Introduction
A new sulfite mineral species, mikenewite, ideally Mn2+(S4+O3)⋅3H2O, was discovered on a specimen collected from the San Judas Chimney, Ojuela mine, Mapimí, Durango, Mexico. It is named in honour of Mr. Michael Edwin New (1943–2022), the owner of ‘Top Gem Minerals Inc. of Tucson’. Mike New was a prominent wholesale mineral dealer and specimen miner. In the past half century, he provided the world with a wide variety of mineral specimens from the Ojuela mine where he had permission to mine and export. Mike New's most spectacular find took place in 1981 in the San Judas chimney where fabulous specimens of purple adamite were encountered. Today, many of these specimens are found worldwide in numerous museums and private collections. The type sample of mikenewite is from a specimen mined by Mike New and his miners. Furthermore, Mike New was kind and generously donated many mineral specimens to local schools for students’ education, including the University of Arizona. The new mineral and its name (symbol Mnw) have been approved by the Commission on New Minerals, Nomenclature and Classification (CNMNC) of the International Mineralogical Association (IMA2022-102, Yang et al., Reference Yang, Jenkins, McGlasson, Gibbs and Downs2023). The co-type samples have been deposited at the University of Arizona Alfie Norville Gem and Mineral Museum (Catalogue # 22724) and the RRUFF Project (deposition # R210021) (http://rruff.info) (Lafuente et al., Reference Lafuente, Downs, Yang, Stone, Armbruster and Danisi2015).
Sulfite minerals are quite rare in Nature and only 12 minerals contain the (S4+O3)2– anionic group in the current IMA-approved mineral list at http://cnmnc.units.it/ (Table 1). Interestingly, all of these minerals were described after the 1980s. Sulfites are generally considered as unstable intermediate products of the oxidation process which transforms sulfides into sulfates and special conditions are necessary to preserve them in Nature, accounting for their paucity (Weidenthaler et al., Reference Weidenthaler, Tillmanns and Hentschel1993; Vignola et al., Reference Vignola, Gatta, Rotiroti, Gentile, Hatert, Baijot, Bersani, Risplendente and Pavese2016). Nevertheless, sulfite materials have been studied extensively because of their high catalytic activity in the oxidation reaction SO2 + ½O2 + H2O → H2SO4, which is of great interest in industrial applications. For example, sulfite-based advanced oxidation processes have been applied for various removals of contaminants and environmental remediations (e.g. Zhou et al., Reference Zhou, Huang, Zeng, Yang, Xu, Zhou and Lyu2022; Ren et al., Reference Ren, Chu, Li, Lai, Zhang, Liu and Li2023). Recovery of tellurium and selenium from (Te,Se)-rich materials by alkaline sulfide leaching followed by sodium sulfite precipitation has been developed (e.g. Zhang and Chen, Reference Zheng and Chen2014; Guo et al., Reference Guo, Xu, Li, Tian, Xu and Zhang2017). Sulfite materials are used widely as antimicrobial agents and antioxidants for food products, beverages and pharmaceutical products (Sezgintürk, Reference Sezgintürk and Dinçkaya2005; Yin et al., Reference Yin, Li, Yue, Chao, Zhang and Huo2017; Fehér et al., Reference Fehér, Sajó, Kótai, Szakáll, Ende, Effenberger, Mihály, Szabó and Koller2021 and references therein). This paper describes the physical and chemical properties of mikenewite and its crystal structure determined from single-crystal X-ray diffraction data.
Table 1. List of IMA approved minerals containing the sulfite anionic group (S4+O3)2–.
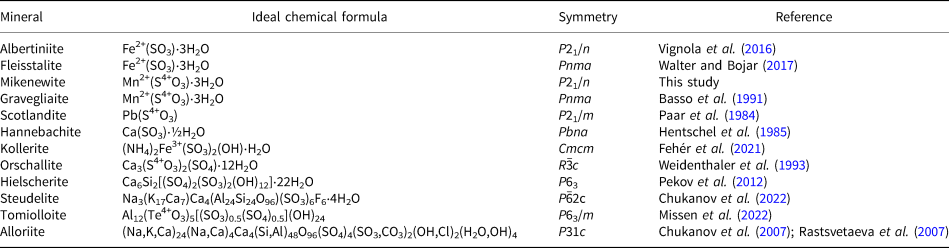
Sample description and experimental methods
Occurrence
Mikenewite was found on a specimen (Fig. 1) collected from the San Judas Chimney, Ojuela mine (25°47'36''N, 103°47'27''W), Mapimí, Durango, Mexico. Associated minerals include goethite FeO(OH), cryptomelane K(Mn4+7Mn3+)O16, adamite Zn2(AsO4)(OH) and lotharmeyerite CaZn2(AsO4)2⋅2H2O. A review on the geology and mineralogy of the Ojuela mine has been given by Moore and Megaw (Reference Moore and Megaw2003), which contains a section of the mining history at the Ojuela mine by Mr. Mike New (pages 47–53), after whom we name the new mineral. The Ojuela mine is the type locality for seven minerals, including lotharmeyerite, CaZn2(AsO4)2⋅2H2O (Dunn, Reference Dunn1983), mapimite, Zn2Fe3+3(AsO4)3(OH)4⋅10H2O (Cesbron et al., Reference Cesbron, Romero and Williams1981), metaköttigite, (Zn,Fe3+)3(AsO4)2⋅8(H2O,OH) (Schmetzer et al., Reference Schmetzer, Amthauer, Stähle and Medenbach1982), miguelromeroite, Mn5(AsO3OH)2(AsO4)2(H2O)4 (Kampf, Reference Kampf2009), ojuelaite, ZnFe3+2(AsO4)2(OH)2⋅4H2O (Cesbron et al., Reference Cesbron, Romero and Williams1981), paradamite, Zn2(AsO4)(OH) (Switzer, Reference Switzer1956) and the new mineral mikenewite, described herein.
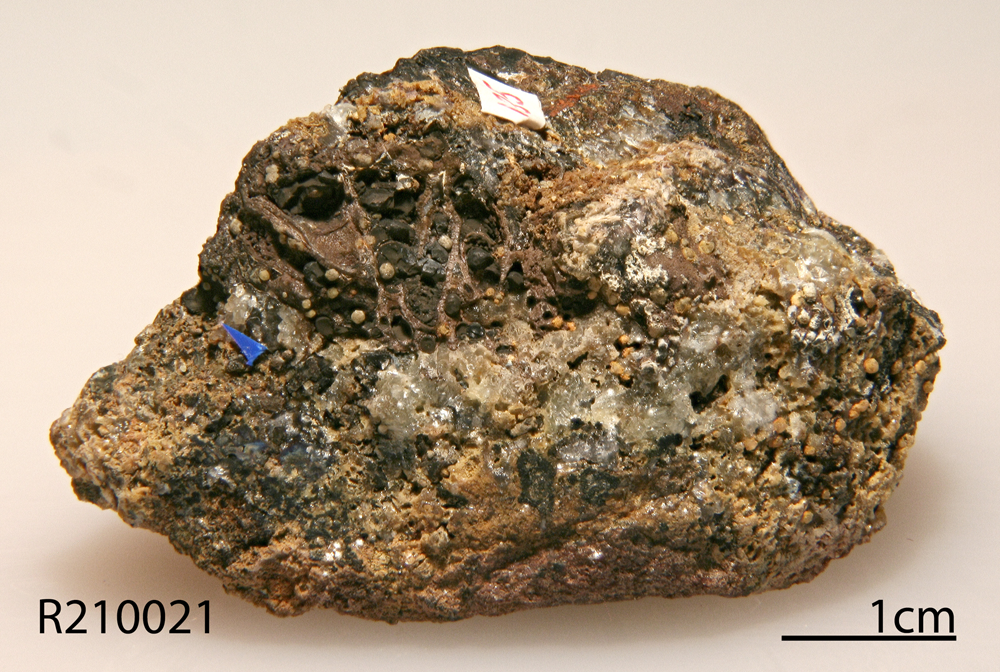
Figure 1. The specimen on which the new mineral mikenewite was found (Specimen number R210021).
Physical and chemical properties and Raman spectra
Mikenewite occurs as spheres of platy crystals (Figs 2 and 3) on a matrix of goethite, cryptomelane, adamite and lotharmeyerite. Individual crystals of mikenewite are up to 0.50 × 0.10 × 0.04 mm. Mikenewite is yellowish in transmitted light, transparent with a white streak and vitreous lustre. It is brittle and has a Mohs hardness of 2½–3. Cleavage is perfect on {101}. The density measured by flotation in heavy liquids is 2.48(5) g/cm3 and the calculated density is 2.467 g/cm3 based on the empirical chemical formula and unit-cell volume from single-crystal X-ray diffraction data. Optically, mikenewite is biaxial (+), with α = 1.606(5), β = 1.614(5), γ = 1.627(1) (determined in white light), 2V(meas.) = 69(3)° and 2V(calc.) = 77°. The pleochroism is from grey to yellowish and dispersion is weak, with r > v. The calculated Gladstone–Dale compatibility index based on the empirical formula is 0.032 (excellent) (Mandarino, Reference Mandarino1981). Mikenewite is insoluble in water, but dissolves slowly in hydrochloric acid.
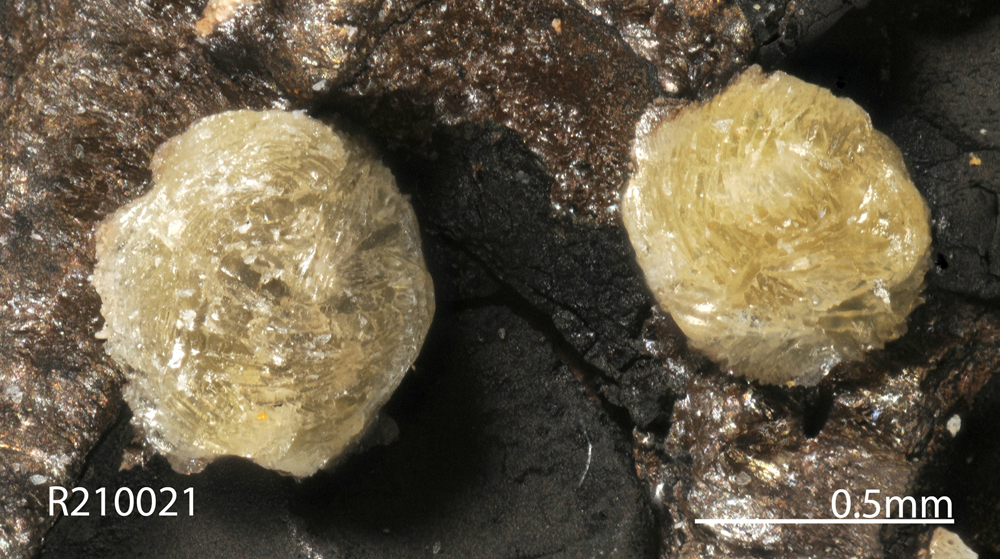
Figure 2. A microscopic view of yellow spheres made of platy mikenewite crystals (Specimen number R210021).
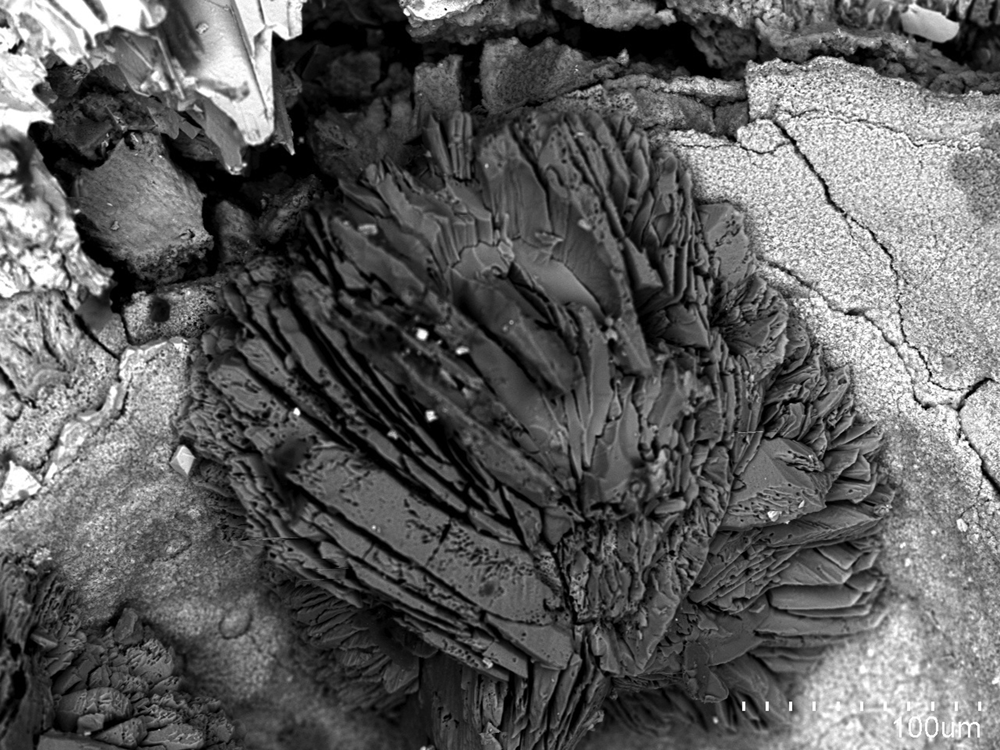
Figure 3. A back-scattered electron image of aggregates (spheres) of platy mikenewite crystals (Specimen number R210021).
The chemical composition of mikenewite was determined using a Cameca SX-100 electron microprobe (wavelength dispersive spectroscopy mode, 15 kV, 4 nA and a beam diameter of 20 μm). The standards used for the probe analysis are given in Table 2, along with the determined compositions (7 analysis points). The resultant chemical formula, calculated on the basis of 6 O atoms per formula unit (from the structure determination), is (Mn0.86Zn0.12Fe0.04Ca0.02)Σ1.04(S0.98O3)⋅3H2O, which can be simplified to (Mn,Zn,Fe)(SO3)⋅3H2O.
Table 2. Analytical chemical data (in wt.%) for mikenewite.
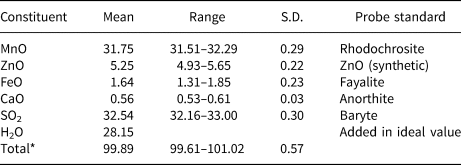
* Mikenewite is extremely prone to the electron beam damage. To minimise the damage by the electron beam, we had to lower the current to 4 nA with a beam size of 20 μm. S.D. – standard deviation.
The Raman spectrum of mikenewite was collected on a randomly oriented crystal with a Thermo Almega microRaman system, using a solid-state laser with a wavelength of 532 nm at 75 mW power and a thermoelectric cooled CCD detector. The laser is partially polarised with 4 cm–1 resolution and a spot size of 1 μm.
X-ray crystallography
Both the powder and single-crystal X-ray diffraction data for mikenewite were collected on a Bruker APEX2 CCD X-ray diffractometer equipped with graphite-monochromatised MoKα radiation. The measured powder X-ray diffraction data are in Table 3. The unit-cell parameters obtained from the powder X-ray diffraction data using the program by Downs et al. (Reference Downs, Bartelmehs, Gibbs and Boisen1993) are: a = 6.640(4), b = 8.878(6), c = 8.789(6) Å, β = 96.08(6)° and V= 514.4(4) Å3.
Table 3. Powder X-ray diffraction data (d in Å, I in %) of mikenewite.*

*The strongest lines are given in bold.
Single-crystal X-ray diffraction data were collected from a 0.06 × 0.05 × 0.05 mm fragment with frame widths of 0.5° in ω and 30 s counting time per frame. All intensity data were corrected for X-ray absorption using the Bruker program SADABS (Bruker, 2001). The systematic absences of reflections suggest the unique space group P21/n. The crystal structure of mikenewite was solved and refined using SHELX2018 (Sheldrick, Reference Sheldrick2015a, Reference Sheldrick2015b). During the structure refinements, the small amount of Ca was ignored and Fe was treated as Mn due to their similar X-ray scattering powers. The refined Mn/Zn ratio at the octahedral site is 0.90/0.10, which is very close to that (0.88/0.12, normalised) measured from the electron microprobe analysis. All H atoms were located through the difference-Fourier syntheses. The positions of all atoms were refined with anisotropic displacement parameters, except for H atoms, which were refined with a fixed isotropic displacement parameter (U iso = 0.05). The refinement statistics are given in Table 4. Final atomic coordinates and displacement parameters are given in Tables 5 and 6, respectively. Selected bond distances and the hydrogen bonding scheme are presented in Tables 7 and 8, respectively. The bond-valence sums were calculated using the parameters given by Brown (Reference Brown2009) (Table 9). The crystallographic information file has been deposited with the Principal Editor of Mineralogical Magazine and is available as Supplementary material (see below).
Table 4. Comparison of crystallographic data of mikenewite with related minerals.

Table 5. Fractional atomic coordinates and equivalent isotropic displacement parameters (Å2) for mikenewite.
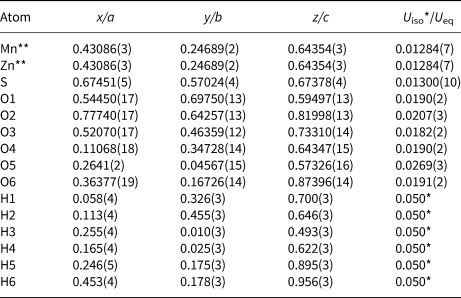
** Occupancies: Mn = 0.904(7); Zn = 0.096(7)
Table 6. Atomic displacement parameters (Å2) for mikenewite.

Table 7. Selected bond distances (Å) for mikenewite and gravegliaite.

*OAV – octahedral angle variance and OQE – octahedral quadratic elongation (Robinson et al., Reference Robinson, Gibbs and Ribbe1971).
Table 8. Hydrogen-bond geometry (Å, °) in mikenewite (D = donor, A = acceptor).
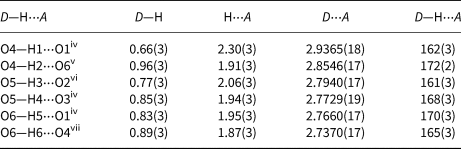
Symmetry codes: (iv): −x + ½, y − ½, −z + 3/2; (v) −x + ½, y + ½, −z + 3/2; (vi) x − ½, −y + ½, z − ½; (vii) x + ½, −y + ½, z + ½.
Table 9. Bond-valence sums for mikenewite.
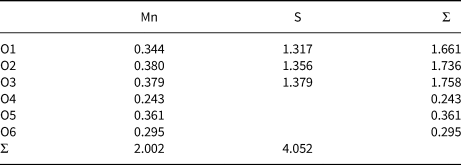
Crystal-structure description and discussion
Mikenewite is the natural analogue of synthetic α-Mn2+(S4+O3)⋅3H2O (Baggio and Baggio, Reference Baggio and Baggio1976; Lutz et al., Reference Lutz, El-Suradi and Engelev1977; Engelen and Freiburg, Reference Engelen and Freiburg1979; Johansson and Lindqvist, Reference Johansson and Lindqvist1980), as well as the Mn-analogue of albertiniite, Fe2+(S4+O3)⋅3H2O (Johansson and Lindqvist, Reference Johansson and Lindqvist1979; Vignola et al., Reference Vignola, Gatta, Rotiroti, Gentile, Hatert, Baijot, Bersani, Risplendente and Pavese2016). It is noteworthy that, in addition to monoclinic P21/n mikenewite and albertiniite, both Mn2+(S4+O3)⋅3H2O and Fe2+(S4+O3)⋅3H2O possess an orthorhombic Pnma polymorph, namely, gravegliaite (Baggio and Baggio, Reference Baggio and Baggio1976; Basso et al., Reference Basso, Lecchetti and Palenzona1991; Gonschorek et al., Reference Gonschorek, Weitzel and Engelen1996; Diaz de Vivar et al., Reference de Vivar M.E., Baggio, Garland and Baggio2006) and fleisstalite (Walter and Bojar, Reference Walter and Bojar2017).
The crystal structure of mikenewite is characterised by each Mn atom coordinated by six O atoms, three from different sulfite O atoms and three from H2O molecules, giving rise to a slightly distorted octahedron (Table 7). Each S4+O3 group is bonded to three Mn atoms, resulting in a sheet parallel to (101) with the sheet composition of Mn2+(S4+O3)⋅3H2O (Fig. 4). Such sheets, stacked along [10$\bar{1}$], are joined together by hydrogen bonds (Fig. 5), accounting for the perfect cleavage of the mineral. In comparison, the crystal structure of gravegliaite consists of chains formed by MnO6 octahedra and S4+O3 groups, which run parallel to [010] and are linked together by hydrogen bonds (Baggio and Baggio, Reference Baggio and Baggio1976; Basso et al., Reference Basso, Lecchetti and Palenzona1991; Diaz de Vivar et al., Reference de Vivar M.E., Baggio, Garland and Baggio2006). Although Mn2+ cations in mikenewite and gravegliaite have a similar coordination environment (3O2– + 3H2O), the MnO6 octahedron in mikenewite appears to be noticeably more distorted than that in gravegliaite in terms of both OAV (octahedral angle variance) and OQE (octahedral quadratic elongation) (Robinson et al., Reference Robinson, Gibbs and Ribbe1971) (Table 7).
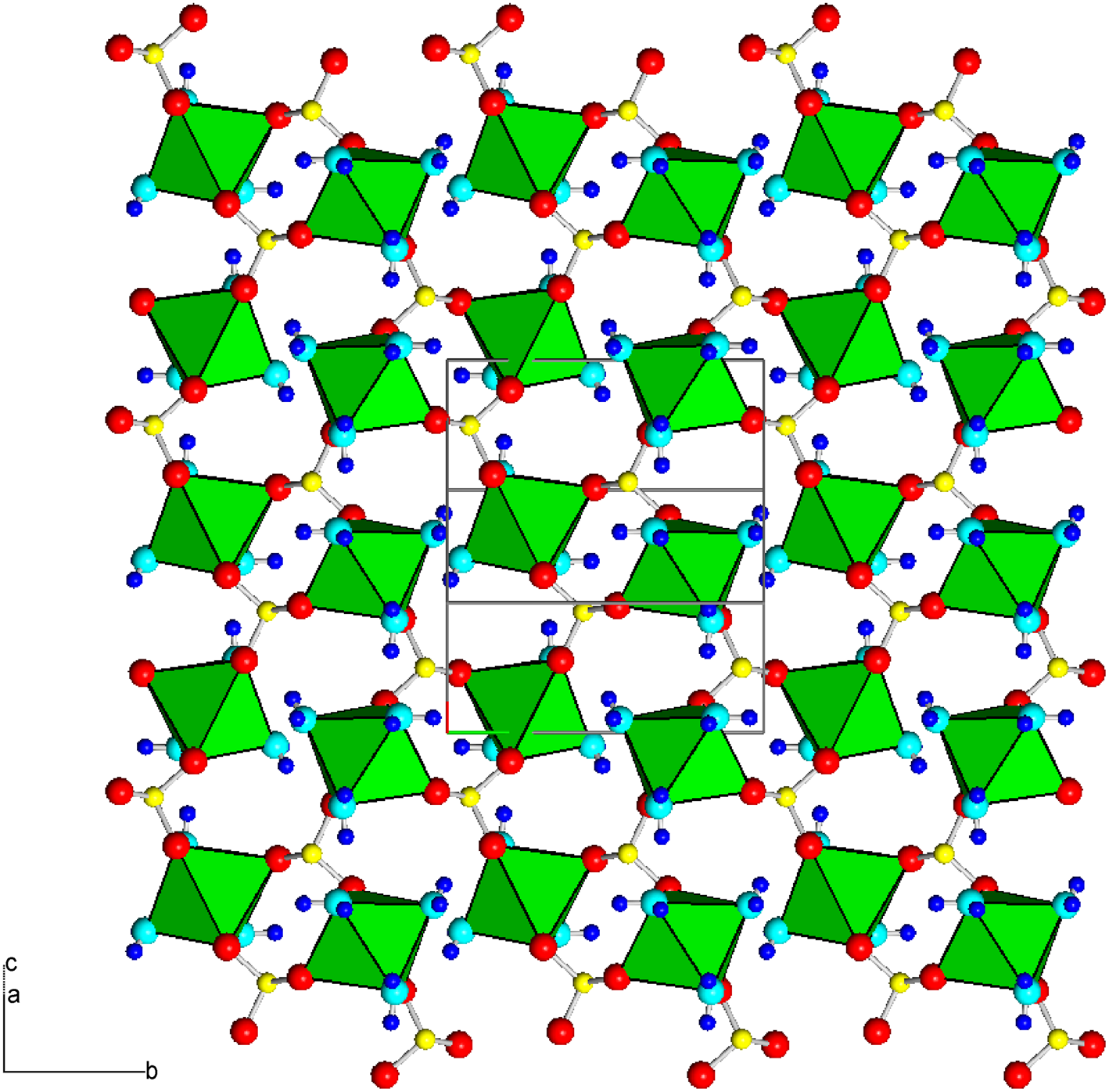
Figure 4. Crystal structure of mikenewite, showing a sheet [‖(101)] consisting of [MnO3(H2O)3] octahedra (green) connected by (S4+O3)2– groups. Red, aqua, yellow and small blue spheres represent O from (S4+O3)2–, O from H2O, S and H atoms, respectively. Drawn using XtalDraw (Downs and Hall-Wallace, Reference Downs and Hall-Wallace2003).
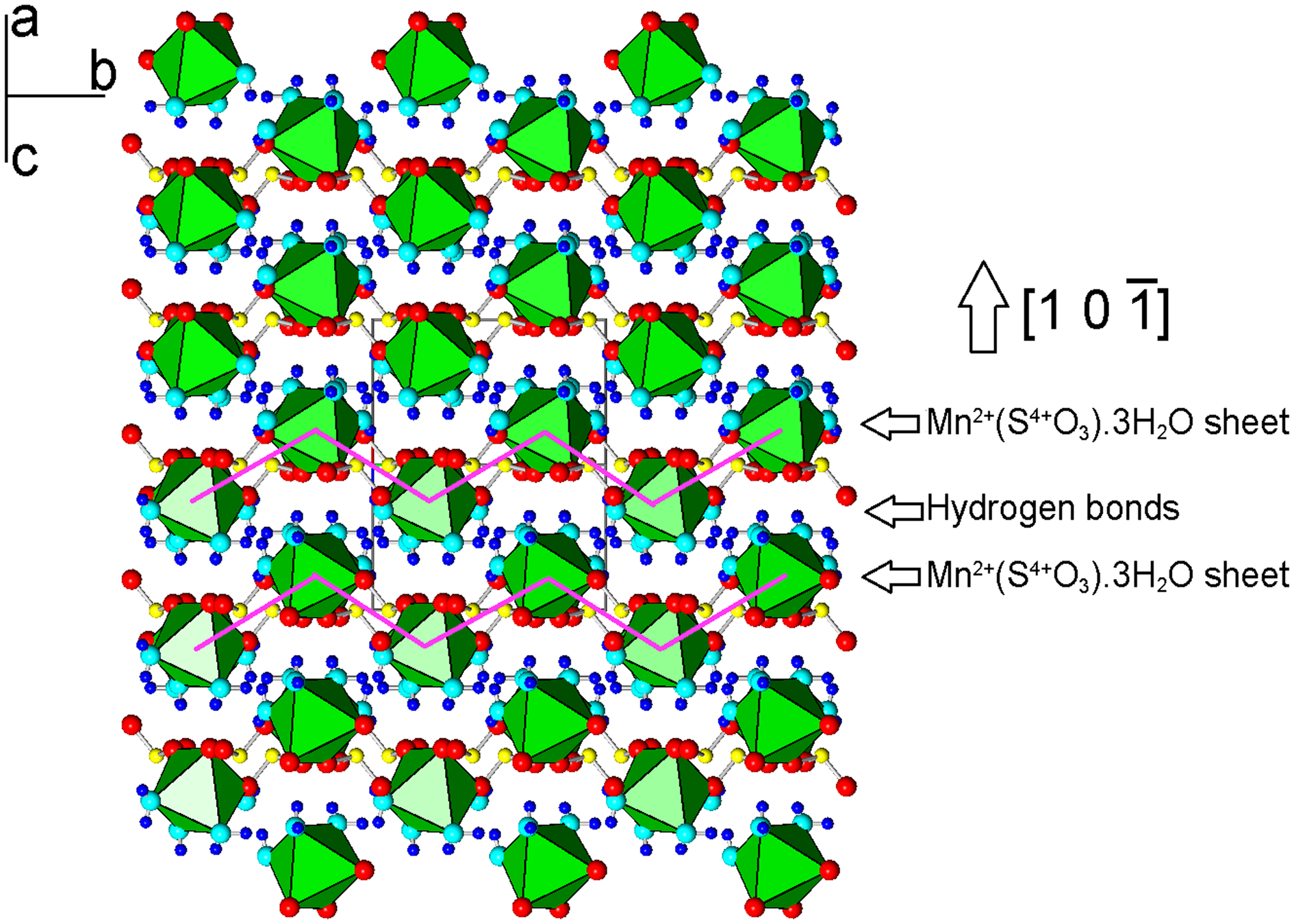
Figure 5. Crystal structure of mikenewite, showing the stacking of sheets consisting of [MnO3(H2O)3] octahedra (green) connected by (S4+O3)2– groups. Two sheets are specifically indicated by the zigzag purple lines. These sheets are stacked along [1 0 $\bar{1}$] and are linked together by hydrogen bonds. Drawn using XtalDraw (Downs and Hall-Wallace, Reference Downs and Hall-Wallace2003). The legends are the same as in Fig. 4.
The Raman spectrum of mikenewite is plotted in Fig. 6, along with that of isostructural albertiniite, Fe2+(S4+O3)⋅3H2O (Vignola et al., Reference Vignola, Gatta, Rotiroti, Gentile, Hatert, Baijot, Bersani, Risplendente and Pavese2016) for comparison. The strong resemblance between the two spectra is evident. Based on the Raman spectroscopic study on albertiniite (Vignola et al., Reference Vignola, Gatta, Rotiroti, Gentile, Hatert, Baijot, Bersani, Risplendente and Pavese2016), we made the following tentative assignments of major Raman bands for mikenewite. The broad bands (relatively strong) centred at 3219 and 3365 cm–1 and those (very weak) between 1500 and 1700 cm–1 are due to the O–H stretching and H–O–H bending modes in H2O groups, respectively. The bands between 730 and 1080 cm–1 are ascribable to the S4+–O stretching vibrations within the S4+O3 groups, whereas those from 430 to 700 cm–1 originate from the O–S4+–O bending modes. The bands below 380 cm–1 are associated mainly with the rotational and translational modes of S4+O3 groups, as well as the (Mn2+)–O interactions and lattice vibrational modes.
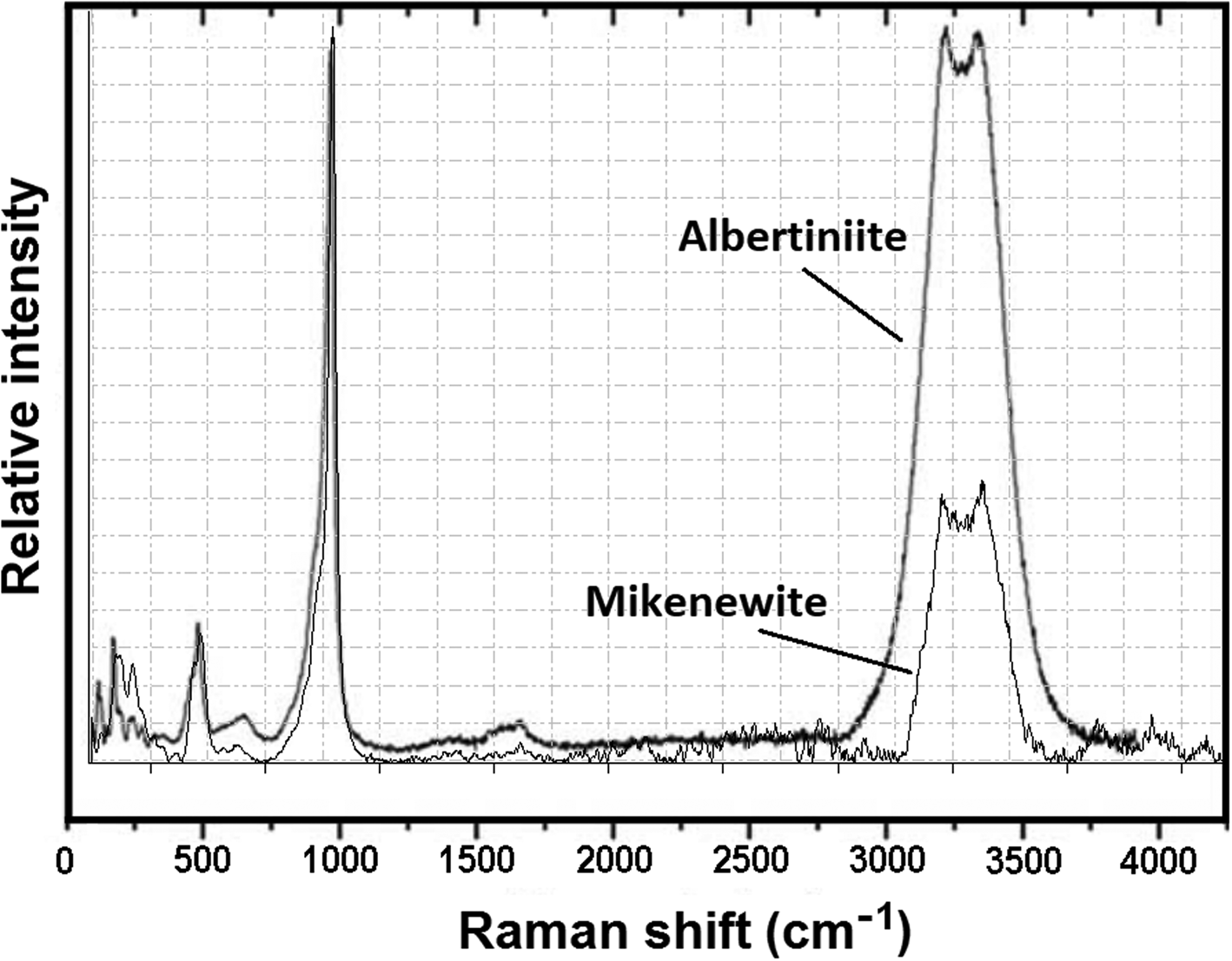
Figure 6. Raman spectrum of mikenewite, along with that of albertiniite (taken from Vignola et al., Reference Vignola, Gatta, Rotiroti, Gentile, Hatert, Baijot, Bersani, Risplendente and Pavese2016) for comparison.
The calculated bond-valence sums for mikenewite (Table 9) indicate that all three O atoms (O1, O2 and O3) in the sulfite S4+O3 group are noticeably under-bonded (1.66, 1.74 and 1.76 valence units, respectively). Their deficiencies in bond-valence sums are compensated by H bonds, as all these O atoms are engaged in the hydrogen bonding as acceptors (Table 8). In particular, O1 is the acceptor for two H bonds (O4–H1⋅⋅⋅O1 = 2.937 Å and O6–H5⋅⋅⋅O1 = 2.766 Å). According to the correlation between O–H stretching frequencies (cm–1) and O—H⋅⋅⋅O distances (Å) for minerals (Libowitzky, 1999), the Raman bands between 3200 and 3380 cm–1 we observed for mikenewite correspond well with the O–O distances between 2.73 and 2.94 Å (Table 8).
In addition to Mn2+- and Fe2+-sulfite trihydrates, a number of hydrous M 2+-sulfite compounds (M = Mg, Zn, Co, Ca, Cu, etc) have also been synthesised, including several Zn sulfites, such as ZnSO3⋅2½H2O, ZnSO3⋅2H2O, ZnSO3⋅½H2O and ZnSO3 (e.g. Lutz et al., Reference Lutz, El-Suradi and Engelev1977; Inoue et al., Reference Inoue, Grijalva, Inoue and Fernando1999; Lagosz and Malolepszy, Reference Lagosz and Malolepszy2003; Giorgi et al., Reference Giorgi, Lyutov and Lyutov2011). Because the Ojuela mine is particularly rich in Zn, as exemplified by numerous Zn-bearing minerals, typically adamite and lotharmeyerite (Moore and Megaw, Reference Moore and Megaw2003), one may postulate, based on the discovery of mikenewite, the possibility of finding Zn-bearing sulfites there. The fact that the mikenewite we examined in this study contains 12% Zn substituting for Mn lends good support to this conjecture.
Acknowledgements
The constructive comments by Drs. Peter Leverett, Pietro Vignola and Oleg Vereshchagin are greatly appreciated. This study was funded by the Feinglos family and Mr. Michael M. Scott.
Supplementary material
The supplementary material for this article can be found at https://doi.org/10.1180/mgm.2023.24.
Competing interests
The authors declare none.