Introduction
Northwestern Europe was partially covered by a shallow sea during the latest Triassic. This Rhaetian Sea was surrounded by several topographic highs (e.g. Suan et al., Reference Suan, Föllmi, Adatte, Bomou, Spangenberg and van de Schootbrugge2012; Fischer et al., Reference Fischer, Voigt, Franz, Schneider, Joachimski, Tichomirowa, Götze and Furrer2012; Fig. 1). Dark shales were deposited in many parts of this sea where coarse clastic input from the continents was limited (e.g. Wright, Reference Wright1874; Macquaker, Reference Macquaker, Swift and Martill1999; Allington-Jones et al., Reference Allington-Jones, Braddy and Trueman2010; Klompmaker et al., Reference Klompmaker, Herngreen and Oosterink2010). Even though the Netherlands was located in the central to central-south part of this sea, sediments of this part of the quarry have received relatively little attention thus far, partly due to limited exposures. Dark shales are, however, known from the eastern part of the Netherlands in the Winterswijk quarry complex (Klompmaker et al., Reference Klompmaker, Herngreen and Oosterink2010). Trace fossils from these deposits have not been studied thus far. The goals of this study are to describe the first trace fossil from these shales, discuss its origin and comment on the palaeoenvironment.

Fig. 1. Palaeogeography of the Rhaetian Sea, with the location of Winterswijk indicated by a red dot (modified from Fischer et al., Reference Fischer, Voigt, Franz, Schneider, Joachimski, Tichomirowa, Götze and Furrer2012, fig. 7).
Geological setting
The Winterswijk quarry complex exposes primarily Middle Triassic (Anisian, ‘Muschelkalk’) grey marlstones and dolo-limemudstones deposited on an epeiric carbonate ramp (Borkhataria et al., Reference Borkhataria, Aigner and Pipping2006). Numerous fossils have been found, including reptiles, reptile footprints, other trace fossils, fishes, bivalves, ammonites, a brachiopod, gastropods, rare plant fossils, chelicerates, crustaceans and possible jellyfish (e.g. Oosterink, Reference Oosterink1986, Reference Oosterink2009; Diedrich, Reference Diedrich2001; Oosterink et al., Reference Oosterink, Berkelder, De Jong, Lankamp and Winkelhorst2003; Klein & Albers, Reference Klein and Albers2009; Klompmaker & Fraaije, Reference Klompmaker and Fraaije2011; Neenan et al., Reference Neenan, Klein and Scheyer2013; Oosterink & Winkelhorst, Reference Oosterink and Winkelhorst2013). Other sediments found in the quarry are Late Triassic (Rhaetian), Jurassic (Hettangian), Oligocene, Pleistocene and Holocene in age (e.g. Oosterink, Reference Oosterink1986; Diedrich, Reference Diedrich2001; Herngreen et al., Reference Herngreen, van Konijnenburg-van Cittert and Oosterink2005; Klompmaker & van den Berkmortel, Reference Klompmaker and van den Berkmortel2007; Klompmaker et al., Reference Klompmaker, Herngreen and Oosterink2010). The Upper Triassic (Rhaetian) shales occurred in two parts of the quarry: as infill of a subrosion pipe or sinkhole in the center of Quarry III of the quarry complex and in Quarry IV, a northward extension of Quarry IV (Herngreen et al., Reference Herngreen, van Konijnenburg-van Cittert and Oosterink2005; Oosterink et al., Reference Oosterink, Simon, Hagdorn and Winkelhorst2006; Klompmaker et al., Reference Klompmaker, Herngreen and Oosterink2010, fig. 1). These shales are a part of the Sleen Formation, which is the lowermost unit of the Altena Group. Fossil evidence indicates that the environment at the time of deposition of these shales was likely near-coastal with marine influences, and possible low oxygen conditions near the bottom (Klompmaker et al., Reference Klompmaker, Herngreen and Oosterink2010). The shales contain bivalves, ophiuroids and fishes (tooth of Hybodus sp., scales of Gyrolepis sp., pers. comm. Henk Diependaal and Jelle Reumer, October 2013), as well as ex situ plant fossils, including palynomorphs and one horsetail fragment (Klompmaker et al., Reference Klompmaker, Herngreen and Oosterink2010; Thuy et al., Reference Thuy, Klompmaker and Jagt2012; Klompmaker & Steur, Reference Klompmaker and Steur2014). The specimen from this study was found in Quarry IV of the Winterswijk quarry complex, 180–200 cm below the Rhaetian–Oligocene unconformity.
Methods and materials
To reveal both sides of the specimen, the fossil was dislodged from its matrix. While looking at the specimen under a microscope, dental picks were used to pry the string out of the shale matrix. High-quality photographs of the specimen using a Canon Eos 5D (Mark II) camera were taken in addition to a series of SEM (Zeiss Evo MA 10) images of the specimen.
Energy-dispersive X-ray spectroscopy (EDS) analysis was performed on both the string of knobs (the inside and outside of the knobs) and on the surrounding matrix. To perform the analysis on the inside of the structure, one end of the string was broken off to reveal a freshly broken surface.
Institutional abbreviation
RGM – Naturalis Biodiversity Center, Leiden, the Netherlands.
Analyses and results
Specimen description
The specimen consists of a string of small sub-circular knobs (Fig. 2). The string is about 20 mm in length, ~1.3 mm in height and ~3.5 mm in width, but the width is not consistent throughout the specimen. The string was oriented parallel to the bedding plane. Knob diameters range between 300 and 500 μm. The knobs are present on the top, bottom and sides of the string. One end of the string (upper right in Fig. 2A) was completely preserved, whereas the other end was incomplete because it was embedded at the margin of the slab in which it was found.

Fig. 2. The studied trace fossil from the Upper Triassic (Rhaetian) shales of the eastern Netherlands (Winterswijk Quarry IV) (RGM.791801). A. SEM image of the full string of knobs. Inset shows the other side of the full string still embedded in the shale matrix. B. SEM image of the string of knobs, close-up on a single area of the specimen. C. SEM image of the specimen in cross section. The inset shows what this freshly broken surface looks like in full colour. EDS analysis shows that the string of knobs is pyritised. D. SEM image of a single knob of the specimen.
EDS analysis
EDS analysis revealed high amounts of Fe and S, suggesting that the specimen was pyritised (FeS2) on the inside of the knobs (Fig. 3). The photo taken of the specimen's freshly broken surface is distinctly gold-coloured and pyrite-like in appearance (inset Fig. 2C). EDS analysis of the sediment next to the string showed high amounts of O, Si and Al, with lesser amounts of C, Fe, Mg, S, K and Ca.

Fig. 3. Graphs of the energy-dispersive X-ray spectroscopy (EDS) analyses. A. EDS graph of the inside of one knob. B. EDS graph of the sediment surrounding the specimen. Elemental abbreviations: O, oxygen; Fe, iron; Al, aluminum; Si, silicon; S, sulphur; C, carbon; Mg, magnesium; K, potassium; Ca, calcium. Given the relative abundance of elements, amuminosilicate clay minerals including kaolinite and illite may be present, as for the Rhaetian shales of the Westbury Formation in nearby Great Britain (Macquaker, Reference Macquaker, Swift and Martill1999).
Discussion and conclusions
Origin of the string
Larvae
With the abundance of bivalves in the Rhaetian shales of the quarry, fossil bivalve larvae may be expected. Additionally, bivalve larvae may ‘raft’ together to form something that resembles the string in the specimen (Helm & Bourne, Reference Helm and Bourne2004, fig. 44D). However, bivalve larvae range in size from 60 to 175 μm on average, and are too small to be considered similar to the specimen.
Eggs
The ammonite eggs shown in Fig. 4A and described by Etches et al. (Reference Etches, Clarke and Callomon2009) show a series of knobs. The group of eggs is similar in length, but wider, and the knobs are larger in diameter (1–3.5 mm) than the knobs in the specimen herein. These eggs have been interpreted as an egg sac due to a jelly-like substance surrounding the eggs, not as a simple string, and show a pimpled microstructure, unlike the specimen herein. Finally, ammonites are not known from these shales.
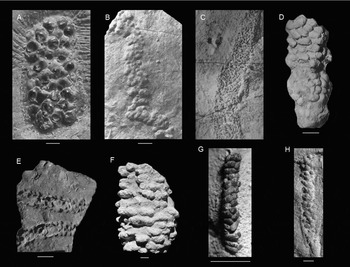
Fig. 4. Other fossils and trace fossils with a knobby morphology. A. Ammonite eggs show a series of knobs (from Etches et al., Reference Etches, Clarke and Callomon2009). B. The trace fossil Neonereites multiserialis, a knobby fossil burrow with knobs of 1–2 mm (from Pickerill, Reference Pickerill1991). C. The fossil burrow Granularia has ellipsoidal knobs, small compared to the tube diameter, mostly arranged perpendicular to the axis burrow; it is also flattened and may contain fecal pellets (from Häntzschel, Reference Häntzschel, Moore and Teichert1975). D. Edaphichnium lumbricatum, a non-marine trace fossil. The fecal pellets are ellipsoidal and can be smaller than 1 mm (from Bown & Kraus, Reference Bown and Kraus1983). E. The non-marine trace fossil Sphaerapus ( = Walpia) larvalis, ellipsoidal or circular in cross-section, with non-coprolitic pellets were usually arranged in two rows (from Lucas et al., Reference Lucas, Voigt, Lerner and Rainforth2013). F. The knobby burrow Ophiomorpha, which usually has a strong vertical component (O. nodosa from de Gibert et al., Reference de Gibert, Netto, Tognoli and Grangeiro2006). G. Tomaculopsis tomaculopsis, ellipsoidal fecal pellets or micro-coprolites with only two to some pellets across (from Breton, Reference Breton2011). H. Strobilorhaphe pusilla exhibits a small knob size, knobs are oval to circular with an irregular arrangement (from Rodríguez-Tovar et al., Reference Rodríguez-Tovar, Uchman, Payros, Orue-Etxebarria, Apellaniz and Molina2010). Scale bars are 2.0 mm for A, G and H, and 10.0 mm for all others.
Because of the abundance of bivalves at the quarry, fossilised remains of their eggs may be expected, although fossilised bivalves eggs are rare. Eggs of the most oviparous bivalves range from 60 to 80 μm (Helm & Bourne, Reference Helm and Bourne2004), much smaller than the diameter of the knobs in the specimen herein. Adult bivalves found in the quarry are also relatively small, 2 cm across or less (Klompmaker et al., Reference Klompmaker, Herngreen and Oosterink2010, figs 4–5), making it unlikely that they would have relatively large eggs, although a predictive relationship between egg length and adult female shell length in bivalves has not been found according to Phillips (Reference Phillips2007). The three most common fossil bivalves found in the Rhaetian shales at the quarry are Isocyprina sp., Tutcheria cloacina and Rhaetavicula contorta (Klompmaker et al., Reference Klompmaker, Herngreen and Oosterink2010, table 1). Given the large difference in size, the specimen is unlikely to represent fossilised bivalve eggs.
Fish eggs are soft, but they can be fossilised due to what is known as the ‘Medusa effect’ or very rapid or instantaneous fossilization (Martill, Reference Martill1989). Because of the well-preserved bivalves in the shales of this quarry, it is likely that rapid burial occurred occasionally, potentially causing this effect to take place. Sizes of fish eggs vary greatly depending of the fish species, but many are on average ~0.5 mm (Duarte & Alcaraz, Reference Duarte and Alcaraz1989), the same size as the knobs in the string. Most fish eggs are found fossilised in an egg sac (Cloutier, Reference Cloutier2010), but not in a string, as is found in this specimen.
Burrows and fecal pellets
The fossil burrow Granularia (Fig. 4C) exhibits ellipsoidal knobs that are very small compared to the tube diameter (> 15 knobs across) and are mostly arranged perpendicular to the burrow axis (see Crimes et al., Reference Crimes, Goldring, Homewood, van Stuijvenberg and Winkler1981, pl. 2.5, p. 969–970), unlike the specimen herein. Granularia is also flattened and may contain faecal pellets (Książkiewicz, Reference Książkiewicz1977, p. 68).
The burrow Ophiomorpha (Fig. 4F) is knobby and thought to be constructed primarily by decapod crustaceans, especially ghost shrimps (Frey et al., Reference Frey, Howard and Pryor1978). However, small burrows by these shrimps do not exhibit knobs typically. Moreover, body fossils of these shrimps are unknown from the Triassic fossil record so far, although Ophiomorpha is also reported from the Permian (Chamberlain & Baer, Reference Chamberlain and Baer1973). Ophiomorpha usually has a strong vertical component, unlike the specimen herein. Tunnels are typically (sub)circular in cross-section, whereas the specimen herein is flattened. This may not be due to compression because there is no evidence for this on individual knobs, and other fossils such as bivalves do not show evidence of compaction either.
Lucas et al. (Reference Lucas, Voigt, Lerner and Rainforth2013) indicated that the cross-section of the non-marine trace fossil Sphaerapus ( = Walpia), shown in Fig. 4E, was ellipsoidal or circular and that the non-coprolitic pellets were usually arranged in two rows. The placement in rows is different from the specimen herein. Pellets of the type species S. larvalis are 2–3 mm in diameter, which is larger than the knobs of the specimen herein. The specimen herein appears more flattened in cross-section and not circular or oval, and it varies more in diameter throughout its course.
The specimen resembles Strobilorhaphe pusilla (Fig. 4H) given the small knob size and knobs being oval to circular with an irregular arrangement (e.g. Książkiewicz, Reference Książkiewicz1968; Rodríguez-Tovar et al., Reference Rodríguez-Tovar, Uchman, Payros, Orue-Etxebarria, Apellaniz and Molina2010). The size of entire specimen also seems to fit. However, a definite ‘stem’ is missing in our specimen (Książkiewicz, Reference Książkiewicz1968, Reference Książkiewicz1977). Also, more knobs seem present across in the specimen herein.
The trace fossil Neonereites multiserialis shown in Fig. 4B is a knobby fossil burrow with knobs of 1–2 mm (Pickerill, Reference Pickerill1991) or 2–8 mm (Orr et al., Reference Orr, Benton and Trewin1996) in diameter. It has been reported that the structure is only in positive hyporelief, but positive epirelief is also possible (Benton, Reference Benton1982; Pickerill, Reference Pickerill1991). The arrangement of the knobs is comparable to that of the specimen herein, although knob size differs slightly.
Bown & Kraus (Reference Bown and Kraus1983) mentioned a circular cross-section for Edaphichnium (Fig. 4D), a non-marine trace fossil. The faecal pellets are ellipsoidal (their p. 100), unlike the specimen herein. The pellets can be smaller than 1 mm, similar in size to our specimen.
Tomaculopsis (Fig. 4G) consists of ellipsoidal faecal pellets or micro-coprolites with only two to three pellets across (Breton, Reference Breton2011). The specimen herein exhibits circular knobs that do not represent faecal pellets, most probably because no phosphorus was found using SEM-EDS.
The Rhaetian sediments in Quarry IV contain small coprolites (Klompmaker et al., Reference Klompmaker, Herngreen and Oosterink2010), but these sub-circular fecal pellets, very abundant in some bedding planes, were scattered throughout the beds and never grouped together as in the string (pers. obs. AAK).
Synthesis
After this investigation into similar fossils and trace fossils, the string of knobs probably represents a burrow, perhaps closest to Neonereites multiserialis. It is the most morphologically similar trace fossil that we have seen so far (sub-circular knobs, irregular arrangement of knobs, horizontal to bedding plane). EDS analysis suggests that the specimen is unlikely to represent a coprolite because of a lack of phosphorus present in the string of knobs (Eriksson et al., Reference Eriksson, Lindgren, Chin and Mansby2011; Zatoń & Rakociński, Reference Zatoń and Rakociński2014). Even though the specimen itself has been pyritised, we would expect to see some phosphorus in the matrix surrounding the specimen, showing up at 2.00 KeV in the EDS graph, in which case it could represent a coprolite. However, no phosphorus was found there either (Fig. 3B). According to Häntzschel et al. (Reference Häntzschel, El-Baz and Amstutz1968), calcium phosphate is the most common composition for coprolites, but there are several other possible compositions, including organic matter and clays. The lack of phosphorus in the specimen, therefore, does not entirely discount the possibility of a coprolite. Vertebrate coprolites do not show clear knobs usually (e.g. Hunt et al., Reference Hunt, Lucas, Spielmann, Lerner, Lucas and Spielmann2007, Reference Hunt, Lucas, Milàn, Spielmann, Hunt, Lucas, Milàn and Spielmann2012). Although the overall shape is often circular among coprolites (Häntzschel et al., Reference Häntzschel, El-Baz and Amstutz1968), it seems less common among the very small forms, which are more likely to be oval or rod-shaped (e.g. Schäfer, Reference Schäfer1953; Häntzschel et al., Reference Häntzschel, El-Baz and Amstutz1968; Arakawa, Reference Arakawa1971). We refrain from ascribing the specimen to a known trace fossil genus because it is only a single specimen and it is incompletely preserved. To our knowledge, the trace fossil studied herein is not reported from the Rhaetian of Great Britain (see Macquaker, Reference Macquaker1987; Swift & Duffin, Reference Swift, Duffin, Swift and Martill1999; Allington-Jones et al., Reference Allington-Jones, Braddy and Trueman2010).
Palaeoenvironment
The specimen studied herein is the only relatively large trace fossil known from the Dutch Rhaetian shales thus far. Additionally, the second author remembers a low number of pyritised, very small, < 10 mm long, sinuous, indeterminable traces along the bedding plane. These traces are not preserved anymore due to pyrite disintegration over time since the specimens were collected in 2007 and 2008. The uncommon presence of trace fossils suggests a low oxygen content of the Rhaetian bottoms at the time of deposition, as does the presence of carbon in the dark shales (see Fig. 3). The relatively low number of other invertebrate species may support this as well, although bivalves can be abundant in some layers (Klompmaker et al., Reference Klompmaker, Herngreen and Oosterink2010). Unlike the probably storm-related bone beds containing coarser sediment particles, these bivalves are unlikely to have been transported by a storm event into an anoxic environment because they are well-preserved (Klompmaker et al., Reference Klompmaker, Herngreen and Oosterink2010, fig. 5). These authors (p. 601) also reported that the valves of the second most common bivalve, Tutcheria cloacina, were articulated in more than 10% of the cases. Moreover, the sediments surrounding the bivalves were the same as in other layers. As for the bivalves and ophiuroids, the burrowing organisms must have been present during an episode of higher oxygen content. Burrow depth may have been restricted given that the fossil burrow parallels the bedding plane. Oxygen may have been present just above the sediment-water interface, whereas the bottom profile was largely devoid of oxygen.
Acknowledgements
Henk Diependaal and Jelle Reumer are thanked for information on fish fossils from the Rhaetian shales. The Working Group Muschelkalk and the exploiting Ankerpoort Company are thanked for permission to access and study the Rhaetian sediments. Ann Heatherington is thanked for helping with SEM and EDS images. We are grateful for permission by the publishers Elsevier, John Wiley and Sons, and Taylor and Francis, and the author G. Breton to use the images in Figs 1 and 4. We thank our reviewers, Spencer G. Lucas and Bert Boekschoten, for useful comments. This work was in part supported by the Jon L. and Beverly A. Thompson Endowment Fund to A.A.K. This is University of Florida Contribution to Paleobiology 686.