Introduction
Millions of people around the world, 74 million in the United States and 24 million people living in the Mexico City Metropolitan Area (MCMA) are involuntarily inhaling ambient concentrations of particulate matter (PM) above safety standards. In the United States, millions of people in busy corridors from New York–New Jersey–Long Island to relatively small cities like Provo, UT are chronically exposed to fine PM year after year (Chen and Kan, Reference Chen and Kan2008). Well-documented air pollution events have been described in United Kingdom including the 1952 London episode with a high impact on public awareness of pollution (Bell et al., Reference Bell, Davis and Fletcher2004; Beelen et al., Reference Beelen, Raaschou-Nielsen, Stafoggia and Andersen2013; Langrish and Mills, Reference Langrish and Mills2014). Cohort longitudinal studies across Europe show 7% increase in natural cause mortality with each 5 μg/m3 in fine PM2.5 (Beelen et al., Reference Beelen, Raaschou-Nielsen, Stafoggia and Andersen2013; Langrish and Mills, Reference Langrish and Mills2014). According to a report by the European Topic Centre on Air and Climate Change (ETC/ACC), fine PM air pollution is associated with 455 000 premature deaths every year in the EU’s 27 member states, including 46 200 in the United Kingdom (ETC/ACC, 2009 report). Air pollution levels have been decreasing in developed countries, while in developing countries and countries in transition, the problem is decreasing at a very low rate or even worsening. MCMA is an example of a polluted megacity with over 40 000 industries, >4 million vehicles that consume more than 40 million liters of petroleum fuels per day and produce thousands of tons of pollutants (INE, 2011). Since MCMA lies in an elevated basin 2240 m above sea level, surrounded on three sides by mountain ridges, surface, as well as surface-based air temperature inversions occur frequently, trapping pollutant emissions close to the surface and aggravating the pollution (Bravo-Alvarez and Torres-Jardón, Reference Bravo-Alvarez and Torres-Jardón2002). MCMA geographical setting and the climatological characteristics along with the relatively little mobility of their residents allows for the opportunity of studying health effects associated to sustained yearlong exposures to concentrations of air pollutants above the current US National Ambient Air Quality Standards.
PM air pollution
Air pollution is a complex mixture of PM, gases, organic and inorganic compounds present in outdoor and indoor air. Urban outdoor pollution is a global public health problem (Molina and Molina, Reference Molina and Molina2004). PM defined by aerodynamic diameter (>2.5 to <10-μm coarse particles, PM10, <2.5-μm fine particles, PM2.5, and <100-nm ultrafine particulate matter (UFPM)) poses a special interest for the brain effects given the capability of fine and UFPM to reach the brain (Block and Calderón-Garcidueñas, Reference Block and Calderón-Garcidueñas2009). The major sources of fine and UFPM in the environment, that is, combustion sources from gas, oil, coal, industry and fires, metals and biological materials are common threat in urban and rural sites (Brook et al., Reference Brook, Rajagopala, Pope, Brook, Bhatnagar, Diez-Roux, Holguin, Hong, Luepker, Mittleman, Peters, Siscovick, Smith, Whitse and Kaufman2010). The smaller the particle, the larger the surface area, the better lung penetration and diffusion and major particle deposition in the respiratory tract and direct translocation into the brain. A significant threat not contemplated by health care workers are man-made particles <100 nm. Nano-sized materials are included in many consumer products and daily use indoor machines produce them in significant amounts, and thus humans are being exposed on daily bases (ie, tooth paste, flavor enhancers, food additives, food and drink containers including baby bottles and pacifiers, laser printers, etc.; Hagens et al., Reference Hagens, Oomen, de Jong, Cassee and Sips2007; Benn et al., Reference Benn, Cavanagh, Hristovski, Posner and Westerhoff2010; Bergin and Witzmann, Reference Bergin and Witzmann2013). Most people spend >90% of their time indoors, so indoor air pollution is very important: smoking, cooking, candle/incense burning, cleaning and use of plastics and conglomerates all contribute to indoor pollution (Habre et al., Reference Habre, Coull, Moshier, Godbold, Nath, Castro, Schachter, Rohr, Kattan, Spengler and Koutrakis2013). Poor air quality in schools and in-vehicle concentrations of pollutants are also of deep concern (Brown et al., Reference Brown, Sarnat and Koutrakis2012; Annesi-Maesano et al., Reference Annesi-Maesano, Baiz, Banerjee, Rudnai and Rive2013).
As in any city, air quality in MCMA is determined by the balance between pollutant emissions and the capacity of the geographical site to eliminate, disperse or concentrate those air pollutants. Despite MCMA rapid growth and development, air quality has improved during the past two decades. Nevertheless, residents remain exposed to concentrations of airborne pollutants exceeding ambient air quality standards, especially for PM and ozone, the two most important pollutants from the standpoint of public health (Brook et al., Reference Brook, Rajagopala, Pope, Brook, Bhatnagar, Diez-Roux, Holguin, Hong, Luepker, Mittleman, Peters, Siscovick, Smith, Whitse and Kaufman2010). The higher socioeconomic status (SES) MCMA population lives toward the south and west of the urban area with access to vegetation, water and better road networks. The industry is located primarily in the northeast and northwest, whereas the east side has been covered by large housing low SES developments in areas of difficult access and poor services. This distribution of the population, as well as the intensity and type of activities carried out, has great influence on the spatial distribution of emissions. According to the most recent emissions inventory for the MCMA (Secretaria de Medio Ambiente, Gobierno de Distrito Federal (SMA-GDF), 2008), the distribution of PM10 depends largely on the activity of vehicles on paved and unpaved streets and roads, and in some urban areas of the northwest, these emissions are added with those generated by heavy industries. The fractional composition of PM2.5 has been dominated by organic and black carbon, sulfate, nitrate, ammonium and crustal components, with site- and time-dependent variations (Aiken et al., Reference Aiken, Salcedo, Cubison, Huffman and DeCarlo2009). Particle species are typically categorized as ‘primary’ if they are emitted in the particle phase and ‘secondary’ if their precursors (volatile organic compounds, NO x , SO2, NH3 and others) are emitted in the gas-phase and subsequent chemical reactions bring them to the particle phase.
Diesel and gasoline exhausts emissions have been responsible for a significant fraction of the fine particle primary emissions in MCMA (Molina et al., Reference Molina, Madronich, Gaffney, Apel, de Foy, Fast, Ferrare, Herndon, Jimenez, Lamb, Osornio-Vargas, Russell, Schauer, Stevens, Volkamer and Zavala2010). Polycyclic aromatic hydrocarbons (PAH) are a family of species, some of which are highly mutagenic and carcinogenic, that are generally associated with black carbon as their emissions are largely from combustion sources (Valle-Hernández et al., Reference Valle-Hernández, Mugica-Alvarez, Salinas-Talavera, Amador-Muñoz, Murillo-Tovar, Villalobos-Pietrini and De Vizcaya-Ruíz2010).
Peak concentrations of PAHs in MCMA are reached during the morning rush hour and are of the order of 120 ngm−3, which is significantly higher than in United States (Marr et al., Reference Marr, Grogan, Wohrnschimmel, Molina, Molina, Smith and Garshick2004; Molina et al., Reference Molina, Madronich, Gaffney, Apel, de Foy, Fast, Ferrare, Herndon, Jimenez, Lamb, Osornio-Vargas, Russell, Schauer, Stevens, Volkamer and Zavala2010). High levels of anthropogenic metals including chromium (Cr), zinc (Zn), copper, lead (Pb), antimony, arsenic, tin and barium complete the toxic potential of fine PM (Molina et al., Reference Molina, Madronich, Gaffney, Apel, de Foy, Fast, Ferrare, Herndon, Jimenez, Lamb, Osornio-Vargas, Russell, Schauer, Stevens, Volkamer and Zavala2010). As expected, these metals exhibit strong temporal variations in concentration and are largely associated with industrial and mobile sources. Elements representing mostly road traffic, that is, Cr, Mn, Zn and Pb, are typically associated with engine emissions and abrasion of tires and brake pads. V and Ni are interpreted as tracers of long-range transport from the use of heavy fuel oil in the north of the basin (Querol et al., Reference Querol, Pey, Minguillón, Pérez, Alastuey, Viana, Moreno, Bernabé, Blanco, Cárdenas, Vega, Sosa, Escalona, Ruiz and Artiñano2008). Pb deserves a special mention. Before 1986, Pb was probably the most harmful pollutant in MCMA, associated with the exclusive use of leaded gasoline and resulting in Pb concentrations three times the air quality standard. In response to a very strong social pressure and to a growing international trend to control car emissions with catalytic converters, PEMEX, the federal Mexican oil company, was forced to reduce the gasoline content of tetra-ethyl-lead (Bravo-Alvarez and Torres-Jardón, Reference Bravo-Alvarez and Torres-Jardón2002) resulting in Pb concentrations∼2 μg/m3 in 1988 and reaching ∼0.5 μg/m3 by 1998. Although Pb is no longer an air pollutant problem, lipopolysaccharides (products of the outer membrane of Gram-negative bacteria) associated with PM, are detected in very high concentrations in southern Mexico City (Rosas-Pérez et al., Reference Rosas-Pérez, Serrano, Alfaro-Moreno, Baumgardner, Garcia-Cuellar, Martin del Campo, Raga, Castillejos, Colin and Osornio-Vargas2007). The grim scenario for MCMA residents is a sustained exposure to PM2.5 several hours per day above the current standards, every year, in such a way that residents are living in a very effective exposure chamber from conception to death. Is this scenario exclusive for Mexico City residents? The answer is no, Salt Lake City, Tacoma, San Francisco Bay, Los Angeles, Fairbanks, London, Greater Manchester and Oxfordshire residents to name a few have similar scenarios, albeit less severe (Bell et al., Reference Bell, Goldberg, Hogrefe, Kinney, Knowlton, Lynn, Rosenthal, Rosenzweig and Patz2007; Maheswarang et al., Reference Maheswarang, Pearson, Smeeton, Beevers, Campbell and Wolfe2010; Williams et al., Reference Williams, Ulrich, Larson, Wener, Wood, Chen-Levy, Campbell, Potter, McTiernan and Roos2011; Beelen et al., Reference Beelen, Raaschou-Nielsen, Stafoggia and Andersen2013; UK Air Data). On the other hand, millions of Shanghai residents are covered with heavy haze substantially increasing cardiovascular, respiratory and cerebrovascular morbidity and mortality (Xu et al., Reference Xu and Ye2013).
The impact of polluted air on an adult brain
The first important statement to be made is that age and disease status are key factors on the impact of air pollutants. Associations between stroke, ambient pollution and coal fumes were suggested in the 1980s (Knox, Reference Knox1981; Zhang et al., Reference Zhang, Yu and Zhou1988). Cigarette smoking as a stroke risk factor (and of course an excellent source of large amounts of PM) was published a decade later (Howard et al., Reference Howard, Wagenknecht, Cai, Cooper, Kraut and Toole1998). Ischemic stroke mortality and transient ischemic attacks relate to fine and UFPM exposures even at concentrations below the current standards (Hong et al., Reference Hong, Lee, Kim and Kwon2002; Kettunen et al., Reference Kettunen, Lanki, Tiittanen, Aaalto, Koskentalo, Kulmala, Salomaa and Pekkanen2007; Lisabeth et al., Reference Lisabeth, Escobar, Dvonch, Sanchez, Majersik, Brown, Smith and Morgenstern2008; Bedada et al., Reference Bedada, Smith, Tyrrell, Hirst and Agius2012; Leiva et al., Reference Leiva, Santibañez, Ibarra, Matus and Seguel2013) and very important from the clinical point of view, outdoor air pollution and proximity to high-traffic roadways impact stroke survival (Maheswarang et al., Reference Maheswarang, Pearson, Smeeton, Beevers, Campbell and Wolfe2010; Wilker et al., Reference Wilker, Mostofsky, Lue, Gold, Schwartz, Wellenius and Mittleman2013). The largest association between PM2.5 and ischemic stroke risk was seen with stroke due to large-artery atherosclerosis and small-vessel occlusion: diabetic patients are particularly at risk (O’Donnell et al., Reference O’Donnell, Fang, Mittleman, Kapral and Wellenius2011). Moreover, the associations involve not only urban pollutants but also dust storms and unfortunate events like 9/11 World Trade Center (Yang et al., Reference Yang, Chen, Chiu and Goggins2005; Brackbill et al., Reference Brackbill, Thorpe, DiGrande, Perrin, Sapp, Wu, Campolucci, Walker, Cone, Pulliam, Thalji, Farfel and Thomas2006). Although most of the literature associating stroke with air pollution emphasizes PM, ozone – a key photochemical pollutant affecting our populations in Mexico City, Los Angeles, San Joaquin Valley, Riverside, Sacramento, Baltimore, Dallas, south-east of England, etc. – is also a big player (Parrish, et al., Reference Parrish, Singh, Molina and Madronich2011; Carlsen et al., Reference Carlsen, Forsberg, Meister, Gislason and Oudin2013; Suissa et al., Reference Suissa, Fortier, Lachaud, Staccini and Mahagne2013). Depression and mood disorders have been associated with air pollution. Increases in PM10, PM2.5, NO2, CO, SO2, O3 and biomass burning are associated with depression in the elderly, and emotional symptoms and suicide attempts in younger subjects (Szyszkowicz et al., Reference Szyszkowicz, Willey, Grafstein, Rowe and Colman2010; Wisnivesky et al., Reference Wisnivesky, Teitelbaum, Todd, Boffetta, Crane, Crowley, de la Hoz, Dellenbaugh, Harrison, Herbert, Kim, Jeon, Kaplan, Katz, Levin, Luft, Markowitz, Moline, Ozbay, Pietrzak, Shapiro, Sharma, Skloot, Southwick, Stevenson, Udasin, Wallenstein and Landrigan2011; Banerjee et al., Reference Banerjee, Siddique, Dutta, Mukherjee and Ray2012; Lim et al., Reference Lim, Kim, Kim, Bae, Park and Hong2012). Exposure of animal models to ambient PM2.5 results in depressive responses and increase hippocampal pro-inflammatory cytokines (Fonken et al., Reference Fonken, Xu, Weil, Chen, Sun, Rajagopalan and Nelson2011), whereas prenatal nano-PM impacts neonatal neurons and adult behavior in mice (Davis et al., Reference Davis, Bortolato, Godar, Sander, Iwata, Pakbin, Shib, Berhane, McConnell, Sioutas, Finch, Morgan and Block2013).
The impact of polluted air on a developing brain
Clean air is fundamental for children’s health and well-being. Millions of children are showing an array of adverse short- and long-term health outcomes related to air pollution exposures. Widely recognized among pediatric health providers is the impact of intrauterine factors, parent–child interactions, cognitive stimulation, maternal SES during pregnancy and the child’s nutrition and exposure to complex learning stimuli, all vital for brain development (Calderón-Garcidueñas and Torres-Jardón, Reference Calderón-Garcidueñas and Torres-Jardón2012a). However, air pollution brain effects rooted in intrauterine life and childhood are not generally acknowledged. Air pollution is not broadly recognized in the context of children’s brain effects including the presence of neuroinflammation, cognitive deficits, structural brain alterations and neuropathological hallmarks of Alzheimer (AD) and Parkinson’s diseases (PD) (Calderón-Garcidueñas et al., Reference Calderón-Garcidueñas, Mora-Tiscareño, Fordham, Valencia-Salazar, Chung, Rodriguez-Alcaraz, Paredes, Variakojis, Villarreal-Calderón, Flores-Camacho, Antunez-Solis, Henríquez-Roldán and Hazucha2003; 2008Reference Calderón-Garcidueñas, Mora-Tiscareño, Ontiveros, Gómez-Garza, Barragán-Mejía, Broadway, Chapman, Valencia-Salazar, Jewells, Maronpot, Henríquez-Roldán, Pérez-Guillé, Torres-Jardón, Herrit, Brooks, Osnaya-Brizuela, Monroy, González-Maciel, Reynoso-Robles, Villarreal-Calderon, Solt and Englea; 2008Reference Calderón-Garcidueñas, Villarreal-Calderon, Valencia-Salazar, Henríquez-Roldán, Gutiérrez-Castrellón, Torres-Jardón, Osnaya-Brizuela, Romero, Torres-Jardón, Solt and Reedb; 2008Reference Calderón-Garcidueñas, Solt, Franco-Lira, Torres-Jardón, Nuse, Herritt, Villarreal-Calderón, Osnaya, Stone, García, Brooks, González-Maciel, Reynoso-Robles, Delgado-Chávez and Reedc; Reference Calderón-Garcidueñas, Macias-Parra, Hoffmann, Valencia-Salazar, Henríquez-Roldán, Monte, Barragán-Mejía, Villarreal-Calderon, Romero, Granada-Macías, Torres-Jardón, Medina-Cortina and Maronpot2009; Reference Calderón-Garcidueñas, Franco-Lira, Henríquez-Roldán, González-Maciel, Reynoso-Robles, Villarreal-Calderon, Herritt, Brooks, Keefe, Palacios-Moreno, Torres-Jardón, Medina-Cortina, Delgado-Chávez, Aiello-Mora, Maronpot and Doty2010; 2011a; 2011Reference Calderón-Garcidueñas, Engle, Mora-Tiscareño, Styner, Gomez-Garza, Zhu, Jewells, Torres-Jardón, Romero, Monroy-Acosta, González-González, Medina-Cortina and D'Angiullib; 2012Reference Calderón-Garcidueñas and Torres-Jardóna; 2012b; 2012Reference Calderón-Garcidueñas, Mora-Tiscareño, Styner, Gómez-Garza, Zhu, Torres-Jardón, Carlos, Solorio-López, Medina-Cortina, Kavanaugh and D’Angiullic; 2013a; 2013Reference Calderón-Garcidueñas, Cross, Franco-Lira, Aragón-Flores, Kavanaugh, Torres-Jardón, Chao, Thompson, Chang, Zhu and D’Angiullib).
A coherent pathway linking exposure to air pollution and brain damage starts with a chronic inflammatory process involving the respiratory upper and lower tracts, which result in a systemic inflammatory response with the production of inflammatory mediators capable of reaching the brain (Calderón-Garcidueñas et al., Reference Calderón-Garcidueñas, Mora-Tiscareño, Fordham, Valencia-Salazar, Chung, Rodriguez-Alcaraz, Paredes, Variakojis, Villarreal-Calderón, Flores-Camacho, Antunez-Solis, Henríquez-Roldán and Hazucha2003; Reference Ou, Hedley, Chung, Thach, Chau, Chan, Yang, Ho, Wong and Lam2008 Reference Calderón-Garcidueñas, Mora-Tiscareño, Ontiveros, Gómez-Garza, Barragán-Mejía, Broadway, Chapman, Valencia-Salazar, Jewells, Maronpot, Henríquez-Roldán, Pérez-Guillé, Torres-Jardón, Herrit, Brooks, Osnaya-Brizuela, Monroy, González-Maciel, Reynoso-Robles, Villarreal-Calderon, Solt and Englea; 2008Reference Calderón-Garcidueñas, Villarreal-Calderon, Valencia-Salazar, Henríquez-Roldán, Gutiérrez-Castrellón, Torres-Jardón, Osnaya-Brizuela, Romero, Torres-Jardón, Solt and Reedb; 2008Reference Calderón-Garcidueñas, Solt, Franco-Lira, Torres-Jardón, Nuse, Herritt, Villarreal-Calderón, Osnaya, Stone, García, Brooks, González-Maciel, Reynoso-Robles, Delgado-Chávez and Reedc; Reference Calderón-Garcidueñas, Macias-Parra, Hoffmann, Valencia-Salazar, Henríquez-Roldán, Monte, Barragán-Mejía, Villarreal-Calderon, Romero, Granada-Macías, Torres-Jardón, Medina-Cortina and Maronpot2009; 2011Reference Calderón-Garcidueñas, D’Angiulli, Kulesza, Torres-Jardón, Osnaya, Romero, Keefe, Herritt, Brooks, Avila-Ramirez, Delgado-Chávez, Medina-Cortina and González-Gonzáleza; 2011Reference Calderón-Garcidueñas, Engle, Mora-Tiscareño, Styner, Gomez-Garza, Zhu, Jewells, Torres-Jardón, Romero, Monroy-Acosta, González-González, Medina-Cortina and D'Angiullib; 2012Reference Calderón-Garcidueñas and Torres-Jardóna; 2012Reference Calderón-Garcidueñas, Kavanaugh, Block, D’Angiulli, Delgado-Chávez, Torres-Jardón, González-Maciel, Reynoso-Robles, Osnaya, Villarreal-Calderon, Guo, Hua, Zhu, Perry and Diazb; 2013Reference Calderón-Garcidueñas, Franco-Lira, Mora-Tiscareño, Medina-Cortina, Torres-Jardón and Kavanaugha; 2013Reference Calderón-Garcidueñas, Cross, Franco-Lira, Aragón-Flores, Kavanaugh, Torres-Jardón, Chao, Thompson, Chang, Zhu and D’Angiullib). Continuous expression of potent inflammatory mediators in the central nervous system (CNS) and the formation of reactive oxygen species are major findings in urban residents (Block and Calderón-Garcidueñas, Reference Block and Calderón-Garcidueñas2009). UFPM, particulate matter-associated lipopolysaccharides, and metal uptake could take place through olfactory neurons, cranial nerves such as the trigeminal and vagus, the systemic circulation and macrophage-like cells loaded with PM from the lungs (Calderón-Garcidueñas et al., Reference Calderón-Garcidueñas, Solt, Franco-Lira, Torres-Jardón, Nuse, Herritt, Villarreal-Calderón, Osnaya, Stone, García, Brooks, González-Maciel, Reynoso-Robles, Delgado-Chávez and Reed2008c; 2012Reference Calderón-Garcidueñas and Torres-Jardóna). Activation of the brain innate immune responses resulting from the interaction between circulating cytokines and constitutively expressed cytokine receptors located in brain endothelial cells is followed by activation of cells involved in adaptive immunity (Lampron et al., Reference Lampron, Elali and Rivest2013). Monocytes are the main innate immune response mediator cells, producing and secreting TNFα, interleukin-1β (IL-1β) and IL-6, which in turn recruit and increase the activity of other immune cells. Fine and UFPM could serve as the crucial trigger for a chain of events leading to endothelial activation, disruption of blood–brain barrier (BBB), altered response of the innate immune system, production of autoantibodies to cell junction and neural proteins, neuroinflammation and neurodegeneration. These early changes, amenable to intervention and viewed initially as a compensatory phenomenon by some groups including ours (Perry et al., Reference Perry, Nunomura, Hirai, Zhu, Pérez, Avila, Castellani, Atwood, Aliev, Sayre, Takeda and Smith2002; Castellani et al., Reference Castellani, Zhu, Zhu, Moreira, Perry and Smith2006; Calderón-Garcidueñas et al., 2012Reference Calderón-Garcidueñas and Torres-Jardóna; Reference Calderón-Garcidueñas, Franco-Lira, Mora-Tiscareño, Medina-Cortina, Torres-Jardón and Kavanaugh2013a) are likely critical to onset and progression of the neurodegenerative changes and the full clinical picture seen in AD patients (Castellani et al., Reference Castellani, Zhu, Zhu, Moreira, Perry and Smith2006).
There is growing recognition of the role of systemic and neural inflammation and the interplay between immunity, neurodegeneration and maladaptive activation of innate/adaptive immunity as key pathogenic phenomenon in AD (Blasko et al., Reference Blasko, Stampfer-Kountchev, Robatscher, Veerhuis, Eikelenboom and Grubeck-Loebenstein2004; Dik et al., Reference Dik, Jonker, Hack, Smit, Comijs and Eikelenboom2005; Finch and Morgan, Reference Finch and Morgan2007; Bonotis et al., Reference Bonotis, Krikki, Holeva, Aggouridaki, Costa and Baloyannis2008; Gomez-Ravetti and Moscato, Reference Gomez-Ravetti and Moscato2008; Cunningham et al., Reference Cunningham, Campion, Lunnon, Murray, Woods, Deacon, Rawlins and Perry2009; Keene et al., Reference Keene, Cudaback, Li, Montine and Montine2011). Likewise, change in immune status has been suggested as a plausible biological mechanism by which PM could cause adverse health effects (Eikelenboom et al., Reference Eikelenboom, van Exel, Veerhuis, Rozemuller, van Gool and Hoozemans2011). PM has the capability of crossing barriers, including the BBB resulting in neuroinflammation and intrinsically disordered neural proteins associated with neurodegenerative diseases (Campbell, Reference Campbell2004; Win-Shwe et al., Reference Win-Shwe, Yamamoto, Fujitani, Hirano and Fujimaki2008; Levesque et al., Reference Levesque, Surace, McDonald and Block2011).
The emerging picture reveals highly exposed urban children exhibit significant neuroinflammation and brain oxidative stress (Calderón-G arcidueñas et al., Reference Calderón-Garcidueñas, Solt, Franco-Lira, Torres-Jardón, Nuse, Herritt, Villarreal-Calderón, Osnaya, Stone, García, Brooks, González-Maciel, Reynoso-Robles, Delgado-Chávez and Reed2008c; 2012Reference Calderón-Garcidueñas and Torres-Jardóna; Reference Calderón-Garcidueñas, Franco-Lira, Mora-Tiscareño, Medina-Cortina, Torres-Jardón and Kavanaugh2013a; 2013Reference Calderón-Garcidueñas, Cross, Franco-Lira, Aragón-Flores, Kavanaugh, Torres-Jardón, Chao, Thompson, Chang, Zhu and D’Angiullib). In addition, these children have extensive abnormal white matter blood vessels, perivascular inflammation and a breakdown of the BBB (Calderón-Garcidueñas et al., Reference Calderón-Garcidueñas, Solt, Franco-Lira, Torres-Jardón, Nuse, Herritt, Villarreal-Calderón, Osnaya, Stone, García, Brooks, González-Maciel, Reynoso-Robles, Delgado-Chávez and Reed2008c). Fine tuning of immune-to-brain communication is crucial to neural networks appropriate functioning, thus our recent finding of autoantibodies to actin and occludin/zonulin in the presence of BBB compromise might represent one more factor contributing to initiation and/or pathogenesis of neurodegenerative changes. Current data support a role for air pollution in CNS damage and urban children shared mechanistic pathways potentially conducting to AD and PD (Calderón-Garcidueñas et al., Reference Calderón-Garcidueñas, Franco-Lira, Mora-Tiscareño, Medina-Cortina, Torres-Jardón and Kavanaugh2013a).
The short- and long-term effects of air pollution upon the CNS
We have discussed plausible mechanistic pathways causing CNS effects associated with sustained exposures of ambient pollutants during a lifetime, however, it should be clear that acute, subchronic or chronic exposures to air pollutants all have detrimental CNS effects. An example of acute and subchronic massive exposures was the World Trade Center 9/11-related environmental disaster (Bills et al., Reference Bills, Levy, Sharma, Charney, Herbert, Moline and Katz2008; Jordan et al., Reference Jordan, Stellman, Morabia, Miller-Archie, Alper, Laskaris, Brackbill and Cone2013; Ozbay et al., Reference Ozbay, Aud der Heyde, Reissman and Sharma2013). Massive exposure to a complex mixture of inhalable fine PM, nanoparticles (NPs) and toxic chemicals, resulted in persistent mental detrimental effects and evolution toward unknown brain health outcomes beyond posttraumatic stress disorder (Bills et al., Reference Bills, Levy, Sharma, Charney, Herbert, Moline and Katz2008; Jordan et al., Reference Jordan, Stellman, Morabia, Miller-Archie, Alper, Laskaris, Brackbill and Cone2013; Ozbay et al., Reference Ozbay, Aud der Heyde, Reissman and Sharma2013).
The olfactory bulb (OB) pathology needs special attention because large segments of the world population inhale toxic substances on daily basis that have the potential for harming the olfactory system and penetrating the brain via the olfactory epithelium (Calderón-Garcidueñas et al., Reference Calderón-Garcidueñas, Franco-Lira, Henríquez-Roldán, González-Maciel, Reynoso-Robles, Villarreal-Calderon, Herritt, Brooks, Keefe, Palacios-Moreno, Torres-Jardón, Medina-Cortina, Delgado-Chávez, Aiello-Mora, Maronpot and Doty2010). The issue is very important in the context of air pollution because olfactory dysfunction is among the earliest ‘pre-clinical’ features of AD and PD, occurring in ~90% of early onset cases (Wang et al., Reference Wang, Eslinger, Doty, Zimmerman, Grunfeld, Sun, Meadowcroft, Connor, Price, Smith and Yang2010; Doty, Reference Doty2012). Early olfactory deficits in MCMA young residents appear to be associated with the presence of β-amyloid, α- synuclein, PM in glomerular structures and the massive distortion of the OB organization (Calderón-Garcidueñas et al., Reference Calderón-Garcidueñas, Franco-Lira, Henríquez-Roldán, González-Maciel, Reynoso-Robles, Villarreal-Calderon, Herritt, Brooks, Keefe, Palacios-Moreno, Torres-Jardón, Medina-Cortina, Delgado-Chávez, Aiello-Mora, Maronpot and Doty2010). The central delayed brainstem auditory evoked potentials (BAEPs), auditory impairment and vestibular dysfunction in exposed children is likely related to the extensive brainstem inflammation with accumulation of β-amyloid and α-synuclein in key auditory and vestibular nuclei (Calderón-Garcidueñas et al., Reference Calderón-Garcidueñas, D’Angiulli, Kulesza, Torres-Jardón, Osnaya, Romero, Keefe, Herritt, Brooks, Avila-Ramirez, Delgado-Chávez, Medina-Cortina and González-González2011). Neurodegenerative changes in the dorsal motor nucleus of the vagus, the nucleus of the solitary tract, arcuate nucleus, raphe midline, and extra-raphe medial and lateral tegmental neurons (Calderón-Garcidueñas et al., Reference Calderón-Garcidueñas, D’Angiulli, Kulesza, Torres-Jardón, Osnaya, Romero, Keefe, Herritt, Brooks, Avila-Ramirez, Delgado-Chávez, Medina-Cortina and González-González2011) are similar to the PD stages I and II of Braak et al. (Reference Braak, Ghebremedhin, Rüb, Bratzke and Del Tredeci2004). The brainstem pathology we are observing in highly exposed children has strong links with both AD and PD (Braak et al., Reference Braak, Ghebremedhin, Rüb, Bratzke and Del Tredeci2004; Reference Braak, Thal, Ghebremedhin and Del Tredeci2011).
Recent studies have reported associations between prenatal and perinatal exposures to air pollutants and autism spectrum disorder (ASD) in children (van den Hazel et al., Reference van den Hazel, Zuurbier, Babisch, Bartonova, Bistrup, Bolte, Busby, Butter, Ceccatelli, Fucic, Hanke, Johansson, Kohlhuber, Leijs, Lundqvist, Moshammer, Naginiene, Preece, Ronchetti, Salines, Saunders, Schoeters, Stilianakis, ten Tusscher and Koppe2006; Larsson et al., Reference Larsson, Weiss, Janson, Sundell and Bornehag2009; Herbert, Reference Herbert2010; Volk et al., Reference Volk, Hertz-Picciotto, Delwiche, Lurmann and McConnell2011; Reference Volk, Lurmann, Penfold, Hertz-Picciotto and McConnell2013; Reference Volk, Kerin, Lurmann, Hertz-Picciotto, McConnell and Campbell2014; Becerra et al., Reference Becerra, Wilhelm, Olsen, Cockburn and Ritz2013; Roberts et al., Reference Roberts, Lyall, Hart, Laden, Just, Bobb, Koenen, Ascherio and Weisskoof2013; Visser et al., Reference Visser, Rommelse, Vink, Schrieken, Oosterling, van der Gaag and Buitelaar2013). ASD is likely caused by complex interactions between genetic and environmental factors. ASD is associated to air pollution exposures during pregnancy in US cities with significant air pollution from traffic sources (Becerra et al., Reference Becerra, Wilhelm, Olsen, Cockburn and Ritz2013). Activation of the maternal immune system during pregnancy and abnormal behavioral development in Rhesus monkey offspring brings up key questions regarding the role of air pollution and autism among vulnerable populations (Bauman et al., Reference Bauman, Iosif, Smith, Bregere, Amaral and Patterson2013). To complicate matters for clinicians in real-world settings, the changes in the diagnostic criteria for autism in DSM-5 is causing significant controversies and concerns among health providers without formal training in research instruments and complicated assessment processes (Hazen et al., Reference Hazen, McDougle and Volkmar2013).
Reflections from practice
This paper is the product of discussions among the authors, all of us familiar with MCMA air pollution. We are physicians, pathologists, neuropathologists, auditory system experts, toxicologists, psychologists and atmospheric researchers dealing with children, worried parents and school officials, looking at neurodegenerative pathologies in autopsy materials from seemingly healthy children dying in accidents and having significant trouble in explaining parents plausible biological pathways that will solve all their questions. Several concerns have been raised in this short review about the influence of air pollutants upon the adult and the developing brain that involve a wide spectrum of pathologies the clinician should take into account for short- and long-term CNS impact.
In adults, it is imperative to take care of chronic diseases that aggravate vascular, metabolic and structural brain changes, while advising the patient about tobacco and cigarette smoking and harmful occupations and hobbies. In children, the cognitive, auditory and vestibular effects have immediate early school negative effects, while the prefrontal lesions and the diffuse cortical neuroinflammation will be reflected in decreased career opportunities, negative social health outcomes, including increases in delinquent or criminal activity and violence and consequently a major negative impact on the economy in which those individuals reside. An issue everybody ignores is that the teens reduced capacity to block impulsive antisocial behavior that accompanies impaired fluid cognition is having a significant impact on society.
Next, we review more in detail the specific air pollution effects of brain impairment on cognition and behavior that support the practical reflections presented in this paper. Table 1 summarizes in schematic form selected studies examining neurocognitive/neurophysiological effects of air pollution in children, adolescents and young adult populations. The table shows the populations and the air pollutants studied, the tests and deficits found, other tests used and the city/cities/country where the study took place. In what follows we will be focusing mainly on the available findings concerning MCMA children and mechanistic studies on brain development in animal research literature.
Table 1 Selected studies examining neurocognitive/neurophysiological effects of air pollution in children, adolescents and young adult populations. The table shows the populations and the air pollutants studied, the tests and deficits found, other tests used and the city/cities/country where the study took place
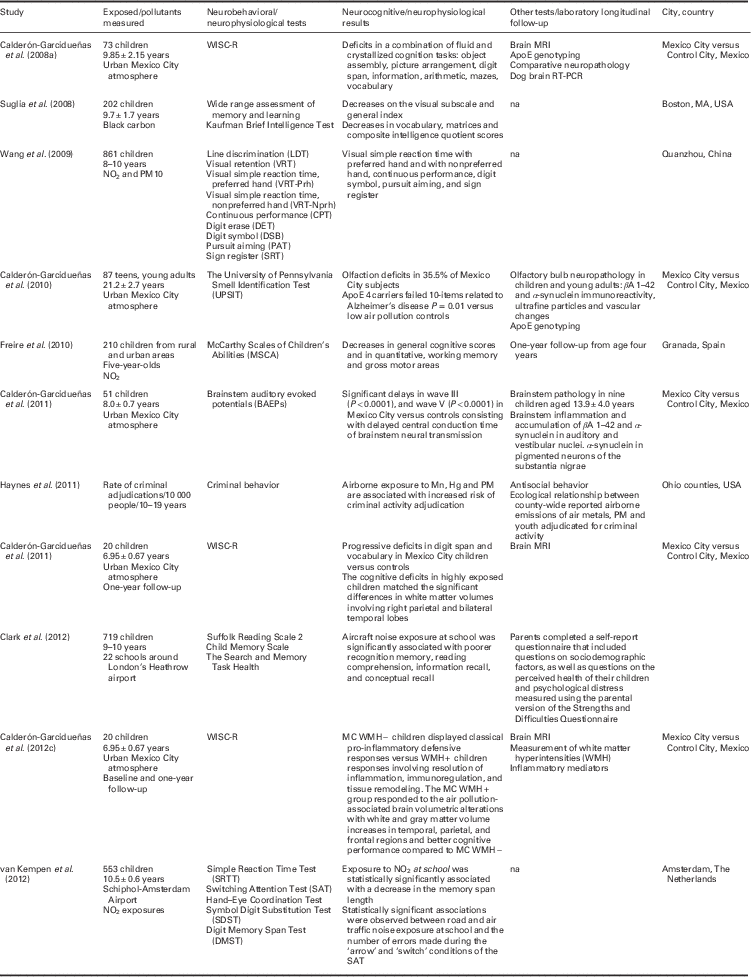
The table shows the populations and the air pollutants studied, the tests and deficits found, other tests used and the city/cities/country where the study took place.
Making the links: detrimental brain effects and cognitive/behavioral functions
As we have already mentioned, depending on the pollutant component, doses, exposure protocol, age and gender, health status, etc., the detrimental effects range from endothelial dysfunction, BBB breakdown, dopaminergic neuronal damage, DNA damage, white matter lesions, neuroinflammation, formation of free radicals and oxidative stress, to the identification of early hallmarks of AD and PD (Campbell et al., Reference Bell, Davis and Fletcher2004; Fonken et al., Reference Fonken, Xu, Weil, Chen, Sun, Rajagopalan and Nelson2011; Levesque et al., Reference Levesque, Surace, McDonald and Block2011; Win-Shwe and Fujimaki, Reference Win-Shwe and Fujimaki2011; Wu et al., Reference Wu, Wang and Sun2011; Brun et al., Reference Brun, Carrière and Mabondzo2012; Guo et al., Reference Guo, Zhu, Guo, Li, Chen, Sang and Yao2012; Sharma and Sharma, Reference Sharma and Sharma2012; Trickler et al., Reference Trickler, Lantz and Schrand2012). The chronic effects of PM2.5 in mice result in spatial learning and memory deficits with neuroinflammation and hippocampal dendritic alterations (Fonken et al., Reference Fonken, Xu, Weil, Chen, Sun, Rajagopalan and Nelson2011). Metal accumulation is associated with memory dysfunction, especially if the metal is administered during the neonatal period, a clear example being iron loading in rodents resulting in impaired spatial memory and in long-term retention of object recognition deficient memory (Schröder et al., Reference Schröder, Silva-Figueiredo and Martins de Lima2013). Equally important in the context of urban environments is the massive exposure to NPs entering the body mostly through inhalation (Win-Shwe et al., Reference Win-Shwe and Fujimaki2011; Brun et al., Reference Brun, Carrière and Mabondzo2012). Gold and silver NPs have an effect on human embryonic neural precursor cell growth, suggesting a negative impact of NPs on the developing CNS (Söderstjerna et al., Reference Söderstjerna, Johansson, Klefbohm and Englund-Johansson2013). Intense oxidative damage and lipid, protein and DNA peroxidation are observed in mice after intranasal administration of TiO2 NPs (Ze et al., Reference Ze, Sheng, Zhao, Ze, Wang, Zhou, Liu, Yuan, Gui, Sang, Sun, Hong, Yu, Wang, Li and Hong2014). Ferromagnetic mineral magnetite (Fe(3)O(4)) NPs cause structural changes of microtubule and tau protein, essential in the memory mechanism and memory dysfunction (Dadras et al., Reference Dadras, Riazi, Afrasiabi, Naghshineh, Ghalandari and Mokhtari2013). Ozone, the main component of photochemical pollution in urban areas has been shown to alter adult neurogenesis and produce progressive hippocampal neurodegeneration along with memory deficits in rats exposed to low subchronic concentrations (Rivas-Arancibia et al., Reference Rivas-Arancibia, Guevara-Gúzman, Lopez-Vidal, Rodriguez-Martinez, Zanardo-Gomez, Angoa-Pérez and Raisman-Vozari2010).
Clinically healthy urban children from MCMA selected by stringent criteria exhibited structural, neurophysiological and cognitive detrimental effects compared with matched SES, gender, age and mother’s IQ low pollution exposed children (Calderón-Garcidueñas et al., 2008Reference Calderón-Garcidueñas, Mora-Tiscareño, Ontiveros, Gómez-Garza, Barragán-Mejía, Broadway, Chapman, Valencia-Salazar, Jewells, Maronpot, Henríquez-Roldán, Pérez-Guillé, Torres-Jardón, Herrit, Brooks, Osnaya-Brizuela, Monroy, González-Maciel, Reynoso-Robles, Villarreal-Calderon, Solt and Englea; Calderón-Garcidueñas et al., Reference Calderón-Garcidueñas, D’Angiulli, Kulesza, Torres-Jardón, Osnaya, Romero, Keefe, Herritt, Brooks, Avila-Ramirez, Delgado-Chávez, Medina-Cortina and González-González2011 Reference Calderón-Garcidueñas, D’Angiulli, Kulesza, Torres-Jardón, Osnaya, Romero, Keefe, Herritt, Brooks, Avila-Ramirez, Delgado-Chávez, Medina-Cortina and González-Gonzáleza). The cognitive deficits in MC children matched the magnetic resonance imaging (MRI) volumetric changes in their right parietal and bilateral temporal areas (Calderón-Garcidueñas et al., Reference Calderón-Garcidueñas, Kavanaugh, Block, D’Angiulli, Delgado-Chávez, Torres-Jardón, González-Maciel, Reynoso-Robles, Osnaya, Villarreal-Calderon, Guo, Hua, Zhu, Perry and Diaz2012b). Highly exposed children without white matter hyperintensities (WMH−) displayed the profile of classical pro-inflammatory defensive responses: high IL-12, production of powerful pro-inflammatory cytokines and low concentrations of key cytokines and chemokines associated with neuroprotection. Conversely, children with WMH+ exhibited a response involved in resolution of inflammation, immunoregulation and tissue remodeling. The WMH+ group responded to the air pollution-associated brain volumetric alterations with white and gray matter volume increases in temporal, parietal and frontal regions and better cognitive performance compared with the WMH− group. We conclude that complex modulation of cytokines and chemokines influences children’s CNS structural and volumetric responses and cognitive correlates resulting from environmental pollution exposures. Regardless of the presence of prefrontal WMH, MC children performed more poorly across a variety of cognitive tests, compared with control children.
We have identified a number of abnormalities also within the auditory brainstem nuclei in children exposed to severe air pollution. Specifically, we have observed that neuronal cell bodies within the medial superior olive (MSO) are significantly smaller and more round than those in age-matched control brains (Calderón-Garcidueñas et al., Reference Calderón-Garcidueñas, Engle, Mora-Tiscareño, Styner, Gomez-Garza, Zhu, Jewells, Torres-Jardón, Romero, Monroy-Acosta, González-González, Medina-Cortina and D'Angiulli2011b). We interpret this dysmorphology to indicate injury and dysfunction in the MSO. The MSO is the largest nucleus within the human superior olivary complex and has clear roles in localization of sound sources, encoding temporal features of sound and likely plays an important role in brainstem encoding of speech. Incidentally, similar morphological alterations were observed in autistic children (Kulesza and Mangunay, Reference Kulesza and Mangunay2008; Kulesza et al., Reference Kulesza, Lukose and Stevens2011). Confirming brainstem pathology, MCMA children showed clearly abnormal BAEPs with delays in wave III and wave V but no delay in wave I. These findings are consistent with delayed central conduction time of brainstem neural transmission, and increased risk for auditory and vestibular impairment (Calderón-Garcidueñas et al., Reference Calderón-Garcidueñas, Engle, Mora-Tiscareño, Styner, Gomez-Garza, Zhu, Jewells, Torres-Jardón, Romero, Monroy-Acosta, González-González, Medina-Cortina and D'Angiulli2011b). We are assessing the integrity of the auditory system in highly exposed children through a number of noninvasive techniques, such as BAEPs, otoacoustic emissions, speech recognition tasks and listening in background noise.
It is clear that air pollution exposed children experience a chronic, intense state of oxidative stress and exhibit an early brain imbalance in genes involved in inflammation, innate and adaptive immune responses, cell proliferation and apoptosis. Neuroinflammation, endothelial activation and the breakdown of the BBB contribute to cognitive impairment and pathogenesis and pathophysiology of neurodegenerative states (Roher et al., Reference Roher, Debbins, Malek-Ahmandi, Chen, Pipe, Maze., Belden, Maarout, Thiyyagura, Mo, Hunter, Kokjohn, Walker, Kruchowsky, Belohlavek, Sabbagh and Beach2012; Jian et al., Reference Jian, Yi-Fang, Qi, Xiai-Song and Gui-Yun2013). The associations between cognition and urban pollution has been established in cities like Boston, where black carbon – a marker for traffic PM – predicted decreased cognitive function across assessments of verbal and nonverbal intelligence and memory in nine-year-olds (Suglia et al., Reference Suglia, Gryparis, Wright, Schwartz and Wright2008).
A working framework for prevention and intervention
Although genetic factors play a key role in CNS responses (as evidenced by the acceleration of neurodegenerative pathology in children carrying an APOE 4 allele), studies such the above mentioned ones in Boston and others, sketch a complex scenario where air pollution and SES can influence neural development and cognition, as well as genetics, nutrition, access to a cognitively stimulating environment, thereby influencing and determining mental health, academic achievements and overall life performance (D’Angiulli et al., Reference D’Angiulli, Warburton, Dahinten and Hertzman2009; Siddique et al., Reference Siddique, Banerjee, Ray and Lahiri2011; Calderón-Garcidueñas & Torres-Jardón, Reference Calderón-Garcidueñas and Torres-Jardón2012a; Becerra et al., Reference Becerra, Wilhelm, Olsen, Cockburn and Ritz2013).
Thus, identification of children at risk for cognitive deficits, brain structural/volumetric and neurodegenerative accelerating changes should be prioritized in populations exposed to significant concentrations of air pollutants. There is growing public concern about the direct and indirect influences air pollution may have on several developmental outcomes such as school performance, behavioral changes and mood disorders in children and teens. Moreover, childhood aggression and teen delinquency are increasing in megacities, establishing early environmental health risk factors for violence prediction and prevention (Haynes et al., Reference Haynes, Chen, Ryan, Succop, Wright and Dietrich2011; Liu, Reference Liu2011) in populations at risk will be absolutely critical. New concerns involve the association between air pollution exposure and increased risk of attention deficit hyperactive disorder (Siddique et al., Reference Siddique, Banerjee, Ray and Lahiri2011) and autism (Becerra et al., Reference Becerra, Wilhelm, Olsen, Cockburn and Ritz2013) in young and older children.
Strong support for the need of neurocognitive screening comes from a growing psychological and epidemiological literature suggesting evidence of suboptimal cognitive functioning across the developmental span in clinically healthy children (Guxens and Sunyer, Reference Guxens and Sunyer2012; Calderón-Garcidueñas et al., Reference Calderón-Garcidueñas, Kavanaugh, Block, D’Angiulli, Delgado-Chávez, Torres-Jardón, González-Maciel, Reynoso-Robles, Osnaya, Villarreal-Calderon, Guo, Hua, Zhu, Perry and Diaz2012b). Importantly, a significant proportion of urban schools are situated near major traffic-related air pollution sources (Amram et al., Reference Amram, Abernethy, Brauer, Davies and Allen2011; Amato et al., Reference Amato, Rivas, Viana, Moreno, Bouso, Reche, Alvarez-Pedrerol, Alastuey, Sunyer and Querol2014), and cognitive outcomes may be partly associated with air pollution levels around schools (Mohai et al., Reference Mohai, Kweon, Lee and Ard2011).
Consistent with these observations, the National Institute of Environmental Health Sciences/National Institute of Health panel on outdoor air pollution indicated cognitive, neuropsychological (and possibly neuroimaging) screening of children as one of the priority target areas for future research advocating a multidisciplinary collaborative approach wherein brain-related cognitive (henceforth, neurocognitive) development testing would have a prominent role (Block et al., Reference Block, Elder, Auten, Bilbo, Chen, Chen, Cory-Slechta, Costa, Diaz-Sanchez, Dorman, Gold, Gray, Jeng, Kaufman, Kleinman, Kirshner, Lawler, Miller, Nadadur, Ritz, Semmens, Tonelli, Veronesi, Wright and Wright2012).
Hence, the use of standard neurocognitive tasks to screen clinically healthy children in schools or pediatric offices seems not only desirable but also highly beneficial. Because the primary goal would be to identify children at risk, possibly at the largest scale, the first step could be to screen entire schools in different neighborhoods with different air pollutant profiles. Multidisciplinary approaches for early risk identification could include using air pollution databases from available monitoring stations to gather: (1) Air pollutant profiles in selected geographic area; (2) exposures to traffic-related air pollutants at each child’s school and current residence with land use regression models that combine a geographic information system with ambient passive monitoring in the target area; (3) cumulative ambient exposures to fine PM2.5; (4) robust baseline information on the oxidative potential and metal content of PM found in the targeted regions; (5) cognitive screening first and, when applicable, more elaborate neurocognitive/neurophysiological follow-ups, which could include EEG/ERPs, BAEPs, MRI, f MRI and MRS.
The first goal in targeted areas will be to define the cohorts with the most risk for neurocognitive deficits based on traffic emissions, fixed sources of contaminants, profile of toxic pollutant components (ie, metals) and cumulative concentrations of fine PM. The initial studies should be followed by interventions aimed at breaking the cycle of air pollution, indoor air pollution, tobacco use, high body mass index, low fruit and vegetable intake and physical inactivity. Since health risk factors are more likely to have a toll on low SES children, identification of spatial concentrations of low SES pediatric populations at risk, should be prioritized (Forastiere et al., Reference Forastiere, Stafoggia, Tasco, Picciotto, Agabiti, Casaroni and Perucci2007; Ou et al., Reference Ou, Hedley, Chung, Thach, Chau, Chan, Yang, Ho, Wong and Lam2008).
According to the American Neuropsychiatric Association and the Shulman criteria (Malloy et al., Reference Malloy, Cummings, Coffey, Duffy, Fink, Lauterbach, Lovell, Royall and Salloway1997; Shulman, Reference Shulman2000), the ideal cognitive screening instrument should (i) be relatively brief to administer; (ii) be easy to score; (iii) well tolerated and accepted by participants, in our cases both child and parent; (iv) test all targeted cognitive domains; (v) be valid and reliable; and (vi) relatively independent of or controlling for age and education.
A possible battery of tests (easily translatable in many languages) for the initial screening of school-aged children is described in Table 2. The measures in this table have reference to previous neuroimaging findings implicating functional, maturational or structural correlates of frontal, parietal and temporal regions and/or neurocognitive tests applied to air pollution studies in children as seen on Table 1. Because several studies link risk of negative effects of air pollution to early development, from infancy to preschool, screening could start in daycare or during transition from kindergarten to first grade, for example using tools such as the Early Development Instrument (D’Angiulli et al., Reference D’Angiulli, Warburton, Dahinten and Hertzman2009). With advances in wireless neuroimaging technology, neurocognitive screening may even incorporate rapid EEG/ERP recording procedures.
Table 2 Suggested battery of neuropsychological and psychoeducational tests for the initial screening of school-aged children and teens exposed to urban air pollution

In summary, air pollution effects on the developing brain may vary along a continuum from minor subclinical deficits in cognitive functioning to significant cognitive deficits that are identified readily by parents and/or teachers. The detrimental effects may also worsen with the age of the child, thus selected neurocognitive tools ought to be useful for longitudinal studies, across educational backgrounds and expecting overlaps in the functional areas and tests affected. Complex cognitive responses that may be affected include attention and short-term memory, information processing speed and executive function, verbal abstraction and visuospatial and motor skills. We should also expect deficits in auditory and vestibular responses and sound localization, along with olfaction deficits. The diffuse nature of the neuroinflammation and the neurodegenerative changes observed in exposed children obligates us not to rely on a single study or measure but rather to employ a weight of evidence approach incorporating current clinical, neurophysiological, radiological and epidemiological research as well as the results of animal exposure studies to single pollutants/mixtures/or pollutant components. Inflammatory biomarkers play a key role in the identification of children with positive volumetric and cognitive responses to their lifelong pollutant exposures (Calderón-Garcidueñas et al., Reference Calderón-Garcidueñas, Kavanaugh, Block, D’Angiulli, Delgado-Chávez, Torres-Jardón, González-Maciel, Reynoso-Robles, Osnaya, Villarreal-Calderon, Guo, Hua, Zhu, Perry and Diaz2012b) and since neuroinflammation/vascular damage/neurodegeneration go hand in hand (Calderón-Garcidueñas et al., 2013Reference Calderón-Garcidueñas, Franco-Lira, Mora-Tiscareño, Medina-Cortina, Torres-Jardón and Kavanaugha and 2013Reference Calderón-Garcidueñas, Cross, Franco-Lira, Aragón-Flores, Kavanaugh, Torres-Jardón, Chao, Thompson, Chang, Zhu and D’Angiullib), definition of inflammatory/endothelial dysfunction biomarkers establishing an association between brain growth and cognition are urgently needed.
Of course, in light of the findings reviewed in the present paper, a pressing important question that jumps to mind is whether the prevalence of AD/PD is increased in MCMA? Unfortunately we do not have accurate records from health institutions. Mexico health care is covered by both private and government hospitals and institutes and there is no universal statistical information regarding AD and PD prevalence. Empirically, however, neurologists and general practitioners report a significant increase in the number of cases for both neurodegenerative diseases (Victor Esquivel MD, Neurologist, personal communication). Death certificates that would be a source of information, unfortunately fail to list AD or PD in the certificate, basically because families are reluctant to link their patients to a disease with genetic implications, and so only allowed for the writing of acute causes of death like pneumonia, but not the base disease. Therefore, the answer to the central issue of incidence of AD/PD in MCMA is very much an open empirical question for future research.
Our ultimate goal is to protect exposed children through multidimensional interventions yielding both impact and reach: cognitive (Diamond and Lee, Reference Diamond and Lee2011), family participation (Josephson, Reference Josephson2013) and modifiable lifestyle factors such as diet and micronutrient supply (Villarreal-Calderon et al., Reference Villarreal-Calderón, Torres-Jardon, Palacios-Moreno, Perez-Guille, Maronpot, Reed, Zhu and Calderón-Garciduenas2010; Calderón-Garcidueñas et al., Reference Calderón-Garcidueñas, Mora-Tiscareño, Styner, Gómez-Garza, Zhu, Torres-Jardón, Carlos, Solorio-López, Medina-Cortina, Kavanaugh and D’Angiulli2012c).
Air pollution brain effects on children and teens ought to be key public health targets.
Key points to remember
-
∙ Risk for stroke and depression are associated with common ambient air pollutants, including fine and UFPM and ozone.
-
∙ The stroke risk is present even at pollutant concentrations below the current standards, meaning there is a wide spectrum of susceptibility to pollutants likely related to factors such as the presence of chronic diseases, and genetic and nutrition variables.
-
∙ Stroke is not a stroke, specific patient characteristics modify associations between air pollution and ischemic stroke (Villeneuve et al., Reference Villeneuve, Johnson, Pasichnvk, Lowes, Kirkland and Rowe2012).
-
∙ Check for factors that will aggravate the neurological/psychiatric effects of air pollution such as diabetes, hypertension, infectious processes, residency in close proximity to a busy road, changes in occupation, etc.
-
∙ Keep an eye on the air quality index of your city, record proximity of the patient to high density traffic (Mohai et al., Reference Mohai, Kweon, Lee and Ard2011; van Kempen et al., Reference Van Kempen, Fischer, Janssen, Houthuijis, van Kamp, Stansfeld and Cassee2012) or fixed sources of pollutants, check for acute events such as forest fires or the arrival of fumes from a distant area (Chen et al., Reference Chen, Villeneuve, Rowe, Liu and Stieb2013).
-
∙ PM occupational exposures and tobacco are to be taken into consideration as important sources of pollutants.
-
∙ The patient’s occupation and hobbies are important. Is she an outdoor person? Is he protected against toxic substances at work? Is there a significant source of PM or NPs at the office? Where is the patient from? A good clinical history is a must.
-
∙ Parents and school officials should be aware of the high pollutant concentrations in their area and keep children indoors.
-
∙ Identification of children at risk for cognitive deficits, brain structural/volumetric and neurodegenerative accelerating changes should be prioritized in populations exposed to significant concentrations of air pollutants.
-
∙ Brain damage is cumulative and has deleterious effects on cognitive, emotional and behavioral areas.
-
∙ Preventive measures against the harmful effects of environmental pollution should be referred to and applied as early as the conception time.
-
∙ Finally, we must consider whether we want to pay the high price that involves ignoring the serious health impacts of environmental pollution.
Acknowledgment
Gratitude is due to all children and their families who have participated in our clinical studies.
Financial Support
None.
Conflicts of Interest
None.
Ethical Standards
The authors assert that all procedures contributing to this work comply with the ethical standards of the institutional guidelines based on the Helsinki Declaration of 1975, as revised in 2008.