four ACTIVATETM (Wexler et al., Reference Wexler, Iseli, Leon, Zaggle, Rush, Goodman and Bo2016) games (Monday, Wednesday and Friday) or a Stop task (Rubia, Smith, & Taylor, Reference Rubia, Smith and Taylor2007) followed by three ACTIVATETM games (Tuesday and Thursday). ACTIVATETM games were played on an iPad Air 2 10.2″, the Stop task on a Dell XPS 15.6″ Touch Laptop (Fig. 2).

Fig. 2. Schematic overview of the study design. ADHD-RS, Attention Deficit Hyperactivity Disorder-Rating Scale; ARI, Affective Reactivity Index; CIS, Columbia Impairment Scale; Conners 3-P, Conners' 3rd Edition Parent Rating; Cognitive battery, Maudsley Attention and Response Suppression task battery, vigilance, Wisconsin Card Sorting Task, visual-spatial working memory, verbal fluency; K-SADS-PL, Kiddie-SADS-Present and Lifetime Version; MEWS, Mind Excessively Wandering Scale; SCQ, Social Communication Questionnaire (Lifetime), SDQ, Social Difficulties Questionnaire (prosocial scale only); WASI-II, Wechsler Abbreviated Scale of Intelligence, 2nd Edition; WREMB-R, Weekly Rating of Evening and Morning Behavior-Revised.
CT games
ACTIVATETM trains multiple EF with engaging video games that increase in complexity as participants improve. Five of the six games were selected to target ADHD-relevant EF, including selective and multiple simultaneous attention, inhibition and cognitive flexibility (i.e. Magic Lens, Peter's Printer Panic, Treasure Trunk) and visual-spatial working memory (i.e. Grub Ahoy, Monkey Trouble). Before each game, participants chose a game to play from three options; the ACTIVATETM programme tracked choices and constrained future options so that multiple EF were twice as likely to be trained as working memory (Wexler et al., Reference Wexler, Iseli, Leon, Zaggle, Rush, Goodman and Bo2016).
The training tracking Stop task trained motor response inhibition by requiring participants to cancel a prepotent motor response to a go signal that is followed shortly by an unexpected and rare (30% of trials) stop signal (Rubia et al., Reference Rubia, Smith and Taylor2007). The delay between go and stop signals is dynamically adjusted to the participant performance, with better inhibition resulting in longer stop signal delays thus increasing the difficulty to withhold a response. Participants were encouraged to wait for the stop signal before responding to the go signal, thereby training inhibition and waiting behaviour/response delay (see online Supplementary material).
tDCS
Direct current was delivered by NovoStim (InnoSphere, Haifa, Israel) via a pair of 25 cm2 brush electrodesFootnote †Footnote 1 dipped in saline solution (0.45 mol). Using the EEG 10–20, the anode was placed over rIFC (F8) (Okamoto et al., Reference Okamoto, Dan, Sakamoto, Takeo, Shimizu, Kohno and Dan2004; Okamoto & Dan, Reference Okamoto and Dan2005), the cathode over the contralateral supraorbital area (Fp1), and held in place by a 10–20 EEG cap. For anodal tDCS, 1 mA was administered for 20 min (current density: 0.04 cm2; total charge: 0.8 C/cm2) with a 30 s fade-in/fade-out. For sham stimulation, only the 30 s fade-in/fade-out was administered (Palm et al., Reference Palm, Reisinger, Keeser, Kuo, Pogarell, Leicht and Padberg2013). After each week, participants, parents and tDCS administrators were asked to guess if participants had received anodal or sham tDCS.
Primary outcomes
Cognitive
The adult version of the Maudsley Attention and Response Suppression (MARS) task battery (Penadés et al., Reference Penadés, Catalán, Rubia, Andrés, Salamero and Gastó2007; Rubia et al., Reference Rubia, Smith and Taylor2007) measured motor response inhibition [GNG; dependent variable (DV): probability of inhibition (PI)] and sustained attention [Continuous Performance Task (CPT); DV: omission and commission errors] (see online Supplementary material).
ADHD symptoms
Parent-rated ADHD Rating Scale-IV (ADHD-RS) Home Version (DV: Total Scores) (DuPaul, Power, Anastopoulos, & Reid, Reference DuPaul, Power, Anastopoulos and Reid1998), a standard measure of treatment effects in DSM-IV ADHD symptoms.
Secondary outcomes
Cognitive measures
MARS tasks (Penadés et al., Reference Penadés, Catalán, Rubia, Andrés, Salamero and Gastó2007; Rubia et al., Reference Rubia, Smith and Taylor2007) measured interference inhibition (Simon Task; DV: Simon reaction time effect) or time discrimination (Time Discrimination Task; DV: percentage correct). The Mackworth Clock Task (Lichstein, Riedel, & Richman, Reference Lichstein, Riedel and Richman2000; Mackworth, Reference Mackworth1948; PsyTookit, 2017) measured vigilance (DV: percentage omissions and commission errors), the Wisconsin Card Sorting Task (PsyToolkit, 2018) (WCST; DV: total and preservative errors) measured cognitive flexibility, and the C8 Sciences version of the NIH List Sorting Working Memory (WM) Task (DV: total score) (Tulsky et al., Reference Tulsky, Carlozzi, Chiaravalloti, Beaumont, Kisala and Gershon2014) measured visuo-spatial working memory. Phonetic and semantic fluency (DV: percentage of correct responses) (Troyer, Reference Troyer2000) were measured to account for potential downregulation of lIFC mediated language production functions via interhemispheric inhibition.
Generic EF
Three intercorrelated generic, task-independent EF were measured, which included: (1) general speed of information processing [i.e. mean reaction times (MRT) for Go (GNG), Congruent (Simon) and Target (CPT) trials, weighted by number of trials] (2); intrasubject response variability [i.e. Coefficient of Variance (CV; s.d. of MRT divided by MRT) for Go (GNG), Congruent (Simon) and Target (CPT) trials]; and (3) prematurity (i.e. premature responses to all trials in GNG, Simon and CPT) (online Supplementary Tables S1–S5 and Figs S1–S3).
ADHD symptoms and related impairments
Caregiver-rated Conners 3-P ADHD Index (Conners et al., Reference Conners, Pitkanen, Rzepa, Kreutzer, DeLuca and Caplan2011) measured ADHD symptoms, Weekly Parent Ratings of Evening and Morning Behaviour-Revised scale (WREMB-R) (Wehmeier, Dittmann, Schacht, Helsberg, & Lehmkuhl, Reference Wehmeier, Dittmann, Schacht, Helsberg and Lehmkuhl2009) and the Columbia Impairment Scale-Parent version (CIS) (Bird et al., Reference Bird, Shaffer, Fisher and Gould1993) measured ADHD-related difficulties and functional impairments, the child- and caregiver-rated Affective Reactivity Index (ARI) measured irritability (Stringaris et al., Reference Stringaris, Goodman, Ferdinando, Razdan, Muhrer, Leibenluft and Brotman2012), and the child-rated Mind Excessively Wandering Scale (MEWS) measured mind-wandering (Mowlem et al., Reference Mowlem, Skirrow, Reid, Maltezos, Nijjar, Merwood and Asherson2019).
Safety, feasibility and tolerability measures
Safety was measured with parent-rated questionnaires on side effects (Hill & Taylor, Reference Hill and Taylor2001) and adverse events (Döpfner, Lehmkuhl, & Steinhausen, Reference Döpfner, Lehmkuhl and Steinhausen2006). Participants rated their mood and wakefulness via a visual analogue scale (Alegria et al., Reference Alegria, Wulff, Brinson, Barker, Norman, Brandeis and Rubia2017; Maurizio et al., Reference Maurizio, Liechti, Heinrich, Jaencke, Steinhausen, Walitza and Drechsler2014) and tDCS tolerability (Antal et al., Reference Antal, Alekseichuk, Bikson, Brockmöller, Brunoni, Chen and Paulus2017). Parents and participants rated the overall impression of tDCS and CT using a rating scale from 0 (never) to 4 (always) designed for this study.
EEG
EEG was measured at rest and during the GNG task at each assessment timepoint but will be reported in a separate publication.
Training outcome measures
The ACTIVATETM DV was the highest game level reached each week averaged across three games that loaded on multiple EF (i.e. Treasure Trunk, Magic Lens, Printer Panic). The Stop task DV was PI on average for each week.
Assessment time points
Primary and secondary outcome measures were collected at baseline, posttreatment and 6-month follow-up. Adverse events and overall impression of tDCS and CT were measured at posttreatment. Ratings of mood, wakefulness and the tolerability of tDCS were measured in each stimulation session. Baseline and posttreatment sessions were scheduled up to 3 weeks before the first and after the last stimulation session, respectively.
Statistical methods
Confirmatory analysis
Repeated-measures analysis of covariance (ANCOVA) tested group differences across posttreatment and follow-up, covarying for age in months, medication status (naïve, on- or off-medication) and baseline value of outcomes where applicable. Sensitivity analyses removed statistical outliers on cognitive tasks, participants who changed medication between posttreatment and follow-up, or participants whose treatment spanned over 4 not 3 weeks (online Supplementary Tables S6–S8). The α level was 0.05, and corrected for multiple testing with False Discovery Rate (FDR) with Benjamini–Hochberg adjustment (Benjamini & Hochberg, Reference Benjamini and Hochberg1995). Only results with adjusted p values are reported (for unadjusted, see Tables 2–3). Analyses were conducted with IBM SPSS Statistics 26 (IBM Corp., Armonk, NY, USA).
Medication status
Medication status was coded as a categorical covariate with three groups: medication-naïve, on-medication and off-medication. Participants who abstained for the treatment trial period were coded as off-medication, participants who abstained at assessment time points only were coded as off-medication for cognitive outcomes and on-medication for clinical outcomes (which can cover 3 weeks).
Missing data and statistical outliers
In primary and secondary outcomes, only posttreatment WM task data for one participant were missing. Missing stop signal task data (1.33%) were random and replaced by group averages.
Power analysis
An a priori power analysis conducted prior to trial registration indicated that a minimum of 40 participants would be adequate to detect a meaningful effect on clinical and cognitive measures (Ditye, Jacobson, Walsh, & Lavidor, Reference Ditye, Jacobson, Walsh and Lavidor2012; Jacobson, Ezra, Berger, & Lavidor, Reference Jacobson, Ezra, Berger and Lavidor2012).
Results
Baseline demographics
Compared to sham tDCS, the anodal tDCS group was significantly younger; had fewer years in education; higher ADHD-RS Total Scores and ODD symptoms; worse performance on the Macworth Clock, Time Discrimination and list sort working memory tasks; and spent more time playing Peter's Printer Panic (Table 1).
Table 1. Baseline demographic, clinical, cognitive measures and medication status; the number of tDCS and CT sessions; and the time spent playing each CT game in the sham and anodal tDCS groups

ADHD-RS, Caregiver-rated ADHD Rating Scale; ARI, Affective Reactivity Index; CIS, Columbia Impairment Scale-Parent; MEWS, Mind Excessively Wandering Scale; s.d., standard deviation; SCQ, Social Communication Questionnaire; SDQ, Social Difficulties Questionnaire; WASI-II, Wechsler Abbreviated Scale of Intelligence; WREMB-R, Weekly Parent Ratings of Evening and Morning Behaviour-Revised. Significance group differences are highlighted in bold.
a One participant could not attend three stimulation sessions due to extreme weather conditions.
Primary outcome measures
Cognitive
There were no significant effects on primary cognitive outcome measures after adjusting for multiple testing (Table 2; online Supplementary Fig. S4).
Table 2. Summary of adjusted average performance on primary cognitive and clinical outcome measures after sham and anodal tDCS combined with CT
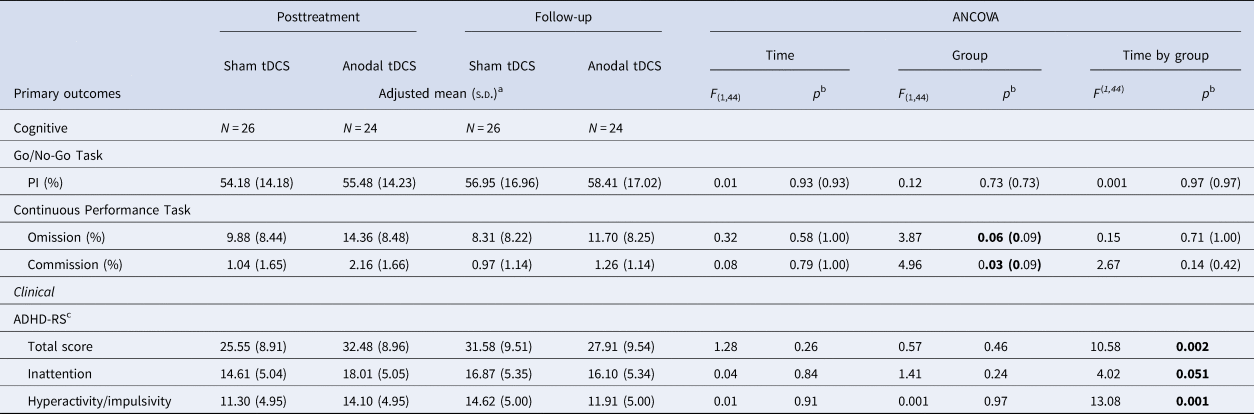
ADHD-RS, ADHD Rating Scale; PI, probability of inhibition; s.d., standard deviation.
Benjamini–Hochberg adjusted p values given in parentheses.
a Adjusted values as predicted by the repeated-measures ANCOVA testing group differences at posttreatment and follow-up, adjusting for baseline, age at entry and medication status (naïve, off-medication, on-medication).
b Benjamini–Hochberg adjustment was applied to p values for time, group and time by group interaction effect separately and was applied separately to primary cognitive, secondary cognitive and secondary clinical outcome measures separately.
c Benjamini–Hochberg adjustment was not applied to these measures.
ADHD symptoms
There was only a significant time by group interaction (F 1,44 = 10.58, p = 0.002, ηp2 = 0.19); simple effects analysis showed that the anodal v. sham tDCS group had higher scores at posttreatment but not at follow-up [posttreatment: p = 0.011 (95% CI 1.65–12.21); follow-up: p = 0.20 (95% CI −9.30 to 1.97)]. To determine what drove this effect, exploratory simple effects analysis of subscales showed that the anodal v. sham tDCS group had higher scores at posttreatment on Inattention (p = 0.03) and Hyperactivity-Impulsivity subscales (p = 0.06), with the latter being lower for active v. sham tDCS at follow-up (p = 0.07) (Table 2; online Supplementary Fig. S5).
Secondary outcomes measures
Cognitive
The were no significant effects on secondary cognitive outcome measures after adjusting for multiple testing (Table 3).
Table 3. Summary of adjusted average performance on secondary cognitive and clinical outcome measures after sham and anodal tDCS combined with CT

ARI, Affective Reactivity Index; CIS, Columbia Impairment Scale-Parent; Corr, Correct; ICV, intrasubject coefficient of variance; MEWS, Mind Excessively Wandering Scale; MRT, mean reaction times; Resp, responses; s.d., standard deviation; WREMB-R, Weekly Parent Ratings of Evening and Morning Behaviour-Revised.
Benjamini–Hochberg adjusted p values given in parentheses.
a Adjusted values as predicted by the repeated-measures ANCOVA testing group differences at posttreatment and follow-up, adjusting for baseline, age at entry and medication status (naïve, off-medication, on-medication).
b Benjamini–Hochberg adjustment was applied to p values for time, group and time by group interaction effects separately and was applied separately to primary cognitive, secondary cognitive and secondary clinical outcome measures separately.
c Benjamini–Hochberg adjustment was not applied to these measures.
ADHD symptoms and related impairments
There was only a significant time by group interaction for Conners 3-P ADHD Index (F 1,44 = 13.726, p = 0.004, ηp2 = 0.238). Simple effects analysis showed significantly higher scores following anodal v. sham tDCS at posttreatment only [p = 0.001 (95% CI 1.92 to −7.11); follow-up: p = 0.73 (95% CI −3.39 to 2.41)] (Table 3).
Exploratory analyses
Separate repeated-measures ANOVAs showed an effect of time (baseline v. posttreatment or follow-up) across groups. In cognitive measures, both groups improved in GNG, Simon, Letter Fluency tasks (baseline v. posttreatment or follow-up); Speed of Processing (baseline v. posttreatment only); and CPT % Omissions and Commissions, Mackworth Clock % Omissions, WM, Response Variability, and Prematurity (baseline v. follow-up only) (online Supplementary Table S9). In clinical measures, both groups improved in ADHD-RS and Conners 3-P Index (baseline v. posttreatment or follow-up); and in ARI Parent and Child, WREMB-R, and CIS (baseline v. posttreatment only).
Group differences in CT performance across the 3 weeks were explored with repeated-measures ANCOVAs covarying for baseline, age, medication status and total time spent playing each game. There were no significant effects after adjusting for multiple testing (online Supplementary Table S10).
We also explored if outcome changes that showed a significant time effect across both groups from baseline to posttreatment or follow-up were correlated with changes in CT performance scores (week 3 minus week 1). No correlations were significant (online Supplementary Table S11).
Given that age was not matched between groups, we conducted a post-hoc moderation analysis (Hayes, Reference Hayes2013), predicting a change in ADHD-RS (baseline minus posttreatment) in a regression analysis of stimulation group, age and a stimulation by age interaction. While the stimulation by age interaction was not significant [β = 0.2, s.e. = 0.12, t(46) = 1.7, p = 0.096], the simple effects showed a significantly reduced improvement for anodal tDCS v. sham in older participants [1s.d. above mean age; β = 9.53, s.e. = 4.26, t(46) = 2.24, p = 0.03], and an opposite but not significant pattern in younger children [1s.d. below mean age; β = −0.6, s.e. = 4.11, t(46) = −0.15, p = 0.88], indicating that older participants benefitted clinically less from anodal v. sham tDCS.
Safety, feasibility, tolerability and blinding integrity.
There were no significant group differences in ratings of mood, wakefulness, overall impression of tDCS and CT (online Supplementary Tables S12 and S13), and side effects (Table 3). Adverse effects were significantly higher after anodal v. sham tDCS at posttreatment (F 1,44 = 4.09, p = 0.05, ηp2 = 0.08), driven mainly by higher parent-ratings for ‘he seems more grumpy and irritable’, ‘has little appetite’ and ‘has more problems falling asleep’ (Table 3). Stimulation was well tolerated, with only significantly higher reports of burning sensation during anodal than sham tDCS (online Supplementary Table S14). Group assignment guesses were not above chance for experimenters [χ2(1) = 3.9, p = 0.28], participants [χ2(1) = 1.85, p = 0.17] and borderline for parent guesses for anodal tDCS [χ2(1) = 3.57, p = 0.06], thus blinding was overall successful.
Discussion
This double-blind, sham-controlled RCT administered 15 sessions of anodal or sham tDCS over rIFC combined with CT in 50 boys with ADHD, and found no improvement in ADHD symptoms or cognitive performance. Although both groups improved in clinical and cognitive measures over time, anodal relative to sham tDCS was associated with higher primary (ADHD-RS) and secondary (Conners 3-P ADHD Index) clinical outcome measures. Side effects did not differ, but at posttreatment, adverse effects relating to mood, sleep and appetite were higher following anodal than sham tDCS.
The lack of an observable clinical or cognitive effect extend previous meta-analytic evidence of no significant cognitive effects and limited evidence of clinical effects in ADHD with 1–5 anodal tDCS sessions over predominantly left dlPFC (Westwood et al., Reference Westwood, Radua and Rubia2020). These findings are unexpected given that rIFC underactivation is consistently associated with poor cognitive control, attention and clinical symptoms in ADHD (Hart et al., Reference Hart, Radua, Nakao, Mataix-Cols and Rubia2013; Lukito et al., Reference Lukito, Norman, Carlisi, Radua, Hart, Simonoff and Rubia2020; Norman et al., Reference Norman, Carlisi, Lukito, Hart, Mataix-Cols, Radua and Rubia2016; Rubia, Reference Rubia2018). While the findings of no clinical effect of tDCS of rIFC are novel, these extend evidence of no or moderate effects from prior 1–5 session sham-controlled tDCS studies stimulating rIFC in ADHD (see introduction) (Breitling et al., Reference Breitling, Zaehle, Dannhauer, Bonath, Tegelbeckers, Flechtner and Krauel2016, Reference Breitling, Zaehle, Dannhauer, Tegelbeckers, Flechtner and Krauel2020; Soltaninejad et al., Reference Soltaninejad, Nejati and Ekhtiari2015a).
Findings are furthermore unexpected given evidence of a synergistic effect of combined CT and tDCS on improving cognition (Allenby et al., Reference Allenby, Falcone, Bernardo, Wileyto, Rostain, Ramsay and Loughead2018; Au et al., Reference Au, Katz, Buschkuehl, Bunarjo, Senger, Zabel and Jonides2016; Katz et al., Reference Katz, Au, Buschkuehl, Abagis, Zabel, Jaeggi and Jonides2017). Although we covaried for age, one possible explanation for the negative findings on clinical symptoms and cognition is that the anodal tDCS group were significantly younger with larger baseline clinical and cognitive impairments compared to sham, both of which could have impaired learning (Loe & Feldman, Reference Loe and Feldman2007). This is supported by evidence that ADHD children with worse neurocognitive skills at baseline show less CT gains (Minder, Zuberer, Brandeis, & Drechsler, Reference Minder, Zuberer, Brandeis and Drechsler2019) and neurofeedback learning (Hammer et al., Reference Hammer, Halder, Blankertz, Sannelli, Dickhaus, Kleih and Kübler2012; Lam et al., Reference Lam, Criaud, Alegria, Barker, Giampietro and Rubia2020; Zilverstand et al., Reference Zilverstand, Sorger, Slaats-Willemse, Kan, Goebel and Buitelaar2017), while healthy controls with poorer cognitive performance or lower education achievement at baseline can nullify or lead to detrimental tDCS effects (Hsu, Juan, & Tseng, Reference Hsu, Juan and Tseng2016; Jones & Berryhill, Reference Jones and Berryhill2012).
Alternatively, given the stronger electric field strengths in children v. adults (Minhas, Bikson, Woods, Rosen, & Kessler, Reference Minhas, Bikson, Woods, Rosen and Kessler2012; Salehinejad et al., Reference Salehinejad, Nejati, Mosayebi-Samani, Mohammadi, Wischnewski, Kuo and Nitsche2020), multiple tDCS sessions may have triggered a homeostatic plasticity response – i.e. the amount and direction of plasticity was attenuated in response to excessive increases in neuronal excitability – thereby temporarily disrupting the excitability of rIFC (Fricke et al., Reference Fricke, Seeber, Thirugnanasambandam, Paulus, Nitsche and Rothwell2011; Hoy & Fitzgerald, Reference Hoy and Fitzgerald2015; Karabanov et al., Reference Karabanov, Ziemann, Hamada, George, Quartarone, Classen and Siebner2015; Wefelmeyer & Burrone, Reference Wefelmeyer and Burrone2015). This is in line with our post-hoc moderation analysis that revealed older but not younger participants improved less in the ADHD-RS Total Scores in the anodal v. sham tDCS group at posttreatment. Future studies should verify if tDCS has differential effects depending on current strength and age of participants with ADHD.
Another possibility is that rIFC stimulation downregulated neighbouring dorsal prefrontal or parietal regions part of the dorsal attention network (Cubillo, Halari, Smith, Taylor, & Rubia, Reference Cubillo, Halari, Smith, Taylor and Rubia2012; Hart et al., Reference Hart, Radua, Nakao, Mataix-Cols and Rubia2013), or left hemispheric prefrontal regions that mediate positive emotions (Gainotti, Reference Gainotti2019; Groenewold, Opmeer, de Jonge, Aleman, & Costafreda, Reference Groenewold, Opmeer, de Jonge, Aleman and Costafreda2013).
Interestingly, however, impulsiveness/hyperactivity symptoms, which are most closely associated with rIFC activation (Rubia, Reference Rubia2018; Rubia, Smith, Brammer, Toone, & Taylor, Reference Rubia, Smith, Brammer, Toone and Taylor2005), were lower at follow-up after anodal relative to sham tDCS. This finding – that needs replication – could suggest longer-term neuroplastic consolidation effects as have been shown in other neurotherapies, such as neurofeedback (Alegria et al., Reference Alegria, Wulff, Brinson, Barker, Norman, Brandeis and Rubia2017; Enriquez-Geppert, Smit, Pimenta, & Arns, Reference Enriquez-Geppert, Smit, Pimenta and Arns2019).
Both groups improved in symptoms and cognitive performance from baseline to posttreatment or follow-up, which could suggest gains due to CT (Wexler et al., Reference Wexler, Iseli, Leon, Zaggle, Rush, Goodman and Bo2016); however, given the lack of correlation with CT performance, placebo effects cannot be ruled out.
The negative findings from this trial are crucial given that tDCS is being increasingly incorporated into clinical practice, is considered an acceptable alternative to medication by parents, and is already commercially available (Buchanan, D'Angiulli, Samson, Maisonneuve, & Robaey, Reference Buchanan, D'Angiulli, Samson, Maisonneuve and Robaey2020; Sierawska et al., Reference Sierawska, Prehn-Kristensen, Moliadze, Krauel, Nowak, Freitag and Buyx2019). Particularly alarming is that parent-rated ADHD symptoms and adverse effects were higher at posttreatment after anodal tDCS relative to sham.
Findings are not encouraging for the efficacy of multi-session tDCS of rIFC combined with CT in ADHD. However, there are limitations. Although our sample of 50 participants is the largest sample of any tDCS study in paediatric ADHD, recent meta-analyses report relatively small cognitive effects of tDCS in ADHD (Westwood et al., Reference Westwood, Radua and Rubia2020), suggesting that larger samples may be required to detect tDCS effects. Unfortunately, despite randomisation, groups were not balanced in several baseline measures which could have confounded the tDCS effects. However, measures that differed (i.e. clinical scores, performance, age and medication status) were covaried, minimising any confounding effects. Also, the anodal group had higher ODD symptoms at baseline, which could have affected emotional lability and confounded tDCS effects. However, no group differences were observed in changes of emotional dysregulation as measured on the ARI or in mood ratings. The findings in this study are limited to male adolescents with ADHD and the adopted protocol, and cannot be generalised to other populations (e.g. females; adults) or other protocols. Although we applied the largest number of sessions with the view of boosting consolidation of tDCS effects, the optimal number of sessions is not known in ADHD, leaving the possibility that fewer or even more sessions would optimise the tDCS effect (Au et al., Reference Au, Katz, Buschkuehl, Bunarjo, Senger, Zabel and Jonides2016; Katz et al., Reference Katz, Au, Buschkuehl, Abagis, Zabel, Jaeggi and Jonides2017). Computational current flow models suggest higher stimulation intensities might be required to modulate clinical symptoms and cognitive functions mediated by rIFC given this is a deeper region compared to the commonly stimulated dlPFC (Salehinejad et al., Reference Salehinejad, Nejati, Mosayebi-Samani, Mohammadi, Wischnewski, Kuo and Nitsche2020). Further, this study stimulated ‘F8’ in line with other studies (Breitling et al., Reference Breitling, Zaehle, Dannhauer, Bonath, Tegelbeckers, Flechtner and Krauel2016, Reference Breitling, Zaehle, Dannhauer, Tegelbeckers, Flechtner and Krauel2020; Campanella et al., Reference Campanella, Schroder, Monnart, Vanderhasselt, Duprat, Rabijns and Baeken2016, Reference Campanella, Schroder, Vanderhasselt, Baeken, Kornreich, Verbanck and Burle2018; Dambacher et al., Reference Dambacher, Schuhmann, Lobbestael, Arntz, Brugman and Sack2015; Soltaninejad et al., Reference Soltaninejad, Nejati and Ekhtiari2015a); however, improved performance on inhibitory control tasks in healthy adults has been reported when stimulating T4-Fz and F8-Cz intersection (Cunillera, Brignani, Cucurell, Fuentemilla, & Miniussi, Reference Cunillera, Brignani, Cucurell, Fuentemilla and Miniussi2016; Jacobson, Javitt, & Lavidor, Reference Jacobson, Javitt and Lavidor2011, Reference Jacobson, Ezra, Berger and Lavidor2012; Stramaccia et al., Reference Stramaccia, Penolazzi, Sartori, Braga, Mondini and Galfano2015) or F6 (Cai et al., Reference Cai, Li, Liu, Li, Feng, Wang and Xue2016; Gómez-Ariza, Martín, & Morales, Reference Gómez-Ariza, Martín and Morales2017; Hogeveen et al., Reference Hogeveen, Grafman, Aboseria, David, Bikson and Hauner2016; Sallard, Mouthon, De Pretto, & Spierer, Reference Sallard, Mouthon, De Pretto and Spierer2018), which cover the rIFC along with areas closer to the surface implicated in motor inhibition (e.g. superior and middle frontal cortex, and the supplementary motor area) (Hart et al., Reference Hart, Radua, Nakao, Mataix-Cols and Rubia2013; McCarthy et al., Reference McCarthy, Skokauskas and Frodl2014; Zhang, Geng, & Lee, Reference Zhang, Geng and Lee2017) and attention (e.g. right dlPFC, part of the dorsal attention network and typically underactivated in ADHD) (Cubillo et al., Reference Cubillo, Halari, Smith, Taylor and Rubia2012; Hart et al., Reference Hart, Radua, Nakao, Mataix-Cols and Rubia2013). Another limitation is that we could not test for weekly dose effects as ADHD symptoms were only measured at baseline, posttreatment and follow-up; yet weekly changes in CT performance did not show dose effects.
Larger, double-blind, randomised-controlled trials should systematically investigate optimal and ideally individualised stimulation protocols (e.g. different stimulation sites, intensity, duration, number of sessions, etc.) measuring clinical, cognitive and possible non-targeted cognitive outcomes. Stimulating T4-Fz and F8-Cz intersection and F6 could potentially be more effective for improving inhibitory control and attention functions in ADHD.
Conclusion
This rigorously conducted double-blind, randomised, sham-controlled trial of 15-weekday sessions of anodal tDCS over rIFC combined with CT in 50 boys with ADHD showed no clinical or cognitive improvement. Findings suggest that rIFC stimulation may not be indicated as a neurotherapy for cognitive or clinical remediation for ADHD.
Supplementary material
The supplementary material for this article can be found at https://doi.org/10.1017/S0033291721001859
Acknowledgements
We would like to thank the families and children who took part in this study, and the South London and Maudsley NHS Trust and local parent groups for their support and participation in this study. We would like to thank Yuanyuan Yang for her help in recruiting participants and data collection.
Author contributions
Conceptualisation: Samuel J. Westwood, Marion Criaud, Sheut-Ling Lam, Steve Lukito, Bruce Wexler, Roi Cohen-Kadosh, Philip Asherson and Katya Rubia. Data curation: Samuel J. Westwood, Sophie Wallace-Hanlon and Afroditi Kostara. Formal analysis: Samuel J. Westwood, Marion Criaud, Sheut-Ling Lam, Steve Lukito, Deborah Agbedjro, Roi Cohen-Kadosh and Katya Rubia. Funding acquisition: Bruce Wexler, Roi Cohen-Kadosh, Philip Asherson and Katya Rubia. Investigation: Samuel J. Westwood, Marion Criaud, Sheut-Ling Lam, Steve Lukito, Sophie Wallace-Hanlon, Olivia S. Kowalczyk, Afroditi Kostara and Joseph Mathew. Methodology: Samuel J. Westwood, Marion Criaud, Sheut-Ling Lam, Steve Lukito, Olivia S. Kowalczyk, Bruce Wexler, Roi Cohen-Kadosh, Philip Asherson and Katya Rubia. Project administration: Samuel J. Westwood and Katya Rubia. Resources: Bruce Wexler, Roi Cohen-Kadosh, Philip Asherson and Katya Rubia. Software: Marion Criaud, Bruce Wexler and Katya Rubia. Supervision: Samuel J. Westwood and Katya Rubia. Validation: Samuel J. Westwood and Katya Rubia. Visualisation: Samuel J. Westwood and Katya Rubia. Writing – original draft preparation: Samuel J. Westwood and Katya Rubia. Writing – review and editing: Samuel J. Westwood, Marion Criaud, Sheut-Ling Lam, Steve Lukito, Sophie Wallace-Hanlon, Olivia S. Kowalczyk, Deborah Agbedjro, Bruce Wexler, Roi Cohen-Kadosh and Katya Rubia.
Financial support
This work was supported by grants from Action Medical Research (GN2426), the Garfield Weston Foundation, and National Institute for Health Research (NIHR) Biomedical Research Centre at South London and the Maudsley NHS Foundation Trust, and King's College London to KR. KR has received additional research support for other projects from the Medical Research Council (MR/P012647/1), and the National Institute for Health Research (NIHR) Biomedical Research Centre at South London and the Maudsley NHS Foundation Trust, and King's College London. The views expressed are those of the author(s) and not necessarily those of the NHS, the NIHR or the Department of Health and Social Care. The funders were not involved in the collection, analysis and interpretation of data; in the writing of the report; or in the decision to submit the article for publication.
Conflict of interest
Bruce E. Wexler is Chief Scientist and an equity holder in the Yale Start Up company C8 Sciences that sells the cognitive training program evaluated in this study. RCK serves on the scientific advisory boards for Neuroelectrics and Innosphere. PA reports honoraria for consultancy to Shire/Takeda, Eli Lilly and Novartis; educational and research awards from Shire, Lilly, Novartis, Vifor Pharma, GW Pharma and QbTech; and speaking at sponsored events for Shire, Lilly, Flynn Pharma and Novartis. KR has received funding from Takeda pharmaceuticals for another project.
Ethical standards
The authors assert that all procedures contributing to this work comply with the ethical standards of the relevant national and institutional committees on human experimentation and with the Helsinki Declaration of 1975, as revised in 2008.