Introduction
Epiphytes are an important part of many tropical forests. Growing on other plants, mainly trees, without tapping into the water and nutrient flow of the host they are not parasites (Zotz Reference Zotz2016). However, a large number of epiphytes in a tree may lead to structural failure of branches (Strong Reference Strong1977). Substrate stability is obviously important for structurally dependent epiphytes and structural failure accounts for a large proportion of deaths (Hietz Reference Hietz1997, Hietz et al. Reference Hietz, Ausserer and Schindler2002, Sarmento Cabral et al. Reference Sarmento Cabral, Petter, Mendieta-Leiva, Wagner, Zotz and Kreft2015, Schmit-Neuerburg Reference Schmit-Neuerburg2002, Zotz Reference Zotz1998, Zotz et al. Reference Zotz, Laube and Schmidt2005). Under extreme conditions, that is, hurricanes (category 1 with wind speeds from 33 m s−1), forest canopies suffer severe damage (e.g., Hirsh & Marler Reference Hirsh and Marler2002, Lugo et al. Reference Lugo, Applefield, Pool and McDonald1983, Vozmishcheva et al. Reference Vozmishcheva, Bondarchuk, Gromyko, Kislov, Pimenova, Salo and Korznikov2019). The mechanical effect of wind on epiphytes themselves is hardly studied (but see Tay et al. Reference Tay, Zotz, Puczylowski and Einzmann2021), although the possibility of epiphytes being dislodged by wind is repeatedly mentioned in the literature, albeit without quantitative data (e.g., Francisco-Ventura et al. Reference Francisco-Ventura, Menchaca-Garcia, Toledo-Aceves and Kromer2018, Lowman & Linneroth Reference Lowman and Linneroth1995, Pett-Ridge & Silver Reference Pett-Ridge and Silver2002, Rodríguez-Robles et al. Reference Rodríguez-Robles, Ackerman and Meléndez1990). Typically, epiphytes growing in a closed forest do not experience very strong wind, although wind speed within a forest is clearly site dependent. The wind speed measured within tropical forests in the studies of Hales (Reference Hales1949, lowland Panama), Baynton et al. (Reference Baynton, Biggs, Hamilton, Sherr and Worth1965, lowland Colombia), Aoki et al. (Reference Aoki, Yabuki and Koyama1975, lowland Malaysia), and Lawton (Reference Lawton1982, lower montane forest Costa Rica) ranged between <1 to ca. 50 % of the mean wind speed measured above the forest, where wind speeds reached 3.5–11 m s−1. In more open forests, the wind speed gradient is less steep as wind enters easier into lower strata as compared to forests with denser vegetation. A mountain ridge forest studied by Lawton (Reference Lawton1982) was very exposed and experienced ca. two to three times higher wind speeds than the mentioned lowland forests. Wind flow in and directly above forests is highly turbulent; therefore, forces acting on the plants in the forest are not simply a function of the mean wind speed. Forces exerted by gusts, that is, a sudden and brief (3–20 s) increase in wind speed (American Meteorological Society 2012), can be 10 times greater than the forces from mean wind speed measured (Moore et al. Reference Moore, Gardiner, Sellier, Geitmann and Gril2018). At least in the topmost layers of the canopy violent wind gusts can be expected. Within tree crowns, turbulent wind may thus play a detrimental role that could possibly cause substrate or attachment failure of epiphytes. Since wind conditions in the upper tree crows are arguably most critical to epiphytes, we will focus on this aspect.
Due to increased human pressure in countries in the tropics, the habitat still available to epiphytes is increasingly of a secondary and fragmented type, and the wind dynamics change in fragmented landscapes. The higher relative wind speeds found within more open forests (Hales Reference Hales1949, Latimer Reference Latimer1950) already hint at this aspect. Epiphytes on isolated trees in, for example, pastures have hardly any protection from wind due to the lack of surrounding vegetation and may also suffer the most in a storm (Lowman & Linneroth Reference Lowman and Linneroth1995). In still forested areas, ongoing fragmentation creates more abrupt edges resulting in wind entering the forest more easily (Laurance & Curran Reference Laurance and Curran2008) leading to an increase in tree mortality and wood-debris production close to the fragment edge (Laurance Reference Laurance, Laurance and Peres2006). Although mean wind loads are larger at the forest edges, the ratio of maximum load to mean load on a tree increases with distance from the edge (Moore et al. Reference Moore, Gardiner, Sellier, Geitmann and Gril2018). Thus, trees and consequently epiphytes, inside the forest, may experience only low mean wind speeds in general but have to cope with comparatively stronger gust loads. With this general background information, we investigated what actually happens to epiphytes in a strong wind flow. The influence of wind on plants has been investigated in a range of plants from small terrestrial seedlings (e.g., Ennos Reference Ennos1991) to large trees (e.g., Hale et al. Reference Hale, Gardiner, Wellpott, Nicoll and Achim2012), but until recently never in epiphytes (but see Tay et al. Reference Tay, Zotz, Puczylowski and Einzmann2021). Wind can cause considerable drag on the overall plant, leading to temporal form changes by reconfiguration of the leaves (Harder et al. Reference Harder, Speck, Hurd and Speck2004, Vogel Reference Vogel1989). Reconfiguration is not always possible, however, and leaves can be damaged due to fluttering in the wind (Skutch Reference Skutch1930, Vogel Reference Vogel1989). Small epiphytes and those that grow close to their substrate, for example, creeping ferns, expose very little frontal area to wind and should experience little force (Tremblay Reference Tremblay2008). In contrast, erect growing bromeliads, with their densely clustered leaves, expose a large frontal area to wind and are limited in their ability to reduce the exposed area, at least around their base (Tay et al. Reference Tay, Zotz, Puczylowski and Einzmann2021). Therefore, in this study we focused on how epiphytic tank bromeliads are affected by strong wind. We expected to observe that they are considerably impacted by wind, that is, showing flapping leaves, bent foliage, and similar distortions.
Material and methods
To observe how tank bromeliads behave during the onset of wind in their natural habitat, we used a custom-built camera set-up that would start taking a minimum 15-second video triggered by a pre-set wind speed. This triggering speed was adjusted so that neither too many nor too few videos would be initiated; therefore, it differed between trees. The set-up used a cup-anemometer on top of an acrylic glass box that held an action camera connected to a rechargeable battery and an electronic unit controlled with an Arduino board (Figure 1). Whenever possible this set-up was installed on the same branches that hosted the focal bromeliads so that the movement of the substrate would be the same for both camera and epiphyte. Next to the camera set-up, within max. 1 m distance, an anemometer (Davis® Wind Speed and Direction Smart Sensor, Bourne – MA, USA) with logger (Onset HOBO Microstation, Bourne – MA, USA) was installed and it recorded the wind speed in the tree close to the observed epiphytes. Wind speed was recorded as mean over 1 minute and as the highest 3-second interval during this logging interval.

Figure 1. Custom-built camera set-up with an anemometer that triggers the camera in the acrylic glass housing to take a short video sequence when the trigger wind speed is reached. A second anemometer with a data logger was installed within 1 m of the camera set-up.
The cameras were set up in the Fortuna Forest Reserve (Dalling & Turner Reference Dalling and Turner2021), Panama, an area that experiences frequent and strong wind impact. The Fortuna Forest Reserve covers 19,500 ha with an elevation range of 700–2,000 m. It encompasses perhumid to superhumid aseasonal and seasonal forests over a complex geology. The present study was conducted at the elevation of ca. 1,050–1,150 m a.s.l. with mean annual rainfall of ca. 5,000–6,000 mm and mean temperature of 19–20 °C (Prada et al. Reference Prada, Morris, Andersen, Turner, Caballero and Dalling2017). In 2019 and 2020, wind was strongest in the months of January through March with mean wind speeds >2.8 m s−1, whereas in the rest of the year the mean was <2.5 m s−1 (Paton Reference Paton2021). The cameras were mounted for one week from 26 March to 01 April 2019. Eight trees were chosen. Three of them grew in a clearing (N8° 43ʼ 20.2" W82° 14ʼ 14.1") between the forest edge and the road on a hillside, two grew in the forest (N8° 45ʼ 26.7" W82° 13ʼ 01.8"), and three grew at the borders of very small patches of forest or bushy area along the road close to a mountain pass (N8° 41ʼ 31.1" W82° 13ʼ 45.8") that experiences strong wind almost constantly (Figure 2). One of the two forest trees, growing further up the slope, had a more exposed crown emerging from the forest canopy, whereas the other one had no emergent crown and grew further down the slope. In all trees, except two bush-like trees along the road, the cameras and anemometer were installed in what would correspond to Johansson zone 3, although none of the trees closely resembled Johansson’s idealised tree form (Johansson Reference Johansson1974). The aim was not to systematically document differences between habitats but to observe epiphytes in relatively strong wind in diverse situations in a montane forest. Three cameras were available at the beginning, so that observations were possible in a maximum of three trees at any time. Due to logistical limitations in regard to climbing and availability of measuring devices, the periods in which data could be collected in the different trees varied considerably from ca. 8 hours to almost 2 days (Figure 3). The observed bromeliads were tank-forming species and ranged in their growth habit from a rather compact form, with erect leaves that did not tilt at their tips, to others with fewer but longer and tilting leaves. It was not relevant for this study to identify individual species, since our focus lies on the mechanical interaction for which only the wind obstructing body on the branch is relevant, not the species as such.
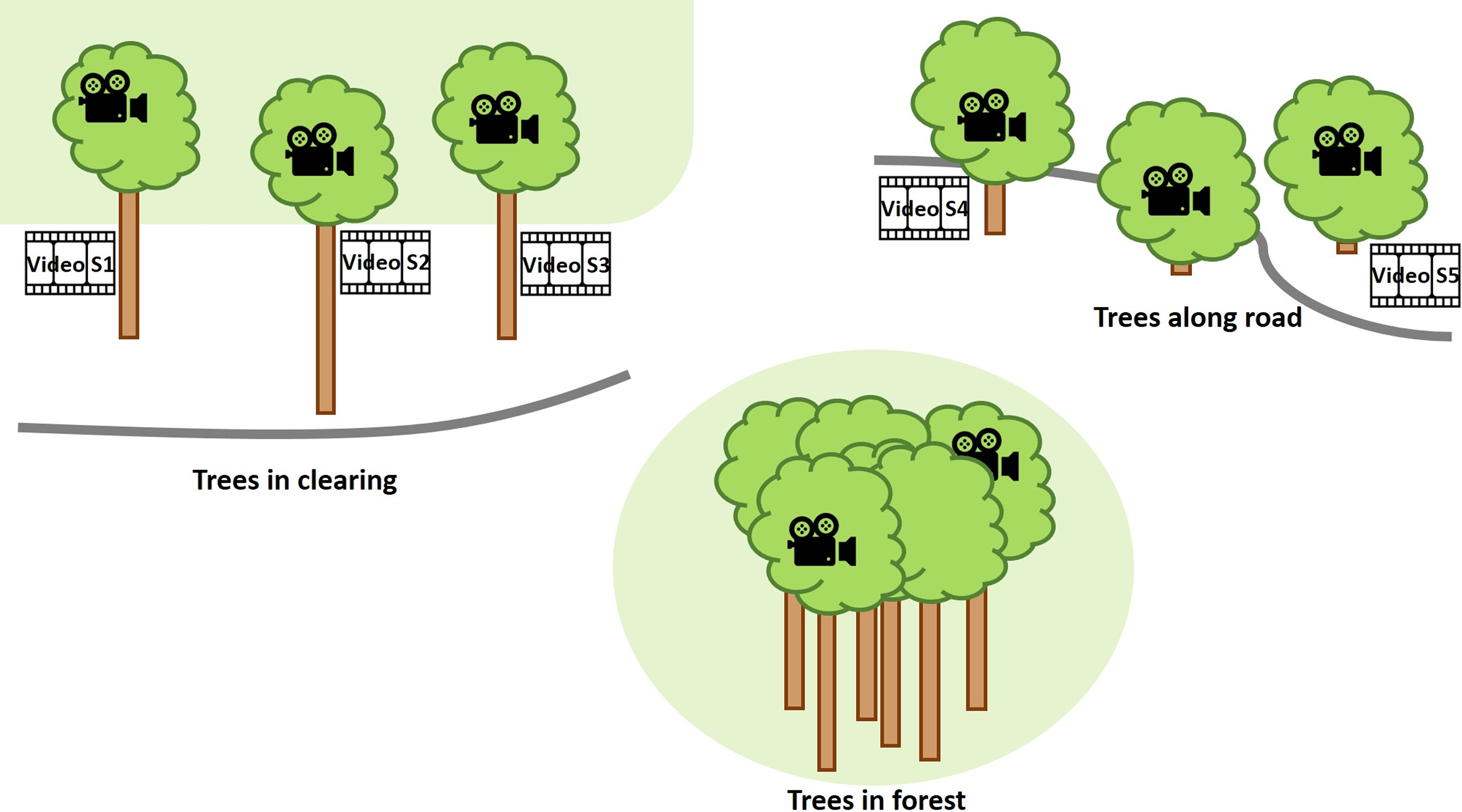
Figure 2. Schematic overview of study sites and trees where videos provided in the supplementary material were recorded.

Figure 3. Wind speed in trees in a clearing between the road and the forest, trees in a forest, and trees along a road close to a mountain pass. Gusts represent the 3-second maximum speed measured over a time interval of 1 minute, and mean wind speed are 1-minute averages. Numbers under boxplots indicate n per boxplot, that is, total time of measurement in minutes. Boxes depict the inter-quartile range (IQR) between the first and third quartiles. The lower and upper whiskers extend from the hinges of the first and third quartiles to the smallest and largest value no further than 1.5*IQR from the hinge, respectively. Data points beyond the whiskers are plotted individually.
Results and discussion
The mean wind speeds measured ranged from 2.0 ± 0.8 to 3.6 ± 1.1 m s−1 (data are means ± standard deviations, n is given in Figure 3) in the trees in the clearing, 0.1 ± 0.3 to 0.3 ± 0.4 m s−1 in the forest trees, and 3.4 ± 0.5 to 5.0 ± 0.7 m s−1 in the trees along the road at the mountain pass. Maximum gust velocities ranged from 12.0 to 13.0 m s−1 in the trees in the clearing, 3.7 to 5.7 m s−1 in the forest trees, and 9.0 to 11.4 m s−1 in the trees along the road at the mountain pass (Figure 3). We compared the wind speed measured in the trees growing in the clearing to the wind speed measured by the anemometer installed in this clearing by the Physical Monitoring Program of the Smithsonian Tropical Research Institute (Paton Reference Paton2021). The average and maximum wind speeds in the crown of tree A during the three days of measurements were very similar to the daily averages and maximums measured in the open clearing (Paton Reference Paton2021). Naturally, the difference depended greatly on the openness of the tree crown. In tree A, in which the set-up was installed in a very exposed position, the difference to the mean wind speed in the clearing was only 0.1 m s−1, whereas in the other two trees differences ranged from 0.7 to 1.7 m s−1.
The crown of tall trees tends to sway in the wind. The relatively slender trees in the study area were very flexible, and the swaying was pronounced. However, the bromeliads themselves growing on these slender hosts were moved very little by the wind (Video S1). The strongest movement we observed was limited vibration of the leaf tips or flapping of a damaged outer leaf (Video S2 and S3). We focused on bromeliads because of their compact habit that exposes a large area to the wind. Long and thin stalks of, for example, Orchidaceae or slender branches of Ericaceae tended to flap a lot more in the wind (Video S1). In smaller-statured stout trees, movement of the main branches was much more limited. Bromeliads growing in these trees showed some flapping of the leaves if these were long and broad. Bromeliads with a compact structure did not show any movement (Video S4). In small-statured slender trees and bush-like trees, the bromeliads themselves moved again very little; however, their substrate moved a lot in the wind (Video S5).
In general, a rigid object that is exposed to wind flow experiences a considerable force on its body that increases quadratically with increasing wind speed (Reynolds numbers from 1 × 103 – 3 × 105; Schlichting & Gersten Reference Schlichting and Gersten2017). But plants are typically able to bend when exposed to wind, thus reducing the total frontal area exposed to wind which in turn decreases the drag force acting on them (de Langre et al. Reference de Langre, Gutierrez and Cossé2012, Harder et al. Reference Harder, Stevens, Speck, Hurd, Herrel, Speck and Rowe2006, Vogel Reference Vogel1984). The videos stress the complexity of the epiphyte–host system by showing that apparently a lot of the force exerted on the epiphytes by wind is mitigated by the movement of the substrate, that is, swaying of the branches or tree crowns. In fact, looking at the entire body of an epiphyte, it seemed that the relatively stiff body of the bromeliads was much less disturbed by wind than that of the more flexible, long-stalked Orchidaceae or Ericaceae that flapped considerably in the wind. However, from our observations we cannot tell if this flapping caused damage to the stalks, but at least no obvious damage was sustained by the movement. Wind tunnel experiments with long- and broadleaved bromeliads showed that excessive flapping of the leaves at high wind speeds (30–50 m s−1, unpubl. data) leads to tearing and consequently considerable damage. Such high wind speeds were not observed in the present study, and only minor damage was observed in a study that tested bromeliads in a wind tunnel with wind speeds up to 22 m s−1 (Tay et al. Reference Tay, Zotz, Puczylowski and Einzmann2021). The maximum wind speed observed during the week of observations was 13 m s−1 in the tree crowns of the clearing. The maximum wind speed measured in the clearing since the start of the wind data collection in 2019 was ca. 19 m s−1 (Paton Reference Paton2021). Therefore, it seems unlikely that wind would damage, let alone detach, epiphytes under normal circumstances even in the exposed sites in Fortuna. In fact, our observations imply that it is highly unlikely that wind alone could dislodge epiphytes, at least at the wind speeds measured here.
During a severe storm like a hurricane, the system is obviously exposed to an entirely different level of forces. It is unclear, however, if the bottleneck is the attachment failure of the epiphytes or substrate failure. Therefore, the implication that epiphytes are blown off by wind (Lowman & Linneroth Reference Lowman and Linneroth1995, Matelson et al. Reference Matelson, Nadkarni and Longino1993, Rodríguez-Robles et al. Reference Rodríguez-Robles, Ackerman and Meléndez1990) may be premature. One also has to consider that the fall of vascular epiphytes is most likely not determined by a single factor, but by the interaction of several, such as wind, precipitation, unstable substrate, arboreal animals, or even the weight of the epiphyte itself (e.g., Francisco-Ventura et al. Reference Francisco-Ventura, Menchaca-Garcia, Toledo-Aceves and Kromer2018, Hietz Reference Hietz1997, Sarmento Cabral et al. Reference Sarmento Cabral, Petter, Mendieta-Leiva, Wagner, Zotz and Kreft2015).
The force acting on a body in a flow environment depends on the frontal area, among other variables. When epiphytes grow on a tree in large numbers, they will increase the overall frontal area that this tree exposes to the wind. Additionally, they increase the load of the branch hosting them, especially when epiphytes are saturated with water after rainfalls. In a study of the role of epiphytes in rainfall interception from the same study region, the water-holding capacity of the epiphytic matter was estimated to be >25,000 L ha−1 (Gómez González et al. Reference Gómez González, Zotz, Bader, Dalling and Turner2021). Looking at the whole epiphyte–host system, how much impact does the increased frontal area and epiphyte load have on the swaying of the trees? The movement of the crown of tall trees is probably mainly initiated by the collective area the leaves expose to the wind (Duryea et al. Reference Duryea, Kampf and Littell2007, Nilsson et al. Reference Nilsson, Stjernquist, Bärring, Schlyter, Jönsson and Samuelsson2004). Whether epiphytes significantly increase the exposed surface in comparison to the area of tree foliage remains unclear. On average, vascular epiphyte biomass is equivalent to about 10% of the green biomass of the co-occurring trees in montane forests (Zotz Reference Zotz2016). If most of these 10% would simultaneously increase the frontal area of their hosts, epiphytes could have a considerable impact on host tree dynamics in wind flow. However, this number is very vague, and there are cases where the green biomass of the epiphytes even exceeds that of the tree foliage (Díaz et al. Reference Díaz, Sieving, Peña-Foxon, Larraín and Armesto2010). Therefore, it is also highly speculative how much of that biomass contributes significantly to the increase of the exposed surface of the host and how much effect the additional weight has. Most likely, the area of tree foliage has such a large impact because it is found in the outermost crown (Video S3) where the exposed area will cause the greatest torque. The bulk of epiphytes, in comparison, grows on large branches and those that grow close to the trunk and in the inner crown may have comparatively little impact on the torque acting on the tree. Extreme swaying may cause some damage to the tree, but gentle swaying as a regular stimulus should already cause some adjustment of the wood body (Niez et al. Reference Niez, Dlouha, Gril, Ruelle, Toussaint, Moulia and Badel2020, Telewski & Pruyn Reference Telewski and Pruyn1998). In contrast, another recent study found that extreme wind events had a greater impact on thigmomorphogenic response in European beech than did daily wind speed peaks (Bonnesoeur et al. Reference Bonnesoeur, Constant, Moulia and Fournier2016). If epiphytes increase the drag force acting on their host during a storm considerably, this could be detrimental to their host and consequently also to them. Several authors have used the term ‘structural parasite’ for the bromeliad species Tillandsia recurvata growing on different hosts (Flores-Palacios Reference Flores-Palacios2016, Montaña et al. Reference Montaña, Dirzo and Flores1997, Perez-Noyola et al. Reference Perez-Noyola, Flores, Yanez-Espinosa, Jurado, Gonzalez-Salvatierra and Badano2020). In these studies, the potential negative effect was caused by the presence of the bromeliad alone, without any further influence of wind. In our scenario, the negative effect could be due to the general presence of many epiphytes on a host in combination with the external wind factor. However, to our knowledge, there are no data on any of the mechanical aspects, for example, how much of the increase in frontal area of the host is caused by epiphytes, how this affects the short- or long-term stability of the host, or how host stability is affected by the additional weight in a static or dynamic situation.
Conclusion
In conclusion, stiff-bodied epiphytes such as bromeliads seem to receive less direct force through wind when growing on a relatively flexible substrate as compared to growing on a fixed substrate. This makes it less likely for them to be blown of individually during strong wind events and it implies that wind alone is unlikely to dislodge them. This is also supported by the study of Sarmento Cabral et al. (Reference Sarmento Cabral, Petter, Mendieta-Leiva, Wagner, Zotz and Kreft2015), which showed that the majority of epiphytes on the floor were found attached to branches and twigs. How much epiphytes contribute or enhance the swaying of their host trees leading to possible branch breakage during extreme gusts remains an open question. If epiphytes increase the likelihood that their host trees are damaged during wind events substantially, another facet would be added to the nature of the interaction described as ‘structural parasitism’. To identify if epiphyte loads contribute to swaying and breakage of the host tree during high winds, a manipulation experiment might be necessary, in which one strips trees of their load and adds loads to other trees. Another approach could be to model flow dynamics using a modelled empty tree (Hackenberg et al. Reference Hackenberg, Morhart, Sheppard, Spiecker and Disney2014, Hackenberg et al. Reference Hackenberg, Spiecker, Calders, Disney and Raumonen2015, Raumonen et al. Reference Raumonen, Kaasalainen, Åkerblom, Kaasalainen, Kaartinen, Vastaranta, Holopainen, Disney and Lewis2013) and then systematically increase epiphyte load.
Supplementary material
To view supplementary material for this article, please visit https://doi.org/10.1017/S0266467422000037
Acknowledgements
We thank Calixto Rodríguez for his untiring assistance during the fieldwork and translation of the abstract into Spanish and Thomas Madena and Janek Willms, Carl von Ossietzky University Oldenburg, for the construction of the ‘wind-cameras’. We also acknowledge the assistance of STRI personnel, Enel Green Power Panamá and the Republic of Panamá for making their natural resources available for research (Research Permit No. SE/P-1-19).
Financial support
This work was supported by the DFG (grant no. EI 1092/1-1).
Competing interests declaration
The corresponding author confirms on behalf of all authors that there have been no involvements that might raise the question of bias in the work reported or in the conclusions, implications, or opinions stated.
Authors’ contribution statement
Conceptualisation: (Helena Einzmann, Gerhard Zotz); data curation, methodology, formal analysis, and visualisation: (Helena Einzmann); investigation: (Helena Einzmann, Jessica Tay); writing – original draft preparation: (Helena Einzmann); writing – review and editing: (Helena Einzmann, Gerhard Zotz, Jessica Tay); funding acquisition: (Helena Einzmann); supervision: (Gerhard Zotz).