Introduction
Schizotypy refers to a set of behaviors, experiences, personality traits and beliefs, each normally distributed in the general population, that together reflect a continuum of psychosis-proneness (Claridge, Reference Claridge1997; Grant, Green, & Mason, Reference Grant, Green and Mason2018). Psychosis risk is influenced by environmental factors such as childhood trauma (Quidé et al., Reference Quidé, Cohen-Woods, O'Reilly, Carr, Elzinga and Green2018; Velikonja, Fisher, Mason, & Johnson, Reference Velikonja, Fisher, Mason and Johnson2015), with exposure to abuse and/or neglect in childhood recognized as a major risk factor for psychotic disorders (McGrath et al., Reference McGrath, Saha, Lim, Aguilar-Gaxiola, Alonso, Andrade and Collaborators2017; Varese et al., Reference Varese, Smeets, Drukker, Lieverse, Lataster, Viechtbauer and Bentall2012). The concept of schizotypy is a useful construct for studying risk for schizophrenia in the general population (Claridge, Reference Claridge1985; Eysenck, Reference Eysenck1998; Kwapil & Barrantes-Vidal, Reference Kwapil and Barrantes-Vidal2015; Meehl, Reference Meehl1990) that avoids the potential confounding effects of clinical factors such as illness duration or medication dosage (Raine, Reference Raine1991). Both schizotypy and childhood trauma exposure have been separately associated with overlapping changes in brain morphology (Teicher, Samson, Anderson, & Ohashi, Reference Teicher, Samson, Anderson and Ohashi2016; Tonini, Quidé, Kaur, Whitford, & Green, Reference Tonini, Quidé, Kaur, Whitford and Green2021a), and childhood trauma is associated with higher levels of schizotypy in people with a psychosis spectrum disorder (e.g. bipolar I disorder, schizophrenia) and in psychiatrically healthy individuals (Quidé et al., Reference Quidé, Cohen-Woods, O'Reilly, Carr, Elzinga and Green2018; Velikonja et al., Reference Velikonja, Fisher, Mason and Johnson2015). It is therefore possible that the differences in brain morphology associated with increasing schizotypy might be modified by exposure to childhood trauma, such that trauma exposure could moderate the relationship between schizotypy and variation in brain morphology. In fact, exposure to childhood trauma may influence the neurodevelopmental trajectories of brain regions critical for adequate affective and cognitive processes of vulnerable persons.
While structural brain abnormalities are ubiquitous among schizophrenia patients, the patterns of morphological differences associated with this illness are heterogeneous (Shepherd, Laurens, Matheson, Carr, & Green, Reference Shepherd, Laurens, Matheson, Carr and Green2012), just as schizotypy has been associated with inconsistent variation of subcortical volumes and/or cortical thickness (Tonini et al., Reference Tonini, Quidé, Kaur, Whitford and Green2021a). A recent meta-analysis from the Enhancing NeuroImaging Genetics through Meta-Analysis (ENIGMA) Schizotypy working group has identified that higher schizotypy scores, assessed using different questionnaires, were associated with thicker right medial orbitofrontal/ventromedial prefrontal cortex (Kirschner et al., Reference Kirschner, Hodzic-Santor, Antoniades, Nenadic, Kircher, Krug and Modinos2022). In studies which used only the Schizotypal Personality Questionnaire (SPQ) (Raine, Reference Raine1991; Raine & Benishay, Reference Raine and Benishay1995), increasing schizotypy levels have been inconsistently associated with smaller thalamus (Kuhn, Schubert, & Gallinat, Reference Kuhn, Schubert and Gallinat2012) and larger striatum (pallidum/putamen) (Meller, Ettinger, Grant, & Nenadic, Reference Meller, Ettinger, Grant and Nenadic2020), as well as thinner pars orbitalis, rostral middle frontal and parahippocampal gyri (DeRosse et al., Reference DeRosse, Nitzburg, Ikuta, Peters, Malhotra and Szeszko2015) and thicker right dorsolateral prefrontal cortex and dorsal premotor cortex/frontal eye fields (Kuhn et al., Reference Kuhn, Schubert and Gallinat2012).
Exposure to childhood trauma is also associated with brain morphology variation, especially in stress-sensitive limbic regions, such as the hippocampus (Hart & Rubia, Reference Hart and Rubia2012; Lim, Radua, & Rubia, Reference Lim, Radua and Rubia2014; Popovic et al., Reference Popovic, Ruef, Dwyer, Antonucci, Eder, Sanfelici and Consortium2020; Teicher et al., Reference Teicher, Samson, Anderson and Ohashi2016; Teicher & Samson, Reference Teicher and Samson2013). In a study with healthy individuals (Quidé, Tonini, Watkeys, Carr, & Green, Reference Quidé, Tonini, Watkeys, Carr and Green2021), childhood trauma exposure was found to moderate associations between schizotypy and gray matter covariation in a network including striatal and limbic regions. In that study, increased levels of schizotypy were associated with decreased gray matter volume in these regions, only in individuals who reported no childhood trauma exposure, but not for those who were exposed to childhood trauma (Quidé et al., Reference Quidé, Tonini, Watkeys, Carr and Green2021). However, due to the large number of voxels included in whole-brain analyses, identified networks of brain regions largely overstep anatomical boundaries compared to clearly delineated regions-of-interest. It is thus possible that the use of whole-brain analyses may not detect effects specific to individual brain regions.
Using harmonized data pooled from nine individual studies of healthy individuals expressing varying levels of schizotypy, contributing to the ENIGMA Schizotypy working group, we aimed to determine whether associations between schizotypy and indices of subcortical volume and cortical thickness are moderated by exposure to childhood trauma measured along a continuum of severity (ranging from absence to very severe). Analyses were conducted to examine interactions between childhood trauma severity and schizotypy in relation to variation in brain morphology (subcortical volumes, cortical thickness), especially in striatal, orbitofrontal/ventromedial and inferior prefrontal (Derome et al., Reference Derome, Zoller, Modinos, Schaer, Eliez and Debbane2020b; Meller et al., Reference Meller, Ettinger, Grant and Nenadic2020), and stress-sensitive limbic (hippocampus, insula), frontal and striatal (caudate, putamen) regions (Popovic et al., Reference Popovic, Ruef, Dwyer, Antonucci, Eder, Sanfelici and Consortium2020). We hypothesized that morphology of the subcortical (hippocampus, striatum) and cortical (insula, middle frontal gyrus) stress-sensitive regions would be differentially associated with schizotypy in individuals exposed to more severe levels of childhood trauma, compared to those who were not exposed. We specifically expected that more pronounced decreases in volume or thickness would reflect the additive effects of schizotypy and childhood trauma on these regions.
Methods and materials
Cohorts
Participants were 1182 healthy individuals aged between 18 and 65 years [mean age = 34.13 years old, standard deviation (s.d.) = 12.35, N = 647 (55%) females], pooled from nine worldwide cross-sectional cohorts contributing to the ENIGMA Schizotypy working group (see online Supplementary Table S1). Data from each cohort was collected with participants' written informed consent and ethical approval from local institutional review boards.
Schizotypy
Schizotypy was measured using either the 74-items self-report SPQ (Raine, Reference Raine1991) or its brief 22-items version (SPQ-B) (Raine & Benishay, Reference Raine and Benishay1995). Only common items pertaining to the SPQ-B were used to calculate schizotypy indices to maintain consistency of measurement across sites. The total schizotypy score using the 22 items from the SPQ-B was calculated and used for focal analyses (score range: 0–22). Within the present dataset, the average SPQ total score was 3.27 (s.d. = 3.35), ranging from 0 to 21. The distribution of the SPQ scores is presented in online Supplementary Fig. S1.
Childhood trauma
The Childhood Trauma Questionnaire (CTQ) (Bernstein et al., Reference Bernstein, Stein, Newcomb, Walker, Pogge, Ahluvalia and Zule2003) is a self-report measure of retrospective childhood trauma spanning domains of emotional, physical, and sexual abuse, as well as physical and emotional neglect. A score for each domain is calculated from five items on a 5-point Likert scale ranging from 1 (never true) to 5 (very often true). The total CTQ score (combining all types of trauma; score range: 25–125) was used for focal analyses. Within the present dataset, the average CTQ total score was 33.36 (s.d. = 9.03), ranging from 25 to 84. The distribution of the CTQ scores is presented in online Supplementary Figs S2 and S3.
Brain imaging
All sites locally processed 3D T1-weighted structural brain MRI scans using FreeSurfer (Fischl et al., Reference Fischl, Salat, Busa, Albert, Dieterich, Haselgrove and Dale2002, Reference Fischl, van der Kouwe, Destrieux, Halgren, Segonne, Salat and Dale2004). Following established ENIGMA protocols (http://enigma.usc.edu/protocols/imaging-protocols), gray matter volumes were extracted for 16 subcortical regions-of-interest (ROIs) from the automated segmentation (aseg) atlas (left and right lateral ventricle, thalamus, caudate, putamen, pallidum, accumbens, hippocampus, and amygdala) and 68 cortical ROIs from the Desikan-Killiany atlas (Desikan et al., Reference Desikan, Segonne, Fischl, Quinn, Dickerson, Blacker and Killiany2006), as well as total intracranial volume (TIV) and mean cortical thickness (MThickness). Quality assessment was performed at each site prior to analyses, following the ENIGMA Quality assessment protocol (http://enigma.ini.usc.edu/protocols/imaging-protocols). Online Supplementary Table S2 summarizes magnetic resonance imaging scanner manufacturers, models, magnet strengths, acquisition sequences, and the FreeSurfer version used to process the data at each site. Before entering statistical analyses, all neuroimaging variables (all subcortical and cortical ROIs, TIV, MThickness) were adjusted for scanning site using the modified empirical Bayes method ComBat for ENIGMA (Radua et al., Reference Radua, Vieta, Shinohara, Kochunov, Quidé and Green2020), as part of the R package ‘sva’ (Leek et al., Reference Leek, Johnson, Parker, Fertig, Jaffe, Zhang and Torres2019).
Statistical analyses
Focal analyses were performed using R (v4.1.0) (R Core Team, 2021) and RStudio (v1.3.1093) (RStudio Team, 2021). Using a series of multiple linear regressions, the main effects of schizotypy (SPQ total score), childhood trauma severity (CTQ total score) and their interaction (the product of the mean-centered SPQ total score and the mean-centered CTQ total score) on indices of brain morphology in each ROI were separately determined. In the case of significant interactions, moderation analyses were performed using the ‘interactions’ R package (Long, Reference Long2021). The moderation analyses tested the effects of schizotypy as the independent variable at three levels of the moderator: 1 s.d. below the mean CTQ score (low CTQ score), mean CTQ score, and 1 s.d. above the mean CTQ score (high CTQ score) (Cohen, Cohen, West, & Aiken, Reference Cohen, Cohen, West and Aiken2003). In addition to the stress-sensitive regions hypothesized to be associated with the additive effects of schizotypy and trauma (i.e. hippocampus, striatum, insula, and middle frontal gyrus), analyses were performed on all subcortical and cortical ROIs, for sake of completeness. For each set of analyses, only models surviving Bonferroni correction to account for the number of models tested (that is the number of ROIs) were considered (subcortical: p = 0.05/16 = 3.13 × 10−3; cortical: p = 0.05/68 = 7.35 × 10−4). The Davidson-McKinnon correction (HC3) was used to account for potential issues related to heteroskedasticity (Hayes & Cai, Reference Hayes and Cai2007) using the R package ‘sandwich’ (Zeileis, Reference Zeileis2004; Zeileis, Köll, & Graham, Reference Zeileis, Köll and Graham2020). Within each significant model, statistical significance was set at a threshold of p < 0.05. Age, quadratic age (age2, to model non-linear associations with age), sex and TIV (subcortical) or Mthickness (cortical) were entered as covariates in all analyses.
Results
Statistical details of all models are presented in Table 1 for subcortical regions and Table 2 for cortical regions.
Table 1. Results of the moderation analyses for all subcortical ROIs
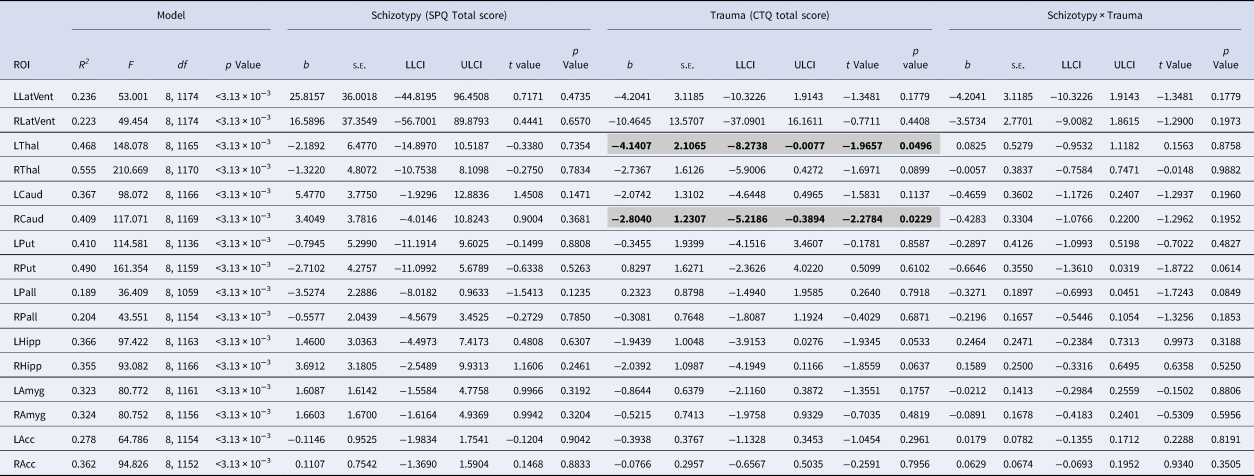
ROI, region of interest; SPQ, schizotypal personality questionnaire; CTQ, childhood trauma questionnaire; LLatVent, left lateral ventricle; RLatVent, right lateral ventricle; LThal, left thalamus; RThal, right thalamus; LCaud, left caudate; RCaud, right caudate; LPut, left putamen; RPut, right putamen; Lpal, left pallidum; RPal, right pallidum; LHipp, left hippocampus; RHipp, right hippocampus; LAmyg, left amygdala; RAmyg, right amygdala; LAcc, left accumbens; RAcc, right accumbens; Adj R 2, adjusted coefficient of determination; s.e., standard error; LLCI, bootstrapped 95% lower levels confidence interval; ULCI, bootstrapped 95% upper levels confidence interval.
Statistically significant associations (p < 0.05 within each model) are in bold and highlighted in gray.
Table 2. Results of the moderation analyses for all cortical ROIs
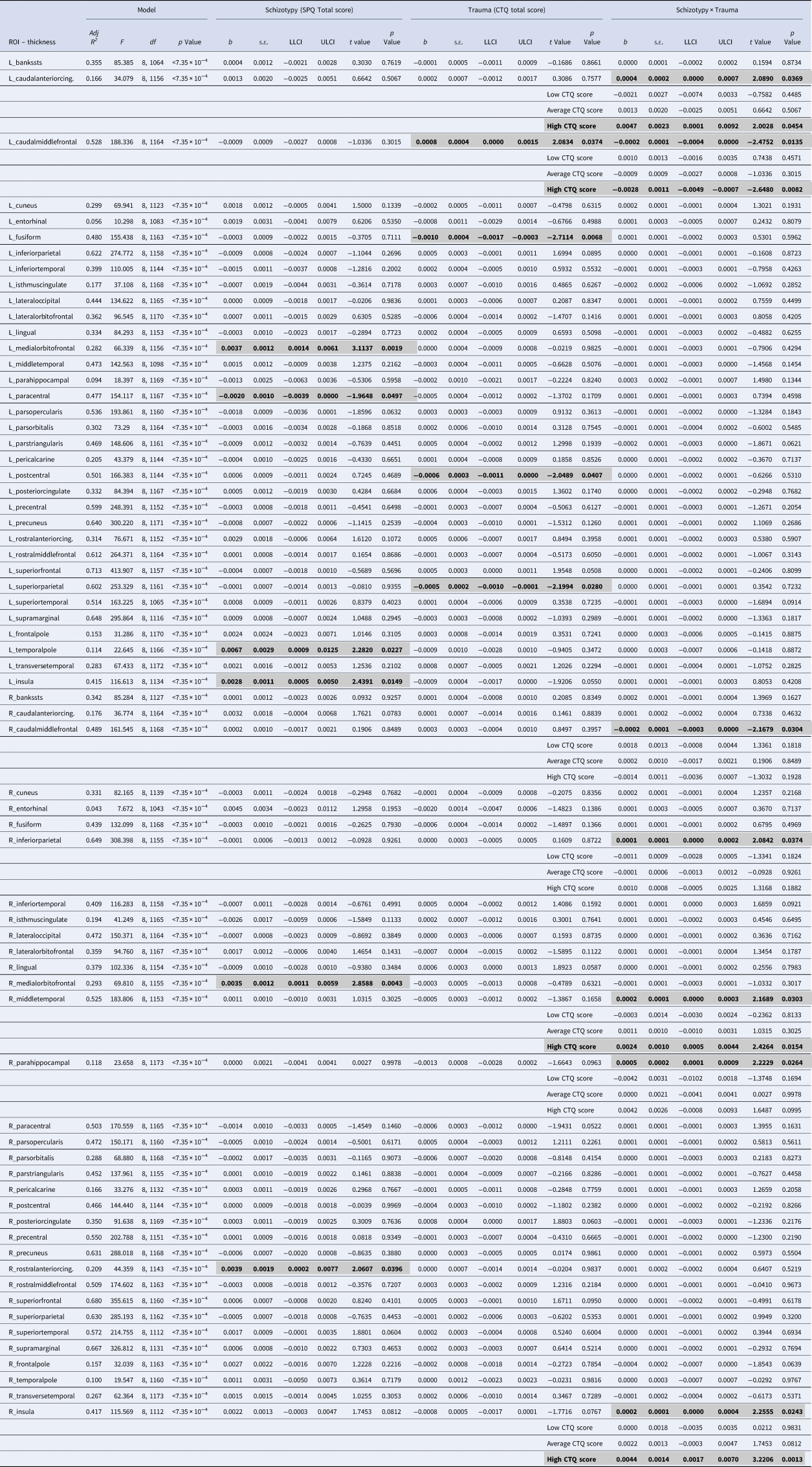
ROI, region of interest; SPQ, schizotypal personality questionnaire; CTQ, childhood trauma questionnaire; Adj R 2, adjusted coefficient of determination; s.e., standard error; LLCI, bootstrapped 95% lower levels confidence interval; ULCI, bootstrapped 95% upper levels confidence interval.
Statistically significant associations (p < 0.05 within each model) are in bold and highlighted in gray
Subcortical volume
All regression models were significant (all p < 3.13 × 10−3; see Table 1). Main effects of trauma were evident in two ROIs, which showed a negative relationship with trauma severity: that is, greater severity of childhood trauma was significantly associated with smaller volumes of the right caudate and left thalamus (see Fig. 1). There were no other significant effects of trauma on other subcortical ROIs, and no significant main effects of schizotypy, or schizotypy-by-trauma interactions.
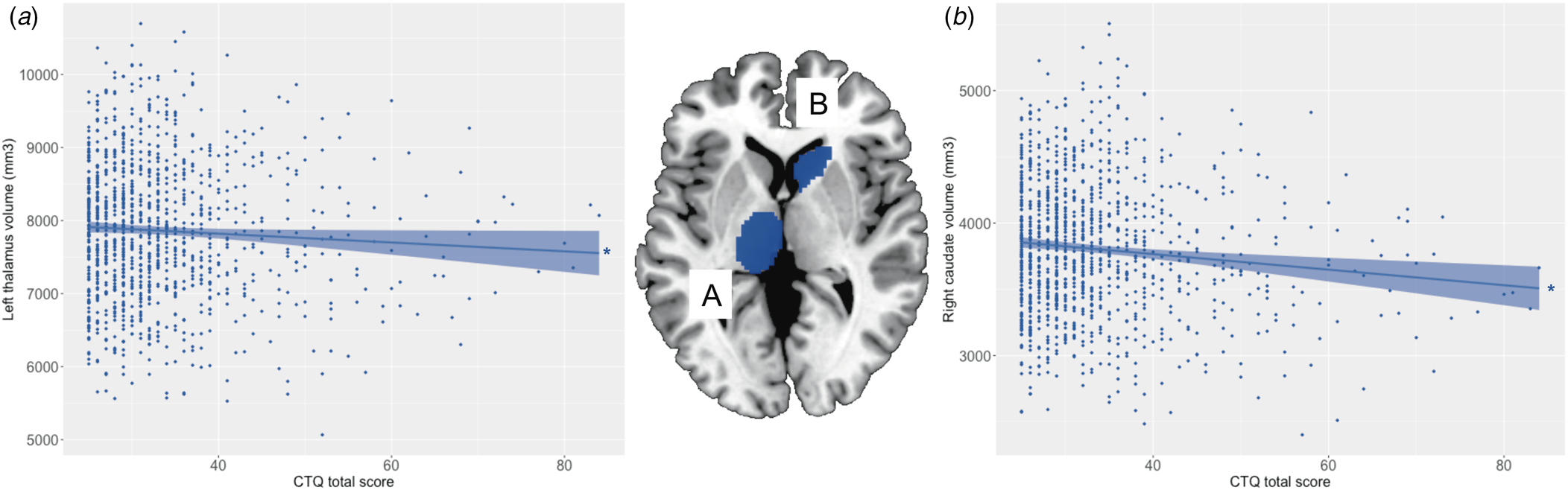
Figure 1. Relationship between severity of childhood trauma exposure and subcortical volumes. The severity of childhood trauma (CTQ total score) was associated with smaller volume of the (a) left thalamus and (b) right caudate. Colored bands represent 95% confidence intervals, blue color indicates negative associations. * p < 0.05.
Cortical thickness
All regression models were significant (all p < 7.35 × 10−4; see Table 2). The interaction between schizotypy and trauma severity was significantly associated with variation in the thickness of the left caudal anterior cingulate gyrus, the left and right caudal middle frontal gyri, the right inferior parietal gyrus, the right middle temporal gyrus, the right parahippocampal gyrus and the right insula. Moderation analyses indicated that higher levels of schizotypy were significantly associated with thicker right middle temporal gyrus, left caudal anterior cingulate gyrus and right insula, as well as thinner left caudal middle frontal gyrus (see Fig. 2), only among individuals exposed to high (but not low or average) trauma severity. Single slope analyses of the other models (right caudal middle frontal, inferior parietal and parahippocampal gyri) were not significant at any level of trauma severity.
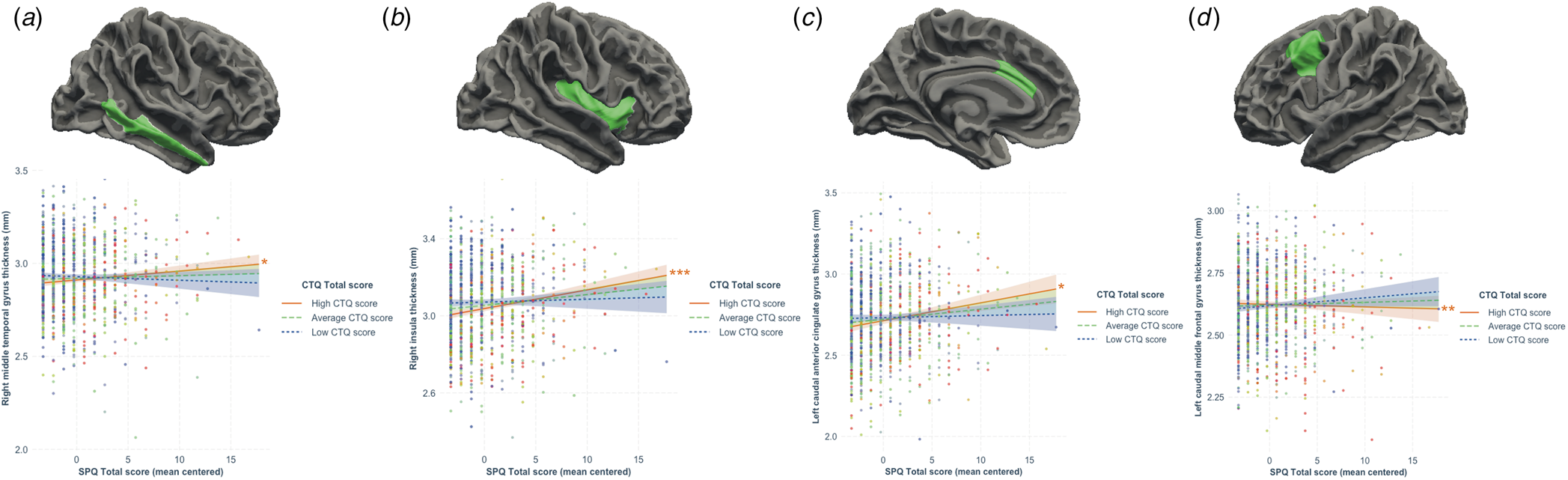
Figure 2. Results from the moderation analyses following significant associations between the schizotypy-by-trauma interaction and cortical thickness. The severity of childhood trauma (CTQ Total score) moderated the relationship between schizotypy (SPQ total score) and thickness of the (a) right middle temporal gyrus, (b) right insula, (c) left caudal anterior cingulate gyrus, and (d) right caudal middle frontal gyrus. Moderation analyses indicated that increasing levels of schizotypy were significantly associated with thicker right middle temporal gyrus, insula and left caudal anterior cingulate gyrus, as well as with thinner left caudal middle frontal gyrus in individuals exposed to higher level (plain red lines), but not average (dashed green lines) or low (dotted blue lines) levels of trauma. Colored bands represent 95% confidence intervals. * p < 0.05; **p < 0.01; ***p < 0.001.
Main effects of schizotypy were also evident for six ROIs with higher levels of schizotypy significantly associated with thicker left and right medial orbitofrontal gyri, left temporal pole, left insula and right rostral anterior cingulate gyrus, as well as thinner left paracentral lobule (see Fig. 3). In addition, main effects of trauma were evident for four cortical ROIs with greater severity of childhood trauma significantly associated with thicker left caudal middle frontal gyrus and thinner left fusiform, left posterior cingulate and left superior parietal gyri (see Fig. 4).
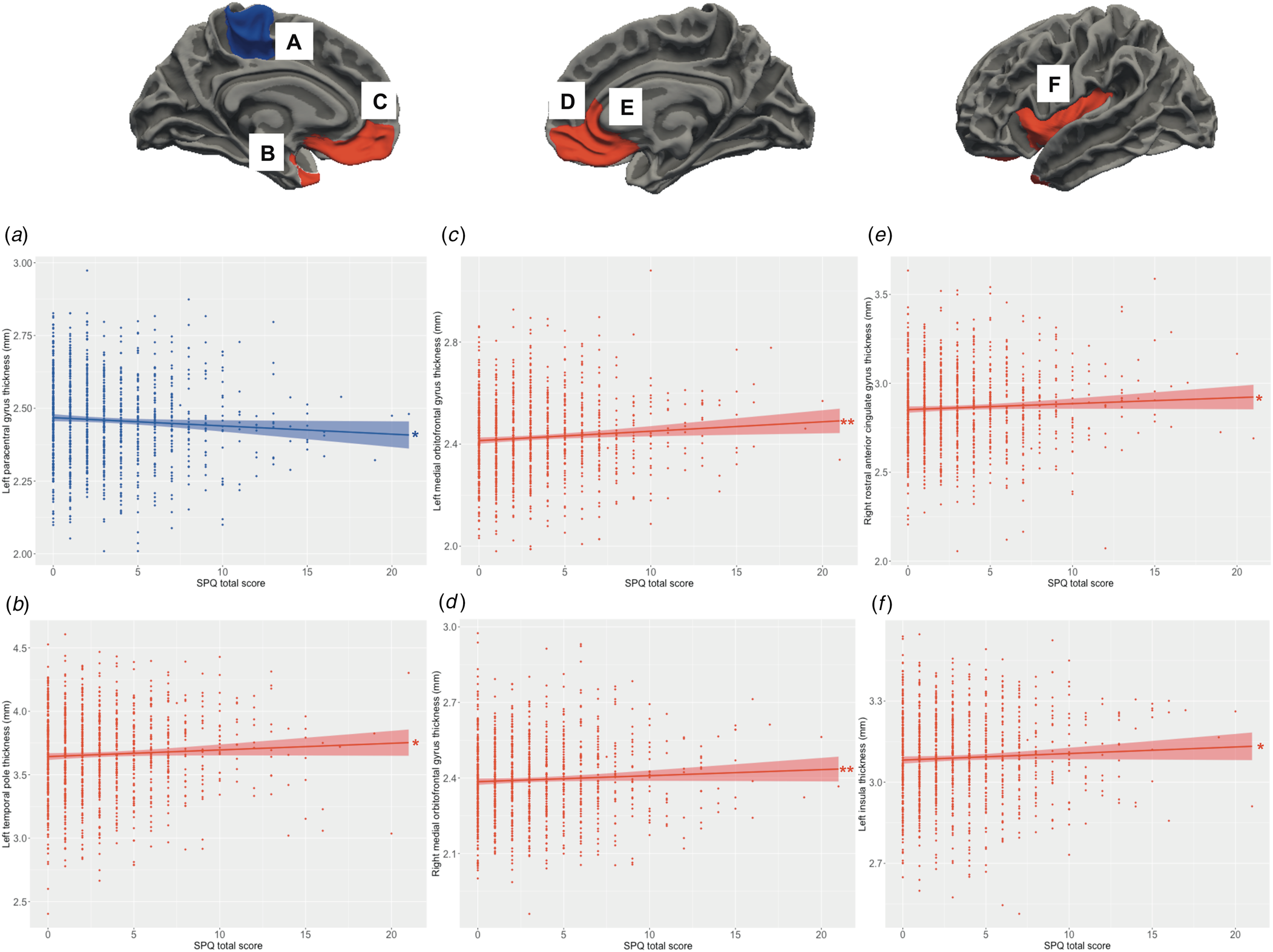
Figure 3. Relationship between schizotypy and cortical thickness. Increasing levels of schizotypy (SPQ total score) were associated with (a) thinner paracentral lobule, (b) thicker temporal pole, (c, d) thicker bilateral medial orbitofrontal gyri, (e) thicker right rostral anterior cingulate gyrus, and (f) thicker left insula. Colored bands represent 95% confidence intervals, blue color indicates negative associations, red color indicates positive associations. *p < 0.05; **p < 0.01.
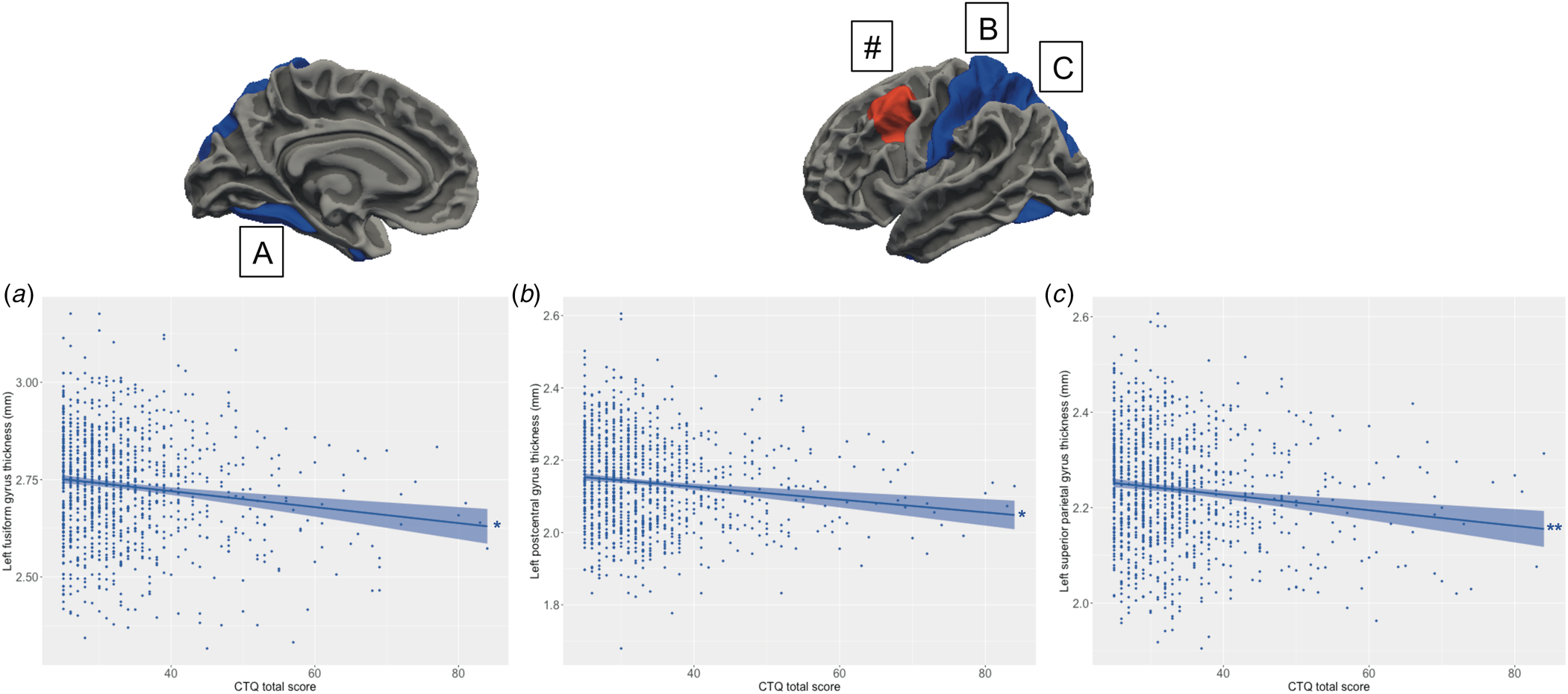
Figure 4. Relationship between the severity of childhood trauma exposure and cortical thickness. Increasing levels of childhood trauma (CTQ total score) were associated with thinner (a) fusiform gyrus, (b) left postcentral gyrus, (c) left superior parietal lobule. (#) The severity of childhood trauma was also associated with thicker left caudal middle frontal gyrus, but this association was confounded by a significant association between the schizotypy-by-trauma interaction and indices of cortical thickness with this region (see Fig. 2). Colored bands represent 95% confidence intervals, blue color indicates negative associations, red color indicates positive associations. * p < 0.05; **p < 0.01.
Discussion
These primary analyses of a large, pooled dataset assembled by the ENIGMA Schizotypy working group revealed that the severity of childhood trauma exposure moderated relationships between schizotypy and brain morphology in psychiatrically healthy adults. In particular, for individuals exposed to greater severity of childhood trauma, higher levels of schizotypy were associated with morphological differences in brain regions critical for higher cognitive processes such as executive functions (thinner caudal middle frontal, thicker caudal anterior cingulate gyri) (Alvarez & Emory, Reference Alvarez and Emory2006), exteroceptive, interoceptive (thicker insula) (Craig, Reference Craig2009) and multimodal integration (visual and auditory cortices, thicker middle temporal gyrus) (Mesulam, Reference Mesulam1998), as well as emotional processing (thicker caudal anterior cingulate gyrus) (Stevens, Hurley, & Taber, Reference Stevens, Hurley and Taber2011). In addition, direct associations were evident between schizotypy and brain morphology, such that higher levels of schizotypy were associated with variation in affective (thicker medial orbitofrontal, rostral anterior cingulate gyri, temporal pole) and somatosensory regions (thinner left paracentral gyrus); direct associations between the severity of childhood trauma and brain morphology were also evident in regions involved in somatosensory processing and integration (smaller thalamus, thinner postcentral gyrus), higher visual and cognitive functions, including reward and social processes (smaller caudate, thinner superior parietal, fusiform, and thicker rostral middle frontal gyri).
This large dataset of psychiatrically healthy individuals revealed alterations in the thickness of cortical regions critical for cognitive and integrative processes in association with schizotypy, when more severe childhood trauma was experienced. These results indicate that the severity of childhood trauma experienced may impact the neurodevelopmental trajectories of these regions in relation to the expression of schizotypy. This is partially consistent with our prediction of additive effects of these two risk factors for psychosis on brain morphology: while decreases in volume or thickness were expected to reflect the deleterious additive effects of schizotypy and trauma on these stress-sensitive regions – which is the case for the left caudal middle frontal gyrus only – results show increased thickness associated with the additive effects of childhood trauma and schizotypy. Increased cortical thickness in multimodal integrative brain regions of people exposed to more severe childhood trauma may reflect a maladaptive integration of external and internal stimuli (Harricharan, McKinnon, & Lanius, Reference Harricharan, McKinnon and Lanius2021), evident in the expression of schizotypy. Indeed, alterations in brain morphology observed in psychiatrically healthy individuals exposed to higher levels of trauma who did not report declared, full-blown psychiatric disorder at time of assessment, could either be associated with the presence of subclinical levels of psychiatric symptoms, or effective adaptation to trauma exposure (Teicher et al., Reference Teicher, Samson, Anderson and Ohashi2016). However, this interpretation is speculative and limited by the cross-sectional nature of the study, as it is possible that some individuals (in particular the younger participants included here) may have developed mental and/or physical health conditions associated with childhood trauma exposure at later stages of their life. In addition, aberrant development of these brain regions in association with schizotypy may reflect the influence of underlying genetic risk and other environmental factors not measured here (Newbury et al., Reference Newbury, Arseneault, Caspi, Moffitt, Odgers, Belsky and Fisher2022; Tonini, Quidé, Whitford, & Green, Reference Tonini, Quidé, Whitford and Green2022; Walter, Fernandez, Snelling, & Barkus, Reference Walter, Fernandez, Snelling and Barkus2016), consistent with results found in clinical high-risk populations (Andreou & Borgwardt, Reference Andreou and Borgwardt2020; de Wit et al., Reference de Wit, Wierenga, Oranje, Ziermans, Schothorst, van Engeland and Durston2016). Future multimodal imaging-genetics studies, at different stages of neural development, are needed to confirm this interpretation.
The brain regions identified here as directly associated with schizotypy are largely consistent with our previous meta-analysis in 3000 individuals (Kirschner et al., Reference Kirschner, Hodzic-Santor, Antoniades, Nenadic, Kircher, Krug and Modinos2022), though not all of the associations reported here were significant in our previous meta-analysis (e.g. insula thickness). This is likely due to methodological differences, as the former study was a meta-analysis of effects across a larger number of cohorts that used various scales to measure schizotypy (i.e. not limited to the SPQ-B, as in the present study). Importantly, there was no overlap between regions associated with schizotypy alone, and those associated with the schizotypy-by-trauma interaction. The regions associated solely with schizotypy are critical for affective integration (Lieberman, Straccia, Meyer, Du, & Tan, Reference Lieberman, Straccia, Meyer, Du and Tan2019), social cognition (Abu-Akel & Shamay-Tsoory, Reference Abu-Akel and Shamay-Tsoory2011), and top-down emotional regulation (Motzkin, Philippi, Wolf, Baskaya, & Koenigs, Reference Motzkin, Philippi, Wolf, Baskaya and Koenigs2015), indicating that schizotypy may represent the long-term consequences of a maladaptive development of the affective brain. This is consistent with prior studies linking affective processes and schizotypy, even in the absence of diagnosed psychiatric conditions (Lewandowski et al., Reference Lewandowski, Barrantes-Vidal, Nelson-Gray, Clancy, Kepley and Kwapil2006). Perhaps more surprisingly, schizotypy was associated with thinner paracentral lobule, a somatosensory region devoted to the representation of the lower limbs and genitals that could reflect movement and/or sexual dysfunctions for those reporting higher levels of schizotypy, as seen in schizophrenia (de Boer, Castelein, Wiersma, Schoevers, & Knegtering, Reference de Boer, Castelein, Wiersma, Schoevers and Knegtering2015; Walther & Strik, Reference Walther and Strik2012). This interpretation remains speculative, and in addition to including other imaging modalities, future studies using scales measuring indices of affective, mood or quality of life (e.g. anxiety, depression, motivation), are necessary to confirm this hypothesis.
Consistent with prior studies (Baker et al., Reference Baker, Williams, Korgaonkar, Cohen, Heaps and Paul2013; Edmiston et al., Reference Edmiston, Wang, Mazure, Guiney, Sinha, Mayes and Blumberg2011; Popovic et al., Reference Popovic, Ruef, Dwyer, Antonucci, Eder, Sanfelici and Consortium2020; Teicher et al., Reference Teicher, Samson, Anderson and Ohashi2016), the present results also suggest that regions critical for somatosensory processes (smaller thalamus, thinner postcentral gyrus) and cognitive functions (smaller caudate, thinner superior parietal lobule, thicker caudal middle frontal gyrus), are sensitive to the severity of childhood trauma exposure. Alterations of the somatosensory pathway may reflect long-term sensitization of this system following childhood trauma exposure, consistent with prior studies (Cassiers et al., Reference Cassiers, Sabbe, Schmaal, Veltman, Penninx and Van Den Eede2018). Smaller caudate may reflect aberrant reward sensitivity (Haber & Knutson, Reference Haber and Knutson2010) that may compromise striatal sensitivity to reward and other positive cues, previously found to be protective of the later development of trauma-related psychopathology (McLaughlin & Lambert, Reference McLaughlin and Lambert2017). Similarly, and consistent with similar previous reports (Everaerd et al., Reference Everaerd, Klumpers, Zwiers, Guadalupe, Franke, van Oostrom and Tendolkar2016), childhood trauma may also have long-term consequences on the development of higher visual/attentional regions, as suggested in the present study by thinner fusiform gyrus and superior parietal lobule, respectively associated with the visual processing of ‘what’ and ‘where’ (Goodale & Westwood, Reference Goodale and Westwood2004). Of note, thickness of the left caudal middle frontal gyrus was associated with both severity of trauma only (increased) and with schizotypy (decreased) when the severity of childhood trauma reported was higher, confirming the additive effects of these risk factors on the development of this critical brain region for higher cognitive functions. There was no other overlap between regions associated with the severity of trauma alone, and those associated with the schizotypy-by-trauma interaction. Unlike the results of a number of prior studies, smaller hippocampal volumes were not directly associated with the severity of trauma exposure (Dannlowski et al., Reference Dannlowski, Stuhrmann, Beutelmann, Zwanzger, Lenzen, Grotegerd and Kugel2012; Teicher et al., Reference Teicher, Samson, Anderson and Ohashi2016); although perhaps surprising, this is consistent with recent meta-analytic results specifically accounting for sex in the context of reported psychopathology (Calem, Bromis, McGuire, Morgan, & Kempton, Reference Calem, Bromis, McGuire, Morgan and Kempton2017), and with a recent study in adult females (Herzog et al., Reference Herzog, Thome, Demirakca, Koppe, Ende, Lis and Schmahl2020). Long-term changes in hippocampal volume are therefore likely to be associated with other factors occurring (e.g. other stressful/traumatic life events) or developing (e.g. state/trait psychopathology) following childhood trauma exposure, rather than with trauma exposure itself. Future studies are warranted to better understand the short and long-term neuroanatomical consequences and dynamic trajectories of trauma exposure when not confounded by other factors (e.g. age, sex, psychopathology, etc.).
Notably, due to large methodological differences, the present results cannot be directly compared to a previous study showing decreased covariation in gray matter volume within a network of subcortical and cortical limbic regions (including the insula) in association with increasing levels of schizotypy, among individuals who reported no exposure to childhood trauma; however, these prior findings deserve mention in relation to the present results (Quidé et al., Reference Quidé, Tonini, Watkeys, Carr and Green2021). While the present study used ROI-based subcortical volumes and cortical thickness derived from FreeSurfer segmentation, the previous study focused on covariation of brain volumetric indices in networks derived from independent component analysis (ICA) performed on whole-brain voxel-based morphometry (VBM) data. In addition, the use of a binary categorical index reflecting exposure/non-exposure to childhood trauma in the previous study meant that the severity of trauma exposure was not investigated, in contrast to the present study where the use of continuous variables is arguably more powerful for moderation analyses. Future studies are warranted that combine continuous independent variables with univariate (VBM) and multivariate (ICA) whole-brain imaging dependent variables, to better understand the role of childhood trauma on schizotypy-related gray matter changes, to be extended to indices of functional and structural connectivity.
This study has important strengths, including harmonized measures of schizotypy, childhood trauma and brain imaging in a large international cohort of medication-naïve healthy participants, but also has several limitations. First, this study used cross-sectional data, which prevented the investigation of developmental changes in brain morphology over time, in relation to schizotypy and exposure to childhood trauma. Age is a critical factor in the measurement of schizotypy: younger people usually present higher levels of schizotypy than older adults, and a peak in the expression of schizotypy is usually observed in adolescence (Debbané, Badoud, Balanzin, & Eliez, Reference Debbané, Badoud, Balanzin and Eliez2013). Different patterns of brain morphology associated with schizotypy may thus be evident at different stages of brain development, as suggested in cohorts of adolescents (Derome et al., Reference Derome, Tonini, Zoller, Schaer, Eliez and Debbané2020a, Reference Derome, Zoller, Modinos, Schaer, Eliez and Debbane2020b). Future longitudinal studies of adolescents and youths transitioning to adulthood are warranted, when levels of schizotypy and risks for first-episode psychosis are higher. Second, the population included in this study was largely comprised of middle-aged adults (average age was 34 years old, ranging from 18 to 65 years old). Although all statistical models included age as a covariate, more subtle effects of schizotypy may be evident at different developmental stages, and closer in time to trauma exposure. Third, this study focused on the overall severity of childhood trauma. For the sake of completeness, the potential unique effects of particular types of childhood trauma (emotional, physical, sexual abuse, or emotional, physical neglect) interacting with schizotypy to influence brain morphology were assessed for the ROIs showing significant main effects of trauma (online Supplementary Table S3) and significant schizotypy-by-trauma interactions (online Supplementary Table S4). These analyses indicated that a number of associations between total trauma severity and cortical thickness may have been underpinned by exposure to physical (direct effect on left fusiform gyrus and interaction with schizotypy on right middle temporal gyrus thickness) and emotional abuse (interactions with schizotypy on left caudal anterior cingulate gyrus and right insula thickness). However perhaps unsurprisingly, as different types of abuse/neglect frequently co-occur (e.g. Gama et al., Reference Gama, Portugal, Goncalves, de Souza Junior, Vilete, Mendlowicz and Pereira2021), the CTQ subscales were moderately correlated to each other (ranging from r = 0.17 to r = 0.64; see Supplementary Fig. S4), such that it is difficult to determine the effects of individual trauma subtypes in this sample. It is also possible that the timing and severity of specific types and combinations of abuse or neglect, during the brain's development, may have different direct and interactive impacts on brain morphology. While a recent study with 643 participants (including people with recent onset psychosis and people at clinical high risk of developing psychosis, amongst others) did not find different patterns of childhood trauma to be associated with differences in brain structure (Haidl et al., Reference Haidl, Hedderich, Rosen, Kaiser, Seves, Lichtenstein and Koutsouleris2023), this needs further scrutiny. Fourth, the retrospective self-report of childhood trauma exposure may have introduced recall biases toward the memory of traumatic events; however, the CTQ shows good reliability even in severely ill psychiatric populations (Fisher et al., Reference Fisher, Craig, Fearon, Morgan, Dazzan, Lappin and Morgan2011), and good similarity with administered interviews (Gayer-Anderson et al., Reference Gayer-Anderson, Reininghaus, Paetzold, Hubbard, Beards, Mondelli and Morgan2020). A recent meta-analysis showed some agreements between prospective and retrospective assessments of childhood trauma (Baldwin, Reuben, Newbury, & Danese, Reference Baldwin, Reuben, Newbury and Danese2019). Another limitation of this study is the relative low severity of childhood trauma exposure (mean CTQ total score = 33.36; range 25–84; online Supplementary Fig. S2) and schizotypy (mean SPQ-B total score = 3.27; range 0–21; online Supplementary Fig. S1) reported by the participants. This indicates that only few participants experienced more severe forms of childhood trauma and schizoptypy, potentially reducing the detection of more subtle effects associated with severe forms of both childhood trauma and schizotypy. The relatively low levels of both self-reported childhood trauma (e.g. 1096 out of 1182 participants reported the lowest possible sexual abuse score; see online Supplementary Fig. S3) and schizotypy in this sample of psychiatrically healthy individuals, warrants future studies with broader score distributions which may identify further relationships between subtypes of trauma, domains of schizotypy and brain development.
In conclusion, this study provides evidence for a moderating role of childhood trauma exposure in the relationship between schizotypy and brain morphology (thickness of middle temporal, insula, caudal anterior cingulate gyrus and caudal middle frontal gyrus) in a large cohort of psychiatrically healthy adults. Future large-scale multimodal imaging (morphological and functional) studies are needed to better understand the functional implications of alterations in brain morphology associated with risk for psychosis in the general population, their developmental timing, and their implications for risk of other mental disorders beyond the schizophrenia spectrum.
Supplementary material
The supplementary material for this article can be found at https://doi.org/10.1017/S0033291723003045
Acknowledgements
The ENIGMA-Schizotypy working group gratefully acknowledges support from the NIH Big Data to Knowledge (BD2K) award (U54 EB020403 to Paul M. Thompson).
FOR2107 – Marburg: This work was funded by the German Research Foundation (DFG grant FOR2107, KI588/14-1 and FOR2107, KI588/14-2 to Tilo Kircher).
FOR2107 – Muenster: This work was funded by the German Research Foundation (DFG, grant FOR2107 DA1151/5-1 and DA1151/5-2 to Udo Dannlowski; SFB-TRR58, Projects C09 and Z02 to Udo Dannlowski) and the Interdisciplinary Centre for Clinical Research (IZKF) of the medical faculty of Münster (grant Dan3/012/17 to Udo Dannlowski).
IGP: The Imaging Genetics in Psychosis (IGP) study was funded by Project Grants from the Australian National Health and Medical Research council (NHMRC; #630471 and #1081603) awarded to Melissa J. Green, and the Macquarie University's Australian Research Council Centre of Excellence in Cognition and its Disorders (CE110001021).
Zurich: This study was financially supported by the Donald C. Cooper-Fonds.
Paris: This study was supported by the French ANR ‘Agence Nationale pour la Recherche’ (ANR MNP VIP and Labex BioPsy).
London: This work was supported by a Brain and Behavior Research Foundation NARSAD Young Investigator Grant (#21200, Lieber Investigator) and a Sir Henry Dale Fellowship jointly funded by the Wellcome Trust and the Royal Society (#202397/Z/16/Z), both awarded to Gemma Modinos.
Roehampton: This work was supported by a British Academy grant awarded to Dr James Gilleen (#SG150457).
Funding statement
Igor Nenadić was supported by German Research Foundation (Deutsche Forschungsgemeinschaft; DFG) grants NE2254/2-1, NE2254/3-1, NE2254/4-1. Tim Hahn was funded by the German Research Foundation (DFG grants HA7070/2-2, HA7070/3, HA7070/4). Emiliana Tonini was supported by the Australian Government Research Training Program (RTP) Scholarship (administered by UNSW Sydney), and a supplementary scholarship administered by Neuroscience Research Australia (NeuRA). Paul M. Thompson received partial grant support from Biogen, Inc., for research unrelated to this manuscript. James Gilleen has received remuneration from Takeda Pharmaceuticals in the past 3 years for consultancy work unrelated to this research.
Competing interest
None.
Ethical standards
The authors assert that all procedures contributing to this work comply with the ethical standards of the relevant national and institutional committees on human experimentation and with the Helsinki Declaration of 1975, as revised in 2008.