Through four seasons of field surveys, excavations, and laboratory analyses (1961–1964), Richard S. MacNeish and the Tehuacan Archaeological-Botanical Project (TABP) were the first to systematically explore the archaeology of the Tehuacan Valley of south-central Mexico, which is today part of the Tehuacan-Cuicatlan Valley UNESCO World Heritage Site (MacNeish Reference MacNeish and Byers1967a). The arid conditions of the valley have resulted in the exceptional preservation of organic materials within a series of dry caves, enabling the TABP to recover and document thousands of specimens of early domesticated plants, including chili peppers, avocados, squash, beans, and maize (Long et al. Reference Long, Benz, Donahue, Timothy Jull and Toolin1989; MacNeish Reference MacNeish and Byers1967b; Mangelsdorf et al. Reference Mangelsdorf, MacNeish, Galinat and Byers1967; Smith Reference Smith and Byers1967, Reference Smith2005). Although nearly 200 radiocarbon ages from archaeological settlements across the valley have been produced by the TABP and subsequent projects (Aiuvalasit et al. Reference Aiuvalasit, Neely and Bateman2010; Johnson and MacNeish Reference Johnson, MacNeish and MacNeish1972; Kaplan and Lynch Reference Kaplan and Lynch1999; Long et al. Reference Long, Benz, Donahue, Timothy Jull and Toolin1989; Neely et al. Reference Neely, Aiuvalasit and Clause2015; Smith Reference Smith2005; Torres-Rodríguez et al. Reference Torres-Rodríguez, Vallebueno-Estrada, González, Cook, Montiel and Vielle-Calzada2018), the chronology for the Preceramic phases and the timing of the beginning of plant cultivation within the Tehuacan Valley have remained topics of scrutiny and debate (Fennell Reference Fennell2001; Flannery and MacNeish Reference Flannery and MacNeish1997; Hardy Reference Hardy1996, Reference Hardy1999; MacNeish Reference MacNeish1997; Rosenswig Reference Rosenswig2015:123–125).
Coxcatlan Cave is a dry rock shelter located within the southern portion of the Tehuacan Valley, just north of the border with Oaxaca (Figure 1). The cave has been the focus for much of the discussion of the chronology of human activities because of its deep stratigraphy and excellent preservation: radiocarbon dating was combined with a stratigraphic record of material culture to establish the foundation of the broader Tehuacan chronology (Johnson and MacNeish Reference Johnson, MacNeish and MacNeish1972). Later studies using direct AMS radiocarbon assays of botanical samples, however, found that approximately 75% of the plant specimens excavated from Coxcatlan Cave were drastically out of sequence (Fritz Reference Fritz1994; Kaplan and Lynch Reference Kaplan and Lynch1999; Long et al. Reference Long, Benz, Donahue, Timothy Jull and Toolin1989; Smith Reference Smith2005), casting doubt on the integrity of the stratigraphy. Hardy (Reference Hardy1996, Reference Hardy1999) further criticized the TABP chronology, arguing that insufficient evidence existed to classify typological changes in the stone tools over time, that the complexity of the stratigraphy in the lower portion of the cave precluded making chronological arguments, and that variation in the proportions of different faunal remains did not represent environmental changes over time. Subsequent assessments of Hardy's (Reference Hardy1996) analysis, however, refuted most of her critiques (Fennell Reference Fennell2001; Flannery and MacNeish Reference Flannery and MacNeish1997). Although MacNeish (Reference MacNeish1997) strongly maintained that the fundamental chronology of Coxcatlan Cave was intact, uncertainties linger about the stratigraphy of the cave and hence the chronology of the region. Additionally, the lowest levels of the rock shelter (Ajuereado phase) have not yet been dated, and the timing of the initial arrival of humans in the area remains speculative.
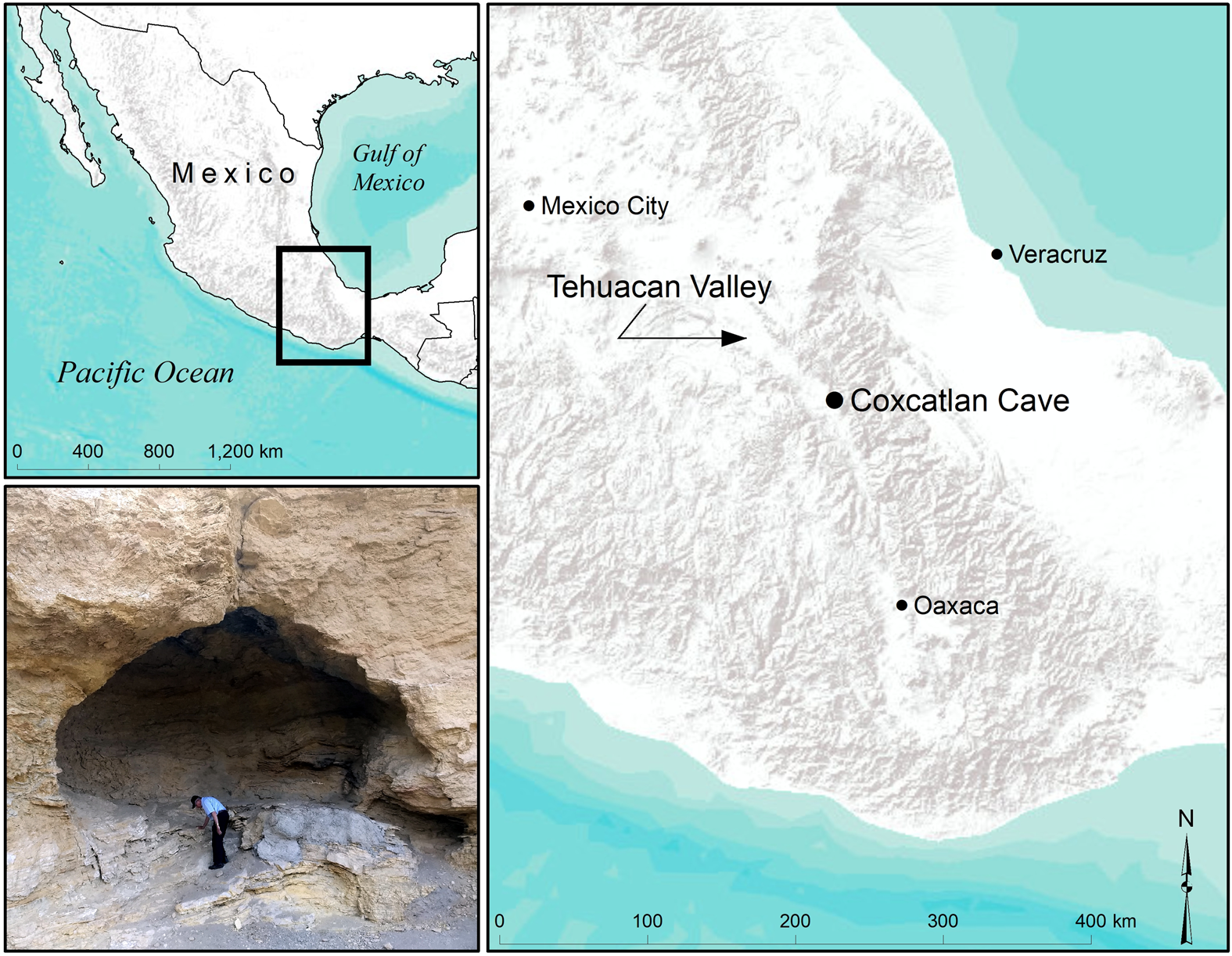
Figure 1. Map displaying the location of Coxcatlan Cave within the Tehuacan Valley (photograph by Andrew Somerville). (Color online)
In an effort to reassess the Preceramic sequence of Coxcatlan Cave and refine the chronology of the Tehuacan Valley, this article presents 14 new 14C radiocarbon ages produced by accelerator mass spectrometry (AMS) of faunal bones excavated from the rock shelter by the TABP. We compare our resulting radiocarbon ages with previously published radiocarbon data from the cave and construct a chronological Bayesian model of the phases of the Preceramic sequence. In our discussion of the data, we pay particular attention to the age estimates and archaeological context from the Ajuereado phase zones (XXVIII–XXIII) at the bottom of the cave.
Coxcatlan Cave and the Preceramic Period
Coxcatlan Cave is a north-facing, dry rockshelter site in the southern portion of the Tehuacan Valley along the alluvial slopes of the Sierra Madre Oriental. The cave is several meters above the valley floor on a low bluff. The shelter extends approximately 30 m in length and 8 m in width. Within the cave, the TABP excavated to a maximum depth of approximately 4 m (Johnson and MacNeish Reference Johnson, MacNeish and MacNeish1972:229), documenting 28 horizontal stratigraphic levels, or “habitation zones,” and 42 discrete occupational episodes (Fowler and MacNeish Reference Fowler, MacNeish and MacNeish1972). The zones occupied by people who did not make or use pottery (XXVIII–VIII), hereafter referred to as the Preceramic zones, are the earliest levels of the rock shelter. These zones have been divided into four cultural phases based on changes in the stone tool technology, basketry and woven matting, and settlement patterns across the valley and through cross-cultural comparisons with sites in Oaxaca (Flannery and Spores Reference Flannery, Spores, Flannery and Marcus1983; MacNeish Reference MacNeish1997). From oldest to youngest, these are the Ajuereado, El Riego, Coxcatlan, and Abejas phases. The characteristics of the Preceramic phases are described in detail by MacNeish and colleagues (MacNeish Reference MacNeish1964, Reference MacNeish and Byers1967c:23–24, Reference MacNeish, Evans and Webster2001a; MacNeish et al. Reference MacNeish, Nelken-Terner and Johnson1967:231–233). Here we provide a brief summary of the material and behavioral correlates.
The earliest evidence for human occupation in the Tehuacan Valley occurred during the Ajuereado phase. This phase was split into Early (Zones XXVIII–XXV) and Late (Zones XXIV and XXIII) components by the TABP based on differences in artifact types and associated fauna. Minimally worked blades, end-scrapers, and choppers are present within the zones of both subphases, but Late Ajuereado deposits also contained bifacial projectile points; that is, Lerma and Abasolo points. In Coxcatlan Cave, the Early Ajuereado phase was associated with remains of Pleistocene fauna, but Late Ajuereado phase deposits contained fauna similar to all subsequent phases (Flannery Reference Flannery and Byers1967). The human population was very small in the valley, and residents were likely organized into microbands composed of small family groups.
During the subsequent El Riego phase, nomadic hunting and gathering continued as the primary subsistence strategies. End-scrapers and choppers continued to be used, but at least 10 new lithic artifact types appeared in the archaeological record, including El Riego, Flacco, and Nogales points; stone mortars; and boulder metate-milling stones (MacNeish et al. Reference MacNeish, Nelken-Terner and Johnson1967:231). Perishable items, including nets, coiled baskets, and mats, were also associated with El Riego phase deposits. MacNeish argued that sites of this period from across the valley included camps for small family units or microbands, as well as camps for macrobands of approximately 15–25 people who gathered seasonally. The presence of avocado seeds (Persea americana), chili peppers (Capsicum annuum), and prickly pear cactus (Opuntia spp.) in deposits associated with the El Riego phase suggests the possibility that people were practicing an incipient form of plant cultivation at this time (Smith Reference Smith and Byers1967:232).
The Coxcatlan phase followed El Riego and was characterized by further increases in the richness and diversity of artifact types, including the addition of oblong manos, stone bowls, and new projectile points such as Coxcatlan points (MacNeish et al. Reference MacNeish, Nelken-Terner and Johnson1967:66). Nomadic hunting and gathering likely remained people's primary subsistence strategies, but the cultivation of several plant species may have increased during this period (MacNeish et al. Reference MacNeish and Byers1967b:23). There were fewer sites within the valley, but they were larger in size and may have been occupied for a greater portion of the year.
The Abejas phase was the final phase of the Tehuacan Preceramic sequence and was characterized by increasing sedentism. People built pit-houses for the first time and may have lived in them year-round. Importantly, this period saw the first introduction of domesticated maize (Zea mays) to the valley and to Coxcatlan Cave in particular. Although MacNeish and the TABP had originally suggested that maize arrived during the Coxcatlan phase, subsequent AMS dating demonstrated that the earliest samples dated to the Abejas phase (Crane and Griffin Reference Crane and Griffin1962; Long et al. Reference Long, Benz, Donahue, Timothy Jull and Toolin1989; Torres-Rodríguez et al. Reference Torres-Rodríguez, Vallebueno-Estrada, González, Cook, Montiel and Vielle-Calzada2018). After the Abejas phase, Coxcatlan Cave experienced an occupational hiatus for up to 2,500 years, after which time it was reoccupied by people who made and used pottery (Fowler and MacNeish Reference Fowler, MacNeish and MacNeish1972:292).
Materials and Methods
Accelerator Mass Spectrometry
We selected a sample of 17 bones—eight lagomorphs (hares [Lepus sp.] and rabbits [Sylvilagus sp.]) and nine deer (Odocoileus virginianus) specimens—from the collection originally excavated and studied by the TABP (Flannery Reference Flannery and Byers1967). Currently the collection is located at the Laboratorio de Arqueozoología in Mexico City. We selected samples based on size, the appearance of preservation, and the absence of any consolidants, adhesives, or glues on the bone surfaces. Represented in the sample were specimens from habitation zones associated with the Early Ajuereado phase (n = 7; Zones XXVI and XXV), the Late Ajuereado phase (n = 1; Zone XXIV), the El Riego phase (n = 5; Zones XVI–XVI and XIV), the Coxcatlan phase (n = 1; Zone XI), and the Abejas phase (n = 3; Zones IX–VIII). Before destructive analysis, skeletal elements were cleaned with ultrapure water in an ultrasonic bath for 15 minutes and photographed; a series of measurements were then taken on each element following standard point locations (von den Driesch Reference Von den Driesch1976).
Specimens were analyzed at the Keck Carbon Cycle AMS Facility of the Earth System Science Department at the University of California, Irvine. Sample preparation was completed by the first author under the direction of John R. Southon and laboratory assistants. First, the surface of the cortical bone of each skeletal element was ablated with a hand drill, and all trabecular bone was removed to reduce the presence of exogenous contaminants. Bone samples were reduced to small particle sizes (~3 mm), decalcified in 1 N HCl, gelatinized at 60°C in pH 2 water, and ultrafiltered, isolating the fraction with high molecular weight (>30 kDa). All decalcified collagen pseudomorphs appeared clear or white and thus none received an NaOH rinse. After sample preparation, three specimens did not yield sufficient collagen for analysis (ADS-0034, ADS-0117, and ADS-0130). The 14 samples with sufficient collagen were combusted, hydrolyzed, and graphitized for analysis on a compact AMS particle accelerator from National Electrostatics Corporation (NEC 0.5MV 1.5SDH-2 AMS system). An aliquot of each sample was also analyzed with a continuous-flow stable isotope ratio mass spectrometer (Delta-Plus CFIRMS) and an elemental analyzer (Fisons NA-1500). The resulting C:N atomic weight ratios were used to assess collagen quality (Brock et al. Reference Brock, Wood, Higham, Ditchfield, Bayliss and Ramsey2012), with acceptable values expected to range from 2.9 to 3.6 (DeNiro Reference Deniro1985).
Bayesian Model of Preceramic Phases
The 14C ages produced from this study were calibrated using OxCal (version 4.4.2) with the IntCal 20 calibration curve for the Northern Hemisphere (Reimer et al. Reference Reimer, Austin, Bard, Bayliss, Blackwell, Ramsey, Butzin, Cheng, Lawrence Edwards, Friedrich, Grootes, Guilderson, Hajdas, Heaton, Hogg, Hughen, Kromer, Manning, Muscheler, Palmer, Pearson, Van Der Plicht, Reimer, Richards, Marian Scott, Southon, Turney, Wacker, Adolphi, Büntgen, Capano, Fahrni, Fogtmann-Schulz, Friedrich, Köhler, Kudsk, Miyake, Olsen, Reinig, Sakamoto, Sookdeo and Talamo2020). We compiled previously published 14C ages from Coxcatlan Cave and added them to our new ages to develop a Bayesian model of the phase chronology for the Preceramic sequence. This radiocarbon sample includes charcoal specimens analyzed as part of the TABP (Buckley and Willis Reference Buckley and Willis1969; Johnson and MacNeish Reference Johnson, MacNeish and MacNeish1972) and directly dated plant samples from later paleobotanical studies (Kaplan and Lynch Reference Kaplan and Lynch1999; Long et al. Reference Long, Benz, Donahue, Timothy Jull and Toolin1989; Smith Reference Smith2005). However, because zones of Coxcatlan Cave are known to exhibit postdepositional stratigraphic mixing, particularly on the western side of the cave (Smith Reference Smith2005), we excluded multiple specimens for our phase model according to the following considerations.
First, we followed Johnson and MacNeish (Reference Johnson, MacNeish and MacNeish1972:17) and excluded the out-of-sequence ages that were initially rejected by the TABP. The criteria they used to reject radiocarbon ages were (a) those that drastically diverged from samples below or above them and (b) ages that drastically diverged from other ages from the same zone (MacNeish Reference MacNeish1997:667). Regarding the greater amount of rejected ages from the Preceramic levels (see Figure 2), Johnson and MacNeish (Reference Johnson, MacNeish and MacNeish1972:16) explained that “below Zone XVIII, the boundaries between zones were sometimes difficult to identify and were interrupted by rock falls from the roof” (see also Hardy Reference Hardy1996). They also suggested that smaller charcoal samples and not artifacts would have filtered through these more porous sediments, resulting in the apparent downward movement of materials visible in Figure 2.

Figure 2. Scatterplot of calibrated 14C ages and their associated stratigraphic zones. Bone samples are from this study. Charcoal and botanical samples are from previous studies (Johnson and MacNeish Reference Johnson, MacNeish and MacNeish1972; Buckley and Willis Reference Buckley and Willis1969; Kaplan and Lynch Reference Kaplan and Lynch1999; Smith Reference Smith2005). Markers represent the median age and error bars display the 95.4% range. (Color online)
Second, we rejected the radiocarbon ages of plant samples analyzed by Long and colleagues (Reference Long, Benz, Donahue, Timothy Jull and Toolin1989) and Kaplan and Lynch (Reference Kaplan and Lynch1999). Most of the analyzed plant samples exhibited 14C ages that were out of sequence, with the majority exhibiting younger than anticipated ages for their zone designation (Figure 2). MacNeish claimed that their estimated ages had been affected by treatment of the plant samples with the chemical preservative Bedacryl (or “metacreal”) in the 1960s (MacNeish Reference MacNeish1997:668–669; MacNeish and Eubanks Reference MacNeish and Eubanks2000). This suggestion, however, was subsequently refuted, because Bedacryl would have resulted in older than expected ages, not younger ones (Long and Fritz Reference Long and Fritz2001). In a later publication, MacNeish (Reference MacNeish2001b:103–105) claimed that the out-of-sequence plant dates were due to flaws in the AMS laboratory procedures. Nonetheless, we agree with Bruce Smith's (Reference Smith2005) suggestion that the out-of-sequence ages were most likely due to the taphonomic processes of rodent burrowing and human disturbances of previous floors, as well as the downward movement of smaller objects through porous cave sediments. A greater percentage of the new plant specimen ages presented by Smith (Reference Smith2005) exhibit viable age estimates, and we thus only reject clear outliers. For instance, plant sample B-123041 (C. argyrosperma) exhibited a 14C age of 470 ± 40 BP, despite being sampled from Zone XIV, a Preceramic El Riego phase context.
Our review of all of the previously published radiocarbon samples from Coxcatlan Cave (N = 71) left us with 34 samples that we suggest represent the chronological sequence of deposition. Of these samples, 17 originate from Preceramic levels. We combined these previously published Preceramic ages with the bone collagen samples of the present study to construct a Bayesian model of the Preceramic sequence. The full list of accepted and rejected radiocarbon ages is provided in Supplemental Table 1. Within our multiphase model, we followed MacNeish (Reference MacNeish1997) and considered the El Riego, Coxcatlan, and Abejas phases as “contiguous” phases with single boundaries between them. Because of the uncertainties of the ages associated with the Ajuereado phase, we delineated it as a “sequential” phase, separating its dates from the El Riego phase by both an end boundary and a start boundary (Bronk Ramsey Reference Bronk Ramsey2009). After initial modeling, radiocarbon sample I-651 was found to be a phase outlier and was marked as such within the final model (Figure 3). Notably, this radiocarbon sample was also rejected by MacNeish in a later publication (Reference MacNeish1997). The OxCal script of the phase model is presented in Supplemental Text 1.

Figure 3. Bayesian model of the Abejas, Coxcatlan, and El Riego phase zones of Coxcatlan Cave. Bars below distributions represent calibrated 95.4% probability ranges. The outlier date (I-651) is indicated by “?”. Gray rectangles indicate the temporal range of phases defined by median values of the probability ranges for phase boundary transitions. (Color online)
Results and Discussion
Of the 17 skeletal specimens that we analyzed, 14 produced sufficient collagen for accelerator mass spectrometry. All C:N atomic weight ratios from analyzed collagen are within the expected range, implying acceptable preservation. All analyzed specimens exhibited >30 kDa collagen yields greater than 1%, with the exception of UCIAMS-215919 (0.8%). Nevertheless, this specimen exhibited an acceptable C:N ratio of 3.4, and the resulting 14C age was within the range of other El Riego phase samples; we therefore retained it in our analysis. All resulting 14C ages and calibrated date ranges are presented in Table 1. The calibrated AMS ages from the El Riego, Coxcatlan, and Abejas phase zones generally fall within the date ranges originally proposed for these phases by the TABP (Johnson and MacNeish Reference Johnson, MacNeish and MacNeish1972:5; Smith Reference Smith2005:9439) and follow a similar depositional sequence (Figure 2). Notably, unlike the botanical samples, no extreme outliers were observed among the analyzed bone specimens. Within phases, however, 14C ages did exhibit temporal inversions. For instance, sample UCIAMS-219920 from Zone XIV exhibited a radiocarbon age of 8045 ± 30 BP, whereas UCIAMS-223945 from the deeper Zone XVI had a younger radiocarbon age of 7475 ± 20 BP (Figure 3). Similar inversions were also observed within the Ajuereado levels (Figure 4). Nevertheless, all specimens exhibited radiocarbon ages within the expected range of their chronological phases. These results support the general stratigraphy of the Preceramic sequence within Coxcatlan Cave and suggest that faunal bone samples are less likely to have suffered from the taphonomic or contamination processes that affected the botanical and charcoal samples.

Figure 4. Bayesian model dates from the Early Ajuereado phase zones. Bars below distributions represent the 95.4% probability ranges. (Color online)
Table 1. Results of AMS Radiocarbon Dating with Associated Sample Information.

Archaic Period Phases
We used the median values of the phase boundary probability distributions that were produced by the Bayesian phase model to determine the temporal range of each cultural phase of the Mesoamerican Archaic period sequence (El Riego, Coxcatlan, Abejas). The model indicated that the El Riego phase ranged from 9893 to 7838 cal BP (7943–5888 cal BC); the Coxcatlan phase ranged from 7838 to 6375 cal BP (5888–4425 cal BC); and the Abejas phase ranged from 6375 to 4545 cal BP (4425–2595 cal BC; Figure 3). The calibrated probability ranges of 14C ages and boundary transitions between phases are presented in Supplemental Table 2. The largest differences between our modeled chronology and the chronology proposed by MacNeish (Johnson and MacNeish Reference Johnson, MacNeish and MacNeish1972; MacNeish Reference MacNeish1997, Reference MacNeish, Evans and Webster2001a) are a shortening of the Coxcatlan phase and a lengthening of the Abejas phase. MacNeish's (Reference MacNeish, Evans and Webster2001a:708) estimated range for Coxcatlan phase was 7650–5775 cal BP, but here we shorten it by approximately 400 years to 7838–6375 cal BP, with a proportional lengthening of the subsequent Abejas phase.
Early Ajuereado Phase
For the Early Ajuereado phase zones, the modeled age range was from 33,228 to 28,279 cal BP (Supplemental Table 2; Figure 4). These ages are much older than anticipated. We nonetheless accept these results as valid because of (a) the apparent lack of adhesives, consolidants, or glues on the surface of the bones; (b) the acceptable collagen yields; (c) the acceptable C:N ratios; (d) the fact that all six Early Ajuereado samples exhibited similar dates; and (e) the observation that all other specimens exhibited ages similar to what was anticipated. MacNeish and the TABP assumed that the Ajuereado phase was associated with the period of approximately 12,000–9500 cal BP (MacNeish Reference MacNeish and MacNeish1972:496–497), though later publications by MacNeish suggested it could be as old as 32,000 cal BP (MacNeish Reference MacNeish1997:670, Reference MacNeish, Evans and Webster2001a). Our results from AMS analyses on samples from Zones XXVI and XXV indicate that the Early Ajuereado period was more consistent with MacNeish's later estimates.
The Pleistocene ages from the Early Ajuereado zones correspond to a period just before the Last Glacial Maximum (LGM; 26–19 ka), when global ice volume was at its peak and sea levels were at their lowest (Clark et al. Reference Clark, Dyke, Shakun, Carlson, Clark, Wohlfarth, Mitrovica, Hostetler and Marshall McCabe2009; Yokoyama et al. Reference Yokoyama, Lambeck, de Deckker, Johnston and Keith Fifield2000). Clark and colleagues (Reference Clark, Dyke, Shakun, Carlson, Clark, Wohlfarth, Mitrovica, Hostetler and Marshall McCabe2009) classify the period of 33,000–26,500 BP as the Local Last Glacial Maxima, a time when multiple ice sheets first reached their greatest extent just prior to the global LGM. The Local Last Glacial Maxima Pleistocene ages from Coxcatlan Cave Zones XXVI and XXV are congruent with the associated faunal remains from these levels. Preserved skeletal elements of mammals and reptiles are representative of more arid and open environmental conditions than the present-day semitropical thorn-cactus-scrub woodland (Flannery Reference Flannery1966). The TABP excavations encountered bones of horses (Equus sp.), pronghorn (Antilocapra americana), and gopher tortoises (Gopherus cf. berlandieri; Table 2). The pronghorn and gopher tortoises are today associated with the open semiarid deserts of Chihuahua and San Luis Potosí to the north; yet their skeletal remains at Coxcatlan (NISP = 102; MNI = 7; Flannery Reference Flannery and Byers1967:160–161) and from other Pleistocene sites of northern Oaxaca (Cruz et al. Reference Cruz, Arroyo-Cabrales and Viñas-Vallverdú2009; Flannery Reference Flannery, Flannery and Marcus1983) indicate that their ranges extended farther south during the late Pleistocene. No deer remains (Odocoileus virginianus) were found within the Early Ajuereado phase deposits, in contrast to the Late Ajuereado phase deposits (Zones XXIV and XXIII) and all subsequent levels, in which deer become one of the most commonly represented mammals (Flannery Reference Flannery and Byers1967:160–161). Moreover, the proportion of small rodent species in these early zones significantly differs from those of later zones, further indicating a different climatic and ecological regime in the Early versus the Late Ajuereado phases (Flannery Reference Flannery and Byers1967:141–144).
Table 2. Counts of the Number of Identified Specimens (NISP) of Vertebrate Fauna Recovered from the Early Ajuereado Phase of Coxcatlan Cave (Zones XXVIII–XXV).

Source: Data from Flannery Reference Flannery and Byers1967:Tables 15 and 16.
The Pleistocene radiocarbon ages of analyzed faunal bones from Coxcatlan Cave Zones XXVI and XXV (Figure 4) are surprising, given that MacNeish and the TABP suggested that these levels represented a period in which humans occupied the cave. These early ages, however, are older than expected in traditional models for the peopling of the Americas. Describing the archaeological evidence, Johnson and MacNeish (Reference Johnson, MacNeish and MacNeish1972:16) stated, “Zones XXVII, XXVI, and XXV contained a few artifacts, and a succession of small floors made up either of combined humic material and finely comminuted charcoal or vegetable matter clearly indicate human occupation.” Additionally, Flannery (Reference Flannery1966:802, Reference Flannery and Byers1967:158) noted a large amount of jackrabbit foot bones (Lepus sp.), mostly excavated from a single 1 × 1 m square unit within the Early Ajuereado deposits, suggesting they represented the butchered remains of jackrabbits acquired through hunting drives. The presence of bone breakage and evidence of thermal alterations to the bones further suggested that they had been processed by humans.
The Early Ajuereado zones were described as being irregular strata, consisting primarily of fallen rocks from the roof of the cave and wind-blown sands, with each level being capped by a thin charcoal floor (MacNeish Reference MacNeish and Byers1967c:15). The lowest level of Coxcatlan Cave, Zone XXVIII, was a thin and compact layer of rock flakes lying directly over the natural bedrock of the cave (Fowler and MacNeish Reference Fowler, MacNeish and MacNeish1972:227, 234). It contained a total of 199 faunal bones (Table 2). Although no stone tools were listed in the original report (MacNeish et al. Reference MacNeish, Nelken-Terner and Johnson1967), the subsequent description of the excavation and the associated plan-view map of Zone XXVIII (Johnson and MacNeish Reference Johnson, MacNeish and MacNeish1972:235) registered four stone flakes and a fragment of a “crude” blade from this level. Overlaying Zone XXVIII was Zone XXVII, a deposit composed of yellow sand mixed with rock flakes from the walls and roof of the cave. This zone was approximately 1 m thick in the eastern portion of the cave but tapered off and disappeared before reaching the midline. From Zone XXVII, the TABP recovered 46 faunal bones, most of which (n = 33) were leporids (rabbits or hares; Table 2), in addition to two chipped-stone artifacts (Table 3) and nine stone flakes (Johnson and MacNeish Reference Johnson, MacNeish and MacNeish1972:236). The two Early Ajuereado zones from which we obtained radiocarbon ages (XXVI and XXV) were described as representing possible wet-season or spring microband encampments. Zone XXVI was a “whitish-to-yellowish” sediment layer composed of loose, fine flakes of rock with a width of approximately 1 m at its thickest (Fowler and MacNeish Reference Fowler, MacNeish and MacNeish1972:229, 236). In total, 616 individual faunal bone specimens, three chipped-stone artifacts (Table 3), and eight stone flakes were recovered from within this zone. Zone XXV was similarly described as containing loose, yellowish laminated rock deposit, but varying to a reddish color in areas, which Fowler and MacNeish (Reference Fowler, MacNeish and MacNeish1972:237) suggest may have resulted from burning. Other oxidizing processes, however, cannot be ruled out. This zone reached approximately 0.75 m at its thickest (Fowler and MacNeish Reference Fowler, MacNeish and MacNeish1972:229) and contained 391 identifiable faunal bone specimens (Table 2), 10 chipped-stone artifacts, and 44 stone flakes (Johnson and MacNeish Reference Johnson, MacNeish and MacNeish1972:238).
Table 3. Chipped-Stone Artifacts from the Early Ajuereado Phase Zones of Coxcatlan Cave.

Source: Data from MacNeish et al. Reference MacNeish, Nelken-Terner and Johnson1967.
In total, 13 stone artifacts, 2 possible stone artifacts, and 65 chipped-stone flakes were categorized as belonging to the Early Ajuereado zones (Table 3; Johnson and MacNeish Reference Johnson, MacNeish and MacNeish1972:234–238; MacNeish et al. Reference MacNeish, Nelken-Terner and Johnson1967). The majority of the stone tools consisted of minimally worked blades, scrapers, and flakes derived from unprepared cores. In consideration of the antiquity of the new AMS radiocarbon ages, the possibility that these artifacts were products of natural forces and not of human labor must be considered. Nevertheless, the experience of the TABP researchers with chipped-stone technologies of Preceramic North America, as well as the observation that the tools were composed of “flint” (MacNeish et al. Reference MacNeish, Nelken-Terner and Johnson1967:9), implying a material external to the cave, makes it difficult to dismiss them as cultural artifacts. Although most of the identified tools were minimally worked, the TABP documented other chipped-stone artifacts from Early Ajuereado phase zones with evidence of retouching and use wear. These included a bifacial chopper, a “spokeshave-like tool,” and laterally worked “thick flakes” with retouched and utilized edges (Table 3; MacNeish et al. Reference MacNeish, Nelken-Terner and Johnson1967). The TABP report, however, did not describe individual specimens from each stratigraphic zone (MacNeish et al. Reference MacNeish, Nelken-Terner and Johnson1967). Reassessing the original collection will be an essential step in testing the presence of a pre-LGM occupation of Coxcatlan Cave.
The traditional model for the peopling of the Americas contends that the first Americans crossed Beringia and the Ice-Free Corridor around 13,500 cal BP and that these populations utilized Clovis chipped-stone tool technology known for its fluted spear points (e.g., Kelly Reference Kelly2003; Meltzer Reference Meltzer2015). However, growing evidence demonstrates the presence of pre-Clovis people in both North and South America who used less elaborate chipped-stone tool technology (Bourgeon et al. Reference Bourgeon, Burke and Higham2017; Davis et al. Reference Davis, Madsen, Becerra-Valdivia, Higham, Sisson, Skinner, Stueber, Nyers, Keen-Zebert and Neudorf2019; Dillehay et al. Reference Dillehay, Ocampo, Saavedra, Sawakuchi, Vega, Pino, Collins, Cummings, Arregui and Villagrán2015; Erlandson Reference Erlandson, Graf, Ketron and Waters2013; Jenkins et al. Reference Jenkins, Davis, Stafford, Campos, Connolly, Cummings, Hofreiter, Hockett, McDonough, Luthe, Graf, Ketron and Waters2013; Waters et al. Reference Waters, Forman, Jennings, Nordt, Driese, Feinberg, Keene, Halligan, Lindquist and Pierson2011). Within Mexico, researchers have argued for Pleistocene ocupations at several locations (González et al. Reference González, Huddart, Bennett and González-Huesca2006; Irwin-Williams and Martin Reference Irwin-Williams, Martin, Martin and Wright1967; Lorenzo and Mirambell Reference Lorenzo and Mirambell1986a), but because of the early period in which these sites were excavated, there are ambiguities in their dating methods or the documented stratigraphy (Meltzer Reference Meltzer2009:105–107; Sánchez Reference Sánchez2001). Currently, the best—though still contested—evidence for pre-Clovis occupations in Mexico comes from three sites: (1) the Rancho La Amapola site at Cedral, San Luis Potosí; (2) the Santa Isabel Iztapan II mammoth kill site near Mexico City; and (3) Chiquihuite Cave in northern Zacatecas. Research at the Rancho La Amapola site produced radiocarbon ages from charcoal sampled from hearths ranging from approximately 45,000–25,000 calibrated radiocarbon years BP (Mirambell Reference Mirambell, Manzanilla and Luján1994:239). These were found associated with a chipped-stone discoidal scraper and Pleistocene faunal bones, including a broken and utilized horse tibia (Lorenzo and Mirambell Reference Lorenzo, Mirambell and Bryan1986b; Mirambell Reference Mirambell, Manzanilla and Luján1994). The Santa Isabel Iztapan II mammoth kill site contained the remains of a disarticulated mammoth (Mammuthus sp.) skeleton associated with three bifacial stone projectile points: Scottbluff, Lerma, and Angostura points. Cut marks on the bones demonstrated that humans had butchered the carcass (Aveleyra Arroyo de Anda Reference Aveleyra Arroyo de Anda1956). González and colleagues (Reference González, Huddart, Israde-Alcántara, Domínguez-Vázquez, Bischoff and Felstead2015) estimated the mammoth to date to 14,500–10,800 BP based on tephra ages. Finally, chipped-stone artifacts from recent excavations at Chiquihuite Cave site in Zacatecas were associated with optically stimulated luminescence and radiocarbon ages ranging from approximately 33,000 to 31,400 cal BP (Ardelean et al. Reference Ardelean, Becerra-Valdivia, Pedersen, Schwenninger, Oviatt, Macías-Quintero, Arroyo-Cabrales, Sikora, Ocampo-Díaz, Rubio-Cisneros, Watling, De Medeiros, De Oliveira, Barba-Pingarón, Ortiz-Butrón, Blancas-Vázquez, Rivera-González, Solís-Rosales, Rodríguez-Ceja, Gandy, Navarro-Gutiérrez, De La Rosa-Díaz, Huerta-Arellano, Marroquín-Fernández, Martín Martínez-Riojas, López-Jiménez, Higham and Willerslev2020). Although our new radiocarbon ages from the Late Ajuereado zones of Coxcatlan Cave remain only tentatively associated with human activities, they join this list of potential pre-Clovis sites from Mexico.
More studies are needed before the Early Ajuereado phase zones can be considered to be the result of human occupation. At present, the earliest directly dated human remains in Mexico come from an individual discovered from the submerged Hoyo Negro cave in Quintana Roo with a radiocarbon age of 10,976 ± 20 (12,910–11,750 cal BP). This date is approximately 20,000 years younger than the radiocarbon ages from the Early Ajuereado phase deposits at Coxcatlan Cave. It remains possible that the Pleistocene fauna of the Early Ajuereado phase zones at Coxcatlan Cave were already within the rock shelter for thousands of years prior to human arrival and that early human activities disturbed the deposits, mixing human artifacts with older sediments. Assessing directly dated faunal skeletal remains for cut marks or other signs of human manipulation will serve as the most productive avenue for resolving this question.
Late Ajuereado Phase
The Late Ajuereado zones (XXIV and XXIII) are more securely associated with human activity than the Early Ajuereado zones. Late Ajuereado deposits contained the first projectile points, including four (possibly five) Lerma points and one Abasolo point (MacNeish et al. Reference MacNeish, Nelken-Terner and Johnson1967:59). No pronghorn or horse remains were represented in the fauna recovered from the Late Ajuereado phase levels, but deer appeared for the first time. This pattern suggests a considerable lapse of time and environmental succession between the Early and Late Ajuereado phase zones. Additionally, a Lerma point similar to those recovered from the Late Ajuereado Zones XXIV and XXIII was directly associated with the butchered mammoth skeleton at the site of Santa Isabel Iztapan II in the Basin of Mexico, mentioned earlier (Aveleyra Arroyo de Anda Reference Aveleyra Arroyo de Anda1956:24). Based on stratigraphy and tephra analysis, the mammoth kill site has an estimated date range of 14,500–10,800 BP (González et al. Reference González, Huddart, Israde-Alcántara, Domínguez-Vázquez, Bischoff and Felstead2015). The fauna and artifacts then tentatively suggest a Late Pleistocene to Early Holocene range for the Late Ajuereado phases.
The radiocarbon evidence for the temporal period of occupation for the Late Ajuereado zones is ambiguous. The O. virginianus skeletal element from Zone XXIV that we attempted to date did not yield sufficient collagen for AMS. The TABP obtained two radiocarbon ages on charcoal samples (I-460, 7150 ± 200 BP [8367–7619 cal BP] and I-676, 8150 ± 340 BP [10,117–8337 cal BP]; Supplemental Table 1) from Late Ajuereado phase zones XXIV and XXIII, respectively, but they rejected them because they exhibited ages younger than those produced from El Riego phase zones in the levels above (Johnson and MacNeish Reference Johnson, MacNeish and MacNeish1972). However, given that the newly calibrated date range for the charcoal specimen I-676 falls between 10,117 and 8337 cal BP (using the IntCal20 calibration curve), its age can be temporally positioned after the El Riego phase, which according to the Bayesian model presented here occurred sometime between 9893 and 7838 cal BP. This radiocarbon sample is thus still consistent with previous estimates for the age of the Late Ajuereado period. The radiocarbon age produced from the charcoal sample of Zone XXIV (I-460), however, remains out of sequence because of its younger-than-expected date.
Given the observations that the earliest radiocarbon age from the superiorly positioned El Riego phase was 8745 ± 20 (UCIAMS-223944; 9890–9557 cal BP) and the latest radiocarbon age of the inferiorly positioned Early Ajuereado phase was 24,660 ± 140 (UCIAMS-223937; 29,180–28,689 cal BP), we may assume that the occupation of the Late Ajuereado zones occurred at some time between ~29,000 and 9000 cal BP. In the Valley of Oaxaca, approximately 100 km south of the Tehuacan Valley, human activities have been dated to this gap in the Tehuacan Preceramic sequence (Flannery and Spores Reference Flannery, Spores, Flannery and Marcus1983). Cueva Blanca, a cave site in the eastern arm of the Valley of Oaxaca, exhibits a very similar chipped-stone technological complex to that of Coxcatlan Cave (Flannery and Hole Reference Flannery, Hole, Flannery and Hole2019). There, Flannery (Reference Flannery, Flannery and Hole2019:53; Flannery and Spores Reference Flannery, Spores, Flannery and Marcus1983:22) reports three conventional radiocarbon ages on charcoal samples excavated from a cooking pit associated with Zone E (Feature 15)—10,910 ± 80 BP (SI-511), 10,730 ± 220 BP (SI-511R), and 11,000 ± 400 BP (M-2094)—which together calibrate to a 95.4% probability range of 13,794–12,665 cal BP. These ages are similar to the tephra dates for the Santa Isabel II mammoth (14,500–10,800 BP) with the associated Lerma point, and we suggest the Late Ajuereado occupations at Coxcatlan Cave may have been similar in age. If correct, then the Late Ajuereado zones would date to approximately 13,500–9900 cal BP, and a gap of approximately 7,500 years (~28,000–13,500 cal BP) would remain in the occupation of the cave between the Early and Late Ajuereado phases. This gap roughly corresponds to the period of the LGM. The timing of the Late Ajuereado phase, however, must remain uncertain until additional radiocarbon ages are produced from these zones.
Conclusions
This article presented 14 new AMS radiocarbon ages from faunal remains excavated from the Preceramic levels of Coxcatlan Cave in the Tehuacan Valley. Our results refine the timing of the Archaic period chronological phases (El Riego, Coxcatlan, and Abejas) by using radiocarbon data to construct a Bayesian multiphase model. Although the Archaic period chronology presented here is generally similar to that originally proposed by the TABP (Johnson and MacNeish Reference Johnson, MacNeish and MacNeish1972), the AMS radiocarbon ages from the Early Ajuereado zones date to a period just before the Last Glacial Maximum (33,448–28,279 cal BP) and are similar to those produced from the early occupation of Chiquihuite Cave in north-central Mexico (Ardelean et al. Reference Ardelean, Becerra-Valdivia, Pedersen, Schwenninger, Oviatt, Macías-Quintero, Arroyo-Cabrales, Sikora, Ocampo-Díaz, Rubio-Cisneros, Watling, De Medeiros, De Oliveira, Barba-Pingarón, Ortiz-Butrón, Blancas-Vázquez, Rivera-González, Solís-Rosales, Rodríguez-Ceja, Gandy, Navarro-Gutiérrez, De La Rosa-Díaz, Huerta-Arellano, Marroquín-Fernández, Martín Martínez-Riojas, López-Jiménez, Higham and Willerslev2020). Because many of the stone tools associated with these early levels are only minimally worked and the antiquity of the ages places these stratigraphic layers many thousands of years before traditional hypotheses posit the arrival of humans in North America, the identity of these objects as tools remains questionable. Nevertheless, the observations that the faunal remains appear to have been processed by humans, that the stone tools are made from flint, and that several stone tools exhibit retouching provide support for the notion of a pre-LGM occupation of Coxcatlan Cave during the Early Ajuereado phase. The radiocarbon ages produced from this study mandate a careful reconsideration of the materials recovered from these zones, including a close inspection of the chipped-stone tools and an analysis of faunal material to document breakage patterns, cut marks, and thermal alterations. The results of this study and those of future reanalyses have important implications for our understanding of several important issues in the archaeology of the New World, including the peopling of the Americas, the timing and causes of the megafaunal extinctions, and the origins of agriculture.
Acknowledgments
This research was made possible by the approval of Dr. Carlos Javier González González, director of the Consejo de Arqueologia (Oficio 401.1S.3-2018/129). We thank Rocio Hernández for assistance with laboratory preparation of the samples and Samantha Negrete for help with the initial sample collection and inventory. Corinna Most, Joyce Marcus, Kent Flannery, and Geoffrey Braswell provided helpful comments and feedback on a previous draft. Matthew G. Hill also provided comments and prepared the map in Figure 1. We thank the editors and three anonymous reviewers for their comments and critiques, which greatly improved the quality of this article. The research was carried out while Andrew Somerville was supported by a UCMEXUS-CONACYT Postdoctoral Research Fellowship and was funded by grants from the National Science Foundation (#1901618) and the Wenner Gren Foundation (Gr. 9655). Any opinions, findings, and conclusions or recommendations expressed in this material are those of the authors and do not necessarily reflect the views of these institutions.
Data Availability Statement
All original data produced as part of this study are presented in Table 1.
Supplemental Materials
To view supplemental material for this article, please visit https://doi.org/10.1017/laq.2021.26.
Supplemental Text 1. OxCal radiocarbon model script.
Supplemental Table 1. All Uncalibrated And Calibrated Radiocarbon Ages from Coxcatlan Cave.
Supplemental Table 2. Calibrated and Modeled Radiocarbon Ages for the Coxcatlan Preceramic Sequence.