Introduction
The vulnerability of species to extinction has traditionally been assessed within three major areas of study: environment, genetics and population dynamics (Gilpin & Soulé, Reference Gilpin, Soulé and Soulé1986), hereafter referred to as the traditional approach. In this context, the study of environment includes investigating the quality and quantity of available and potential habitat, and thus all aspects of species’ relationships with abiotic and biotic factors. Genetic studies focus on the genetic diversity of species and populations (including adaptation to change) and population bottlenecks from small effective population sizes. Research on population dynamics investigates species’ demography and persistence in a given environment manifested via population structure, fecundity and, ultimately, fitness. The traditional approach has proved successful in studies of threatened and non-threatened plant and animal species (e.g. Oostermeijer et al., Reference Oostermeijer, Luijten and Den Nijs2003; Conard, Reference Conard2009; Kalkvik, Reference Kalkvik2012). However, other components of a more comprehensive assessment of the viability of populations (some of these originally proposed by Gilpin & Soulé, Reference Gilpin, Soulé and Soulé1986), such as species’ functional responses to a changing environment, their ecological interactions, and their role in ecosystem functioning, have not been formally incorporated into conservation research, particularly regarding threatened taxa (Schemske et al., Reference Schemske, Husband, Ruckelshaus, Goodwillie, Parker and Bishop1994). These additional research areas can be unified in a trait-based approach, a recent methodological advance in ecology that is being used to link individual-level functions (measured with functional traits) to species, community and ecosystem processes, to provide mechanistic explanations for the observed patterns, particularly in changing environments (McGill et al., Reference McGill, Enquist, Weiher and Westoby2006; Violle et al., Reference Violle, Navas, Vile, Kazakou, Fortunel, Hummel and Garnier2007; Garnier & Navas, Reference Garnier and Navas2012; Shipley et al., Reference Shipley, De Bello, Cornelissen, Laliberté, Laughlin and Reich2016).
From the perspective of trait-based ecology, a trait is ‘any morphological, physiological or phenological feature measurable at the individual level, from the cell to the whole-organism level, without reference to the environment or any other level of organization’ (Violle et al., Reference Violle, Navas, Vile, Kazakou, Fortunel, Hummel and Garnier2007). These traits are called functional traits when they influence fitness through their effects on growth, reproduction and survival, which are the three components of individual performance (Violle et al., Reference Violle, Navas, Vile, Kazakou, Fortunel, Hummel and Garnier2007). Species traits are usually assessed as mean values of individual traits per population, and such values can vary with the environment. Thus, information on the local environment of populations is needed to interpret the ecological and evolutionary meaning of measured traits and to make useful predictions under changing environmental conditions (McGill et al., Reference McGill, Enquist, Weiher and Westoby2006; Violle et al., Reference Violle, Navas, Vile, Kazakou, Fortunel, Hummel and Garnier2007; Garnier & Navas, Reference Garnier and Navas2012; Shipley et al., Reference Shipley, De Bello, Cornelissen, Laliberté, Laughlin and Reich2016).
Trait-based ecology is a relatively new discipline that is increasingly used in studies of community assembly (e.g. Ackerly & Cornwell, Reference Ackerly and Cornwell2007), biotic interactions (e.g. Wardle et al., Reference Wardle, Barker, Bonner and Nicholson1998) and ecosystem functioning (e.g. Díaz & Cabido, Reference Díaz and Cabido2001), and in predicting species’ responses to climate warming (e.g. Diamond et al., Reference Diamond, Nichols, McCoy, Hirsch, Pelini and Sanders2012). Chown (Reference Chown2012) presented a trait-based framework for use in conservation, specifically in conservation physiology, to forecast the risk of climate and habitat changes to species and higher ecological levels (communities, ecosystems). A major challenge of the trait-based approach to conservation is the identification and measurement of functional traits relevant to individual threatened species, particularly those traits related to responses to changing environments, species’ ecological interactions, and ecosystem functioning. Here we propose and illustrate how a functional trait-based approach can be used to increase our understanding of the vulnerability of threatened species to extinction. We introduce the steps and structure for implementing a trait-based approach in the conservation of threatened species, and illustrate this approach in a case study of Dioon sonorense, an Endangered cycad endemic to north-western Mexico, including a discussion of the potential applicability of the trait-based approach in guiding the conservation of this species.
A trait-based approach to the conservation of threatened plant species
By definition, threatened species are already at risk of extinction as a result of species-specific extrinsic and intrinsic factors. A more complete understanding of species’ vulnerability to extinction requires knowledge not only of their environment, genetics and demography but also of functional traits related to their responses to changing environments, their interactions and their role in ecosystem functioning (Gilpin & Soulé, Reference Gilpin, Soulé and Soulé1986; Oostermeijer et al., Reference Oostermeijer, Luijten and Den Nijs2003; Chown, Reference Chown2012). This information can be translated into more integrative management strategies to increase the viability of threatened species (Fig. 1). We focus on illustrating the conceptual importance of studying functional traits to inform the conservation of threatened species rather than on the methodological peculiarities of measurement and analysis of functional traits, which can be found elsewhere (e.g. Díaz et al., Reference Díaz, Lavorel, De Bello, Quétier, Grigulis and Robson2007; Bernhardt-Römermann et al., Reference Bernhardt-Römermann, Römermann, Nuske, Parth, Klotz, Schmidt and Stadler2008; Kraft & Ackerly, Reference Kraft and Ackerly2010; Pérez-Harguindeguy et al., Reference Pérez-Harguindeguy, Díaz, Garnier, Lavorel, Poorter and Jaureguiberry2013).
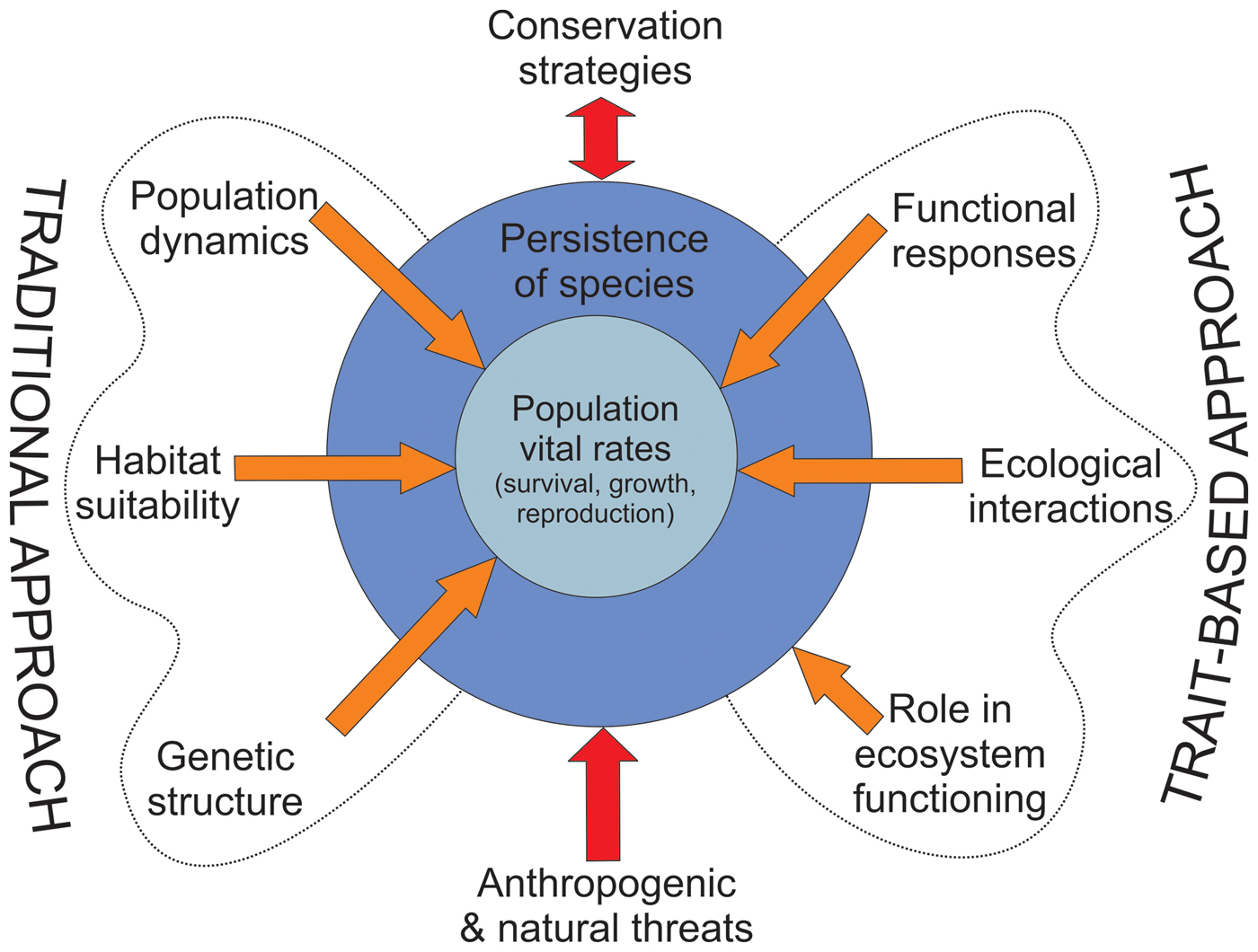
Fig. 1 How the trait-based approach complements the traditional approach to the conservation of threatened species. The traditional approach focuses on species population dynamics, habitat suitability and genetic structure, and has provided essential information for guiding conservation strategies for threatened species. The trait-based approach has been formally included in conservation research to a lesser extent, even though it can provide information on physiological responses to environmental variability and ecological interactions of species, and their roles in ecosystem functioning (which may indirectly influence species persistence). Combining these approaches may lead to more integrative conservation strategies to promote the long-term persistence of target species exposed to anthropogenic and natural threats. There is a dynamic feedback (double arrow) between conservation strategies and species persistence, as they can affect each other.
We synthesized basic information from published studies for both the traditional approach and the trait-based approach, to illustrate how these approaches differ and how they can be useful in guiding the conservation of a target threatened species (Table 1). The information for the trait-based approach can be formalized as a series of distinct and well-defined activities required to gather scientific information on the functional traits and factors most likely to affect population fitness and species persistence, and thus facilitate science-based species conservation (Table 1). Although the collected information should be species-specific, a multi-species framework by phylogenetic group may also be possible when related species display parallel responses to similar threats.
Table 1 Summary of the main steps for implementing a trait-based approach (steps 5–7) that complements the traditional approach (steps 1–4) for the conservation of threatened plant species.

Our case study illustrates how the trait-based approach can provide information to understand (1) the individual physiological responses to environmental stress imposed by water, light and nutrient limitations that may be caused by the changing environment (e.g. Álvarez-Yépiz et al., Reference Álvarez-Yépiz, Búrquez and Dovčiak2014a; Williams et al., Reference Williams, Hultine and Dettman2014; Díaz-Álvarez et al., Reference Díaz-Álvarez, Lindig-Cisneros and de la Barrera2015; Urban, Reference Urban2015), (2) the importance of maintaining species’ ecological interactions (e.g. plant–plant, plant–pollinator/seed disperser, and plant–herbivore), as the loss of these interactions usually results in species extinctions (e.g. Tilman et al., Reference Tilman, May, Lehman and Nowak1994; Álvarez-Yépiz et al., Reference Álvarez-Yépiz, Cueva, Dovčiak, Teece and Yepez2014b; Valiente-Banuet et al., Reference Valiente-Banuet, Aizen, Alcántara, Arroyo, Cocucci and Galetti2015), and (3) the importance of the role of individual species in ecosystems, because threatened species can increase carbon storage, biomass accumulation and ecosystem stability by increasing plant functional diversity, facilitating a broader use of available resources (Lyons et al., Reference Lyons, Brigham, Traut and Schwartz2005; Mouillot et al., Reference Mouillot, Bellwood, Baraloto, Chave, Galzin and Harmelin-Vivien2013; Álvarez-Yépiz & Dovčiak, Reference Álvarez-Yépiz and Dovčiak2015; Leitão et al., Reference Leitão, Zuanon, Villéger, Williams, Baraloto and Fortunel2016).
A trait-based approach for the cycad Dioon sonorense
Description of the target species
Cycads are one of the most threatened groups of vascular plants (Donaldson, Reference Donaldson2003; IUCN, 2017). These gymnosperms evolved c. 300 million years ago and were widespread during the Jurassic but declined dramatically during the mass extinction of the Cretaceous, when they apparently contracted to rocky or nutrient-poor marginal habitats (Norstog & Nicholls, Reference Norstog and Nicholls1997; Crisp & Cook, Reference Crisp and Cook2011; IUCN, 2017). Many cycad species occur in small, isolated populations in tropical and subtropical regions, often in marginal rocky habitats, where they can obtain nitrogen through symbiotic N2-fixing cyanobacteria (Norstog & Nicholls, Reference Norstog and Nicholls1997; Lindblad & Costa, Reference Lindblad and Costa2002; Álvarez-Yépiz et al., Reference Álvarez-Yépiz, Búrquez and Dovčiak2014a).
Dioon sonorense (De Luca, Sabato & Vasquez Torres, Zamiaceae) is a cycad species endemic to the arid lands of north-western Mexico (essentially to the state of Sonora, Fig. 2). It is an evergreen, long-lived understorey species of the northernmost tropical dry forest of the Americas and is valued as an ornamental plant (Plate 1). There are five confirmed localities where this species still occurs, in c. 50 subpopulations, mostly restricted to subtropical vegetation (Fig. 2). Each locality contains 5–20 subpopulations, usually with 10–100 mature individuals each but frequently with < 30 (González-Astorga et al., Reference González-Astorga, Vovides, Cabrera-Toledo and Nicolalde-Morejón2008; Álvarez-Yépiz et al., Reference Álvarez-Yépiz, Dovčiak and Búrquez2011). The total estimated population size is c. 2,400 mature individuals, with an apparent decreasing population trend since the 1960s (Chemnick & Gregory, Reference Chemnick and Gregory2010). Dioon sonorense is dioecious, and female plants under optimal conditions produce a single cone (strobilus) every 2 years, which restricts its regeneration potential and population persistence (Norstog & Nicholls, Reference Norstog and Nicholls1997; Álvarez-Yépiz et al., Reference Álvarez-Yépiz, Dovčiak and Búrquez2011). It is categorized as Endangered on the IUCN Red List (Chemnick & Gregory, Reference Chemnick and Gregory2010). This species is exposed to (1) extrinsic threats, including illegal collecting (seeds, whole plants), land-use change (land clearing, grazing), and extreme natural events (hurricanes, fires, frosts), and (2) intrinsic threats, including small adult population sizes, little or no recruitment to the adult stage, and a distribution restricted to marginal habitats (Norstog & Nicholls, Reference Norstog and Nicholls1997; González-Astorga et al., Reference González-Astorga, Vovides, Cabrera-Toledo and Nicolalde-Morejón2008; Chemnick & Gregory, Reference Chemnick and Gregory2010; Álvarez-Yépiz et al., Reference Álvarez-Yépiz, Dovčiak and Búrquez2011).

Fig. 2 The five confirmed localities (black dots) of the cycad Dioon sonorense in the state of Sonora, Mexico, and one (San Javier) where it is locally extinct; and the potential distribution of the species (hatched, following elevations of 750–1,250 m), based on past and present known occurrences (González-Astorga et al., Reference González-Astorga, Vovides, Cabrera-Toledo and Nicolalde-Morejón2008; Álvarez-Yépiz et al., Reference Álvarez-Yépiz, Dovčiak and Búrquez2011). The species does not occur in the wild elsewhere.

Plate 1 A male individual of the Endangered cycad Dioon sonorense, a long-lived species with a narrow geographical range in north-western Mexico (Fig. 2). Photograph by J.C. Álvarez-Yépiz.
Description of the trait-based approach
Any contributions of a trait-based approach (or any other new approach) should be evaluated in terms of what new information or insight it yields relative to the traditional (or previously used) research approaches. For example, the traditional approach has produced key scientific insights about D. sonorense population dynamics, habitat suitability and genetic structure. A matrix analysis indicated decreasing finite population growth rates (λ < 1) for small populations (< 30 adults; Álvarez-Yépiz et al., Reference Álvarez-Yépiz, Dovčiak and Búrquez2011), with larger population sizes (≥ 50 adults) required for population stability (λ c. 1) or growth (λ > 1). Values of λ of 0.86–1.047 have been reported for other, more abundant cycad species (Raimondo & Donaldson, Reference Raimondo and Donaldson2003; Pérez-Farrera et al., Reference Pérez-Farrera, Vovides, Octavio-Aguilar, González-Astorga, de la Cruz-Rodríguez, Hernández-Jonapá and Villalobos-Méndez2006). Elasticity analysis indicated that adults contribute most to population growth rates (Álvarez-Yépiz et al., Reference Álvarez-Yépiz, Dovčiak and Búrquez2011), similar to other cycads and long-lived plant species in general (Silvertown et al., Reference Silvertown, Franco and Menges1996; Raimondo & Donaldson, Reference Raimondo and Donaldson2003). Habitat analyses indicated that D. sonorense may occur in low abundance on poor soils where angiosperm competition is low, but it can reach higher abundance, recruitment and population growth on more fertile soils where nurse plants can ameliorate heat stress and poor soil conditions (Bertness & Callaway, Reference Bertness and Callaway1994; Álvarez-Yépiz et al., Reference Álvarez-Yépiz, Dovčiak and Búrquez2011, Reference Álvarez-Yépiz, Cueva, Dovčiak, Teece and Yepez2014b). Genetic analyses indicated that the species has a robust genetic structure (high genetic diversity, slightly positive global inbreeding and zero local inbreeding; González-Astorga et al., Reference González-Astorga, Vovides, Cabrera-Toledo and Nicolalde-Morejón2008), and thus a good genetic baseline for conservation.
The traditional approach to conservation has not, however, provided detailed information on the species functional or physiological responses to environmental variability, ecological interactions or the species roles in ecosystem functioning that may influence its persistence. As discussed below, some key traits of D. sonorense that have been identified as important because of their effect on population vital rates and broader ecosystem functions include physiological (e.g. high water-use efficiency and symbiotic N2 fixation, which increase the efficiency of resource use), morphological (e.g. sclerophylly, which decreases water loss and increases protection against herbivory), and phenological traits (e.g. evergreen leaves, which increase nutrient retention).
Functional traits related to water conservation and nutrient acquisition are particularly important in D. sonorense because they can affect population vital rates in the water- and nutrient-limited habitats where this species occurs. Dioon sonorense seems to be able to access more atmospheric nitrogen at sites with low soil fertility through symbiotic associations with cyanobacteria, and this ability increases with ontogeny (Álvarez-Yépiz et al., Reference Álvarez-Yépiz, Búrquez and Dovčiak2014a). Seedlings of D. sonorense (and other species in this genus) are especially vulnerable to seasonal drought, with higher water loss leading to lower water-use efficiency (i.e. the ratio of photosynthesis to transpiration rates) and subsequently higher seedling mortality as a result of desiccation (Álvarez-Yépiz et al., Reference Álvarez-Yépiz, Búrquez and Dovčiak2014a; but see also Yáñez-Espinosa et al., Reference Yáñez-Espinosa, Flores, Millán and Méndez2014; Yáñez-Espinosa & Flores, Reference Yáñez-Espinosa and Flores2016). Sclerophylly in leaves of D. sonorense seedlings is a trait that helps them to reduce water loss (by transpiration) and, at least in seedlings, requires 60 days to develop fully (Álvarez-Yépiz et al., Reference Álvarez-Yépiz, Búrquez and Dovčiak2014a). Therefore, reducing light intensity through artificial shading or protection from nurse plants should increase seedling survival, especially during the first few months of development.
Important ecological interactions of D. sonorense with other species include facilitation by angiosperms, which can be especially important at the seedling stage, when the angiosperm canopy provides shade that reduces transpiration and increases water-use efficiency and seedling survival (Álvarez-Yépiz et al., Reference Álvarez-Yépiz, Búrquez and Dovčiak2014a,Reference Álvarez-Yépiz, Cueva, Dovčiak, Teece and Yepezb; but see also Yáñez-Espinosa et al., Reference Yáñez-Espinosa, Flores, Millán and Méndez2014; Yáñez-Espinosa & Flores, Reference Yáñez-Espinosa and Flores2016). However, the growth and survival of D. sonorense at different life stages vary with the identity of neighbouring plants (Álvarez-Yépiz et al., Reference Álvarez-Yépiz, Cueva, Dovčiak, Teece and Yepez2014b); for example, intraspecific competition is strongest at the seedling stage, especially in sheltered microsites densely colonized by cycad seedlings, whereas interspecific competition is strongest at the adult stage. The type of neighbour (conspecific vs heterospecific) and associated neighbourhood interactions (competition, facilitation) have important conservation implications, as they can be used to guide the selection of appropriate microsites for seedling establishment for species restoration or reintroduction. For example, rocky areas with conspecific canopies tend to facilitate the survival of D. sonorense seedlings by reducing interspecific competition (Álvarez-Yépiz et al., Reference Álvarez-Yépiz, Cueva, Dovčiak, Teece and Yepez2014b), and thus they may be suitable sites for successful conservation or reintroduction efforts attempting to increase the number of populations. Conservation strategies accounting for species interactions may include maintaining or promoting greater canopy cover to protect seedlings from heat and drought stress. However, subsequent management should reduce canopy cover (e.g. via silviculture) to decrease interspecific competition and promote recruitment of juveniles into adults, especially in productive sites with denser canopies. Other biotic interactions that are important in cycads but unknown for D. sonorense include relationships with herbivores, pollinators, seed dispersers and N2-fixing cyanobacteria (Norstog & Nicholls, Reference Norstog and Nicholls1997; Pérez-Farrera et al., Reference Pérez-Farrera, Vovides, Octavio-Aguilar, González-Astorga, de la Cruz-Rodríguez, Hernández-Jonapá and Villalobos-Méndez2006; Snow & Walter, Reference Snow and Walter2007; Hall & Walter, Reference Hall and Walter2013).
Key ecosystem functions such as carbon storage can be disproportionately enhanced locally by threatened species such as D. sonorense (e.g. Álvarez-Yépiz & Dovčiak, Reference Álvarez-Yépiz and Dovčiak2015; Leitão et al., Reference Leitão, Zuanon, Villéger, Williams, Baraloto and Fortunel2016) because rare species often possess unique combinations of functional traits that increase functional diversity and resource use (Lyons et al., Reference Lyons, Brigham, Traut and Schwartz2005; Mouillot et al., Reference Mouillot, Bellwood, Baraloto, Chave, Galzin and Harmelin-Vivien2013; Álvarez-Yépiz et al., Reference Álvarez-Yépiz, Búrquez, Martínez-Yrízar, Teece, Yépez and Dovciak2017). The evergreen and long-lived habit of D. sonorense implies the species contributes to the regional carbon pool and cycling through longer-term carbon storage (for hundreds of years) and slow carbon release. In tropical dry forest stands where D. sonorense occurs, up to 20% of the above-ground biomass has been attributed to this species (Álvarez-Yépiz & Dovčiak, Reference Álvarez-Yépiz and Dovčiak2015). In addition, D. sonorense may facilitate the survival of other species by providing permanent shade as a result of its evergreen nature, reducing heat and drought stress, thus contributing to the maintenance of biodiversity in tropical dry forests (Álvarez-Yépiz et al., Reference Álvarez-Yépiz, Búrquez and Dovčiak2014a,Reference Álvarez-Yépiz, Cueva, Dovčiak, Teece and Yepezb).
Discussion
The trait-based research approach can provide key information to better understand the persistence mechanisms of a threatened species. Examples of relevant trait-based information in our D. sonorense case study highlight that (1) seedlings (especially those with immature leaves, < 60 days old) rather than juveniles or adults are most sensitive to resource limitation and environmental conditions, making seedling functional responses particularly important for species range shifts as a result of changing climate (Álvarez-Yépiz et al., Reference Álvarez-Yépiz, Búrquez and Dovčiak2014a,Reference Álvarez-Yépiz, Cueva, Dovčiak, Teece and Yepezb); (2) relatively low water-use efficiency of seedlings coupled with the lack of microclimatic amelioration by nurse plants contributes to high seedling mortality rates, reduced adult recruitment and consequently reduced population viability (Álvarez-Yépiz et al., Reference Álvarez-Yépiz, Búrquez and Dovčiak2014a,Reference Álvarez-Yépiz, Cueva, Dovčiak, Teece and Yepezb; Yáñez-Espinosa et al., Reference Yáñez-Espinosa, Flores, Millán and Méndez2014; Yáñez-Espinosa & Flores, Reference Yáñez-Espinosa and Flores2016); and (3) large populations with many mature individuals contribute locally to the maintenance of ecosystem function and stability by augmenting long-term carbon storage and functional diversity (Álvarez-Yépiz & Dovčiak, Reference Álvarez-Yépiz and Dovčiak2015).
The information gathered from the trait-based research approach coupled with existing knowledge derived from the traditional approach can help us define more integrative conservation actions. For example, (1) guarding the most favourable habitats, especially where D. sonorense co-occurs with appropriate nurse plants; (2) protecting adult plants across all populations, given their importance for maintaining long-term population viability, genetic and functional diversity, and ecosystem functioning; (3) increasing seedling survival to larger size classes by augmenting canopy shading during early seedling development; (4) managing for lower canopy cover at the juvenile and adult stages to reduce the negative effects of interspecific competition; and (5) producing seedlings of both the threatened target species and its nurse plant species (if necessary) for their reintroduction into suitable habitats, or for augmenting their small natural populations. It may also be necessary to identify and assess the role of local and long-distance dispersers of pollen and seeds, to protect them. All of the above guidelines could be implemented in all five tropical dry forest localities where D. sonorense occurs in Mexico (Fig. 2). However, the habitat of this species is under official protection in only one of the localities, Alamos (through the federal ecological reserve Sierra de Alamos–Rio Cuchujaqui, which is also included in UNESCO's Man and the Biosphere Programme). Paradoxically, the populations that require more active conservation management do not have permanent monitoring, as they occur outside the reserve, thus emphasizing the need to designate new protected areas and to work with local communities to increase public awareness in unprotected areas.
The steps used to implement a trait-based approach coupled with the traditional approach for conservation of D. sonorense may be applicable to other cycads, especially those of the genus Dioon; the 13 species of Dioon in Mexico exhibit similar population dynamics, habitat preferences and responses to threats (Vovides, Reference Vovides1990; Norstog & Nicholls, Reference Norstog and Nicholls1997; Yáñez-Espinosa et al., Reference Yáñez-Espinosa, Flores, Millán and Méndez2014). The value of including the trait-based approach as an additional conservation tool is particularly important considering that, despite the long history of conservation efforts, 21% of all known vascular plant species are currently considered to be threatened with extinction (RBG Kew, 2016; IUCN, 2017). Some taxonomic groups are particularly at risk; for example, 82% of cycad species and 31% of cacti are categorized as threatened on the IUCN Red List (Donaldson, Reference Donaldson2003; Goettsch et al., Reference Goettsch, Hilton-Taylor, Cruz-Piñón, Duffy, Frances and Hernández2015). The trait-based approach can be a valuable methodology for guiding the conservation of threatened species (sensu IUCN, 2012), especially given the freely accessible, extensive global databases of functional traits available for many plant species (Kattge et al., Reference Kattge, Díaz, Lavorel, Prentice, Leadley and Bönisch2011). A comprehensive database of functional traits of threatened species would be particularly helpful in assisting the implementation of the trait-based approach. This approach goes beyond species’ intrinsic values, as it emphasizes the responses of functional species to changing environments, and species’ functions within ecosystems, providing useful new information that complements traditional research approaches to species conservation.
Acknowledgements
JCÁY is grateful for the support from Fulbright-Garcia Robles (#15086997), and Doctoral (#214564) and Postdoctoral (#179045) fellowships from Consejo Nacional de Ciencia y Tecnología. Additional funding was provided by PROFAPI-ITSON, Dence Fellowship, PLACA and Pack summer research grants to JCÁY, and by PAPIIT DGAPA-UNAM #IN207315 to AMY. ABM and AMY thank the Dirección General de Asuntos del Personal Académico–Programa de Apoyos para la Superación del Personal Académico de la Universidad Nacional Autónoma de México for a sabbatical fellowship at the University of Arizona. Enriquena Bustamante provided technical assistance.
Author contributions
Formulation of original idea, writing and revision of article: JCÁY; writing and revision: MD; contribution of ideas, revision of article: AB and AMY.
Conflicts of interest
None.
Ethical standards
This research complied with the Oryx Code of Conduct for authors.