Introduction
The Oligocene/Miocene boundary in the southern North Sea Basin often coincides with an important hiatus related to the Savian phase. The Savian tectonic pulse is believed to be caused by the regional stress regime induced by the Alpine orogeny, but also sea-level changes may have played a role (Wong et al., Reference Wong, Batjes and De Jager2007). Most of the southern North Sea area emerged above sea level (Fig. 1A). In the city of Antwerp in the western Campine area (Fig. 1B), this hiatus spans the (late) Chattian (late Oligocene) to early Burdigalian (early Miocene; Louwye, Reference Louwye2005). A recent publication by Munsterman & Deckers (Reference Munsterman and Deckers2020) showed that the range of this hiatus decreases from the western Campine area towards boreholes in the eastern Campine area, where it coincides with a gravel layer within shallow marine glauconitic sands of the Veldhoven Formation. Even further to the east, in boreholes in the northern Roer Valley Graben (RVG; Fig. 1B), Munsterman et al. (Reference Munsterman, ten Veen, Menkovic, Deckers, Witmans, Verhaegen, Kerstholt-Boegehold, van de Ven and Busschers2019) did not find indications for a major Oligocene/Miocene hiatus within the Veldhoven Formation (Fig. 2). This is consistent with renewed activity of the RVG since the start of the late Oligocene along a well-pronounced fault (zone), which enabled continuous sedimentation across the Oligocene/Miocene boundary compared to more discontinuous sedimentation with hiatus(es) in the Campine area along its western flank.
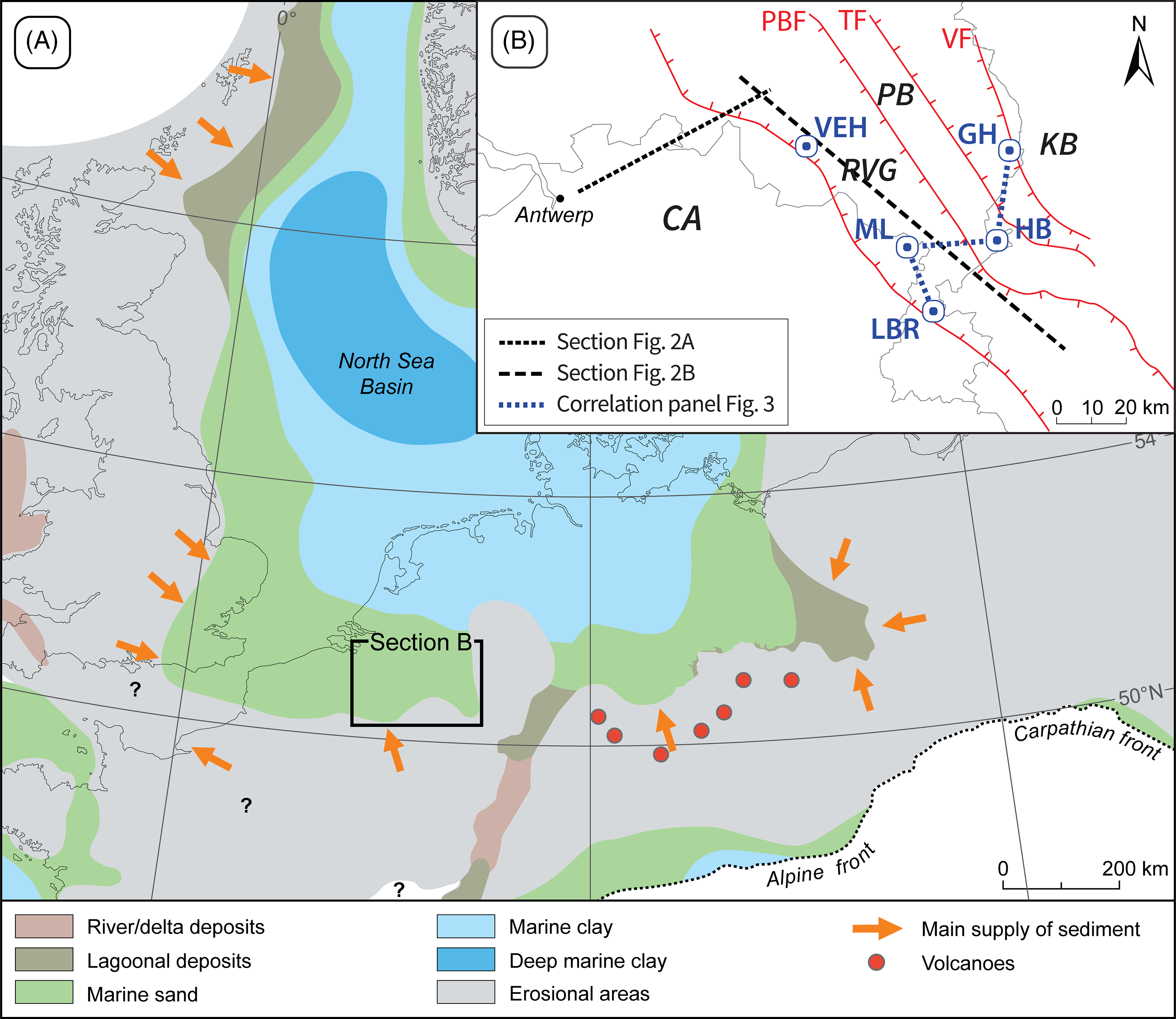
Fig. 1. (A) The late Oligocene (middle Chattian) palaeogeography of the North Sea Basin and surrounding areas, modified from Gibbard & Lewin (Reference Gibbard and Lewin2016). (B) The study area with the main structural elements of the Roer Valley Rift System. The location of the schematic cross-sections of Fig. 2A and 2B are shown with the two black dotted lines and the correlation panel of Fig. 3 is shown as a blue dotted line. CA, Campine area; GH, Groote Heide borehole; HB, Herkenbosch borehole; ML, Molenbeersel borehole; LBR, Limbricht borehole; KB, Krefelt Block; PB, Peel Block; RVG, Roer Valley Graben; VG, Venlo Graben; PBF, Peel Boundary Fault, TF, Tegelen Fault, VF, Viersen Fault. The fault lines were modified after Bense et al. (Reference Bense, Van Den Berg and Van Balen2003).

Fig. 2. Schematic cross-section of the upper Oligocene to middle Miocene lithostratigraphy and chronostratigraphy. (A) from the Antwerp area (left side), across the Campine area towards the northern Roer Valley Graben (RVG) (right side). (B) Along the RVG, from north (left side) to south (right side). The lithostratigraphy is modified after Van Adrichem Boogaert & Kouwe (Reference Van Adrichem Boogaert and Kouwe1993–1997), Deckers and Louwye (Reference Deckers and Louwye2019) and Munsterman et al. (Reference Munsterman, ten Veen, Menkovic, Deckers, Witmans, Verhaegen, Kerstholt-Boegehold, van de Ven and Busschers2019). The location of these cross-sections is shown on Fig. 1B. The NSO dinocyst zones are those of Van Simaeys et al. (Reference Van Simaeys, Munsterman and Brinkhuis2005), while the M-dinocysts zones are those of Munsterman et al. (Reference Munsterman, ten Veen, Menkovic, Deckers, Witmans, Verhaegen, Kerstholt-Boegehold, van de Ven and Busschers2019).
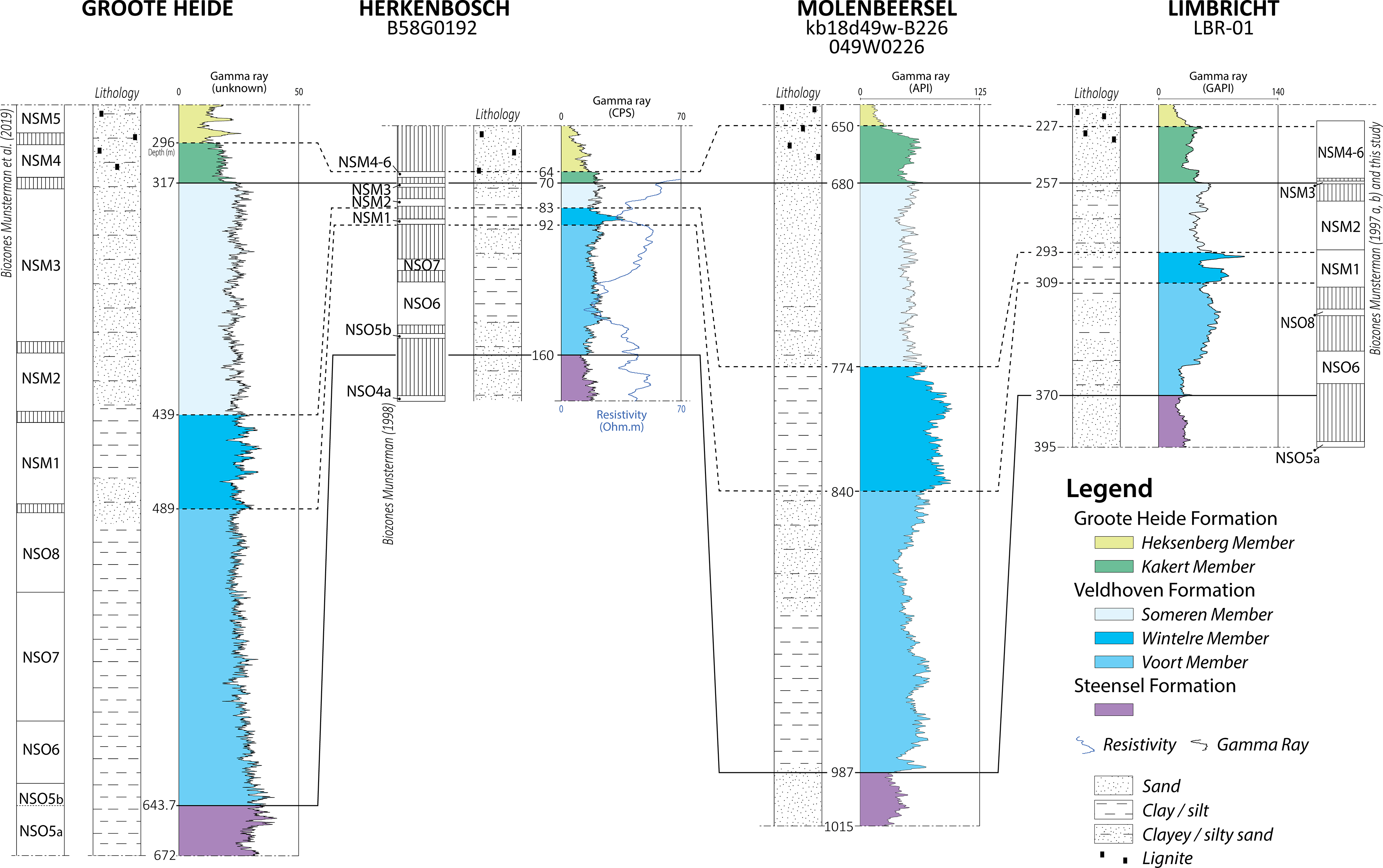
Fig. 3. Correlation panel between the gamma-ray log of the analysed boreholes by this study in the central Roer Valley Rift System. The (NSM and NSO) dinocyst biozones as interpreted by Munsterman et al. (Reference Munsterman, ten Veen, Menkovic, Deckers, Witmans, Verhaegen, Kerstholt-Boegehold, van de Ven and Busschers2019) for the Groote Heide borehole, by Munsterman (Reference Munsterman1997a, b) and this study for the Limbricht borehole and by Munsterman (Reference Munsterman1998) for the Herkenbosch borehole are shown next to the gamma- (and resistivity-)logs of these boreholes. The location of this panel is indicated on Fig. 1B.
In the Miocene, subsidence shifted towards the northern RVG (Michon et al., Reference Michon, Van Balen, Merle and Pagnier2003) and sedimentation became more proximal in a south-eastern direction (Hager et al., Reference Hager, Vandenberghe, Van den Bosch, Abraham, Von der Hocht, Rescher, Laga, Nickel, Verstrealen, Leroi and Van Leeuwen1998), which may give rise to larger sedimentary hiatuses in the central and southern RVG. One of the few boreholes that penetrates the Oligocene/Miocene boundary in the central RVG is the Molenbeersel borehole (DOV kb18d49w-B226, BGD 049W0226; Fig. 1B), which unfortunately lacks age information. Estimates of possible hiatuses in the Veldhoven Formation of this key borehole could therefore only be indirectly derived from log-interpretations and correlations with similar stratigraphic successions that were biostatigraphically analysed in nearby areas (Hager et al., Reference Hager, Vandenberghe, Van den Bosch, Abraham, Von der Hocht, Rescher, Laga, Nickel, Verstrealen, Leroi and Van Leeuwen1998; Verbeek et al., Reference Verbeek, De Leeuw, Parker and Wong2002; Deckers, Reference Deckers2016). The uncertainty of these indirectly derived age interpretations is expressed by the different views that each of the above-mentioned authors has on the age of the Veldhoven Formation and its members in this borehole. This is unfortunate, as it not only causes major uncertainty on the late Oligocene to early Miocene depositional evolution but also the tectonic evolution of the central RVG, as Deckers (Reference Deckers2016) identified an important change in fault kinematics in this stratigraphic interval of the Molenbeersel borehole.
The Veldhoven Formation, originally defined by NAM & RGD (1980), was amended by Van Adrichem Boogaert & Kouwe (Reference Van Adrichem Boogaert and Kouwe1993–1997) to include a coarsening upward sequence previously considered part of the former Breda Formation (now separated by the mid-Miocene Unconformity – MMU – into the formations of Groote Heide and Diessen by Munsterman et al., Reference Munsterman, ten Veen, Menkovic, Deckers, Witmans, Verhaegen, Kerstholt-Boegehold, van de Ven and Busschers2019). The Someren Member was introduced as a new member to accommodate the sands and sandy clays that constitute the upper part of the Veldhoven Formation in the south-eastern Netherlands (Van Adrichem Boogaert & Kouwe, Reference Van Adrichem Boogaert and Kouwe1993–1997). The Veldhoven Clay Member (middle unit of the Veldhoven Formation) originally also defined by NAM & RGD (1980) is recently renamed (to avoid confusion with the same name for the formation) in the Wintelre Member by the Dutch Stratigraphic Commission and officially published in the Dutch Stratigraphic Nomenclator online (Stratigraphic Nomenclature of the Netherlands online, 2021; June 2021).
The Chattian succession in Belgium was recorded in the past century by correlation of their mollusc faunas with the type Chattian in Germany (Schmitz & Stainier, Reference Schmitz and Stainier1909; Stainier, Reference Stainier1911). Consequently, the Voort Formation, for example in the Campine area, was established and assigned to a Chattian age. This succession becomes thicker in the RVG (borehole Molenbeersel), showing a more continuously development in clayey and sandy units above the Voort Sands. Lithostratigraphically, this sequence is similar to the more extensively studied Veldhoven Formation in the Netherlands, where biostratigraphy revealed that the Veldhoven Formation grades into the early Miocene, crossing the Chattian-Aquitanian boundary, and is separated from middle Miocene deposits by the Early Miocene Unconformity (EMU; Munsterman et al., Reference Munsterman, ten Veen, Menkovic, Deckers, Witmans, Verhaegen, Kerstholt-Boegehold, van de Ven and Busschers2019). Hence, it was proposed to harmonise Belgian and Dutch stratigraphic nomenclatures, making the more complete Veldhoven Formation applicable both in the Campine area and the Belgian and Dutch RVG (Dusar & Vandenberghe, Reference Dusar and Vandenberghe2020). After this new classification scheme, the Belgian Voort Formation became the Voort Member as the lower part of the Veldhoven Formation, of which the middle Wintelre clayey and upper Someren sandy members are only recognised in the RVG (Dusar & Vandenberghe, Reference Dusar and Vandenberghe2020).
Two boreholes located south and north the Molenbeersel borehole, respectively, that are provided with biostratigraphic age interpretations and log data of the Veldhoven Formation are the Limbricht (NLOG code: LBR-01) and Herkenbosch (Dinoloket code: B58G0192) boreholes (Fig. 1B). These boreholes were analysed for their content of dinoflagellate cysts by Munsterman (Reference Munsterman1997a, b) and Munsterman (Reference Munsterman1998), respectively. The aims of this study are (1) to update the results of Munsterman (Reference Munsterman1997a, b) and Munsterman (Reference Munsterman1998) to the biozonation currently in use (Munsterman et al., Reference Munsterman, ten Veen, Menkovic, Deckers, Witmans, Verhaegen, Kerstholt-Boegehold, van de Ven and Busschers2019), (2) to perform additional dinoflagellate cyst analyses for better insight in the late Oligocene to early Miocene depositional evolution of the Veldhoven Formation in the central RVG at the location of these boreholes, and (3) to correlate the studied boreholes with the previously biostratigraphically analysed Groote Heide borehole (Dinoloket code: B58F0064) in the Venlo Graben, located to the north of the Herkenbosch borehole, and with the key Molenbeersel borehole in the central RVG to provide a better understanding of the late Oligocene to early Miocene depositional environments in the area of the Roer Valley Rift System.
Geological background
The Roer Valley Rift System developed as a north-west trending branch of the Rhine-Graben-System (Ziegler, Reference Ziegler1994) from the late Oligocene onwards, throughout the south-eastern part of the Netherlands, the northeastern part of Belgium and adjacent parts of Germany. In the Netherlands and Belgium, the Roer Valley Rift System can be subdivided into the Campine Block in the west, the RVG in the centre and the Peel High and Venlo Graben in the east (Fig. 1B). These blocks are bounded by major NW-SE striking fault systems. The Campine Block is separated from the differentially subsiding RVG by the Feldbiss Fault System (Dusar et al., Reference Dusar, Rijpens, Sintubin and Wouters2001). The Peel High is a horst block (relatively high platform, Van den Berg, Reference Van den Berg1994) that is separated from the RVG by the Peel Boundary Fault and from the Venlo Graben by the Tegelen Fault (Van Adrichem Boogaert & Kouwe, Reference Van Adrichem Boogaert and Kouwe1993–1997). The Viersen Fault is the principal displacement zone that separates the Venlo Graben and the northeastern Krefeld Block (Munsterman & Brinkhuis, Reference Munsterman and Brinkhuis2004). The RVG itself is about 30 km wide and 130 km long and is bounded by NW-SE striking border fault systems with vertical throws of several hundred metres. Subsidence strongly increased in the RVG during the Chattian initial rifting (Demyttenaere, Reference Demyttenaere1989; Geluk, Reference Geluk1990). The start of the Chattian also marks an important decrease in sea level that resulted in a shift in lithology from the clayey silts of the Steensel Member in the late Rupelian to the glauconite- and fossil-rich sands of the Voort Member (Van Simaeys, Reference Van Simaeys2004; Van Simaeys et al., Reference Van Simaeys, De Man, Vandenberghe, Brinkhuis and Steurbaut2004; De Man et al., Reference De Man, Van Simaeys, Vandenberghe, Harris and Wampler2010), which forms the lower part of the Veldhoven Formation (Fig. 2). The base of the Voort Member is characterised by marginal, brackish-marine conditions, with water depths not exceeding 20 m, while higher up in the Chattian successions, conditions return to a normal marine environment (De Man & Van Simaeys, Reference De Man and Van Simaeys2004). In large parts of the Campine area, the (upper) Chattian and Aquitanian strata are represented by a hiatus (Louwye, Reference Louwye2005) that coincides with a gravel layer (Munsterman & Deckers, Reference Munsterman and Deckers2020; Fig. 2A). At the base of the Miocene in the Limburg Campine (Schmitz & Stainier, Reference Schmitz and Stainier1909), at the base of the Bolderberg (Halet, Reference Halet1920), and at the base of the Kakert Member (Van Staalduinen & Zagwijn, Reference Zagwijn and Van Staalduinen1975; Kuyl, Reference Kuyl1980), this gravel bed is called the Elsloo Gravel Bed. This hiatus is known from large parts of the North Sea Basin and is related to the Savian tectonic phase (Utescher et al., Reference Utescher, Mosbrugger and Ashraf2000, Wong et al., Reference Wong, Parker and Horst2001; Verbeek et al., Reference Verbeek, De Leeuw, Parker and Wong2002; Munsterman & Brinkhuis, Reference Munsterman and Brinkhuis2004; Knox et al., Reference Knox, Bosch, Rasmussen, Heilmann-Clausen, Hiss, De Lugt, Kasińksi, King, Köthe, Słodkowska, Standke, Vandenberghe, Doornenbal and Stevenson2010, Rasmussen et al., Reference Rasmussen, Dybkjær and Piasecki2010; Eidvin et al., Reference Eidvin, Fridtjof and Rasmussen2014; King et al., Reference King, Gale and Barry2016; Dybkjær et al., Reference Dybkjær, Rasmussen, Eidvin, Grøsfjeld, Riis, Piasecki and Śliwińska2020). At this boundary, an important change in the direction of maximum extension took place, which also coincides with an important shift in depocenter towards the northern RVG (Michon et al., Reference Michon, Van Balen, Merle and Pagnier2003) and the stronger subsidence along the larger fault systems at the expense of the smaller ones in the RVG (Deckers, Reference Deckers2016). Around the Oligocene/Miocene (O/M) boundary, a phase of high δ18O occurred, interpreted as a brief period of Antarctic ice sheet expansion, associated with global cooling (Steinthorsdottir et al., Reference Steinthorsdottir, Coxall, De Boer, Huber, Barbolini, Bradshaw, Burls, Feakins, Gasson, Henderiks, Holbourn, Kiel, Kohn, Knorr, Kürschner, Lear, Liebrand, Lunt, Mörs, Pearson, Pound, Stoll and Strömberg2020). The δ18O maximum is referred to as Mi-1 (Miller et al., Reference Miller, Wright and Fairbanks1991) or O/M (Climate) Transition (OMT; Beddow et al., Reference Beddow, Liebrand, Wilson, Hilgen, Sluijs, Wade and Lourens2018). Current studies show that the highest δ18O values occur in the latest Oligocene (Liebrand et al., Reference Liebrand, Beddow, Lourens, Pälike, Raffi and Bohaty2016). The event is recorded at several locations world-wide, indicating that cooling can be correlated on a global scale. A climatic deterioration is supported by investigations of successions around Antarctica, which point to ice sheets covering large parts of Antarctica around the O/M transition (e.g. Kennett, Reference Kennett1977; Naish et al., Reference Naish, Wilson, Dunbar and Barret2008; Barret, Reference Barret, Hambrey, Christoffersen, Glasser and Hubbard2009). The storage of water into ice resulted in a pronounced eustatic sea-level fall of 50–70 m at this time (e.g. Haq et al., Reference Haq, Hardenbol and Vail1987; Miller et al., Reference Miller, Kominz, Browning, Wright, Mountain, Katz, Sugarman, Cramer, Christie-Blick and Pekar2005). The North Sea Basin probably became a (semi-)enclosed basin during the latest Oligocene-earliest Miocene, with a narrow connection to the North Atlantic Ocean between the Shetland Isles and Norway (Rasmussen et al., Reference Rasmussen, Heilmann-Clausen, Waagstein and Eidvin2008; Knox et al., Reference Knox, Bosch, Rasmussen, Heilmann-Clausen, Hiss, De Lugt, Kasińksi, King, Köthe, Słodkowska, Standke, Vandenberghe, Doornenbal and Stevenson2010). In the latest Chattian, a shallowing occurred, probably reflecting the OMT glaciation event, as was also recorded in Denmark (Dybkjær & Rasmussen, Reference Dybkjær and Rasmussen2007; Dybkjær et al., Reference Dybkjær, King and Sheldon2012). The last occurrences of the dinoflagellate cysts Distatodinium biffii and Chiropteridium spp. were used to identify the broader O/M boundary interval. This interval is referred to Chiropteridium galea dinocyst Zone in Denmark and Zone SNSM1 in the Netherlands. In very shallow marine settings, that is along the southern boundary of the North Sea Basin, this sea-level fall caused unconformities. The range of the Oligocene/Miocene hiatus decreases from west to east across the Campine area (Munsterman & Deckers, Reference Munsterman and Deckers2020; Fig. 2A). In the northern RVG, this hiatus is absent or of limited extent, as the Voort Member (c. 27.3–23.4 Ma) here is overlain by the uppermost Chattian to lower Aquitanian clays of the Wintelre Member (c. 23.4–21.5 Ma; Munsterman et al., Reference Munsterman, ten Veen, Menkovic, Deckers, Witmans, Verhaegen, Kerstholt-Boegehold, van de Ven and Busschers2019). During the late Aquitanian to middle Burdigalian, the Wintelre Member was transgressed and covered by the glauconitic sands of the Someren Member (c. 21.5–17.5 Ma), the uppermost member of the Veldhoven Formation. Contrary to the Wintelre Member, the age equivalent deposits to the Someren Member in the RVG, included in the lower part of the Berchem Formation (Fig. 2), covers most of the Campine area.
During the late Burdigalian, the Veldhoven Formation was overlain by the Groote Heide Formation (Munsterman et al., Reference Munsterman, ten Veen, Menkovic, Deckers, Witmans, Verhaegen, Kerstholt-Boegehold, van de Ven and Busschers2019; Fig. 2). In the distal northern RVG, the Groote Heide Formation consists of homogeneous sequences of glauconite-rich sands that were deposited up to late in the Serravallian. In the more near-shore central RVG, it represents more heterogeneous sequences of the upper Burdigalian silty, glauconitic sands of the Kakert Member, followed by Langhian glauconite-poor and lignite-rich sands of the Heksenberg Member and finally the return of glauconite and decrease of lignite into the Serravallian Vrijherenberg Member (Munsterman et al., Reference Munsterman, ten Veen, Menkovic, Deckers, Witmans, Verhaegen, Kerstholt-Boegehold, van de Ven and Busschers2019; Fig. 2B).
Dataset and methodology
Dataset and correlations
The Limbricht borehole is located in the western flank of the intra-graben area of the central RVG (Fig. 1B). The Herkenbosch borehole is situated further north on the south-eastern part of the Peel High. Besides of lithological descriptions, both boreholes are provided with log data (Fig. 3). A schematic column of the lithological development of the successions (including photos of the sample material) in the boreholes, and interpreted lithostratigraphy, used for this study are publicly provided on internet by the Geological Survey of the Netherlands (DINOloket, n.d.; NLOG, n.d.). Detailed lithological descriptions of air-lifted (semi-cored) boreholes Groote Heide and Herkenbosch are also studied and available on request (DINOloket). For the purpose of this study, gamma-ray log patterns of the successions will be leading. The log data are cross-validated against lithological logs for consistency. Munsterman (Reference Munsterman1997a) and Munsterman (Reference Munsterman1998) performed dinoflagellate cyst analyses on some of the samples from the Oligocene to middle Miocene succession in the Limbricht and Herkenbosch boreholes (palynological reports, including number and type of samples used are available on NLOG). For the interval around the Oligocene/Miocene boundary in the Limbricht borehole, Munsterman (Reference Munsterman1997b) made a revision of the results of Munsterman (Reference Munsterman1997a: see report on NLOG).
Based on these data, a dinoflagellate cyst zonation scheme (M zones) was defined for the Miocene in the southern North Sea Basin by Munsterman & Brinkhuis (Reference Munsterman and Brinkhuis2004). For the purpose of the present study, the results by Munsterman (Reference Munsterman1997a, b) and Munsterman (Reference Munsterman1998) were integrated in the updated version of this dinoflagellate cyst zonation (Munsterman et al., Reference Munsterman, ten Veen, Menkovic, Deckers, Witmans, Verhaegen, Kerstholt-Boegehold, van de Ven and Busschers2019). In addition, the interval between 244.5 and 271.5 m depth in the Limbricht borehole was resampled and the dinoflagellate cyst content was reanalysed for the purpose of the present study.
The gamma-ray logs of the Limbricht and Herkenbosch boreholes are correlated with those of the Groote Heide and Molenbeersel boreholes (Fig. 3). The Groote Heide borehole is located in the Venlo Graben and was selected because it was analysed biostratigraphically (based on dinoflagellate cysts) for the Oligocene and Miocene interval by Munsterman et al. (Reference Munsterman, ten Veen, Menkovic, Deckers, Witmans, Verhaegen, Kerstholt-Boegehold, van de Ven and Busschers2019). The Molenbeersel borehole is located in the central RVG and was selected because it is provided with log data over the thick upper Oligocene and Miocene sedimentary successions, crossed by seismic lines and was used as a key borehole in a large number of studies on the sedimentary and tectonic evolution of the central RVG (Demyttenaere & Laga, Reference Demyttenaere and Laga1988; Hager et al., Reference Hager, Vandenberghe, Van den Bosch, Abraham, Von der Hocht, Rescher, Laga, Nickel, Verstrealen, Leroi and Van Leeuwen1998; Verbeek et al., Reference Verbeek, De Leeuw, Parker and Wong2002; Vandenberghe et al., Reference Vandenberghe, Van Simaeys, Steurbaut, Jagt and Felder2004; Deckers, Reference Deckers2016; Deckers & Munsterman, Reference Deckers and Munsterman2020; Dusar & Vandenberghe, Reference Dusar and Vandenberghe2020).
Finally, well Veldhoven-1 (VEH-01) in northern part of the RVG is added, because it comprises the type section of the Veldhoven Formation [see Van Adrichem Boogaert & Kouwe (Reference Van Adrichem Boogaert and Kouwe1993–1997, Annex I–6), for spontaneous potential (SP) and resistivity (RES) logs and lithological development; DINOloket and Munsterman (Reference Munsterman2001) for palynological report (NLOG)].
Dinoflagellate cyst analyses and zonation
Organic-walled dinoflagellate cysts analysis was utilised to support the interpretations of the Limbricht and Herkenbosch boreholes. This type of analysis led to significant improvements in dating and correlation of Neogene successions in NW Europe and beyond, and in understanding their palaeoenvironmental setting (e.g. Powell, Reference Powell1986, Reference Powell and Powell1992; Head et al., Reference Head, Norris and Mudie1989; Brinkhuis et al., Reference Brinkhuis, Powell, Zevenboom, Head and Wrenn1992; Zevenboom, Reference Zevenboom1995; De Verteuil & Norris, Reference De Verteuil and Norris1996; Head, Reference Head, Van Kolfschoten and Gibbard1998; Dybkjær & Rasmussen, Reference Dybkjær and Rasmussen2000; Louwye, Reference Louwye2002; Louwye et al., Reference Louwye, Head and De Schepper2004; Köthe, Reference Köthe2007; Köthe et al., Reference Köthe, Gaedicke and Lutz2008; De Schepper & Head, Reference De Schepper and Head2009; Dybkjær & Piasecki, Reference Dybkjær and Piasecki2010; Anthonissen, Reference Anthonissen2012; Quaijtaal et al., Reference Quaijtaal, Donders, Persicoc and Louwye2014; De Schepper et al., Reference De Schepper, Schreck, Beck, Matthiessen, Fahl and Mangerud2015, Reference De Schepper, Beck and Mangerud2017; Dybkjær et al., Reference Dybkjær, Rasmussen, Śliwińska, Esbensen and Mathiesen2019; Dybkjær et al., Reference Dybkjær, Rasmussen, Eidvin, Grøsfjeld, Riis, Piasecki and Śliwińska2020). Figure 4 schematically displays the depositional environments used as standard for interpretations in this paper.

Fig. 4. Schematically displayed depositional environments used as standard for interpretations in this paper (modified after Munsterman et al., Reference Munsterman, Verreussel, Mijnlieff, Witmans, Kerstholt-Boegehold and Abbink2012; Verreussel et al., Reference Verreussel, Bouroullec, Munsterman, Dybkjær, Geel, Houben and Kerstholt-Boegehold2018). At the base of this scheme, the extent of the depositional environments inferred from palynological analysis are compared with the detailed depositional setting, as can be inferred for instance from core description analysis.
The potential of dinoflagellate cyst analyses in (bio)stratigraphic differentiation for upper Palaeogene and Neogene successions in the Netherlands has been proven (e.g. Munsterman & Brinkhuis, Reference Munsterman and Brinkhuis2004; Van Simaeys et al., Reference Van Simaeys, Munsterman and Brinkhuis2005; Munsterman et al., Reference Munsterman, ten Veen, Menkovic, Deckers, Witmans, Verhaegen, Kerstholt-Boegehold, van de Ven and Busschers2019). Standard palynological techniques, including HCL and HF digestion, and 15 μm sieving, were applied. No oxidation was used. The slides were mounted in glycerine jelly. Dinoflagellate cyst taxonomy is according to that cited in Fensome et al. (Reference Fensome, Williams and MacRae2019). One microscope slide per sample was counted until a minimum of 200 palynomorphs (spores, pollen, and dinoflagellate cysts) had been identified. The remainder of the slides were scanned for rare taxa. Miscellaneous fossils (e.g. Pediastrum, Botryococcus) were also counted, but kept outside the total sum of 200 specimens.
The Miocene dinoflagellate cyst zonation is based on Munsterman & Brinkhuis (Reference Munsterman and Brinkhuis2004), recalibrated to the Geological Time Scale of Ogg et al. (Reference Ogg, Ogg and Gradstein2016) by Munsterman et al. (Reference Munsterman, ten Veen, Menkovic, Deckers, Witmans, Verhaegen, Kerstholt-Boegehold, van de Ven and Busschers2019; Fig. 5). This zonation is based on consistent dinoflagellate cyst events (mainly on last occurrence datums) from available peer-reviewed palynological contributions from NW Europe and also uses a global compilation calibrated to palaeomagnetic, calcareous plankton and/or foraminifera/bolboforma stratigraphy (Zevenboom, Reference Zevenboom1995; De Verteuil and Norris, Reference De Verteuil and Norris1996; Van Leeuwen, Reference Van Leeuwen2000; and references therein). The age assessments have been cross-validated by correlation to NW European sea-level fluctuations (Hardenbol et al., Reference Hardenbol, Thierry, Farley, Jacquin, De Graciansky, Vail, Graciansky, Hardenbol, Jacquin and Vail1998; Munsterman & Brinkhuis, Reference Munsterman and Brinkhuis2004). For the Oligocene the dinoflagellate cyst zonation North Sea Oligocene (NSO) scheme of Van Simaeys et al. (Reference Van Simaeys, Munsterman and Brinkhuis2005) was used (Fig. 5).

Fig. 5. Composition of relevant parts of dinoflagellate cyst zonation schemes of Munsterman et al. (Reference Munsterman, ten Veen, Menkovic, Deckers, Witmans, Verhaegen, Kerstholt-Boegehold, van de Ven and Busschers2019) and Van Simaeys et al. (Reference Van Simaeys, Munsterman and Brinkhuis2005).
Results
Borehole Limbricht-01 (RVG; Fig. 3)
Age assessment
The palynological (dinoflagellate cyst) analyses for the samples at different measured depths along the Limbricht borehole indicated the following results (Table 1):
Table 1. Limbricht-01 borehole.

The dating is based on:
*1: Last occurrence datums (LOD’s) of Distatodinium paradoxum and Apteodinium spiridoides both at 224.5 m (Munsterman et al., Reference Munsterman, ten Veen, Menkovic, Deckers, Witmans, Verhaegen, Kerstholt-Boegehold, van de Ven and Busschers2019).
*2: The occurrences of several Cordosphaeridium cantharellum at 255 m (Munsterman & Brinkhuis, Reference Munsterman and Brinkhuis2004; Dybkjær & Piasecki, Reference Dybkjær and Piasecki2010).
*3: LOD of Ectosphaeridium picenum at 266 m (Zevenboom, Reference Zevenboom1995; Munsterman et al., Reference Munsterman, ten Veen, Menkovic, Deckers, Witmans, Verhaegen, Kerstholt-Boegehold, van de Ven and Busschers2019).
*4: LOD of Thalassiphora pelagica at 271.5 m (Dybkjær & Piasecki, Reference Dybkjær and Piasecki2010).
*5: The presence of dinoflagellate cyst Homotryblium vallum at 283 m (De Verteuil & Norris, Reference De Verteuil and Norris1996; Dybkjær & Piasecki, Reference Dybkjær and Piasecki2010; Munsterman et al., Reference Munsterman, ten Veen, Menkovic, Deckers, Witmans, Verhaegen, Kerstholt-Boegehold, van de Ven and Busschers2019).
*6: LOD of Chiropteridium galea at 291.5 m (De Verteuil & Norris, Reference De Verteuil and Norris1996; Munsterman et al., Reference Munsterman, ten Veen, Menkovic, Deckers, Witmans, Verhaegen, Kerstholt-Boegehold, van de Ven and Busschers2019). The LOD of Hystrichokolpoma pseudooceanica at 291.5 m confirms the age assessment (Zevenboom, Reference Zevenboom1995). Reworking (Deflandrea phosphorica, Enneadocysta pectiniformis, Wetzeliella spp.) from the Rupelian is recorded.
*7: LOD of Distatodinium biffii at 322.5 m (Brinkhuis et al., Reference Brinkhuis, Powell, Zevenboom, Head and Wrenn1992; Van Simaeys et al., Reference Van Simaeys, Munsterman and Brinkhuis2005; Dybkjær & Piasecki, Reference Dybkjær and Piasecki2010).
*8: LOD’s of Areoligera semicirculata at 344.5 m, Deflandrea phosphoritica at 357.5 m, Glaphyrocysta cf. semitecta at 344.5 m and Wetzeliella symmetrica at 344.5 m (Van Simaeys et al., Reference Van Simaeys, Munsterman and Brinkhuis2005; Sliwinska, Reference Sliwinska2019). At the base of the current interval, 357.5–361.5 m, reworking from Late Jurassic – Early Cretaceous successions (Chlamydophorella nyei, Dingodinium cerviculum, Gochteodinium mutabilis and Perisseiasphaeridium spp.) is noticed.
*9: LOD of Achilleodinium biformoides at 392 m (Stover and Hardenbol, Reference Stover and Hardenbol1994).
Stratigraphy, log patterns and depositional environments
The low gamma-ray values of the Steensel Member on top of the high gamma-ray values of the Boom Member are a good reference for the identification of these Rupelian strata. The Steensel Member shows a late Rupelian age based on the NSO5a dinoflagellate cyst biozone at 392–434.5 m. High numbers of the genus Homotryblium at the top of the Steensel Member indicate marginal to restricted marine conditions (Dybkjær, Reference Dybkjær2004). The Steensel Member is correlated to the Eigenbilzen Member in Belgium (Van Simaeys et al., Reference Van Simaeys, Munsterman and Brinkhuis2005; Stratigraphic Nomenclature NL online, 2021).
The transition from the Steensel Member towards the superjacent Voort Member of the Veldhoven Formation coincides with an increase in gamma-ray values as the result of an increase in clay content according to the borehole descriptions and resistivity log (the latter is not vectorially available and therefore not included in Fig. 3 of this study).
The increase in clay content may indicate a return of more open marine conditions. Indeed, an increase in relative abundance of Spiniferites and in the species diversity at 344.0–348.0 m suggests a development towards a more open marine depositional environment in the basal part of the Voort Member (Brinkhuis, Reference Brinkhuis1994). The common occurrence of Lingulodinium machaerophorium and Homotryblium floripes/plectilum indicates, however, a near-shore depositional environment (Dybkjær, Reference Dybkjær2004; Dybkjær et al., Reference Dybkjær, Rasmussen, Śliwińska, Esbensen and Mathiesen2019). This interval is dated as early Chattian based on the presence of the NSO6 dinoflagellate cyst biozone.
Within the lower part of the Voort Member, gamma-ray values show a gradual increase upward, which could be indicative for a continued deepening depositional environment. Maximum gamma-ray values are reached at 324–330 m, which may thus represent the maximum flooding surface. Indeed, the high numbers of Spiniferites and very rare occurrences of the genus Homotryblium at 322.5–326 m depth confirm a more open marine depositional environment (Brinkhuis, Reference Brinkhuis1994). The latter interval was dated late Chattian based on the NSO 8 dinoflagellate cyst biozone.
From 324 m to 309 m, the gamma-ray values decrease upward. Decreasing gamma-ray values are indicative for a decrease in clay content, which – in view of the cross-correlation with lithofacies analysis – could be interpreted as a shallowing up. This shallowing is confirmed by the presence of P1 and P2 macerals (relatively dark coloured and large pieces of organic (continental) debris with low buoyancy properties) in the interval between 305 and 326 m (Whittaker, Reference Whittaker1984). At 309 m, the gamma-ray values strongly increase towards a high plateau that continues up to 302 m, then drop towards lower values before rising again towards high gamma-ray values around 296 m (Fig. 3). The high gamma-ray values coincide with increase in clay content. In contrast to the interpretation of the results for the basal part of the Voort Member, that is more clay reflecting a phase of deepening of the depositional environment, here we interpret the results as indicating the establishment of lagoonal conditions for the clayey interval 305–296 m. The increase in clay content correlates with a distinct increase in abundance of Homotryblium floripes/plectilum (41% of the total sum of dinoflagellate cysts) and Lingulodinium machaerophorum (6%). Paralecaniella is also represented (4%). This palaeoenvironmental interpretation is supported by the regional geological development indicating a sea-level fall (Munsterman & Deckers, Reference Munsterman and Deckers2020 and refs. herein). The Mi-1 glacial event resulted in a major global eustatic sea-level fall at the Oligocene-Miocene boundary (Miller et al., Reference Miller, Browning, Kopp, Montain and Wright2020). This interval with high gamma-ray values between 309 and 293 due to increased clay content in the central part of the Veldhoven Formation is typically identified as the Wintelre Member. The age of this interval is latest Chattian to earliest Aquitanian, based on the identification of the M1 dinoflagellate cyst biozone. Reworking (ca. 3% of the total sum of palynomorphs) with an origin from Carboniferous and Eocene to lower Oligocene deposits was recorded.
The boundary between the Wintelre and Someren Members is picked at 293 m, where gamma-ray values have returned to lower values (Fig. 3). From here, the gamma-ray values gradually increase upward towards 272 m. At sample locations at 291.5–283.0 m and 274.5–271.5 m depths, the abundant presence of Homotryblium floripes/plectilum (43% of the total sum of dinoflagellate cysts) remains indicating lowered salinity/restricted marine (possibly lagoonal) environment. This entire interval belongs to the late Aquitanian to early Burdigalian M2 dinoflagellate cyst biozone. The zone which has on average higher gamma-ray values between 272 and 257 m coincides with a more clayey-silty zone in the upper part of the Someren Member. The restricted marine influence has disappeared here and the genus Spiniferites dominates (56% of the total sum dinoflagellate cysts) the palynospectrum again, pointing to open marine conditions. In the uppermost part of the Someren Member at sample location 255.5–257 m, the middle Burdigalian M3 dinoflagellate cyst biozone was identified. The dominant palynomorphs in the associations hardly changed, showing for example 60% Spiniferites spp.
The top of the Someren Member is picked at the turn-around point from increasing to decreasing gamma-ray values in gamma-ray values at 257 m. Here, the Someren Member of the Veldhoven Formation changes into the Kakert Member of the Groote Heide Formation. In the Kakert Member, the gamma-ray values continue to decrease, with drops at 250 m and 228 m towards very low gamma-ray values of the superjacent Heksenberg Member. Our dinoflagellate cyst analyses show that the Kakert Member has an age not younger than dinoflagellate cyst zone M6 and no older than dinoflagellate cyst biozone M4. A dominance of terrestrial spores and pollen indicates that the terrestrial influence increases. Furthermore, the variety in marine species is reduced, while Paralecaniella, a near-coastal marine indicator, increase in number. All these observations point towards a coastal marginal marine setting (Louwye et al., Reference Louwye, Marquet, Bosselaers and Lambert2010; Dybkjær et al., Reference Dybkjær, Rasmussen, Śliwińska, Esbensen and Mathiesen2019).
Borehole Herkenbosch (Peel High; Fig. 3)
Age assessment
The palynological analyses for the samples from the borehole Herkenbosch gave the following results (see Table 2):
The dating is based on:
-
*1: LOD’s of Distatodinium paradoxum and Apteodinium tectatum both at 64.2 m (Munsterman et al., Reference Munsterman, ten Veen, Menkovic, Deckers, Witmans, Verhaegen, Kerstholt-Boegehold, van de Ven and Busschers2019).
-
*2: LOD of Cordosphaeridium cantharellum at 70.2 m (Munsterman & Brinkhuis, Reference Munsterman and Brinkhuis2004; Dybkjær & Piasecki, Reference Dybkjær and Piasecki2010).
-
*3: LOD’s of Ectosphaeridium picenum (Zevenboom, Reference Zevenboom1995; Munsterman et al., Reference Munsterman, ten Veen, Menkovic, Deckers, Witmans, Verhaegen, Kerstholt-Boegehold, van de Ven and Busschers2019), Thalassiphora pelagica (Dybkjær & Piasecki, Reference Dybkjær and Piasecki2010), and the presence of dinoflagellate cyst Homotryblium vallum all at 78.2 m (De Verteuil & Norris, Reference De Verteuil and Norris1996; Munsterman et al., Reference Munsterman, ten Veen, Menkovic, Deckers, Witmans, Verhaegen, Kerstholt-Boegehold, van de Ven and Busschers2019).
-
*4: LOD’s of Chiropteridium galea and Hystrichokolpoma cinctum both at 88.7 m (De Verteuil & Norris, Reference De Verteuil and Norris1996; Munsterman et al., Reference Munsterman, ten Veen, Menkovic, Deckers, Witmans, Verhaegen, Kerstholt-Boegehold, van de Ven and Busschers2019).
-
*5: LOD of Glaphyrocysta cf. semitecta at 109.75 m (Van Simaeys et al., Reference Van Simaeys, Munsterman and Brinkhuis2005).
-
*6: LOD’s of Areoligera semicirculata at 121.75 m, Deflandrea phosphoritica at 141.75 m, and Wetzeliella symmetrica at 141.75 m (Van Simaeys et al., Reference Van Simaeys, Munsterman and Brinkhuis2005).
-
*7: LOD of Rhombodinium draco at 148.75 m (Van Simaeys et al., Reference Van Simaeys, Munsterman and Brinkhuis2005).
-
*8: LOD’s of Achilleodinium biformoides and Enneadocysta pectiniformis both at 181.25 m (Stover & Hardenbol, Reference Stover and Hardenbol1994; Van Simaeys et al., Reference Van Simaeys, Munsterman and Brinkhuis2005).
Table 2. Herkenbosch borehole.

Stratigraphy, log pattern and depositional environment
Low gamma-ray values of the sandy Steensel Member, interval 163.25–217.75 m, on top of the high gamma-ray values of the clayey Boom Member, interval 217.75–310.1 m, are once again good reference for the identification of the Rupelian strata. The Steensel Member is inferred as late Rupelian, based on the presence of the NSO 4a-5b dinoflagellate cyst interval biozones. The interpretation fits with Van Simaeys et al. (Reference Van Simaeys, Munsterman and Brinkhuis2005), more detailed showing dinoflagellate zone NSO5b for the base of the Voort Member and NSO5a for the Eigenbilzen Formation. The abundance of the genus Dapsilidinium (up to 35% of the total sum of dinoflagellate cysts) at the top of the Steensel Member (interval 163.25–178.25 m) indicates marginal to restricted marine conditions (Brinkhuis, Reference Brinkhuis1994; Munsterman & Brinkhuis, Reference Munsterman and Brinkhuis2004).
The transition from the Steensel Member towards the superjacent Voort Member of the Veldhoven Formation shows a sharp increase in gamma-ray values, unlike in borehole Limbricht, where a more gradual transition occurs. This increase in gamma-ray values is related to the upward increased clay content, which is expressed by an upward decrease in resistivity values. The increase in clay content coincides with a development towards more open marine conditions. Indeed, an increase in the relative abundances of the dinoflagellate cyst genera Spiniferites, Systematophora and in dinoflagellate cyst species diversity suggests an increased sea level in the Voort Member (Brinkhuis, Reference Brinkhuis1994).
About 20 m above the base, the gamma-ray values increase and resistivity values decrease upward towards a 20 m thick clayey interval of early Chattian age (dinoflagellate cyst biozone NSO 6). From this clayey interval, the gamma-ray values decrease and the resistivity values increase upward again towards the upper part, more sandy part of the Voort Member, suggesting gradual shallowing upward. Despite the upward coarsening trend, shallow to open marine conditions still prevail at depth 103.75–100.75 m, mirrored by a relatively common occurrence of the genera Operculodinium, Hystrichokolpoma and 37% of Spiniferites. The interval between 148.75 and 109.75 m is dated as Chattian based on the presence of the NSO6 and NSO7 dinoflagellate cyst biozones.
From 101 m upward, the gamma-ray values increase again and the resistivity values flatten in the top of the Voort Member. At 92 m depth a sandstone is present. From this level and upward, an increase in gamma-ray values takes place, together with a sharp upward decrease in resistivity values. This boundary marks the transition from sand of the Voort Member towards the clay of the Wintelre Member. The interval of the Wintelre Member between 91.6 and 88.7 m is dated as latest Chattian to earliest Aquitanian (dinoflagellate cyst zone SNSM 1). As was the case in the Limbricht borehole, also here a lagoonal depositional setting is interpreted by palynological analysis of the clays of the Wintelre Member. Very high values of genus Homotryblium (>50% of the total sum dinoflagellate cysts) strongly indicate the presence of restricted marine conditions (Dybkjær, Reference Dybkjær2004).
From about 84 m upward, the resistivity values increase and the gamma-ray values decrease upward, which marks the transition from the silty clays of the Wintelre Member towards the sandy clays and sands of the Someren Member. Restricted marine conditions prevail at the base (82.1–78.2 m) of the Someren Member, which was dated as dinoflagellate cyst biozone SNSM2 (late Aquitanian). Higher in the Someren Member, at depths between 72.2 and 70.2 m, the relative abundance of Homotryblium decreases and the diversity of dinoflagellate cyst species increases. The near-coastal marine dinoflagellate cyst genus Apteodinium comprises up to 15% of the total dinoflagellate cyst assemblage, which indicates a return to open marine conditions (Brinkhuis, Reference Brinkhuis1994). This higher part of the Someren Member is dated as dinoflagellate cyst biozone SNSM3 (mid-Burdigalian in age).
The transition from the Someren Member (Veldhoven Formation) to the Kakert Member of the Groote Heide Formation is marked by the appearance of humic fragments. The boundary is expressed by lowering of gamma-ray values combined with the start of a further increase in values of the resistivity log. The boundary further coincides with a shift from dinoflagellate cyst biozone SNSM3 (middle Burdigalian) in the upper part of the Someren Member towards dinoflagellate cyst biozone SNSM4-6 (late Burdigalian-early Langhian) in the Kakert Member. Sea level is decreasing from the Someren Member towards the Kakert Member as shown by the occurrence of an acme of the marginal marine dinoflagellate cyst genus Polysphaeridium at depth 67.2–64.2 m (Brinkhuis, Reference Brinkhuis1994).
Borehole correlations (Fig. 3)
Based on lithological descriptions and the gamma-ray log pattern, the Veldhoven Formation and its three members were identified and correlated between the Groote Heide, Herkenbosch, Limbricht and Molenbeersel boreholes, located in the Venlo Graben, on the Peel High and in the RVG, all part of the central Roer Valley Rift System (Fig. 1). For the Groote Heide, Herkenbosch and Limbricht boreholes, the three members of the Veldhoven Formation were identified for the first time in the present study. For the Molenbeersel borehole, the three-fold subdivision of the Veldhoven Formation was proposed by Broothaers et al. (Reference Broothaers, Deckers, Lagrou and Matthijs2012).
Correlations with borehole Groote Heide (the Netherlands)
In the Groote Heide borehole, from which the dinoflagellate cyst content was studied previously by Munsterman & Brinkhuis (Reference Munsterman and Brinkhuis2004), the basal Veldhoven Formation is underlain by late Rupelian silty clay instead of sand as is the case for the Limbricht and Herkenbosch boreholes. Therefore, the lower boundary of the Veldhoven Formation in the Groote Heide borehole does not coincide with a major change in gamma-ray patterns. The gamma-ray values at the Steensel/Voort Member boundary are also influenced by a lithified shell bed at interval depth 642.25–643.7 m. The Steensel Member, below this bed, is once again interpreted as dinoflagellate cyst Zone NSO5a.
A three-fold subdivision of the Veldhoven Formation can be made, based on the lithological development in the Groote Heide borehole, albeit the gamma-ray pattern is much more subtle than within the Limbricht and Herkenbosch boreholes.
-
Lower part or Voort Member consists of sandy clay in the lower part, which is reflected by relatively high gamma-ray values, gradually changing upward to very fine sand, as seen as lower gamma-ray values. The Voort Member is referred to the biozones NSO5b-NSO8. The palaeoenvironment is interpreted as fluctuating marginal to open marine. Shallow marine conditions are indicated in particular by the dinoflagellate cyst genus Delflandrea and less frequently also by the genus Wetzeliella (Brinkhuis, Reference Brinkhuis1994). These genera disappear at c. 590 m. Above this level, the more open marine dinoflagellate cyst genus Spiniferites increases occasionally in relative abundance, interspersed with increases in shallow and restricted marine species. Incidental assemblages show high concentrations of the genus Homotryblium, representing restricted marine conditions (silty clay sample interval at 564.75–566.65 m: 50% of the total sum dinoflagellate cysts). The relative sea level is finally decreasing towards the uppermost of the succession at c. 510 m. Shallow marine genera like Chiropteridium and Apteodinium relatively increase at the cost of Spiniferites. The sediments show an increase in mollusc content.
-
Central part or Wintelre Member consists of very fine sand and sandy clay, reflected by high, but also very variable, gamma-ray values. The Wintelre Member is referred to the M1 dinoflagellate cyst zone. The palynofacies indicates restricted marine, possibly lagoonal conditions: the dinoflagellate cyst assemblage within the interval 470–446 m comprises 40–70% Homotryblium floripes/plectilum. Compared to the clays in the Voort Member, higher concentrations of shelves are evidently present.
-
Upper part or Someren Member consists of fine sand with some silty intercalations and is referred to the dinoflagellate cyst zones M2–M3. It shows moderate and generally upward increasing gamma-ray values. In the basal part (interval 439-–425 m) of this lithostratigraphic unit, marginal marine conditions are maintained, shown by relatively high values of Homotyblium and Paralecaniella, but in the overlying succession these taxa decrease in number and Spiniferites becomes the dominant genus, indicating a return to an open marine setting.
The upper boundary of the Veldhoven Formation is characterised by a decrease in gamma-ray values towards the Kakert Member of the Groote Heide Formation (Munsterman et al., Reference Munsterman, ten Veen, Menkovic, Deckers, Witmans, Verhaegen, Kerstholt-Boegehold, van de Ven and Busschers2019), which is very similar to the Limbricht and Herkenbosch boreholes. In the Groote Heide borehole, the upper boundary of the Veldhoven Formation coincides with the boundary between the dinoflagellate cyst zones M3 and M4. The Kakert Member shows the first continuous freshwater influence (continuous occurrence of Pediastrum) pointing to a change into an estuarine or an embayment setting.
Correlations with borehole Molenbeersel (Belgium)
The low gamma-ray values of the Steensel Formation on top of the high gamma-ray values of the Rupel Formation are a good reference for correlations of the Rupelian strata between the Limbricht, Herkenbosch and Molenbeersel boreholes. Based on correlations with surrounding boreholes, the low gamma-ray interval of the Molenbeersel borehole was referred to the Eigenbilzen Formation by Vandenberghe et al. (Reference Vandenberghe, Brinkhuis, Steurbaut, Prothero, Ivany and Nesbitt2003). The late Rupelian age for the Eigenbilzen Formation suggested by them and the late Rupelian NSO5a dinoflagellate cyst zone found in the Steensel Formation in the Limbricht and Herkenbosch boreholes in the present study support a correlation between the Belgian Eigenbilzen and the Dutch Steensel Formation.
The transition from the Steensel Member towards the overlying Veldhoven Formation coincides with an increase in gamma-ray values, reflecting an increase in glauconite content. The three-fold subdivision of the Veldhoven Formation in the Molenbeersel borehole into the Voort, Wintelre and Someren Members was introduced by Broothaers et al. (Reference Broothaers, Deckers, Lagrou and Matthijs2012). The gamma-ray patterns on which this three-fold subdivision in the Molenbeersel borehole is based are similar to the patterns seen in the Limbricht and Herkenbosch boreholes: the sandy Voort and Someren members show moderate gamma-ray values and are separated by the clayey, high gamma-ray interval referred to the Wintelre Member.
Correlation of the Wintelre Member in borehole Molenbeersel with the German lithostratigraphic subdivision, represented by Schneider and Thiele hydrostratigraphic codes (S&T) of the Lower Rhine Basin and Chattian age assessment based on correlation with type-sections in the Lower Rhine coal and salt districts, corresponds to S&T01 (Schneider and Thiele, Reference Schneider and Thiele1965; Hager et al., Reference Hager, Vandenberghe, Van den Bosch, Abraham, Von der Hocht, Rescher, Laga, Nickel, Verstrealen, Leroi and Van Leeuwen1998; Dusar & Vandenberghe, Reference Dusar and Vandenberghe2020). These S&T units in the Köln Formation (Germany) are interpreted as marginal to lagoonal environments (see also Schäfer & Utescher, Reference Schäfer and Utescher2014).
The upper boundary of the Someren Member or Veldhoven Formation coincides with a gamma-ray spike in all three boreholes, and from there on, the gamma-ray values drop towards lower values in the lower part of the Kakert Member of the Groote Heide Formation. The transition from the Kakert Member towards the Heksenberg Member coincides with a further reduction towards very low gamma-ray values in all the above-mentioned boreholes as a result of a strong decrease in clay and glauconite content.
Correlation with holotype section well Veldhoven-1 (VEH-01) in the RVG (the Netherlands)
The holostratotype section of the Veldhoven Formation is established in well Veldhoven (NLOG code VEH-01) interval 860–1103 m (Van Adrichem Boogaert & Kouwe Reference Van Adrichem Boogaert and Kouwe1993–1997, Annex I–6). This well is drilled in the late 1950s. Unfortunately, the material of the well consists primarily of ditch cuttings, hampering lithological description and biostratigraphic interpretation by caving. Cores are only available at two intervals, viz. 907–913 m and 1041–1047 m. Furthermore, the type-well does not provide a gamma-ray log, but SP and resistivity (RES) logs, which make comparison with other wells challenging (Fig. 6).
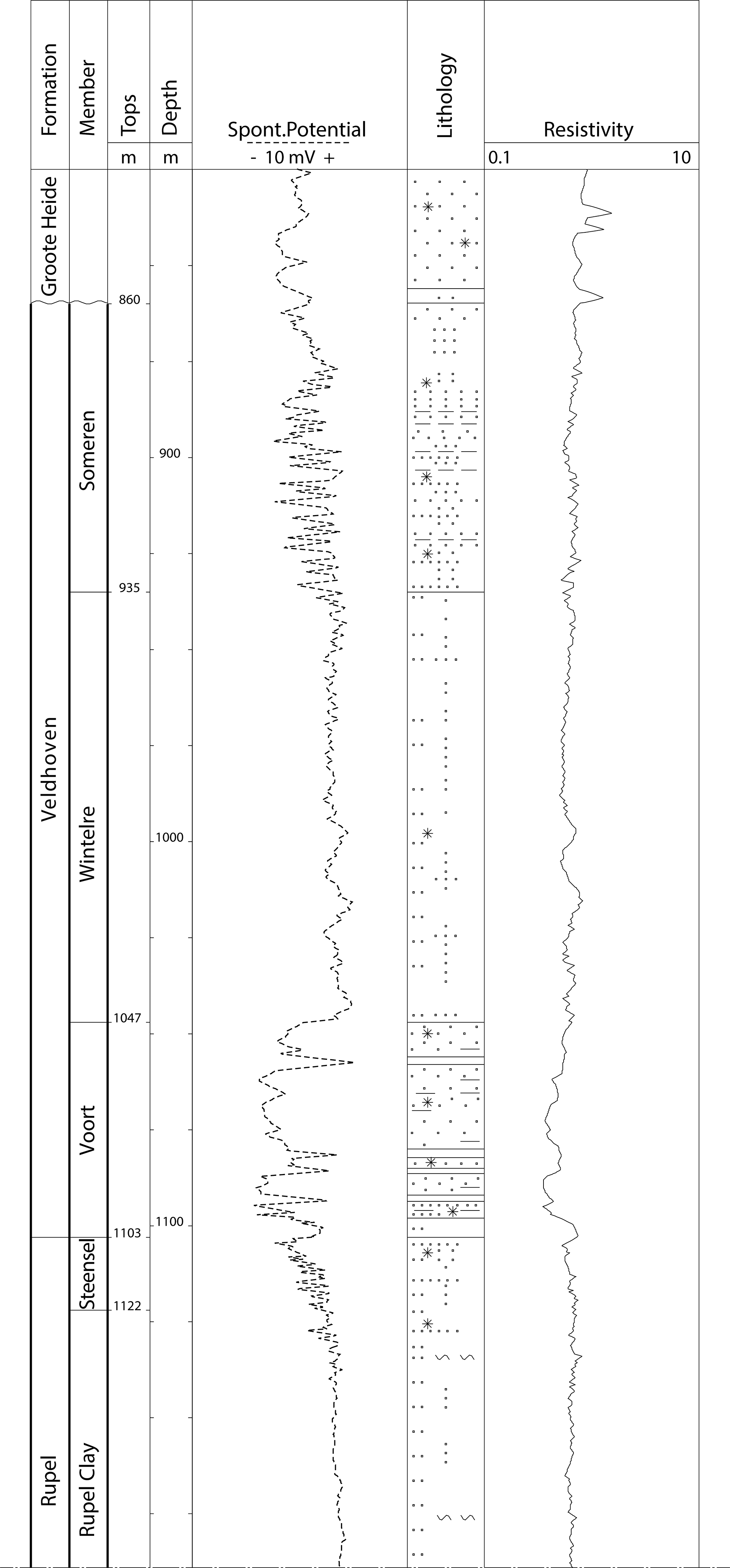
Fig. 6. Holostratotype well Veldhoven-1 (VEH-01) sensu Van Adrichem Boogaert & Kouwe (Reference Van Adrichem Boogaert and Kouwe1993–1997) and Stratigraphic Nomenclature of the Netherlands online.
In this well, the Veldhoven Formation overlies the Steensel Member of the Rupel Formation and its lower boundary is at the base of a sandy clay bed. Because of all limitations referred to caving, only several cuttings samples are palynologically analysed (Munsterman, Reference Munsterman2001). At the top of the Steensel Member at 1105 m, the NSO5b Zone, or older is interpreted, which matches with wells Groote Heide, Herkenbosch and LBR-01. The upper boundary of the Veldhoven Formation (with Groote Heide Formation) at 860 m is interpreted at the shift of decreasing concentrations of silts and clays within the sandy succession. This corresponds to a lowering in the values on the SP log.
The SP log of the Veldhoven Formation in well VEH-01 shows a four-fold subdivision:
-
Voort Member, interval 1047–1103 m: Low SP values, including very irregular high peaks in the lowest part. Best described as a stacking of coarsening upward sequences at the scale of tens of metres. The proportion of clays is relatively high in the lower part of the member. The basal clays are also shown in well Groote Heide, sandy clays are shown in the lower part of the other wells, although on the Peel High (well Herkenbosch) and in southern RVG (well LBR-01) the proportion of argillaceous sediments is very low. The middle part of VEH-01 stands out as a homogeneous clean sand, which is also represented on the SP log as low values. The interval is palynologically interpreted as Zone NSO6, like all basal successions of the Voort Member in the other wells. The sands are fine-grained, grey-green and glauconitic. Locally, the sands may have a dark grey-green to black colour and comprise glauconites and marine molluscs. The uppermost part is fining upward into silts and becomes more clayey (in particular well-shown in well Molenbeersel).
-
A transitional interval 995–1047 m: Serrated pattern of high SP values. The lithostratigraphy of this interval is doubtfully interpreted as Wintelre Member. The cuttings in this succession consist of changing proportions of sands, clays and glauconites. At 1009 m dinocyst Zone NSO8, or older is reached, which is based on the LOD of Distatodinium biffii at 1009 m (core section). The upper part (995–1009 m) is interpreted as dinocyst Zone M1.
-
Wintelre Member, interval 995–935 m: generally a very regular high signal is mirrored on the SP log. The sediments are grey to greenish-grey sandy clays, locally with brownish colours. The succession becomes more silty and sandy towards the top. Most typically developed in wells VEH-01 and Molenbeersel. This and most other trends are shown in a condensed manner in well LBR-01. The coarsening upward grain-size trend to the top is also shown in well Groote Heide (Venlo Graben). On the Peel High it is a relatively thin clay interval in between the Voort and Someren members. The dating is latest Chattian-earliest Aquitanian, dinocyst Zone M1.
-
An irregular low pattern for the uppermost part corresponds to the Someren Member, interval 860-935 m. The succession consists of clayey-silty sands, like in all other studied wells here. The colour of the sand is greenish-grey, and the glauconite content is low. Marine shells occur locally. The palynological association of the cuttings samples indicates M2 and M3 dinocyst zones, which are in agreement with the recorded zones of the Someren Member in all wells of this study.
Discussion
Age interpretations
The existing biostratigraphic interpretations of the Groote Heide borehole by Munsterman et al. (Reference Munsterman, ten Veen, Menkovic, Deckers, Witmans, Verhaegen, Kerstholt-Boegehold, van de Ven and Busschers2019) and of the Limbricht and Herkenbosch boreholes by Munsterman (Reference Munsterman1997a, b; Reference Munsterman1998) combined with the new analyses of the present study provide consistent age information on the different members of the Veldhoven Formation: The Voort Member is of Chattian age (dinoflagellate cyst zones NSO5b-8), the Wintelre Member is of latest Chattian to earliest Aquitanian age (dinoflagellate cyst zone NSM1), and the Someren Member is of late Aquitanian to middle Burdigalian age (dinoflagellate cyst zones NSM2-3). This confirms the ages of the members of the Veldhoven Formation as shown in Figure 8 of Munsterman et al. (Reference Munsterman, ten Veen, Menkovic, Deckers, Witmans, Verhaegen, Kerstholt-Boegehold, van de Ven and Busschers2019) for the northern part of the RVG (Fig. 2).
Nearly all the late Oligocene to early Miocene dinoflagellate cyst zones were identified in the Limbricht, Herkenbosch and Groote Heide boreholes excluding zone NSO7 in the Limbricht borehole and zone NSO8 in the Herkenbosch borehole. The missing zone is most probably due to the low sample resolution. It is therefore believed that no major haiti (of longer duration than a dinoflagellate zone) occurred in the late Oligocene to early Miocene deposits in the central RVG, similar to what was found in the northern RVG by Munsterman et al. (Reference Munsterman, ten Veen, Menkovic, Deckers, Witmans, Verhaegen, Kerstholt-Boegehold, van de Ven and Busschers2019).
The correlations of the biostratigraphically analysed Groote Heide, Herkenbosch and Limbricht boreholes with the Molenbeersel borehole allow age estimates for the upper Oligocene-lower Miocene succession in the latter key borehole. These results can then be compared with previously suggested ages for this succession by Hager et al. (Reference Hager, Vandenberghe, Van den Bosch, Abraham, Von der Hocht, Rescher, Laga, Nickel, Verstrealen, Leroi and Van Leeuwen1998), Verbeek et al. (Reference Verbeek, De Leeuw, Parker and Wong2002) and Deckers (Reference Deckers2016) as shown in Fig. 7. Similarly to the present study, the previous studies made age estimates for the stratigraphic successions in the Molenbeersel borehole based on correlation of log patterns with nearby boreholes that were provided with age information. The improvement and strength of this study compared to the previous ones is the proximity of the Limbricht borehole to the Molenbeersel borehole and its location in the same structural entity, namely the RVG. Our datings are not in agreement with those of Hager et al. (Reference Hager, Vandenberghe, Van den Bosch, Abraham, Von der Hocht, Rescher, Laga, Nickel, Verstrealen, Leroi and Van Leeuwen1998) who referred to the whole interval here currently interpreted as the Veldhoven Formation to the Chattian. Our results also partly contradict those of Verbeek et al. (Reference Verbeek, De Leeuw, Parker and Wong2002) since they suggested a Chattian age for the interval here referred to the Wintelre Member and a middle Burdigalian age for the Someren Member. Our results also contradict Deckers (Reference Deckers2016) for his proposed Aquitanian age for the entire Someren Member, while his suggested Chattian and latest Chattian to Aquitanian ages for the Voort and Wintelre Members are confirmed by this study. Consequently, the change in fault kinematics in the RVG that Deckers (Reference Deckers2016) observed around the stratigraphic level of the Wintelre Member is confirmed by this study to have an age spanning the Oligocene/Miocene boundary.

Fig. 7. Comparison of the past and presently presumed ages of the Veldhoven Formation and its members in the Molenbeersel borehole.
Depositional environment
The gamma-ray log patterns and relative position of biozones within the members of the Veldhoven Formation vary between the four studied boreholes. This shows that whereas the subdivision of the Veldhoven Formation in its three members was of a regional extent, the members themselves show lateral facies changes. This is in agreement with the results of Deckers (Reference Deckers2016) who observed patterns of north-west progradation within both the Voort and Wintelre members in the area south and west of the Molenbeersel borehole. This progradation pattern confirms the idea of progressive infill of the RVG from a south-eastern source area as stated by Hager et al. (Reference Hager, Vandenberghe, Van den Bosch, Abraham, Von der Hocht, Rescher, Laga, Nickel, Verstrealen, Leroi and Van Leeuwen1998) whereby the type of sediment is dependent on the interaction between subsidence rates, eustatic sea-level and sediment input. The different subsidence rates and proximity to the sediment source area at the locations of the boreholes could explain the different gamma-ray log patterns for the members of the Veldhoven Formation in these boreholes. For each borehole, variation in lithology within the Veldhoven Formation seem, however, limited to in between the sandy clays and fine sands.
Despite these limited facies variations, the different members were deposited in similar environments, with predominantly shallow marine (fluctuating between open and restricted marine as shown in borehole Groote Heide) conditions in the Voort Member, restricted marine for the Wintelre Member that continue in the lower part of the Someren Member before returning again towards shallow marine conditions in the upper part of the Someren Member.
The marginal marine conditions during the earliest Chattian (NSO5b) followed by a short-term deepening in the early Chattian (NSO6) are in agreement with analyses of boreholes in northern Belgium by De Man & Van Simaeys (Reference De Man and Van Simaeys2004). The latter authors stated that the early Chattian Voort Member was deposited in a shallower environment compared to the underlying Eigenbilzen Formation in boreholes in northern Belgium. In the Limbricht and Herkenbosch boreholes, further to the south-east and thus closer to the sediment source, on the other hand, the Steensel Member (Eigenbilzen Formation in Belgium) was deposited in a marginal to restricted marine depositional environment and the Voort Member represents a relative deepening of the depositional environment. The increase in water depths in the basal Chattian Voort Member coincides with an increase in clay content, expressed by an increase in gamma-ray values. For the overlying Wintelre Member, the increase in clay content also reflects a shallowing from shallow marine environments in the upper part of the Voort Member towards restricted marine environments. The clays of the Wintelre Member are therefore interpreted as lagoonal clay. These clays can be distinguished from most clays in the Voort Member by a higher concentration of shells as is obviously demonstrated in the lithological description of borehole Groote Heide. The dinoflagellate cyst assemblages are here dominated by a single genus indicating extreme depositional environments, including salinities that deviate from normal marine conditions, most probably due to minor ventilation by narrow or lack of connection to the Atlantic Ocean. The glacio-eustatic sea-level fall around the Oligocene/Miocene boundary (e.g. Miller et al., 2020; Steinthorsdottir et al., Reference Steinthorsdottir, Coxall, De Boer, Huber, Barbolini, Bradshaw, Burls, Feakins, Gasson, Henderiks, Holbourn, Kiel, Kohn, Knorr, Kürschner, Lear, Liebrand, Lunt, Mörs, Pearson, Pound, Stoll and Strömberg2020) most likely limited the sea coverage to the strongest subsiding areas, where deposition of the Wintelre Member took place, while non-deposition or erosion occurred in the surrounding highs, hence creating an isolated (sub)basin. The lagoonal depositional environment of the Wintelre Member spanning the Oligocene/Miocene boundary, in the differentially subsiding Roer Valley Rift System, is in agreement with the missing late(st) Chattian-(early) Aquitanian sections at the relatively high flanks of the Rift System as observed by Louwye et al., (Reference Louwye, Deckers, Verhaegen, Adriaens and Vandenberghe2020 and references therein; Fig. 2A).
The lateral persistence of the clayey Wintelre Member (unit S&T01) across all structural settings point to a eustasy-based model (Hager et al., Reference Hager, Vandenberghe, Van den Bosch, Abraham, Von der Hocht, Rescher, Laga, Nickel, Verstrealen, Leroi and Van Leeuwen1998). This is in contrast to the sedimentological model of Schäfer et al. (Reference Schäfer, Utescher, Klett and Valdivia-Manchego2005), which proposes a lateral transition from marginal facies of the Köln Formation in the Lower Rhine Basin (Germany) across the shallow marine Dutch Voort Member in the Venlo/Peel Blocks to the deeper water Wintelre (Veldhoven Clay) Member in the RVG of the Netherlands. The latter is shared by the dynamic concept in De Mulder et al. (Reference De Mulder, Geluk, Ritsema, Westerhof and Wong2003, fig. 172); Wong et al., (Reference Wong, Batjes and De Jager2007, fig. 9) suggesting that the Wintelre Member is a deeper marine facies compared to the Someren and Voort members (Dusar & VandenBerghe, Reference Dusar and Vandenberghe2020). This study disproves the latter vision by indicating restricted marine conditions for the Wintelre Member, interpreted as result of the associated glacio-eustatic sea-level fall around the Oligocene/Miocene boundary. The S&T06 unit (Hager et al., Reference Hager, Vandenberghe, Van den Bosch, Abraham, Von der Hocht, Rescher, Laga, Nickel, Verstrealen, Leroi and Van Leeuwen1998) is not systematically recorded as a clayey interval of consistent age in our studied wells.
Marginal marine conditions prevailed during the rest of the Aquitanian when the sands in the lower part of the Someren Member were deposited. As sea-level rose, the upper, more clayey part of the Someren Member was deposited in a shallow marine environment, and the flanking highs of the Roer Valley Rift System were transgressed during the Burdigalian (Fig. 2A). During a latest Burdigalian shallowing, the Veldhoven Formation became covered by the sands and clays of the Kakert Member (Groote Heide Formation). Input of freshwater is consistently recorded here.
Conclusions
New information on the bio- and lithostratigraphy and facies from Dutch boreholes Herkenbosch (Peel High) and Limbricht (RVG) and their lithological and gamma-ray log correlation to the boreholes Molenbeersel (RVG, Belgium) and Groote Heide (Venlo Graben, the Netherlands) provide good consistency on stratigraphy and depositional environments for the members of the Veldhoven Formation in the Roer Valley Rift System during the late Oligocene-early Miocene. Based on the new results of this study we were able to convincingly revise the previous views on, in particular, the Wintelre Member not as a deeper marine facies compared to the Someren and Voort members, but representing restricted marine conditions. Dinoflagellate cyst assemblages in the Wintelre Member are mostly dominated by a single genus, indicating a restricted marine depositional setting with salinities that deviate from normal marine conditions, most probably due to minor ventilation by narrow or lack of connection to the Atlantic Ocean. These circumstances would have resulted from the associated glacio-eustatic sea-level fall around the Oligocene/Miocene boundary in concert with the Savian tectonic phase. Both factors limited preserved marine successions to the strongest subsiding areas, where deposition of the Wintelre Member is recorded, while non-deposition or erosion occurred in the surrounding highs, hence creating an isolated (sub)basin.
Acknowledgements
The authors gratefully acknowledge financial support from the Land and Soil Protection, Subsoil, and Natural Resources Division of the Flemish Government. We would like to thank Katleen van Baelen for her excellent work on the figures. The three reviewers, Karin Dybkjær, Noël Vandenberghe and Frank Wesselingh are thanked for their very helpful suggestions and corrections improving the manuscript substantially. Finally we are much obliged to our editor Rick Donselaar (TU-Delft, the Netherlands) for his logistical support and corrections in the text.