Introduction
Background
There is little doubt that the advent of computerized tomography (CT) and other diagnostic radiologic imaging has revolutionized the practice of medicine; CT scans have essentially become ubiquitous in the United States, where approximately 74 million scans were performed in 2017.1 While this imaging technique offers benefits, the potential cancer risk associated with these studies remains controversial. If the wide-spread use of this technology is really inducing thousands of future cancer deaths, as suggested by Brenner, Berrington de Gonzalez, Smith-Bindman, and others, then the restrictive clinical guidelines being recommended by multiple organizations would be justified to protect the general population.Reference Brenner and Hall2–Reference Smith-Bindman, Lipson and Marcus4 However, if the risk is inaccurately represented, then the mandate to reduce CT scanning regardless of indication may not be rational. It is imperative that clinicians, as well as the public, understand the risks and benefits of diagnostic studies used in everyday practice. Therefore, an objective analysis of the evidence to date is necessary to evaluate the risk of cancer from CT scans.
To help understand the approach used in the following investigation, some background information is needed. For general purposes, when quantifying radiation exposure, the units of measure used are the Sievert (Sv) and Gray (Gy). The Sv quantifies the amount of damage done to the human body while the Gy is the energy absorbed in the body per unit mass. For both x-rays and gamma rays, the two units of measure are numerically equivalent (ie, one Gy of radiation dose causes one Sv of damage). In addition, both gamma rays and x-rays are thought to behave the same within human tissue, causing equivalent injury. Therefore, cancer studies involving either entity apply to both. The dose from a single CT scan ranges from two millisieverts (mSv) to 20 mSv, with an average of two mSv per head CT and 10 mSv per CT scan of the abdomen and pelvis. As a comparison, most Americans receive three mSv per year from natural background radiation sources.Reference Mettler, Huda and Yoshizumi5
The most important study in estimating the cancer risk from radiation exposure is the continuous long-term follow-up investigation of Japanese survivors from the atomic bombs dropped on Hiroshima and Nagasaki in 1945. This longitudinal study is known as the Life Span Study, or LSS. In this Japanese population, cancer mortality and incidence have been studied as a function of radiation dose. Periodic reports on the data from this study are published by the Radiation Effects Research Foundation (Hiroshima, Japan).6 The health effects of radiation have been reviewed and published periodically by the Biological Effects of Ionizing Radiation Committee, or BEIR, of the National Academy of Sciences (Washington, DC USA), with the latest iteration of the report being BIER VII in 2006.7
Since the first assessments of cancers in LSS participants, the analyses have reported a linear relationship between cancer incidence/mortality and increasing radiation dose when examining exposures of approximately one Gy and above. A report published in 2012 analyzing LSS data from 1950–2003 used a linear quadratic approach but asserts a similar overall relationship.Reference Ozasa, Shimizu and Suyama8 However, a separate evaluation focused on the cancer risk at lower doses that did not rely on the linear model, especially at 200 milligray (mGy) and below, was not performed.
Due to the observed linear relationship at higher doses, and the potential for large errors in estimating excess cancer risk at low-doses, the authors of the serial BEIR Committee reports have extrapolated the observed linear relationship between radiation dose and excess cancers to apply to all doses. This approach has led to the linear-no-threshold (LNT) model relating cancer risk to exposure, illustrated in Figure 1. While this figure is derived from earlier data, it depicts the concept. The theory states that any amount of radiation increases cancer risk and no safe level of exposure exists. In other words, no threshold exists below which radiation exposure does not cause cancer. This concept of a linear relationship between cancer risk and radiation dose across all exposure levels has essentially been used for estimations of health risks from radiation for the last 60 years. Calculations of cancers caused by radiation exposure and safety limits for exposed populations were all derived from the LNT model.
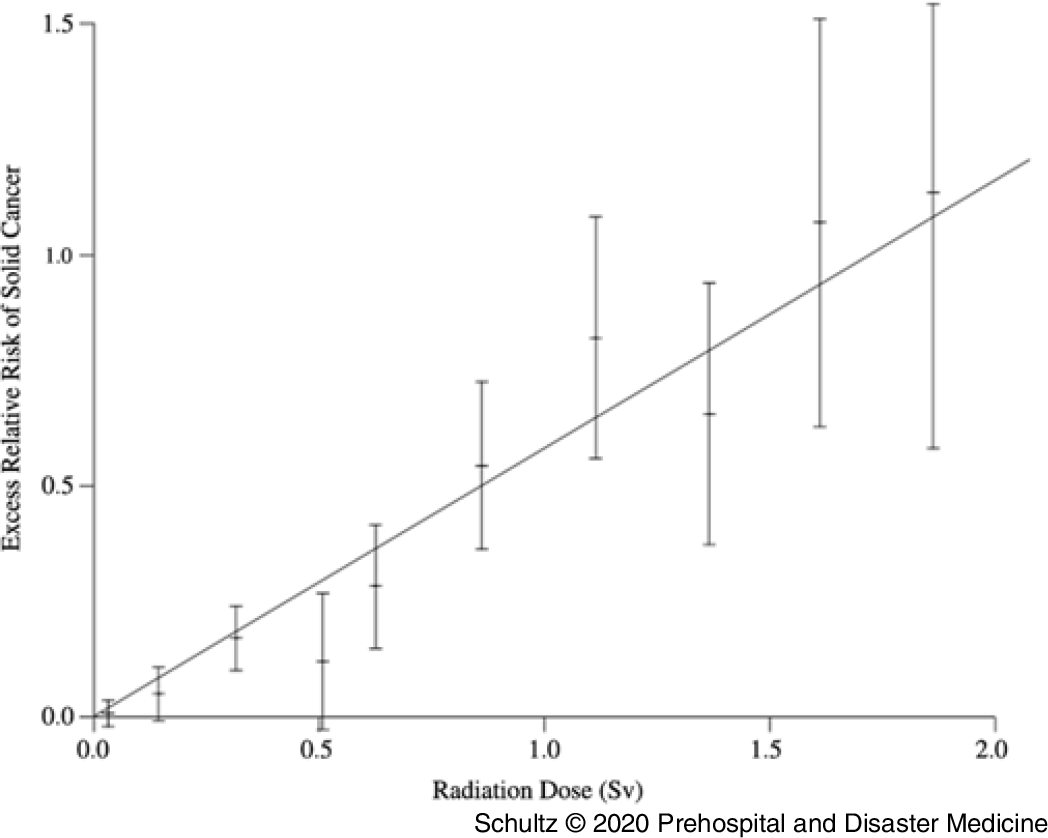
Figure 1. Linear-No-Threshold (LNT) Model.
Importance
For the first few decades after the model was proposed, little reason existed to challenge it. Only a minute segment of the population was at-risk for significant radiation exposure. All this changed with the invention of the CT scan in 1972, and the exponential rise in its utilization since. Now, very large segments of the population are receiving higher radiation exposures, and the potential risk of cancer in the dose range of 100–200 mSv has become a very important issue. Recent publications have reached conflicting conclusions with respect to the cancer risk from low-dose exposure, resulting in intense debate regarding the validity of the LNT model.Reference Ozasa, Shimizu and Suyama8,Reference Grant, Brenner and Sugiyama9 As such, the presence or absence of a threshold exposure limit for radiation-induced cancer has become a critical issue for the medical community and the public. Significant effort and progress have been made by the medical community to reduce radiation exposure, as documented by the National Council on Radiation Protection 160 (Bethesda, Maryland USA) and benchmarks such as the American College of Radiology’s (Reston, Virginia USA) CT Dose Registry.
The latest analysis of the LSS data published by Grant, et al in 2017 only fuels the debate. Investigators demonstrated a threshold for cancer risk in men below 100 mGy.Reference Grant, Brenner and Sugiyama9 However, the authors were unable to make firm conclusions stating: “Findings from the current analysis regarding the dose-response shape were not fully consistent with those previously reported, raising unresolved questions. At this time, uncertainties in the shape of the dose response preclude definitive conclusions to confidently guide radiation protection policies.”Reference Grant, Brenner and Sugiyama9 Nonetheless, the authors supported the use of a linear approach to data analysis consistent with the LNT model.
It is difficult to digest the large amount of data available and the conflicting conclusions stated in these publications. The authors considered using a meta-analytic approach for evaluating study outcomes but rejected it. All the investigations of human low-dose radiation exposure in the world’s literature involve only cohort or case control studies. No prospective randomized trials exist. Cohort and case control studies are considered less robust designs, making meta-analysis of their results of questionable value. While some cohort and case control studies follow appropriate design rules, many are poorly constructed and highly flawed. As such, applying meta-analytic techniques to this mixture will yield results heavily confounded by lack of controls, poor study design, and flawed statistical evaluations. The resulting odds ratios and confidence intervals would have, at best, weak validity and at worst, be completely meaningless. In addition, the value of combining such studies is questionable. Given many involve different populations, outcome measures, sources of radiation, duration of exposure, sample size, and length of follow-up, the measurement of their heterogeneity would be very high, further compromising the validity of any findings.
It might be possible, however, to approximate an answer by identifying all the case control and cohort studies that more rigorously adhere to sound methodological design (utilize higher quality methodologies) and determine the number that either support or do not support the hypothesis that low-dose radiation causes cancer. A large preponderance of such investigations that endorse one or the other perspective would provide useful guidance.
Study Objective
Toward this end, the authors proposed examining the methodologic quality of investigations evaluating low-dose radiation and cancer induction published from 1975 through 2017. The articles’ methodologies are scored using two separate tools to create a quantitative assessment measuring the strength of study design and identifying higher quality investigations. This will permit direct comparison between the number of studies using higher quality methodologies supporting cancer induction by low-dose radiation, and those refuting it, allowing assessment of the risks of cancer from low-dose radiation.
Methods
Study Design and Article Selection
Following the PRISMA guidelines, a systematic search was conducted in MEDLINE/PubMed (US National Library of Medicine, National Institutes of Health; Bethesda, Maryland USA); Scopus (Elsevier; Amsterdam, Netherlands; which includes coverage of EMBASE); Web of Science (Thomson Reuters; New York, New York USA); and the Cochrane Library (The Cochrane Collaboration; London, United Kingdom) for studies published in English from January 1975 through October 2017 evaluating the risk of cancer from low-dose radiation. The authors began the review in 1975 to allow sufficient time after the 1972 invention of the CT scan for research publications to appear that might include the device. A professional health sciences librarian (LM) designed and conducted the initial search on PubMed, and then applied the search strategy (Appendix A; available online only) to subsequent selected databases. All search results were imported into EndNote (Clarivate Analytics; Philadelphia, Pennsylvania USA), wherein duplicate studies were removed. For the search string, low-dose was defined as less than 200 mSv for the purposes of article inclusion. The current definition of low-dose is up to 100 mSv; however, the authors wanted to ensure identification of all potential studies.10 In addition, articles investigating low-dose radiation were not restricted to diagnostic medical devices. Analyses of low-dose gamma radiation, such as studies examining cancer rates in nuclear power industry workers or those exposed to elevated levels of background radiation, were included. Other publications from government and regulatory agencies were added as well, as those identified from selected article bibliography reviews conducted manually by the authors.
Eligibility Criteria for Inclusion
To be included in the study, articles had to address the potential role or impact of low-dose gamma or x-ray radiation on the risk of developing any type of cancer. A list of article inclusion and exclusion criteria are contained in Table 1. First, two reviewers (CHS and RF) independently screened the titles and abstracts based on the criteria in Table 1. If this evaluation suggested the publications were potentially eligible for inclusion, the same reviewers examined the full-texts to make a final determination. If the two reviewers disagreed regarding study eligibility, differences of opinion were resolved by consensus. Any persistent disagreement was resolved by a third independent reviewer (MD). For datasets with multiple publications that serially evaluated the same population over time, only the most recent publication was considered for inclusion in the study.
Table 1. Article Inclusion and Exclusion Criteria

Measurements or Key Outcomes
After identifying the final set of articles, the methodology of each paper was independently scored by two reviewers (CHS and RF) using two separate tools to determine the quality of the research approach. Reviewers used a scoring template to reduce inconsistency. The first tool is the Newcastle Ottawa Scale (NOS) initially created by George Wells, PhD.Reference Wells, Shea and O’Connell11 In an extensive review of approaches used for evaluation of non-randomized studies, 194 tools were identified that could be or had been used to assess such trials. Of these, only six were found to be suitable for systematic reviews, with the NOS being one.Reference Deeks, Dinnes and D’Amico12 The NOS was chosen as it is specifically designed to evaluate the methodologies of cohort and case control studies, has been in use for over 15 years, and is frequently referenced in the emergency medicine literature. It scores articles on a range of zero to nine based on evaluations in three categories: (1) Selection of cases/controls or cohorts; (2) Comparability of case/controls or cohorts based on design; and (3) Exposure or Outcome (Appendix B; available online only). Articles with scores of seven or greater were considered higher quality, given that most authors using the NOS identify this number as the lower limit characterizing good studies. However, the NOS does not evaluate all methodological aspects. To capture potential weaknesses the NOS misses, a second tool is required to fully characterize the studies by examining data analysis. This tool was developed by the authors and adapted from established resources in research methodology (ie, statistics texts and review articles). It identifies 11 lower quality indicators that are used to score the studies. Examples of these indicators include use of 90% confidence intervals, one-tailed T tests, failure to address confounding variables or predisposing factors, and no adjustment for multiple comparisons (Appendix C; available online only). An article found to contain two or fewer indicators is considered higher quality, mirroring the NOS that allows a reduction of up to two points for higher quality methodologies. An article’s methodology was considered higher quality if: (1) it scored seven or more on the NOS, and (2) it contained two or fewer lower quality indicators. Failure to meet both metrics resulted in a study’s methodology evaluated as lower quality. After independent scoring of all articles, both reviewers compared their results to reach consensus.
For meta-analytic studies, the NOS is not appropriate. To score these investigations, the authors used the Quality Assessment of Systematic Reviews and Meta-Analyses developed by the National Institute of Health’s (Bethesda, Maryland USA) National Heart, Lung, and Blood Institute. This tool evaluates eight criteria as present or absent and yields a rating of good, fair, or poor.13 Articles containing a minimum of six criteria were considered higher quality, to maintain consistency with the other scoring tools. The lower quality indicator tool was applied to these studies without modification. Only one article required assessment using this tool.
Data Analysis
The articles were then divided into two groups: those supporting the risk of cancer from low-dose radiation, and those that did not. Investigators determined the support status by examining the conclusion section of the abstract, and when necessary, the conclusion section in the manuscript. The conclusion had to state or imply that all doses of radiation were associated with increased cancer risk. In some studies, the data reported in the results potentially contradicted the authors’ conclusions. For the purposes of this investigation, however, the authors’ statements were used to characterize the article. This same approach was used for studies that found an increase in some cancers but not others. Articles identified as not supporting the risk of cancer had to demonstrate a threshold for cancer induction at some level up to and including100–200 mSv, and that increased cancer rates were not observed or existed only above the threshold value. Finally, the number of higher quality articles supporting or not supporting cancer induction by low-dose radiation was counted, and the difference between the two groups was evaluated using a chi-squared test for independence (Stata, Statacorp; College Station, Texas USA) with a P value of less than .05 considered significant. Since the study involved only de-identified data, the research team was not required to obtain Institutional Review Board approval.
Results
Characteristics of Selected Articles
The literature search identified 4,382 potential articles for consideration: 4,361 retrieved from electronic databases and 21 obtained from bibliography reviews. After removal of duplicates, 3,578 publications remained. Initial screening of titles and abstracts reduced the number of articles further to 237. Detailed, independent full-text review of these remaining publications resulted in identification of 62 articles for inclusion in the study. Consensus was reached on all articles except one, which required intervention by a third author to adjudicate its disposition. A detailed graphic representation of the process following the PRISMA guidelines is listed in Figure 2.
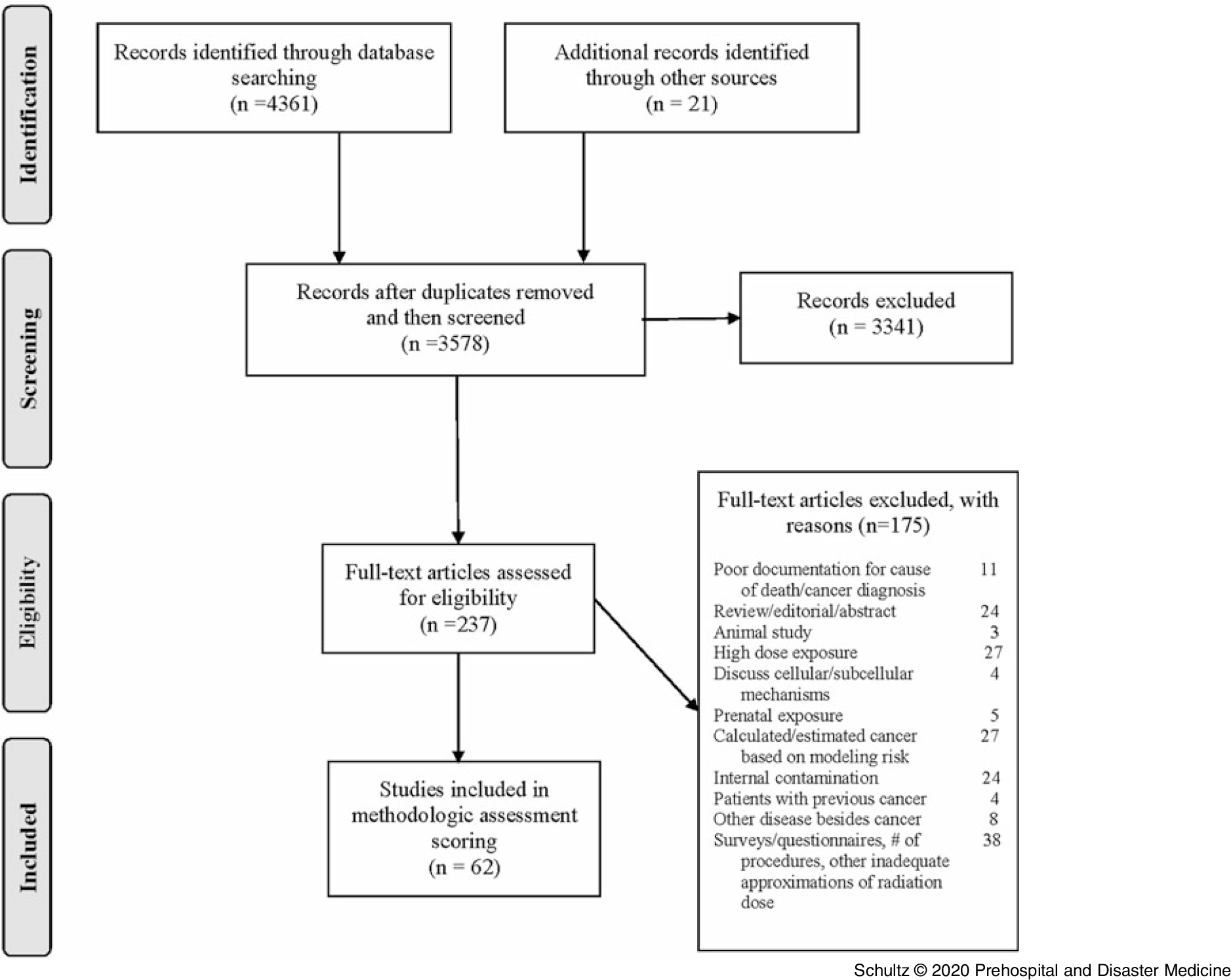
Figure 2. PRISMA Flow Diagram of Study Selection.
Main Results
After evaluating and scoring the methodologic quality of all 62 articles, researchers identified 25 articles as utilizing higher quality approaches (Table 2Reference Grant, Brenner and Sugiyama9,Reference de Gonzalez, Salotti and McHugh14–Reference Storme, Iversen and Boice37 ) and 37 characterized by lower quality approaches (Table 3Reference Daniels, Bertke and Richardson38–Reference Jablon and Boice74). These were fairly evenly divided with 27 articles endorsing cancer induction by low-dose radiation and 35 rejecting it. Examining the 25 higher quality methodology publications, the authors found 21 did not support the risk of cancer from low-dose radiation and four supported it. Using a chi-squared test for independence, this finding was significant with P = .0003. The only publications to receive perfect scores on the two methodologic evaluations both did not support cancer risk. For investigations using lower quality methodologies, 23 supported cancer induction while 14 rejected it. Details of the scoring evaluation for each article are found in Appendix D (available online only).
Table 2. Articles with Higher Quality Methodologies Characterized by Supporting or Not Supporting Cancer Induction by Low-Dose Radiation

Abbreviations: CNS, Central Nervous System; CT, Computed Tomography; NHL, Non-Hodgkin’s Lymphoma; NOS, Newcastle Ottawa Scale; US, United States.
Table 3. Articles with Lower Quality Methodologies Characterized by Supporting or Not Supporting Cancer Induction by Low-Dose Radiation
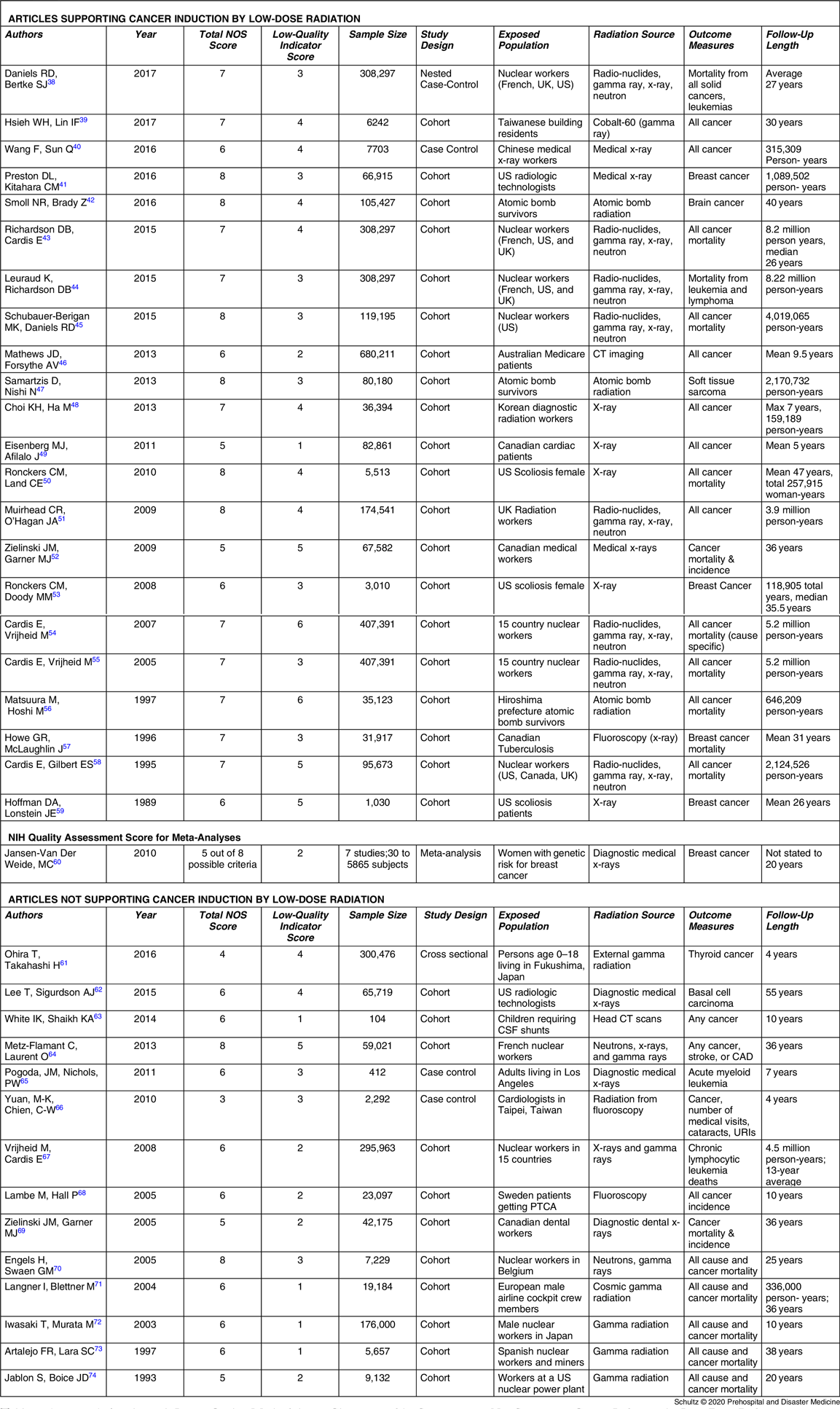
Abbreviations: CAD, Coronary Artery Disease; CSF, Cerebral Spinal Fluid; CT, Computed Tomography; NOS, Newcastle Ottawa Scale; PTCA, Percutaneous Transluminal Coronary Angioplasty; UK, United Kingdom; URI, Upper Respiratory Infection; US, United States.
Discussion
The initial possibility that radiation might increase mutations, and subsequently the risk of cancer, was raised by the work of HJ Muller, published in 1927.Reference Muller75 He demonstrated a linear increase in fruit fly mutations with exposure to rising doses of x-rays, but used only high doses with the lowest dose being four Gy. A later study performed by Spencer during the Manhattan Project showed a similar linear relationship of mutations to radiation dose for short-duration exposures, but did not expose the fruit flies to low-dose radiation, stopping at a dose of 250 mGy.Reference Calabrese76 The influence of the Spencer investigation, in combination with the advocacy by Muller and others, resulted in the formal acceptance of the LNT model (all radiation causes cancer and the risk increases as a linear function of increasing radiation dose) by the National Academy of Sciences Genetics Panel on the Biological Effects of Atomic Radiation (BEAR I Committee, later to become the BEIR Committee) in 1956.77
This linear model has remained the pivotal explanation for the relationship between radiation and cancer. The entire premise behind articles such as the one by Brenner, et al and others, that CT scans are causing cancer, is based on this model.Reference Brenner and Hall2,Reference Berrington de Gonzalez, Mahesh and Kim3 If this model is false, however, his estimate that possibly as many as 1.5% to 2.0% of all cancers in the United States are caused by CT scans would be wrong. The purpose of this study was to examine the methodologic strengths of the literature and determine if the evidence from investigations using higher quality case control and cohort study methodologies supports or does not support the risk of cancer from low-dose radiation.
Researchers identified only four out of 25 studies characterized by higher quality methodologies that endorsed a risk of cancer from low-dose radiation. While no standard exists that can absolutely indicate when a sufficient quantity of higher quality studies has been performed to change practice, one can consider a change if the clear preponderance of these studies suggests a new direction is indicated. In this analysis, 21 out of 25 investigations characterized as using higher quality methodologies demonstrated the existence of a threshold for cancer induction. These studies demonstrated no increased cancer risk for individuals experiencing radiation exposures up to at least 100 mGy, and possibly as high as 200 mGy.
The authors acknowledge that attempting a quantitative analysis of methodologic quality will incorporate a certain amount of subjectivity. No tool currently available can completely eliminate this risk. However, significant guidance on use of the NOS exists, including structured scoring tools that can increase consistency in evaluations. These data collection sheets were employed throughout the entire evaluation (Appendix E; available online only). In addition, the use of the lower quality indicator tool to supplement the NOS provides a method of evaluation that is less subject to interpretation and more objective. This second evaluation tool is needed because the NOS does not evaluate how data are analyzed. Even if a study received a perfect score on the NOS, it can still have significant weaknesses in the way data are evaluated. Common errors such as use of 90% confidence intervals (CIs), failure to control for reverse causation, and failure to adjust for significant predisposing factors beyond the two that are addressed in the NOS all can result in lower quality methodologic approaches. These are the types of indicators used to create the second tool. To improve transparency, the individual scores for all articles are included in Appendix D (available online only), so readers can assess the study methodology evaluations for themselves.
Evaluation tools such as Grades of Recommendation, Assessment, Development, and Evaluation and others were not used for this study. They lack sufficient granularity to address the wide-range of study designs and subjects involved. As essentially none of the articles reviewed were randomized, controlled, prospective studies, they all would receive lower marks. This makes discrimination between cohort and case control studies using higher and lower quality methodologies difficult. Given all but one included article was either a case control or cohort study, investigators needed evaluations tools that would be effective in scoring these papers. That is why researchers selected the NOS and Lower Quality Indicator Scale.
The most widely cited data supporting the hypothesis that all radiation causes cancer comes from analysis of the LSS. While the data collected in this study are accurate, the linear and linear quadratic interpretation of the relationship between radiation and cancer across all levels of exposure is potentially flawed. In the latest examination of the LSS data by Grant, et al, a linear or curvilinear relationship does exist between increasing doses of radiation and an increased risk of cancer starting above 200 mGy.Reference Grant, Brenner and Sugiyama9 However, as one moves down the graphic line to lower doses of radiation below 200 mGy, a clear risk of increased cancer induction disappears. The graphs depicting the relationship between absorbed dose and cancer risk illustrate this observation (Figure 3). The dashed line illustrating the lower bounds of the 95% confidence interval for excess relative risk for cancer in men remains below zero until past 200 mGy, while that for women does not become positive until past 100 mGy.Reference Grant, Brenner and Sugiyama9 This fairly clearly suggests the possibility of a threshold for cancer risk if these data were evaluated as a separate entity rather than in totality with all the other data. Indeed, the conclusion of this latest LSS study shows no increased cancer risk in men for doses up to 100 mGy (excess relative risk of 0.010 with 95% confidence intervals of −0.0003 to 0.021).Reference Grant, Brenner and Sugiyama9
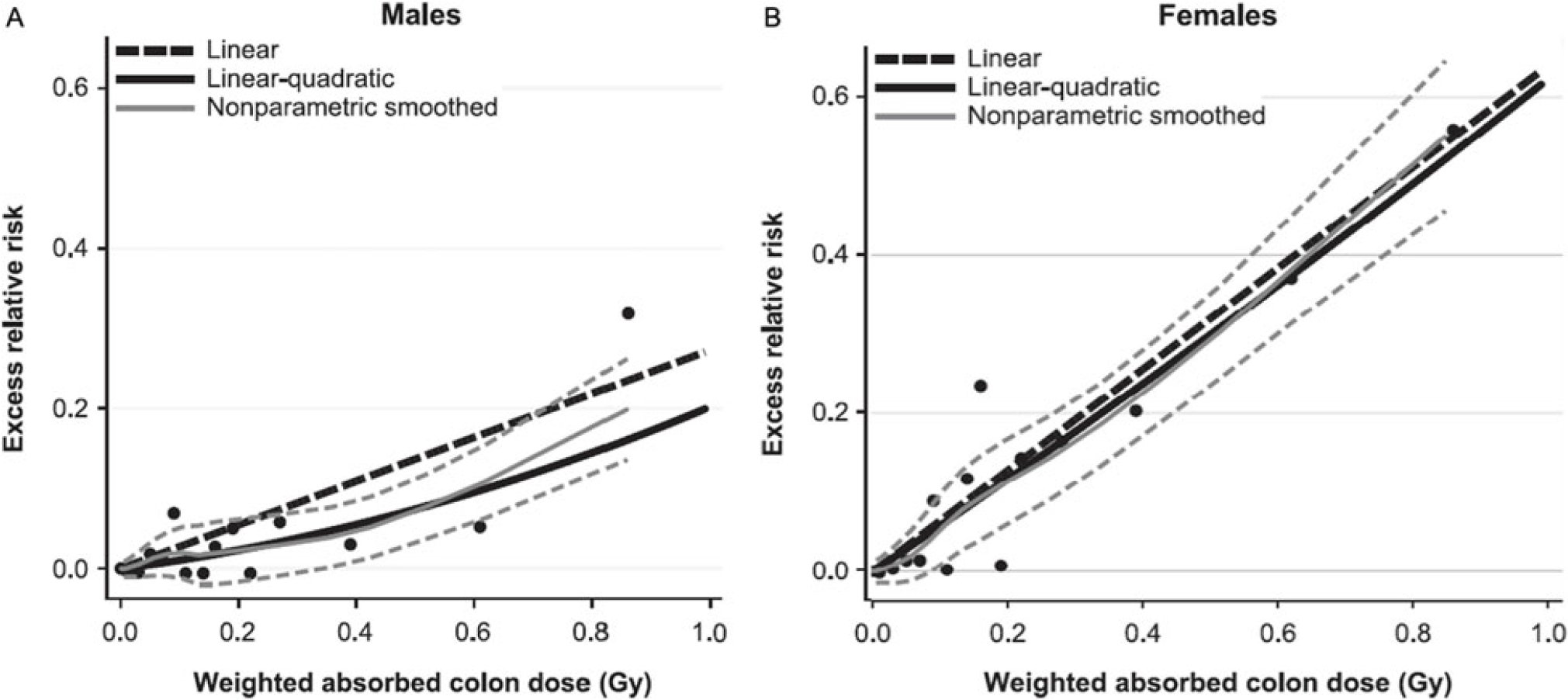
Figure 3. Cancer Risk for Males and Females as a Function of Increasing Radiation Exposure Up to 1 Gy.
It appears possible that two separate phenomena occur across the range of radiation exposure; one manifesting at low doses (100 mSv, possibly extending to 200 mSv) and a second process manifesting at higher doses. This is similar to the impact of sunlight on the human body. Low doses of ultraviolent radiation create vitamin D3 without causing significant skin damage. High doses will cause sunburn and increase the risk of skin cancer. Applying this concept, researchers would need a new objective model that could depict both processes while making no assumptions about the data. In fact, such models exist. Doss, et al used a regression approach in which the radiation dose in Gy was entered into the model using a restricted cubic spline transformation with four knots. This method of data analysis is flexible and allows the line of best fit to cross the Y axis at any location and also allows for negative predicted excess relative risk values. When this model is applied to the data from a previous analysis of the LSS, the 95% CIs for line of best fit are below zero for doses under 200 mGy, clearly demonstrating the possibility of a threshold.Reference Doss, Egleston and Litwin78
Sasaki, et al, concerned about the same issue of constrained data analysis, used a different approach. They employed an artificial neural network to examine the relationship between radiation dose and cancer risk. This method can identify changes in the dose-response relationship and is not limited to one type of graphical representation, allowing the line of best fit to follow the data. Using this model, the authors also identified a threshold effect, with no increased risk for radiation-induced cancer below 100–200 mSv.Reference Sasaki, Tachibana and Takeda22
It might be useful to illustrate the difference found between studies using higher quality and lower quality methodologies by describing several investigations involving the risk of cancer from workplace radiation exposure from sources used to generate nuclear power. Studies concluding no cancer threshold exists suffer from significant flaws, including the use of spurious data, 90% confidence intervals, one-tailed tests of significance, and outcome measures that obscure the presence of a low-dose threshold, such as increased cancer risk per Gy or use of a restricted linear approach for data representation.Reference Richardson, Cardis and Daniels43,Reference Leuraud, Richardson and Cardis44,Reference Cardis, Vrijheid and Blettner55,Reference Preston, Mattsson, Holmberg, Shore, Hildreth and Boice79 Re-analysis of a study using information on occupational radiation exposure in the nuclear power industry from 15 countries that initially found a significant increase in cancer risk from low-dose exposure found no significantly increased risk after spurious Canadian data were excluded.Reference Zablotska, Lane and Thompson21 In contrast, the Johns Hopkins School of Public Health (Baltimore, Maryland USA) conducted a study of cancer incidence in nuclear shipyard workers. This investigation was a rigorously performed search for health risks of radiation to civilian employees of eight shipyards that overhauled and repaired nuclear-propelled US Navy ships and submarines. They compared cancer rates experienced by badged nuclear workers servicing nuclear powered vessels who had exposure to low radiation doses with their colleagues who did not enter the radiation areas and so were not exposed to the low radiation doses. This eliminated any impact from the “healthy worker effect” (the phenomenon that employed individuals are healthier than the general population and so comparisons between the two can lead to inaccurate results). The authors used 95% confidence intervals and non-linear restricted methods of data analysis, addressed such confounders as socio-economic status, and stratified worker analysis by radiation dose. These investigators found no increased risk of cancer from exposure to low-dose radiation.Reference Sponsler and Cameron33
Limitations
Several limitations exist that potentially impact the study results. First, quantitative evaluation of methodologic quality incorporates a certain degree of subjectivity. Some criteria are difficult to objectively describe and so variability can occur in the quantification process. Investigators minimized this by using a scoring template that helped reduce inconsistency and by requiring consensus for each score. Second, the study authors performed the quantitative analysis. While it would be desirable to use another group blinded to the study’s goal, it was not possible to find other emergency physicians that were unbiased. All the physicians contacted candidly admitted either a bias for or against the concept that all radiation causes cancer. None honestly believed that a state of equipoise could exist for either outcome. The authors were the only emergency physicians identified that believed that both outcomes were possible. Since scoring bias could not be completely eliminated, the raw scores for all 62 articles are included in the appendix so readers can assess the scoring accuracy for themselves. Third, some studies, if only modestly-powered, may have failed to identify a significant risk of cancer at lower doses due to the difference in the effect size. If there was a significant, but small, association between low-dose radiation and cancer, this might have required a larger sample to detect than associations at higher doses, reflecting a Type II error. However, this is unlikely as most studies included participants in the tens of thousands. In addition, the LSS just reported no evidence for cancer at doses below 100 mSv in men, after reporting just the opposite for decades. This change reflects results from a now adequately powered study. Fourth, some studies may have yielded data that may not have easily fit within the binary outcomes of “support” or “not support.” However, only three of the 62 articles produced mixed results, and none of these were in the higher quality study group. Even for these investigations, the authors’ conclusions easily placed them into one of the two categories. Fifth, the authors analyzed studies documenting chronic exposure and acute intermittent exposure together. Very limited data exist quantifying the difference between cumulative low-dose exposure and single dose. Given the majority of studies evaluating both exposure types found no increased cancer rates, this is less likely to impact the results. Lastly, the lower quality indicator tool has not been used previously. Nonetheless, all 11 criteria are well-known, validated methodology flaws and are documented in research design textbooks and multiple review articles. It would seem difficult to argue that the results from studies with methods containing three or more of these known flaws could produce a meaningful evaluation.
Conclusion
This investigation demonstrates that a preponderance of studies using higher quality case control and cohort methodology support the existence of a threshold for cancer induction by radiation up to a dose of 100 mSv, and possibly as high as 200 mSv. These findings suggest that the cancer risk from this radiation dose is minimal and exposure to at least 10 CT scans, and possibly as many as 20, is unlikely to cause cancer.
Conflicts of interest/funding
This project did not receive funding from any source. The authors have no conflicts of interest to declare.
Author Contributions
CHS, RF, and LS-LM are responsible for the conception and design of the study. LS-LM programmed the software to search the literature and provided data acquisition. Data analysis and interpretation was done by CHS, RF, and MD. Drafting and revision of the manuscript was performed by CHS, RF, LS-LM, and MD with all authors making substantial contributions. CHS takes responsibility for the manuscript content as a whole.
Supplementary Material
To view supplementary material for this article, please visit https://doi.org/10.1017/S1049023X1900520X