Background
Iron deficiency is the most common nutritional deficiency in the world (CDC, 2002; Looker, Cogswell, & Gunter, Reference Looker, Cogswell and Gunter2002). After initially decreasing with the introduction of food enrichment in the United States, the prevalence of iron deficiency has resurged, particularly among certain age, sex, and racial/ethnic groups (Gupta, Hamner, Suchdev, Flores-Ayala, & Mei, Reference Gupta, Hamner, Suchdev, Flores-Ayala and Mei2017; Looker et al., Reference Looker, Cogswell and Gunter2002; Sun & Weaver, Reference Sun and Weaver2021). For instance, between the 1988–1994 and the 1999–2000 NHANES survey, the prevalence of iron deficiency increased from about 1% to 5% in 12- to 15-year-old males. Moreover, while the prevalence of iron deficiency ranges between 9% and 16% in 12- to 19-year-old females, it is nearly twice as prevalent in non-Hispanic Black and Mexican American females compared to their non-Hispanic White counterparts (Gupta et al., Reference Gupta, Hamner, Suchdev, Flores-Ayala and Mei2017; Looker et al., Reference Looker, Cogswell and Gunter2002; WHO, 2001).
Iron deficiency may have significant implications for mental health. Iron is an essential micronutrient, involved in oxygen transport, cellular respiration, and DNA synthesis (Beard & Connor, Reference Beard and Connor2003; Youdim, Reference Youdim2008). The main mechanism for the brain to uptake iron primarily involves endocytosis of transferrin bound to its receptor (TfR1), with a significant contribution by the divalent metal transporter 1 (DMT1) (Rouault & Cooperman, Reference Rouault and Cooperman2006; Wade, Chiou, & Connor, Reference Wade, Chiou and Connor2019). Ferritin can also be directly transported across the blood-brain barrier (BBB) (Wade et al., Reference Wade, Chiou and Connor2019). Oligodendrocytes have both a high content and utilization rate of iron (Moller et al., Reference Moller, Bossoni, Connor, Crichton, Does, Ward and Ronen2019). In contrast, while neurons have a high iron requirement, they have little capacity to store it (Connor & Menzies, Reference Connor and Menzies1996), making them particularly vulnerable to iron deficiency. Additionally, brain iron content differs by anatomical region and age, with the basal ganglia and red nucleus containing the most iron, while the cortical gray and white matter have low iron content (Haacke et al., Reference Haacke, Cheng, House, Liu, Neelavalli, Ogg and Obenaus2005; Hallgren & Sourander, Reference Hallgren and Sourander1958). This distribution, already apparent in childhood and adolescence (Peterson et al., Reference Peterson, Kwon, Luna, Larsen, Prouty, De Bellis and Pfefferbaum2019), accentuates with age (Sedlacik et al., Reference Sedlacik, Boelmans, Lobel, Holst, Siemonsen and Fiehler2014).
Mechanistic studies have implicated iron deficiency in monoaminergic signaling impairment, partially mediated by the fact that iron is a cofactor for tyrosine hydroxylase (Anderson et al., Reference Anderson, Fordahl, Cooney, Weaver, Colyer and Erikson2009; Baumgartner et al., Reference Baumgartner, Smuts, Malan, Arnold, Yee, Bianco and Zimmermann2012a, Reference Baumgartner, Smuts, Malan, Arnold, Yee, Bianco and Zimmermann2012b; Baumgartner, Smuts, & Zimmermann, Reference Baumgartner, Smuts and Zimmermann2014; Beard, Erikson, & Jones, Reference Beard, Erikson and Jones2002; Burhans et al., Reference Burhans, Dailey, Beard, Wiesinger, Murray-Kolb, Jones and Beard2005; Coe, Lubach, Bianco, & Beard, Reference Coe, Lubach, Bianco and Beard2009; Jellen et al., Reference Jellen, Lu, Wang, Unger, Earley, Allen and Jones2013). Iron deficiency is associated with alterations in the expression of dopamine-related genes and decreased density of dopamine transporters and dopamine D1 and D2 receptors in the basal ganglia (Beard, Chen, Connor, & Jones, Reference Beard, Chen, Connor and Jones1994; Burhans et al., Reference Burhans, Dailey, Beard, Wiesinger, Murray-Kolb, Jones and Beard2005; Erikson, Jones, & Beard, Reference Erikson, Jones and Beard2000; Erikson, Jones, Hess, Zhang, & Beard, Reference Erikson, Jones, Hess, Zhang and Beard2001; Jellen et al., Reference Jellen, Lu, Wang, Unger, Earley, Allen and Jones2013; Nelson, Erikson, Pinero, & Beard, Reference Nelson, Erikson, Pinero and Beard1997; Pino et al., Reference Pino, da Luz, Antunes, Giampa, Martins and Lee2017). Iron deficiency is also associated with disrupted serotoninergic and noradrenergic function as well as with impaired total mitochondrial oxidative capacity at the beginning of peak dendritic growth (Bastian, von Hohenberg, Georgieff, & Lanier, Reference Bastian, von Hohenberg, Georgieff and Lanier2019; Baumgartner et al., Reference Baumgartner, Smuts, Malan, Arnold, Yee, Bianco and Zimmermann2012a, Reference Baumgartner, Smuts, Malan, Arnold, Yee, Bianco and Zimmermann2012b, Reference Baumgartner, Smuts and Zimmermann2014; Mohamed, Unger, Kambhampati, & Jones, Reference Mohamed, Unger, Kambhampati and Jones2011). These abnormalities result in cognitive and behavioral deficits, including inattention and anxiety-like behaviors (Beard et al., Reference Beard, Chen, Connor and Jones1994, Reference Beard, Erikson and Jones2002; Carlson, Stead, Neal, Petryk, & Georgieff, Reference Carlson, Stead, Neal, Petryk and Georgieff2007; Fretham et al., Reference Fretham, Carlson, Wobken, Tran, Petryk and Georgieff2012; Golub, Hogrefe, & Germann, Reference Golub, Hogrefe and Germann2007; Kennedy et al., Reference Kennedy, Dimova, Siddappa, Tran, Gewirtz and Georgieff2014; Mohamed et al., Reference Mohamed, Unger, Kambhampati and Jones2011; Schmidt, Waldow, Grove, Salinas, & Georgieff, Reference Schmidt, Waldow, Grove, Salinas and Georgieff2007; Tran et al., Reference Tran, Kennedy, Pisansky, Won, Gewirtz, Simmons and Georgieff2016).
Consistent with these preclinical findings, low body iron has been associated with attention-deficit hyperactivity disorder (ADHD) and several observational and intervention studies in women of reproductive age have implicated iron deficiency in depressive symptoms (Beard et al., Reference Beard, Hendricks, Perez, Murray-Kolb, Berg, Vernon-Feagans and Tomlinson2005; Corwin, Murray-Kolb, & Beard, Reference Corwin, Murray-Kolb and Beard2003; Fordy & Benton, Reference Fordy and Benton1994; Karl et al., Reference Karl, Lieberman, Cable, Williams, Young and McClung2010; Low, Speedy, Styles, De-Regil, & Pasricha, Reference Low, Speedy, Styles, De-Regil and Pasricha2016; Rangan, Blight, & Binns, Reference Rangan, Blight and Binns1998; Vahdat Shariatpanaahi, Vahdat Shariatpanaahi, Moshtaaghi, Shahbaazi, & Abadi, Reference Vahdat Shariatpanaahi, Vahdat Shariatpanaahi, Moshtaaghi, Shahbaazi and Abadi2007). Surprisingly, however, little research has examined the association of iron deficiency with internalizing (i.e. depressive and anxiety) symptoms in school-aged children and adolescents (Matsuo et al., Reference Matsuo, Rosenberg, Easter, MacMaster, Chen, Nicoletti and Soares2008; Vulser et al., Reference Vulser, Lemaitre, Artiges, Miranda, Penttilä, Struve and Paillère-Martinot2015). One retrospective Japanese study in 6- to 15-year-old children with serum ferritin concentration (sF) <50 ng/mL, referred for a child and adolescent psychiatric evaluation, found iron supplementation effective at increasing sF and reducing psychiatric symptoms (Mikami et al., Reference Mikami, Okazawa, Kimoto, Akama, Onishi, Takahashi and Matsumoto2019). Two randomized double-blind placebo-controlled iron supplementation studies in adolescent females examining cognitive outcomes reached divergent conclusions, with only one reporting an improvement in ‘mood, lassitude, and concentration’ following replenishment of iron stores (Ballin et al., Reference Ballin, Berar, Rubinstein, Kleter, Hershkovitz and Meytes1992; Bruner, Joffe, Duggan, Casella, & Brandt, Reference Bruner, Joffe, Duggan, Casella and Brandt1996). Finally, one study utilized the Taiwanese national health insurance database, finding that iron deficiency anemia was associated with more than twofold increased risk for depressive or anxiety disorders, compared to those without anemia (Chen et al., Reference Chen, Su, Chen, Hsu, Huang, Chang and Bai2013).
Importantly, anemia (regardless of its etiology) is known to be associated with irritability, apathy, fatigue, low mood, and concentration difficulties (Murray-Kolb, Reference Murray-Kolb2011), complaints that overlap with internalizing symptoms, making it necessary to characterize the psychiatric effects of iron deficiency in the absence of anemia.
In this pilot study, we examined the prevalence and clinical correlates of iron deficiency in adolescent girls with and without anxiety or depressive disorders, who were otherwise healthy. We hypothesized that iron deficiency would be associated with more severe internalizing symptoms. Given that altered basal ganglia morphometry, metabolism, and perfusion have been implicated in internalizing disorders (Bastian et al., Reference Bastian, von Hohenberg, Georgieff and Lanier2019; Beard et al., Reference Beard, Chen, Connor and Jones1994; Bourre et al., Reference Bourre, Pascal, Durand, Masson, Dumont and Piciotti1984; Cammer, Reference Cammer1984a; Connor & Menzies, Reference Connor and Menzies1996; Matsuo et al., Reference Matsuo, Rosenberg, Easter, MacMaster, Chen, Nicoletti and Soares2008; Tansey & Cammer, Reference Tansey and Cammer1988; Vulser et al., Reference Vulser, Lemaitre, Artiges, Miranda, Penttilä, Struve and Paillère-Martinot2015) and that these structures are key nodes in the fronto-subcortical neural circuits, underlying various processes relevant to mood regulation (Williams, Reference Williams2016) and because iron deficiency disrupts neurotransmitter signaling in the basal ganglia (Baumgartner et al., Reference Baumgartner, Smuts, Malan, Arnold, Yee, Bianco and Zimmermann2012a, Reference Baumgartner, Smuts, Malan, Arnold, Yee, Bianco and Zimmermann2012b, Reference Baumgartner, Smuts and Zimmermann2014), we further sought to examine the association between iron deficiency and basal ganglia volumes.
Methods
Participants
This analysis used data collected in the context of two studies, with the first participant enrolled on 02/12/2016. Both enrolled participants with the same demographic and clinical characteristics, with one focused on examining gut permeability in major depressive disorder (MDD) (Calarge, Devaraj, & Shulman, Reference Calarge, Devaraj and Shulman2019), while the other focused on brain imaging (Calarge et al., Reference Calarge, Gandy, Barba Villalobos, Nguyen, Kim and Maletic-Savatic2017). In both studies, 12- to 17-year-old unmedicated females with MDD, anxiety disorders (i.e. separation anxiety disorder, generalized anxiety disorder, or social phobia), or with no psychiatric disorders (i.e. healthy controls) were enrolled from general pediatrics clinics if they had a normal body mass index (BMI, i.e. between the 5th and 85th percentile for age and sex). The presence of bipolar disorder, autistic disorder, schizophrenia, obsessive-compulsive disorder, ADHD, and/or eating disorder led to exclusion. Additional exclusionary criteria included the presence of intellectual disability or language barrier due to inability to complete study procedures, treatment with psychotropics within 6 months before study entry, and presence of a serious general medical condition (e.g. involving a vital organ) or pregnancy. Individuals exposed to major traumatic events (e.g. death of loved ones, natural disaster, etc.) in the prior 6 months were also excluded (Calarge et al., Reference Calarge, Devaraj and Shulman2019). Furthermore, the use of non-steroidal anti-inflammatory drugs or medications for seasonal allergies or asthma in the prior week, of pre/probiotics in the prior 6 weeks, or of antibiotics in the prior 6 months; or a major change in diet (e.g. switching to vegetarian or excluding a food group, like eggs or dairy products) in the prior 6 weeks or the presence of functional gastrointestinal disorders all led to exclusion from the first study, as these factors may alter gut permeability. Participants wearing braces or having any contraindication for undergoing magnetic resonance imaging (MRI) were excluded from the brain imaging study.
The study was approved by the Baylor College of Medicine Institutional Review Board. After the study details were reviewed, written consent was obtained from parents or legal guardians and verbal assent from the participants.
Procedures
A board-certified child and adolescent psychiatrist conducted an unstructured interview with the adolescent and parent. The MINI International Psychiatric Interview V6.0, a structured clinical interview based on the fifth edition of the Diagnostic and Statistical Manual for Mental Disorders (DSM-5) (American Psychiatric Association, 2013), was administered to the parent by trained research staff. Also, the participants completed the Center for Epidemiological Studies Depression Scale for Children (CESD-C) and the Screen for Child Anxiety-Related Disorders (SCARED), both well-validated and widely used measures of depressive and anxiety symptoms, respectively (Birmaher et al., Reference Birmaher, Brent, Chiappetta, Bridge, Monga and Baugher1999, Reference Birmaher, Khetarpal, Brent, Cully, Balach, Kaufman and Neer1997; Faulstich, Carey, Ruggiero, Enyart, & Gresham, Reference Faulstich, Carey, Ruggiero, Enyart and Gresham1986; Weissman, Orvaschel, & Padian, Reference Weissman, Orvaschel and Padian1980). Best-estimate DSM-5-based diagnoses were generated, using all available clinical information.
Race and ethnicity were self-reported. The parents also reported their household income and educational level. Participants rated their sexual maturity using a validated form (Calarge, Acion, Kuperman, Tansey, & Schlechte, Reference Calarge, Acion, Kuperman, Tansey and Schlechte2009; Calarge, Mills, Ziegler, & Schlechte, Reference Calarge, Mills, Ziegler and Schlechte2018). When applicable, they also noted the first day of their last menstrual period, the typical duration of their cycle, and the average number of sanitary pads used per day, during their menses. Participants 13 years of age and older were also queried about the use of hormonal contraception. The parents completed a questionnaire about birth history, including prenatal care, in-utero prescribed or illicit drug exposure, and pre/perinatal complications. The parents were also asked to rate their confidence level in the information recalled. Additionally, medical records were reviewed, since birth when available, to extract information related to body iron status (i.e. hemoglobin and history of anemia or transfusion).
Height was measured to the nearest 0.1 cm with a wall-mounted stadiometer (Ayrton Model S100, Hamburg, Germany) and weight was recorded to the nearest 0.1 kg (Seca 220 digital scale, Hamburg, Germany) with participants in indoor clothes without shoes. These measurements were obtained in duplicate, and the average was used.
The 2004 Block Food Frequency Questionnaire (FFQ) for Ages 8–17 was completed by the participants, with parents assisting as needed (D'Occhio, Fordyce, Whyte, Aspden, & Trigg, Reference D'Occhio, Fordyce, Whyte, Aspden and Trigg2000). The FFQ includes 77 food items, developed based on the NHANES 1999–2002 dietary recall data. The nutrient database was developed from the USDA Nutrient Database for Dietary Studies, version 1.0. Individual portion size is asked, and pictures are provided to enhance the accuracy of quantification. When available, the FFQ allowed estimating daily iron intake. Poor iron intake was defined as an estimated daily intake <8 mg/day for 12- and 13-year-olds and <15 mg/day for older female participants (Trumbo, Schlicker, Yates, & Poos, Reference Trumbo, Schlicker, Yates and Poos2002).
Participants underwent a venous blood draw, in the morning, after at least a 9 h fast. Serum was used to measure sF (Immunoassay on Vitros 5600 Chemistry System, Ortho Clinical Diagnostics, Raritan, NJ, USA).
Brain imaging acquisition and segmentation
MRI of the brain was obtained using a 3 T Siemens PRISMA scanner equipped with a 64-channel head-neck coil. Anatomical imaging included a 3D MPRAGE T1-weighted scan sequence (TR/TI/TE = 2400/1000/2.24 ms, 0.8 mm isotropic resolution). The raw MRI data were inspected by a trained operator for scanner-related artifacts (including head motion) immediately following scan acquisition. T1-weighted MRI scans were preprocessed and analyzed using Freesurfer version 6.0 (http://surfer.nmr.mgh.harvard.edu), a brain imaging software designed to characterize the morphometric properties of the brain (Fischl et al., Reference Fischl, Salat, Busa, Albert, Dieterich, Haselgrove and Dale2002, Reference Fischl, Salat, van der Kouwe, Makris, Segonne, Quinn and Dale2004). Subcortical brain volumes were segmented based on the standardized Aseg atlas. For this study, we focused on basal ganglia volume including the putamen, globus pallidus, and the caudate (online Supplementary Fig.), given these structures' higher iron content and to minimize the risk for type 1 error (Haacke et al., Reference Haacke, Cheng, House, Liu, Neelavalli, Ogg and Obenaus2005; Hallgren & Sourander, Reference Hallgren and Sourander1958). Total intracranial volume was adjusted for in the analysis of these basal ganglia subregions.
Statistical analysis
BMI was computed as weight/height2 (kg/m2) and age-sex-specific BMI Z-scores were generated based on the 2000 Centers for Disease Control and Prevention normative data (Ogden et al., Reference Ogden, Kuczmarski, Flegal, Mei, Guo, Wei and Johnson2002). Following published guidelines, iron deficiency was defined as sF < 15 ng/mL (WHO, 2011). However, in light of evidence suggesting that such cutoff may be too conservative (Garcia-Casal et al., Reference Garcia-Casal, Pena-Rosas, Urrechaga, Escanero, Huo, Martinez and Lopez-Perez2018; Mast, Blinder, Gronowski, Chumley, & Scott, Reference Mast, Blinder, Gronowski, Chumley and Scott1998; North, Dallalio, Donath, Melink, & Means, Reference North, Dallalio, Donath, Melink and Means1997), we also examined the association of iron deficiency with outcomes of interest using the more liberal cutoff of 20 ng/mL.
Given the comorbidity between depressive and anxiety disorders, we computed an internalizing symptoms composite z-score capturing the symptoms severity on both the SCARED and CESD-C (Song, Lin, Ward, & Fine, Reference Song, Lin, Ward and Fine2013). Group differences between participants with and those without internalizing disorders were compared using the Wilcoxon rank-sum test for continuous variables and χ2 or Fisher's exact test for categorical variables. Multivariable regression analyses examined the associations between iron deficiency status and outcomes of interest (e.g. depression or anxiety symptom severity or basal ganglia volume), accounting for relevant confounders. Cohen's d effect size was computed (Cohen, Reference Cohen1988). Analyses used procedures from SAS version 9.4 for Windows (SAS Institute Inc, Cary, NC, USA).
Results
Participants
Table 1 summarizes the demographic and clinical characteristics of the 40 participants contributing data to this analysis. Although no significant differences in demographic characteristics between participants were found, participants with internalizing disorders tended to be older, and more likely to be Hispanic and post-menarchal. Although participants with internalizing disorders had lower sF and a numerically higher prevalence of iron deficiency (whether defined as sF < 15 or 20 ng/mL), these differences did not reach statistical significance (Table 1).
Table 1. Demographic and clinical characteristics of female adolescents (n = 40) with internalizing disorders v. healthy controls

Mean ± s.d., unless otherwise specified.
SCARED, Screen for Child Anxiety Related Disorders; CESD-C, Center for Epidemiological Studies Depression Scale for Children.
a BMI Z-score: age-sex-specific body mass index.
b Dietary data were available for only 15 participants with internalizing disorders and 6 healthy controls.
c Luteal phase was defined as within 14 days of the end of each participant's ‘average’ menstrual cycle length.
d Menorrhagia was defined as duration of menses of >7 days or use of >12 sanitary pads per day.
e Only participants ⩾13 years old (n = 35) were queried about hormonal contraceptives use.
Association between iron markers and internalizing symptoms
Serum ferritin concentration was inversely correlated with internalizing symptom severity as captured by the composite z-score (r = −0.36, p < 0.03), the SCARED (r = −0.34, p < 0.04), and the CESD-C (r = −0.30, p < 0.06). Notably, compared to those without iron deficiency (defined as sF < 20 ng/mL), participants with iron deficiency had significantly higher internalizing symptom composite z-score (z-score = 0.73 v. −0.73, respectively, Cohen's d = 0.82, p < 0.02) as well as higher scores on the SCARED (p < 0.002) and the CESD-C (p < 0.03) (Fig. 1A). Moreover, the magnitude of this association was even greater in participants with sF < 15 ng/mL compared to those with sF ⩾ 15 ng/mL (Cohen's d = 1.01, p < 0.005 for the composite z-score; d = 1.08 for the SCARED, p < 0.003; and d = 0.83 for the CESD-C, p < 0.02; Fig. 1B).

Fig. 1. (A) Least squares means for internalizing symptom severity in unmedicated adolescent females with sF < (ID+, blue) v. ⩾20 ng/mL (ID−, orange), adjusted for age. (B) Same comparison but between those with sF < (ID++, green) v. ⩾15 ng/mL (ID−−, purple).
Iron markers and basal ganglia morphology
Of the 24 participants who underwent brain imaging, 16 (67%) had an internalizing disorder and nine (38%) had sF < 15 ng/ml. Differences in demographic variables or in iron deficiency prevalence between participants with v. those without internalizing disorders were not statistically significant (all p values >0.05).
After adjusting for age and total intracranial volume, sF was inversely associated with the volume of the left caudate (Spearman's r = −0.46, p < 0.04, Table 2), left putamen (r = −0.58, p < 0.005), and the right putamen (r = −0.53, p < 0.01). Similarly, there was a statistical trend for the left putamen and caudate volumes to be larger in participants with iron deficiency, as defined by sF < 15 ng/mL (Fig. 2A). The effect sizes were somewhat smaller for the right basal ganglia structures, compared to the left (Fig. 2B).
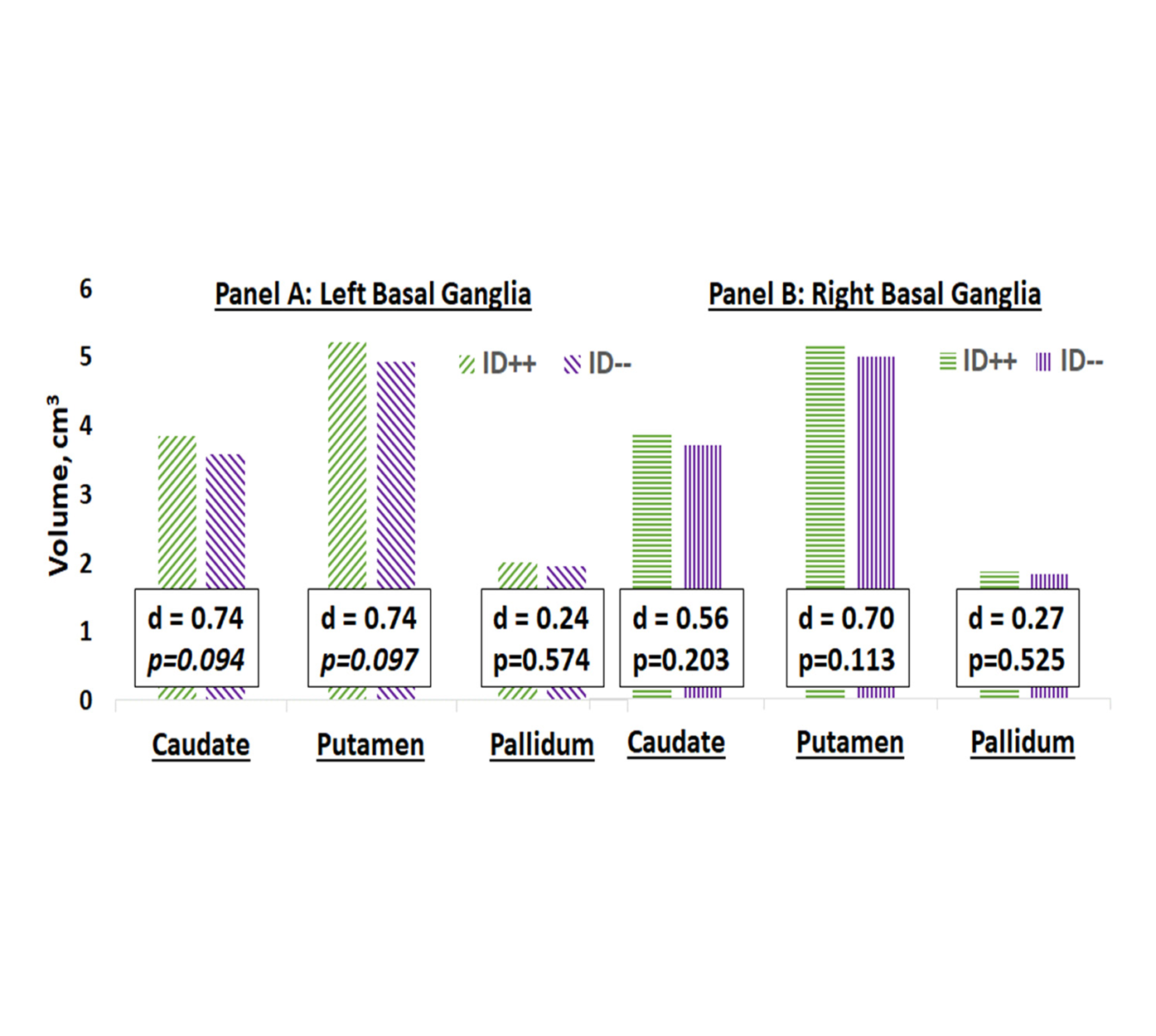
Fig. 2. Cohen's d for the differences in least squares means for basal ganglia structures volumes in females with sF < (ID++, green) v. ⩾15 ng/mL (ID−−, purple), accounting for age and total intracranial volume.
Table 2. Least squares means for basal ganglia structures volumes (mL) in unmedicated female adolescents (n = 24) with iron deficiency v. those without, adjusted for age and intracranial volume

Discussion
To our knowledge, this pilot study is the first to examine the association between body iron stores, internalizing symptoms severity, and brain structure in unmedicated adolescent females, who have undergone a thorough psychiatric assessment. Body iron stores were inversely associated with more severe anxiety and depressive symptoms and positively associated with basal ganglia morphometry.
Iron deficiency and internalizing disorders in youth
Internalizing disorders in adolescents are common and impairing, with recent data suggesting increasing incidence (Twenge, Cooper, Joiner, Duffy, & Binau, Reference Twenge, Cooper, Joiner, Duffy and Binau2019). Many factors are likely implicated, given the heterogeneous nature of depressive and anxiety disorders. That iron deficiency would contribute to the recent change in the prevalence of internalizing disorders in adolescents is plausible for several reasons: (1) iron plays a critical role in the brain, potentially impacting the structure and function of mood-relevant areas, (2) iron deficiency is common in adolescence (CDC, 2002, 2014; Gupta et al., Reference Gupta, Hamner, Suchdev, Flores-Ayala and Mei2017; Looker et al., Reference Looker, Cogswell and Gunter2002), and (3) available evidence suggests that replenishing iron stores may improve internalizing symptoms (Mikami et al., Reference Mikami, Okazawa, Kimoto, Akama, Onishi, Takahashi and Matsumoto2019).
To our knowledge, however, the prevalence of iron deficiency in adolescents with internalizing disorders has not been examined. We found that 32% of our participants had sF < 15 ng/mL. This high prevalence of iron deficiency, which may be partially accounted for by the over-representation of Hispanic females in our study (Gupta et al., Reference Gupta, Hamner, Suchdev, Flores-Ayala and Mei2017; Looker et al., Reference Looker, Cogswell and Gunter2002; WHO, 2001), is particularly troubling given that our participants had undergone a thorough screening to rule out many general medical conditions that could cause iron deficiency. Moreover, the pre/perinatal history and the medical record review exclude the possibility that our findings represent chronic sequelae of early-life or concurrent anemia (online Supplementary Data). As such, to the best of our knowledge, our participants were healthy, had received good prenatal care, had not had perinatal anemia, but did have low iron intake despite coming from households with a median income nearly double the national average (Table 1). This low iron intake is consistent with recent data showing a trend for reduction in iron intake in the US population, with an associated increase in the prevalence of iron deficiency anemia. The large effect sizes we found for the association between iron deficiency and internalizing symptoms highlight the potential for replenishing iron stores to reverse the recently documented increase in the prevalence of depressive and anxiety disorders in adolescents (Mojtabai & Olfson, Reference Mojtabai and Olfson2020; Mojtabai, Olfson, & Han, Reference Mojtabai, Olfson and Han2016)
Brain iron homeostasis during iron deficiency
Understanding the interplay between iron deficiency and brain structure and function across the lifespan requires one to consider three inter-related factors: (1) iron transport into the brain, (2) hierarchy of iron distribution to bodily systems, and (3) how the presence of iron deficiency has been defined. Once the BBB matures during infancy, iron transport into the brain becomes tightly regulated, protecting it from daily fluctuations in systemic iron levels (Wade et al., Reference Wade, Chiou and Connor2019). Preclinical and clinical studies have established that iron is prioritized for erythropoiesis, for evident survival advantage. As the body is faced with insufficient iron intake to meet its needs, iron reserves are tapped in a relatively hierarchical order (e.g. liver, skeletal muscle, heart, etc.) and, with increasing iron deficiency severity, iron is eventually diverted even from the brain to the bone marrow (Ennis, Dahl, Rao, & Georgieff, Reference Ennis, Dahl, Rao and Georgieff2018; Guiang, Georgieff, Lambert, Schmidt, & Widness, Reference Guiang, Georgieff, Lambert, Schmidt and Widness1997; Zamora, Guiang, Widness, & Georgieff, Reference Zamora, Guiang, Widness and Georgieff2016). Finally, historically, iron deficiency has been the focus of public health interventions due to its association with anemia. Current guidelines to diagnose ID have been based on anemia-relevant markers, such as bone marrow iron content and sF below which anemia develops (WHO, 2011). They are not based on the assessments of brain iron content or the emergence of neuropsychiatric manifestations. Considering these three factors together, it thus follows that, compared to infants who may not benefit from the protective effect of the BBB yet, brain iron content in adolescents will only be impacted once a threshold (in terms of severity of body iron stores depletion) has been crossed. However, what severity of iron deficiency is needed to overcome the homeostatic mechanisms of the BBB, resulting in brain iron depletion, is unknown. What appears certain is that brain iron is drawn upon before anemia develops. This is supported by studies in adolescents and young adults with iron deficiency but without anemia finding improvement in neurocognitive and emotional functioning following iron supplementation (Ballin et al., Reference Ballin, Berar, Rubinstein, Kleter, Hershkovitz and Meytes1992; Beard et al., Reference Beard, Hendricks, Perez, Murray-Kolb, Berg, Vernon-Feagans and Tomlinson2005; Corwin et al., Reference Corwin, Murray-Kolb and Beard2003; Fordy & Benton, Reference Fordy and Benton1994; Karl et al., Reference Karl, Lieberman, Cable, Williams, Young and McClung2010; Low et al., Reference Low, Speedy, Styles, De-Regil and Pasricha2016; Rangan et al., Reference Rangan, Blight and Binns1998; Vahdat Shariatpanaahi et al., Reference Vahdat Shariatpanaahi, Vahdat Shariatpanaahi, Moshtaaghi, Shahbaazi and Abadi2007; Verdon et al., Reference Verdon, Burnand, Stubi, Bonard, Graff, Michaud and Favrat2003). This appears consistent with our findings both concerning internalizing symptom severity and subcortical structures volumes.
Notably, we found a larger association between internalizing symptom severity and iron deficiency (a categorical variable) compared to sF (a continuous variable). This is consistent with the BBB's role in protecting the brain from fluctuations in body iron until the homeostatic mechanisms are overwhelmed (Wade et al., Reference Wade, Chiou and Connor2019). When iron deficiency is defined more liberally, as sF < 20 ng/mL, the association with symptom severity remains significant, albeit of a smaller magnitude, suggesting that the cutoff for sF of <15 ng/mL to define iron deficiency may be overly conservative, having been established based on hematological outcomes without regard to the function of other organs, like the brain. In fact, for those participants with a hemoglobin level obtained within one year before study entry, anemia was quite uncommon with only one person affected (online Supplementary Data). In patients with restless leg syndrome, iron supplementation aims to raise sF > 50 ng/mL. However, the association between peripheral and brain iron content has not been well examined in general and not at all in adolescents. Identifying the threshold below which iron deficiency starts drawing on brain iron is an area that requires urgent attention, with potentially widespread clinical implications.
Iron deficiency and brain structure and function
Brain iron is also critical for myelin formation (Bastian et al., Reference Bastian, von Hohenberg, Georgieff and Lanier2019; Beard & Connor, Reference Beard and Connor2003; Lange & Que, Reference Lange and Que1998). Oligodendrocytes are enriched with iron-requiring enzymes involved in lipid metabolism, needed for initial myelin deposition as well as for maintaining its integrity (Bourre et al., Reference Bourre, Pascal, Durand, Masson, Dumont and Piciotti1984; Cammer, Reference Cammer1984a, Reference Cammer and Norton1984b; Connor & Menzies, Reference Connor and Menzies1996; Tansey & Cammer, Reference Tansey and Cammer1988). Two brain imaging studies have explored the association between brain iron content and brain function in children. Peterson et al. used data collected in healthy 12- to 21-year-olds, enrolled in the National Consortium on Alcohol and Neurodevelopment in Adolescence study (Peterson et al., Reference Peterson, Kwon, Luna, Larsen, Prouty, De Bellis and Pfefferbaum2019). They repurposed scans obtained for functional MRI and diffusion tensor imaging (DTI) to track brain iron distribution, replicating the fact that several subcortical nuclei are iron-rich, and that iron accumulates with increasing age but plateaus by early adulthood. Additionally, working memory speed was inversely associated with iron signal in the left dentate nucleus and substantia nigra (Peterson et al., Reference Peterson, Kwon, Luna, Larsen, Prouty, De Bellis and Pfefferbaum2019). Similarly, Carpenter et al. used brain imaging to estimate brain iron content in 39 healthy children (56% female, mean age 9.5 ± 1.3 years), finding again a positive association between age and iron content in the basal ganglia (Carpenter et al., Reference Carpenter, Li, Wei, Wu, Xiao, Liu and Egger2016). Moreover, the right caudate iron content was positively associated with spatial IQ (Carpenter et al., Reference Carpenter, Li, Wei, Wu, Xiao, Liu and Egger2016).
Using structural brain imaging, we found an inverse association between body iron status and the volumes of the putamen and the left caudate. The effect sizes were smaller for the right hemisphere structures, perhaps reflecting the lateralization in brain iron content observed in some studies (Langkammer et al., Reference Langkammer, Schweser, Krebs, Deistung, Goessler, Scheurer and Reichenbach2012; Xu, Wang, & Zhang, Reference Xu, Wang and Zhang2008). This inverse association may reflect the fact that brain iron moderates the decrease in subcortical structures volume observed during late childhood and adolescence (Raznahan et al., Reference Raznahan, Shaw, Lerch, Clasen, Greenstein, Berman and Giedd2014; Wierenga et al., Reference Wierenga, Langen, Ambrosino, van Dijk, Oranje and Durston2014). It may also reflect iron deficiency-induced impairment in dopaminergic signaling (Beard et al., Reference Beard, Chen, Connor and Jones1994; Burhans et al., Reference Burhans, Dailey, Beard, Wiesinger, Murray-Kolb, Jones and Beard2005; Erikson et al., Reference Erikson, Jones and Beard2000, Reference Erikson, Jones, Hess, Zhang and Beard2001; Jellen et al., Reference Jellen, Lu, Wang, Unger, Earley, Allen and Jones2013; Nelson et al., Reference Nelson, Erikson, Pinero and Beard1997; Pino et al., Reference Pino, da Luz, Antunes, Giampa, Martins and Lee2017). Preclinical studies have linked stimulant-induced reduction in dopamine D2 receptor density in the ventral striatum with an increase in putamen volume (Chang et al., Reference Chang, Cloak, Patterson, Grob, Miller and Ernst2005; Churchwell, Carey, Ferrett, Stein, & Yurgelun-Todd, Reference Churchwell, Carey, Ferrett, Stein and Yurgelun-Todd2012; Groman, Morales, Lee, London, & Jentsch, Reference Groman, Morales, Lee, London and Jentsch2013; Jan, Lin, Miles, Kydd, & Russell, Reference Jan, Lin, Miles, Kydd and Russell2012; Jernigan et al., Reference Jernigan, Gamst, Archibald, Fennema-Notestine, Mindt, Marcotte and Grant2005). Similarly, clinical studies have found enlarged putamen in patients with stimulant use disorders (Ersche et al., Reference Ersche, Acosta-Cabronero, Jones, Ziauddeen, van Swelm, Laarakkers and Williams2017, Reference Ersche, Barnes, Jones, Morein-Zamir, Robbins and Bullmore2011, Reference Ersche, Jones, Williams, Turton, Robbins and Bullmore2012; Jacobsen, Giedd, Gottschalk, Kosten, & Krystal, Reference Jacobsen, Giedd, Gottschalk, Kosten and Krystal2001). Finally, our finding is also consistent with increased basal ganglia volumes following extended treatment with ‘typical’ antipsychotics, characterized by potent dopamine D2 antagonist activity (Corson, Nopoulos, Miller, Arndt, & Andreasen, Reference Corson, Nopoulos, Miller, Arndt and Andreasen1999; Navari & Dazzan, Reference Navari and Dazzan2009). The basal ganglia are a key node in the cortico-striato-thalamo-cortical loops, subserving a multitude of neuropsychological processes implicated in psychopathologies, such as inhibitory control and reward processing (Drysdale et al., Reference Drysdale, Grosenick, Downar, Dunlop, Mansouri, Meng and Liston2017; Janiri et al., Reference Janiri, Moser, Doucet, Luber, Rasgon, Lee and Frangou2019; Pizzagalli et al., Reference Pizzagalli, Holmes, Dillon, Goetz, Birk, Bogdan and Fava2009; Wei & Wang, Reference Wei and Wang2016; Williams, Reference Williams2016). Whether iron supplementation would reverse these structural changes requires future studies.
Timing of iron deficiency and persistence of neuropsychiatric sequelae
Given the key role iron plays in a broad set of metabolic processes, it is not surprising that iron deficiency would be associated with cognitive and neuropsychiatric deficits (Vulser et al., Reference Vulser, Wiernik, Hoertel, Thomas, Pannier, Czernichow and Lemogne2016). However, the nature, severity, and chronicity of these effects are closely tied to the time during development when iron deficiency ensues (Barks, Hall, Tran, & Georgieff, Reference Barks, Hall, Tran and Georgieff2019; Georgieff, Reference Georgieff2017). The earlier the exposure, the broader the impact on neurocognitive functioning given the brain's substantial iron-dependent metabolic needs early in life, when brain structures underlying basic neurocognitive processes are rapidly developing (Barks et al., Reference Barks, Hall, Tran and Georgieff2019; Georgieff, Reference Georgieff2017; Georgieff, Brunette, & Tran, Reference Georgieff, Brunette and Tran2015; Lozoff & Georgieff, Reference Lozoff and Georgieff2006). This is thought to involve irreversible impairment in gene expression, affecting neuronal growth and plasticity (Georgieff et al., Reference Georgieff, Brunette and Tran2015). These ‘sensitive periods’ have been shown both in animal and clinical studies (Barks et al., Reference Barks, Hall, Tran and Georgieff2019; Georgieff, Reference Georgieff2017; Mudd et al., Reference Mudd, Fil, Knight, Lam, Liang and Dilger2018). For instance, children exposed to iron deficiency in-utero or in infancy, show motor, cognitive, emotional, and social deficits long after iron stores had been replenished (Barks et al., Reference Barks, Hall, Tran and Georgieff2019; Doom et al., Reference Doom, Richards, Caballero, Delva, Gahagan and Lozoff2018; Lozoff, Jimenez, Hagen, Mollen, & Wolf, Reference Lozoff, Jimenez, Hagen, Mollen and Wolf2000; Lozoff et al., Reference Lozoff, Smith, Kaciroti, Clark, Guevara and Jimenez2013). Additionally, in children with ADHD, Turner et al. found that a small mean corpuscular volume (a marker of iron deficiency) in the toddler years predicted poor response to psychostimulant treatment in elementary school (Turner, Xie, Zimmerman, & Calarge, Reference Turner, Xie, Zimmerman and Calarge2010). However, in contrast to what appears as persistent sequelae when iron deficiency occurs early in life, cognitive deficits in children and adults with iron deficiency, with or without anemia, can improve with iron repletion (Chmielewska et al., Reference Chmielewska, Dziechciarz, Gieruszczak-Bialek, Horvath, Piescik-Lech, Ruszczynski and Szajewska2019; Grantham-McGregor & Ani, Reference Grantham-McGregor and Ani2001; Low et al., Reference Low, Speedy, Styles, De-Regil and Pasricha2016; McCann & Ames, Reference McCann and Ames2007). For example, iron supplementation in 5- to 8-year-old children with ADHD and women with postpartum depression reduces symptom severity, particularly in those with iron deficiency (Konofal et al., Reference Konofal, Lecendreux, Deron, Marchand, Cortese, Zaim and Arnulf2008; Sever, Ashkenazi, Tyano, & Weizman, Reference Sever, Ashkenazi, Tyano and Weizman1997; Wassef, Nguyen, & St-Andre, Reference Wassef, Nguyen and St-Andre2019). In other words, which sequelae arise closely depend on which brain areas are most metabolically actively and/or rapidly developing at the time of iron deficiency, making them particularly vulnerable to its impact (Lozoff & Georgieff, Reference Lozoff and Georgieff2006; Vulser et al., Reference Vulser, Wiernik, Hoertel, Thomas, Pannier, Czernichow and Lemogne2016).
Some limitations of our pilot study must be acknowledged. First, our findings are based on a relatively small sample size, requiring replication in a larger study. Second, given that internalizing disorders disproportionately affect adolescent females, we did not recruit males. As such, whether our findings extend to males remains to be seen. Third, because this was a cross-sectional evaluation, the direction of the causal association between iron deficiency and our outcomes of interest cannot be established. While low sF is the most specific non-invasive marker of iron deficiency, with excellent reproducibility (Belza, Ersboll, Henriksen, Thilsted, & Tetens, Reference Belza, Ersboll, Henriksen, Thilsted and Tetens2005), neither the duration of iron deficiency was available, nor the presence of anemia ruled out as we did not measure hemoglobin. Because some symptoms associated with anemia may overlap with internalizing symptoms (Murray-Kolb, Reference Murray-Kolb2011), future studies should exclude the confounding effect of anemia to best examine the independent effect of iron deficiency on the brain. Given the exploratory nature of this study, no correction for multiple comparisons was made. As shown in Table 1, 62% of the participants were estimated to be in the luteal phase of their menstrual cycle, a time when ferritin tends to be higher (Kim, Yetley, & Calvo, Reference Kim, Yetley and Calvo1993). As such, the rate of iron deficiency may have been even higher had we enrolled the participants upon the onset of their menstrual phase. Finally, measuring C-reactive protein would have ruled out cases of inflammation, where ferritin would have been elevated. However, the stringent inclusion/exclusion criteria and the high prevalence of iron deficiency suggest that our participants did not have acute inflammation.
In summary, given iron's role in multiple metabolic processes affecting brain structure and function, iron deficiency can have a wide-ranging impact on brain development. In youth, this may compound their proclivity to develop internalizing disorders and compromise treatment response. Future studies, ideally longitudinal, should examine how changes in iron status during pubertal maturation may moderate brain development and the emergence of psychopathology. This risk may impact males and females differently, given that iron deficiency disproportionately affects menstruating females, particularly of minority background. Moreover, future interventions should seek to examine the clinical benefits of replenishing iron stores.
Supplementary material
The supplementary material for this article can be found at https://doi.org/10.1017/S0033291721004098.
Acknowledgements
The authors thank the families and the research team members.
Financial support
This research received no specific grant from any funding agency, commercial or not-for-profit sectors.
Conflict of interest
None.