Introduction
By strict definition, Endocrine Disrupting Chemicals (EDCs) are defined as (1) ‘exogenous substances that cause adverse health effects in an intact organism, or its progeny, consequent to changes in endocrine function’ (EU, 1997), or as (2) ‘exogenous agents that interfere with the production, release, transport, metabolism, binding and action or elimination of the natural hormones in the body responsible for the maintenance of homeostasis and regulation of developmental processes’ (US EPA, Reference Tyler and Routledge1997). Some EDCs can act directly on receptors, either as hormone mimics (agonists) or blockers (antagonists); others may act indirectly by acting on molecules and pathways that control delivery or action on its target tissue. Either way, sensitivity is often highest during a ‘critical window’ in early developmental stages. In laboratory-exposed mammals, effects on developing gonadal tissue include cryptorchidism (failure of testes to descend from the abdomen into the scrotum), hypospadias (impaired foetal development of the penis and urethral opening), altered testis pathology and low hormone/sperm count in males; ovarian pathologies, dysfunction and lowered fertility are analogous defects in females (EEA, 2012). There is reason to hypothesize that some of these effects could also be manifested in invertebrates living in contaminated environments.
The most commonly considered EDCs in higher organisms are natural hormones such as oestrogens (e.g. 17β-oestradiol (E2), oestrone and progesterone) and testosterone (T) – all associated with domestic sewage. Synchronization of oestrous cycles in domestic animals (e.g. to ensure calving at the most appropriate time) may involve treatment with 17β-oestradiol, progesterone or synthetics such as oestradiol benzoate which can reach the environment in excreta, resulting in disruptive effects in wildlife. In addition there are various hormone mimics present in domestic, industrial, shipping and agricultural wastes. These latter xeno-oestrogens and androgens include the contraceptive pill component 17α-ethinyloestradiol (EE2), diethylstilbesterol (DES), phyto-oestrogens, bisphenol A (BPA, a synthetic oestrogen mimic previously used widely in food packaging), phthalates and other plastic additives, non-ionic surfactants such as the alkylphenols nonylphenol (NP) and octylphenol (OP), pesticides such as DDT, herbicides (e.g. atrazine, glyphosate), together with active ingredients in pharmaceuticals and personal care products. Various metals including arsenic, cadmium, lead, mercury and zinc (Lavicoli et al., Reference Langston, Pope, Davey, Langston, O'Hara, Gibbs and Pascoe2009) and a host of industrial chemicals, past and present, including polychlorinated biphenyls (PCBs), polybrominated flame retardants and the antifoulant TBT can be added to this long list. Indeed, there are a growing number of chemicals (perhaps of the order of 1000 according to Schug et al., Reference Schlumpf, Lichtensteiger, Jarry, Seidlova-Wuttke, Wuttke, Oetken, Bachmann, Schulte-Oehlmann and Oehlmann2016) and environmental factors, which may cause perturbations in the development of marine invertebrates and should be considered alongside established EDCs (capable of interfering with hormone receptors) given that they may influence ‘fitness’ of populations in similar ways (i.e. ‘chemicals toxic to reproduction’ in EU REACH parlance – see EEA, 2012), despite alternative modes of action. Examples which reflect key research developments in relation to marine invertebrates (and, to a lesser extent, fish), and future research and testing needs to help monitor and maintain healthy environments, are included in this review.
Screening assays and threshold concentrations of EDCs
Threshold and No Observable Effects Concentrations (NOEC) have been derived for some of the more established EDCs (Table 1) and have been used effectively to monitor and manage environmental risk – notably in the case of TBT where a marine Environmental Quality Standard (EQS) of 2 ng l−1 was set based on a combination of effects levels in species such as oysters Crassostrea gigas (Thunberg, 1793) and dogwhelks Nucella lapillus (Linnaeus, 1758) and, pragmatically, on what could actually be measured in the environment. However, for many EDCs no such relevant thresholds exist, and may be dependent on exposure timing/duration (Oehlmann et al., Reference Oehlmann, Schulte-Oehlmann, Tillmann and Markert2006, Reference Oehlmann, Schulte-Oehlmann, Bachmann, Oetken, Lutz, Kloas and Ternes2007; Kortenkamp & Faust, Reference Kortenkamp and Faust2018), the nature of the receiving environment and the presence of synergetic or antagonistic agents. Furthermore, dose-response relationships may not follow linear form: activity may become relatively reduced at high (toxic) doses compared with highly sensitive chronic response thresholds. For example, embryo production in aquatic snails Potamopyrgus antipodarum (Gray, 1843) is increased by the oestrogenic sunblock component 3-benzylidene camphor at low, but not high concentrations (Table 1; Schlumpf et al., Reference Schlenk2004). Simple extrapolations are clearly not justified in such circumstances.
Table 1. Effective concentrations of some known ED-like compounds to fish and aquatic invertebrates
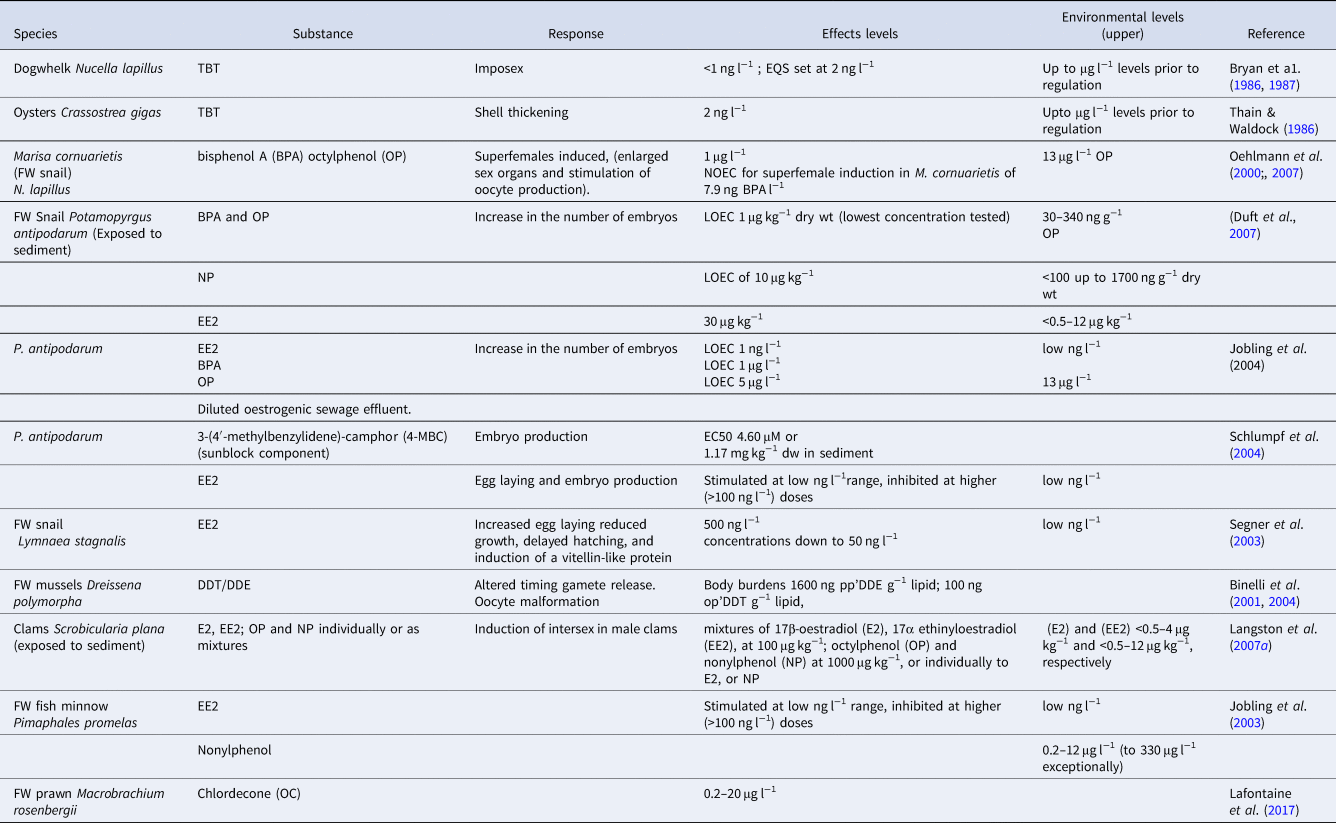
Faced with the potential for additive/interactive effects in environmental mixtures, chemical thresholds may be of questionable value as a regulatory tool in isolation without accompanying biological monitoring. In such circumstances procedures such as TIE (Toxicity, Identification and Evaluation) which combine chemical fractionation with bioassays to identify biologically active components, may (sometimes) be effective in assessing relevant EDCs and their thresholds in extracts of environmental samples (Schlenk, Reference Sanchez, Sremski, Piccini, Palluel, Maillot-Maréchal, Betoulle, Jaffal, Aït-Aïssa, Brion, Thybaud, Hinfray and Porcher2008). Methods may involve in vivo or, more frequently, in vitro bioassays including ER competitive ligand binding assays (Schwartz & Skafar, Reference Schultz, Minarik, Martinovic-Weigelt, Curran, Bartell and Schoenfuss1993) and oestrogen/androgen receptor-based cell-lines such as the yeast oestrogen/androgen screens (YES/YAS) described by Routledge & Sumpter (Reference Reijnders1996). In these, human ER/AR genes are coupled to a reporter gene initiating a colour change upon activation (in the analogous ER-CALUX assay described by Legler et al. (Reference Lee, Lee, Kang, Park, Lee, Jeon and Jual1999), reporter gene activation triggers a response in luminescence). An example of both types of response showing a relationship between oestrogenic activity in sediment extracts measured in vitro by the YES assay and in the in vivo response intersex (oocyte development in testis) in clams Scrobicularia plana (da Costa, 1788) from UK estuarine sites, is described later in this review.
Reporter-gene assays in yeast, fish or mammalian cells, along with competitive binding assays with the oestrogen and androgen receptor have been applied widely to screen for androgenic and oestrogenic stimuli. Establishing such relationships may add to the weight of evidence where ED-like effects are suspected. Nevertheless, although sensitive to changes in endocrine status from a range of potential EDC these in vitro assays are not proof of causality in nature and, if used in isolation, may be of limited value in predicting in vivo effects including altered sexual characteristics, impaired development and reproductive function, and population-level consequences.
Endocrine disruption and reproductive effects in aquatic biota
Alongside suspicions of serious defects in humans (e.g. testicular cancers, low sperm counts, hypospadias and cryptorchidism), and the realization that ED-like chemicals impact a range of charismatic wildlife including mink, seabirds, alligators, turtles, frogs and toads (EEA, 2012), a variety of (mainly feminizing) effects, including vitellogenesis and intersex (ovotestis – occurrence of eggs in testis), have been reported to be widespread in many aquatic organisms, both freshwater and marine (Sumpter & Jobling, Reference Sumpter1995; Jobling et al., Reference Jobling, Nolan, Tyler, Brighty and Sumpter2003; Matthiessen et al., Reference Matthiessen and Gibbs2018).
At the population level, perturbations to normal sex ratios have been attributed to EDCs, both in nature and in the laboratory, along with impaired fertility rates (Langston et al., Reference Langston, Bryan, Burt and Gibbs2005; Sumpter, Reference Stentiford and Feist2005; Lewis & Ford, Reference Legler, van den Brink, Brouwer, Murk, van der Saag, Vethaak and van der Burg2012). It is therefore not inconceivable that EDCs could contribute to species decline and diminishing wildlife diversity, particularly when combined with other stressors such as climate change, over-exploitation and habitat loss. Faced with such complexity, it is easy to see why it may be difficult to isolate and prove involvement of EDCs in such declines. Nevertheless, it is instructive to consider some of the key observations and trends in ED-like effects, highlighted by freshwater and marine fish, and in relatively under-studied invertebrate taxa.
Fish
Because of clear gender-related differences in both external and internal morphologies, coupled with a well-studied, steroidally based endocrine system, some of the strongest indications of reproductive impairment in aquatic ecosystems have involved fish species. As a result, ED in wild freshwater and marine fish is now well-characterized – often associated with exposure to effluent from waste water treatment works (WWTW) where steroidal oestrogens may be present at concentrations significantly above background (Table 1). It is possible that other modes of action contribute to effects in fish though emphasis of research to date has focused on the actions of steroidal oestrogens. In nature a complex mixture with xeno-oestrogens, androgens, anti-oestrogens and anti-androgens may add to the spectrum of observed effects which may, consequently, be difficult to interpret in terms of causality.
There are numerous ways in which fish reproduction can be disturbed by contaminants, and effects detected range from altered secondary sexual characteristics and gonad histopathology to disruption of subtle mechanisms of endocrine regulation. Examples at the biochemical level include: altered steroid titres; induction of synthesis of the egg-yolk precursor vitellogenin (VTG) in males (or inhibition in females in the case of trenbolone – a synthetic androgen agonist); and/or induction of chorionic proteins within male or juvenile animals. At higher levels of organization, pathological changes in reproductive organs include altered number/size of eggs, skewed sex ratios, increased frequency of intersexuality (ovotestis) and reductions in sperm density and motility. A resultant shift in the reproductive cycle may ultimately affect population size and diversity. For example, delayed hatching has been described in marine medaka Oryzias melastigma (McClelland, 1839) (and offspring) following exposure to the surfactant perfluorobutanesulfonate, primarily as a result of disruption to the thyroid system (Chen et al., Reference Chen, Hu, Tsui, Wan, Peterson, Shi, Lam, Au, Lam and Zhou2018). Other population-level effects are reviewed by Marty et al. (Reference Lye, Bentley, Clare and Sefton2017) and some are discussed later in this review.
Amongst the first and most significant examples of ED in wild fish populations was that seen in roach, Rutilus rutilus (Linnaeus, 1758), in UK rivers influenced by WWTW and industrial effluents (Sumpter & Jobling, Reference Sumpter1995; Jobling et al., Reference Jobling and Tyler1998). Manifestations including VTG induction, gonadal intersex, lowered sperm counts in male fish and reduced fertility are now considered as classic indicators of environments containing high levels of sewage-derived oestrogens (notably the natural steroids 17β-oestradiol (E2), oestrone (E1) and the synthetic hormone (EE2)) or anti-androgens. Controlled, lake-scale experiments with fathead minnows Pimaphales promelas (Rafinesque, 1820) exposed to EE2 have since confirmed potential for population collapse due to such EDCs (Kidd et al., Reference Keay and Thornton2007, described later in this paper). Xeno-oestrogens – compounds that mimic or enhance the effects of endogenous oestrogens (Tyler & Routledge, Reference Thomas and Budiantara1998) – such as the surfactants nonylphenol NP and octylphenol OP, were also implicated in the feminization of fish populations (Sumpter & Jobling, Reference Sumpter1995; Jobling et al., Reference Jobling and Tyler1998): though their comparative activity appears to be considerably lower than steroidal oestrogens, concentrations of these synthetic chemicals in detergent-rich effluent may be considerably higher than natural hormones (Table 1). Fortunately, recognition of their impact has led to regulations and voluntary restrictions by industry, which has lessened their threat, at least throughout Europe. (It is hoped that the UK's exit from EU legislation will not reverse such improvement.)
Given the large number of potentially active chemicals used by humans, which may enter waterways via WWTW and other inputs, causality of ED is likely to be site specific and dependent on the composition of the chemical cocktail present (EEA, 2012). Incidence and severity of ED is thus highly variable and although widespread in UK rivers (with some fish populations exhibiting high percentages of affected individuals), elsewhere only small proportions of wild populations may be affected. For example, a US survey by Hinck et al. (Reference Hazlerigg, Tyler, Lorenzen, Wheeler and Thorbek2009) found intersex in only 3% of the fish collected and in only 4 of 16 species examined at 34 of the 111 sites: intersex was most prevalent in SE USA, in largemouth bass Micropterus salmoides (Lacépède, 1802) (18% of males) and smallmouth bass M. dolomieu (Lacépède, 1802) (33% of males). Despite this variance, the potential threat of ED for aquatic species is virtually ubiquitous, as illustrated by stickleback (Pungitius pungitius Linnaeus, 1758) from remote rivers in the Arctic (St Lawrence Island, Alaska) where expression of VTG in male fish was consistent with exposure to oestrogenic PCBs – a legacy from previous military installations. Gene expression changes and DNA methylation in gonads were also allied to PCB exposure (von Hippel et al., Reference Vogeler, Galloway, Isupov and Bean2018). Higher in the food chain, terns from PCB-contaminated locations, feeding on fish and aquatic invertebrates such as molluscs and crustaceans, exhibited skewed sex ratios and elevated intersex frequencies –supporting evidence of ED and highlighting the potential threat of bioaccumulation and trophic transfer of EDCs (Hart et al., Reference Hansson, Thain, Martínez-Gómez, Hylland, Gubbins and Balk2003).
Marine fish appear to be equally sensitive to the threat of ED. One of the simplest manifestations is reduced gonad size, measured as the gonadosomal index (GSI – gonad weight expressed as a proportion of somatic weight), or in extreme cases immaturity (no mature gonad) as in Atlantic croaker Micropogonias undulatus (Linnaeus, 1766) exposed experimentally to naphthalene and water-soluble fractions of diesel oil (Thomas & Budiantara, Reference Thomas1995). PCBs (Thomas, Reference Thitiphuree, Nagasawa and Osada1988), pesticides (Ram & Sathyanesan, Reference Raleigh and Keegan1986) and some metals (Singh, Reference Simpson, Bain, Brown, Brown and Lacey1989) have also been shown to influence GSI in the laboratory. In aquatic monitoring programmes poor gonad development has been observed in response to pollution in burbot Lota lota (Oken, 1817) from the Gulf of Bothnia, English sole Parophrys vetulus (Girard, 1854) in Puget Sound, and white sucker Catostomus commersonii (Lacépède, 1803) in Canada (reviewed in Hansson et al., Reference Hansen, vonWesternhagen and Rosenthal2017).
ED, manifested as low frequencies (<17%) of intersex (ovotestis) and elevated VTG concentrations (in males), occurs in marine fish such as flounders Platichthys flesus (Linnaeus, 1758), especially in estuaries subjected to EDC-containing WWTW and industrial effluents, which in the UK includes the Tyne, Tees, Wear and Mersey (Lye et al., Reference Love1997; Kirby et al., Reference Kidd, Blanchfield, Mills, Palace, Evans, Lazorchak and Flick2004). VTG induction and intersex levels in male flounder from the Mersey were found to be particularly elevated in the 1990s, raising concerns over links between endocrine disruption and environmental quality. The influence of hormone-containing sewage wastes and the presence of ubiquitous persistent organic compounds such as alkylphenols (considered by the EA as being at medium or high risk of EQS failure in the Mersey) were suggested as possible causes of ED. Since then, time-series monitoring indicates declining levels of VTG in male flounder (Kirby et al., Reference Kidd, Blanchfield, Mills, Palace, Evans, Lazorchak and Flick2004) consistent with reduced concentrations of EDCs following industrial decline and large-scale efforts to clean up domestic sewage and trade inputs, implemented in the Mersey basin at the end of the last century. More recent fish monitoring under the UK Clean Seas environmental monitoring programme (Defra) indicates continuing improvements – with <10% of male flounder exhibiting egg-yolk protein levels above 1 μg ml−1 in 2010, compared with >90% in 1996 (Defra 2009–2012).
Fish from more remote UK offshore sites, such as flounder and dab from the North Sea's Dogger Bank, also exhibit ovotestis and malformation of the male reproductive ducts (Allen et al., Reference Allen, Scott, Matthiessen, Haworth, Thain and Feist1999; Stentiford & Feist, Reference Spooner, Gibbs, Bryan and Goad2005). Unfortunately, from a monitoring viewpoint, these fish migrate extensively, and there may be uncertainty over the exposure site and regime during the critical gonad developmental period. The use of fish in marine monitoring programmes is therefore circumspect, to an extent, although they undoubtedly highlight important regional differences in ED.
Similar, if less pronounced, oestrogenic effects have been detected in offshore species in the USA, Japan and the Mediterranean, in addition to the UK (EEA, 2012). Pleuronectids from PCB-/PAH-contaminated parts of Puget Sound exhibited ED-like effects in terms of reduced E2 titres in blood and reduced fecundity (Johnson et al., Reference Jobling, Casey, Rodgers-Gray, Oehlmann, Schulte-Oehlmann, Pawlowski, Baunbeck, Turner and Tyler1988a, Reference Johnson, Casillas, Collier, McCain and Varanasi1988b); in contrast, flounder exposed to harbour dredge spoil had elevated plasma E2 and VTG levels (Janssen et al., Reference Janer and Porte1997). Induction of both VTG and ovotestis (2%) were detected in contaminated male flounder Pleuronectes yokohamae (Günther, 1877) from Tokyo Bay (Hashimoto et al., Reference Hart, Nisbet, Kennedy and Hahn2000). In vivo treatment of Atlantic salmon Salmo salar (Linnaeus, 1758) with nonylphenol has been shown to induce VTG and ER upregulation (along with a reduction of steroid metabolizing enzymes), signifying its oestrogen-mimicking properties, though no effects on reproduction were evident (Arukwe et al., Reference Arukwe, Forlin and Goksoyr1997). Direct suppression of hormones (testosterone and oestradiol) has also been observed in Atlantic croaker in response to laboratory exposure to PCB and PAH (Thomas, Reference Thitiphuree, Nagasawa and Osada1988).
Although oestrogenic effects predominate the ED literature on fish, a small number of masculinization events have been reported in several FW species (mosquitofish, killifish and catfish) in conjunction with exposure to pulp mill effluent (possibly due to androstenedione from bacterial degradation of plant sterols) and also from inputs of androgenic anabolic steroids from intensive cattle farming (Howell, Reference Horiguchi, Ohta, Nishikawa, Shiraishi, Shiraishi and Morita1999). Downstream, estuarine and coastal fauna could be vulnerable to these and other androgenic EDC (Alvarez-Munoz et al., Reference Alvarez-Muñoz, Indiveri, Rostkowski, Horwood, Greer, Minier, Pope, Langston and Hill2015), given that the androgen receptor (AR) in some marine species is known to bind contaminants such as organochlorines, albeit weakly, which may subsequently impair the gamete-maturation signalling pathway (Oberdorster & Cheek, Reference Noel, Barrett-Lennard, Guinet, Dangerfield and Ross2001).
Invertebrates
In parallel with warning signs that reproductive effects such as intersex and VTG induction may occur in fish subjected to anthropogenic waste, there is evidence that chemicals can influence growth, development and reproduction in invertebrates, though the support for an endocrine disrupting mechanism is generally weak – with the possible exception of TBT-induced imposex (in neogastropod snails) and intersex in certain molluscs, crustaceans and rotifers. Paucity of evidence for ED in invertebrates is partly due to an absence of a defined endocrine-mediated mode of action (MOA) but is hindered, generally, by a lack of targeted pollution research on invertebrate populations – particularly marine species. This omission should be rectified, particularly given the importance of coastal and estuarine habitats for wildlife biodiversity and food chains, and the increase in coastal urbanization (and associated contamination) worldwide. The following examples of imposex and intersex phenomena in invertebrate taxa are now well established and illustrate why it is important to include diverse organisms in environmental assessment programmes even in the absence of an established endocrine MOA. Notably, in the case of TBT-induced imposex, without the development and application of relevant diagnostic molluscan indices (i.e. reliance on routine in-vitro/vertebrate testing), the damage inflicted on marine ecosystems would have been more extensive and remained ‘under the radar’ for much longer.
Imposex and effects of TBT in molluscs
Tributyltin-induced imposex, observed in certain neogastropod molluscs, represents the most significant and specific manifestation of ED in the marine environment. The rise of the organotin (OT) ‘problem’ stemmed from the widespread use of TBT and, to a lesser extent, triphenyltin (TPT), as biocides in antifouling preparations (used to protect boat hulls and fish cages). Over the four decades since the initial discovery of the pollution-related origin of imposex in female dogwhelks Nucella lapillus – at the MBA's Plymouth Laboratory in the 1980s – more than 150 prosobranch species worldwide have been shown to exhibit similar effects. Specificity of the response to OT is matched by its sensitivity – initiated at concentrations of 1 ng TBT l−1 and possibly lower. Details are described in numerous papers (e.g. Bryan et al., Reference Bryan, Gibbs, Hummerstone and Burt1986, Reference Bryan, Gibbs, Burt and Hummerstone1987; Langston et al., Reference Langston, Pope, Arai, Harino, Ohji and Langston1990, Matthiessen & Gibbs, Reference Matthiessen1998; Langston & Pope, Reference Langston and Pope2009). A brief account of key features is warranted here to highlight the nature of the response, spatial and temporal trends, and the value of research in guiding regulatory action to remediate EDCs.
Imposex – the imposition of male characteristics (penis and vas deferens development) on females – was first noticed extensively in dogwhelks along the English Channel coast, and progresses along established indices of severity, culminating, at higher exposure levels, in the prevention of egg-laying and localized extinction of populations. The development of the Relative Penis Size Index (RPSI – penis size of females in a population compared with that of males) and Vas deferens Sequence Index (Figure 1) helped to quantify the extent and scale of impact and inform the development of TBT legislation (Bryan et al., Reference Bryan, Gibbs, Hummerstone and Burt1986, Reference Bryan, Gibbs, Burt and Hummerstone1987; Langston et al., Reference Langston, Pope, Arai, Harino, Ohji and Langston1990; Matthiessen & Gibbs, Reference Matthiessen1998).

Fig. 1. Nucella lapillus. Development of imposex, based on the VDSI and RPSI (after Gibbs et al., Reference Gibbs, Bryan, Pascoe and Burt1987, with permission).
Statutory measures invoked to halt damage from TBT to vulnerable marine species and ecosystems are detailed elsewhere (Champ & Seligman, Reference Champ and Seligman1996; Bray, Reference Bray and Langston2006; Arai et al., Reference Arai, Harino, Ohji and Langston2009). Studying the various aspects and timescales of recovery has provided a unique opportunity to understand the efficacy of TBT regulations. Two crucial pieces of legislation have influenced recovery from TBT pollution in the UK (with similar measures being adopted in many countries worldwide). The first of these measures, in 1987 in the UK, banned the use of TBT on vessels <25 m (the leisure market), but allowed continued use on larger vessels (commercial and naval fleets), based on the assumption that, since they spend most time on the open seas, biocide leachates from large ships would be diluted, harmlessly. However, doubts over this hypothesis, based on long-term surveillance of TBT concentrations and biological effects near shipping routes and ports, led to comprehensive legislation to remove, or seal in, tin-based coatings on the global fleet during the 2000s (final adoption and ratification of the IMO antifouling (AF) treaty in 2008, along with incorporation in UK/EU law).
The physical manifestations of imposex are irreversible, and recovery of affected populations is therefore dependent on recruitment from unaffected individuals by inward migration, or rafting of eggs and juveniles. Even at relatively ‘clean’ coastal sites, dogwhelk populations exhibited moderate imposex during the 1980s (>50% at VDS stage 3&4) and surprisingly few females (<1%) were without imposex, leading to extinction of many populations of N. lapillus subjected to boating traffic and near major shipping lanes – such as Plymouth Sound and many other locations along the south coast of England. Despite this extensive impact, recovery from imposex in populations of N. lapillus (and other neogastropods worldwide), is now evident at open coastal sites (few females now affected; see examples at Widemouth, Renney and Bude, Figure 2; Langston et al., Reference Langston, Chesman and Vane2015). Indeed, recruitment may be surprisingly rapid (~10 years), given that the species has no planktonic phase (Colson & Hughes, Reference Colson and Hughes2004; Bray et al., Reference Bray, McVean, Nelson, Herbert, Hawkins and Hudson2012). However, near ports – for example the Fal Estuary, UK – recovery of dogwhelks has been delayed by shipping impacts (Falmouth Docks), including the legacy of TBT retained by fine sediments with the potential for re-suspension and re-release, especially during dredging (Langston et al., Reference Langston, Chesman and Vane2015): enhanced VDSI and RPS were still present in N. lapillus near the port of Falmouth, 25 years after the height of the TBT problem, with >75% of females still affected (Figure 2). This represents limited improvement; higher upstream in the Fal Estuary, dogwhelks have still to recolonize previously inhabited sites.

Fig. 2. Nucella lapillus. Changes in mean VDSI at four sites in SW England over a 25 year period following introduction of TBT regulations in 1987 (after Langston et al., Reference Langston, Chesman and Vane2015, with permission).
Whilst reproductive traits in these gastropods are clearly highly sensitive to gender-influencing organotins, bivalves – particularly those in contact with, or feeding on, contaminated sediment – may be highly responsive to TBT through processes such as survival, growth and developmental effects. Notably, shell-thickening – observed in filter-feeding oysters Crassostrea gigas – is induced at comparable TBT concentrations to those causing imposex, and is due to interference with calcium channelling and deposition (Waldock & Thain, Reference von Hippel, Miller, Carpenter, Dillon, Smayda, Katsiadaki, Titus, Batzel, Postlethwait and Buck1983). Steroidal involvement has not been linked with this particular response (unlike imposex in snails, as discussed later in this paper). However, the presence and influence of sex-hormones in bivalves is intriguing and a cause for debate, given that testosterone metabolism and steroid titres have been shown to be affected by TBT in clams Ruditapes decussatus (Linnaeus, 1758) (Morcillo et al., Reference Morcillo and Porte1998).
The long-term impacts of TBT have been evaluated in estuarine populations of the sediment-dwelling gonochoristic clams S. plana. As a deposit-feeder, S. plana is responsive to various sediment-associated contaminants, including TBT (Langston & Burt, Reference Lai, Johnson, Scrimshaw and Lester1991). At heavily TBT-contaminated sites, typified by the Solent area of the English Channel, clam numbers declined considerably during the 1980s, as TBT concentrations peaked (Figure 3A, reproduced from Langston et al., Reference Langston, Chesman and Vane2015). The subsequent efficacy of TBT regulatory measures in achieving chemical and ecological improvements is also demonstrated in these long-term observations. As with TBT-impacted dogwhelks, clam recruitment patterns and abundance have recovered (slowly – over 25 years) as TBT concentrations fall in response to pollution control measures, confirming the close link between TBT and reproductive success in these bivalves. The underlying mode of action in these bivalves is unclear since, in contrast to TBT-induced imposex in neogastropods, there are no analogous reproductive structures (penis, vas deferens) in S. plana and therefore no comparable indices of masculinization. However, analysis of sex ratios in clams from the Solent area indicated deviation from the expected ratio of 1:1 (Langston et al., Reference Langston, Chesman and Vane2015). At sites where TBT concentrations in sediments were highest, there was a significant bias towards males (2:1) compared with the Channel average (N = 107 sites) of 0.998:1 (Figure 3B). Influence of TBT on reproductive development and success in bivalves such as S. plana is therefore likely. Masculinization and reduced larval production was similarly thought to have accompanied TBT-related decline in UK native oysters Ostrea edulis (Linnaeus, 1758) (Thain & Waldock, Reference Tankoua, Amiard-Triquet, Denis, Minier, Mouneyrac and Berthet1986).

Fig. 3. Scrobicularia plana. (A) Abundance of clams (numbers collected per man hour) at Northam in the Itchen Estuary 1978–2009. Data in 1978 are total clam numbers; numbers of different sized clams (10 mm intervals) are indicated at other times. Upper numbers above bars are mean TBT seawater concentrations (ng l−1 as Sn) for periods 1986–1988, 1990–1992, 2000–2009 (left to right). Lower numbers are mean sediment TBT concentrations (μg g−1 as Sn) for the same periods. (B) Relationship between sex ratio in S. plana and TBT content of sediment, Solent Area, July 2009. Dashed line is mean (± SE) sex ratio for English Channel (2009–2012, N = 107 sites). (after Langston et al., Reference Langston, Chesman and Vane2015, with permission).
Intersex and other effects in invertebrates
Intersex is a condition found in normally gonochoristic species (separate sexes) where both male and female gametes are present in the same individual. In contrast to TBT-induced imposex, precise causality of intersex is seldom definite (European Environment Agency, 2012). The appearance of oocytes in the testes (ovotestis) is regarded as an endpoint of endocrine disruption in male fish and has been linked to the presence of endocrine disrupting compounds which are thought to mimic the actions of the female sex hormone 17β-oestradiol, and thus disrupt natural hormonal functioning (Bateman et al., Reference Bateman, Stentiford and Feist2004). In contrast to fish, unequivocal confirmation of environmentally relevant ED effects on the reproductive systems of aquatic invertebrates is scarce because of the lack of basic knowledge of invertebrate endocrine systems. Another issue concerns frequency of intersex – which may be present in only a proportion of the population – and in some species is not much lower at perceived non-contaminated sites than in polluted areas. The presence of intersex at so-called pristine sites, in gonochoristic species, does not necessarily signify inherent hermaphroditism but could reflect that there may be few sites where pollution is truly absent. Nevertheless, there are invertebrate examples which imply that the intersex condition is a response to environmental quality – one of which is the estuarine clam S. plana which, as discussed above, is sensitive to TBT.
Initial survey of S. plana populations in the Avon Estuary, SW England, chosen to represent a relatively uncontaminated reference site, showed unexpectedly that intersex, in the form of ovotestis, was present in varying degrees of severity in males (Chesman & Langston, Reference Chesman and Langston2006; Langston et al., Reference Langston, Burt, Chesman and Vane2007a). This feature, together with the fact that these clams are intrinsically gonochoristic (Hughes, Reference Howell1971; Ruiz, Reference Routledge and Sumpter1993), widely distributed, sedentary deposit-feeders make S. plana a potentially valuable monitoring species – prompting the development of an intersex index (Figure 4) to assess the severity of the condition, and offering potential for widespread screening and investigation.

Fig. 4. Scrobicularia plana. (A) Gonadal follicles containing sperm or oocytes in normal male and female, respectively. Scale bars = 120 μm. (B) Oocyte distribution patterns in gonads of clams exhibiting increasing severity of ovotestis: examples illustrate the intersex index scores 1–6 (Scale bars 1–4 = 80 μm, Scale bars 5–6 = 120 μm) (after Chesman & Langston, Reference Chesman and Langston2006, with permission).
Long-term observations in the Avon, a rural south Devon estuary with little industry or urbanization, revealed further insights into the sexuality of these clams. Gametogenesis commences in April and by early July most of the adults have mature gonadal cells, which in many individuals were either sperm or oocytes (Figure 4A). However, ovotestis was manifested in a percentage of individuals (Figure 4B), increasing in frequency until spawning (late July) and subsequently receding, as the gonads regressed (Figure 5). The proportion of females in the sampled populations remained unchanged at ~50% throughout, whilst the proportion of unaffected males dropped, especially in July, implying that intersex represents feminization of males. The affected males exhibited varying degrees of ovotestis with an index of severity ranging from a single oocyte in otherwise normal testicular tissue, to large regions of mature ovarian tissue interspersed within testicular tissue (Figure 4B). The causes and consequences of the ovotestis condition in S. plana are speculative, though given its undeveloped catchment, one of the most likely causes of intersex in the Avon appears to be agriculture, notably livestock (cattle) which, at the time, were able to access the waterway unhindered.

Fig. 5. Scrobicularia plana. Seasonal development and declining trends in intersex in the Avon estuary (SW England) coinciding with local efforts to fence off access to the waterway by cows. Inset: the decline in cattle numbers in the UK dairy herd.
Nearby commercial oyster beds in the Avon have been closed periodically because of high bacterial (Escherichia coli) counts, and though there is ongoing debate as to the importance of wastewater and agricultural sources, bacterial DNA analysis points to bovine sources. The implication is that even ‘undeveloped’ estuaries can be impacted by a relatively moderate level of anthropogenic activity, including dairy farming. Annual trends in intersex in Avon clams, with respect to cattle statistics, add credence to this hypothesis; proportions of affected male clams have declined over several years since initial intersex observations in 2004 (Figure 5), coinciding with efforts by the farming community to prevent cattle directly accessing the waterway (by fencing off pastureland). Given that cows (especially pregnant females) may excrete milligram quantities of oestradiol during micturition, removal of this source could clearly have benefits to clams and other fauna inhabiting adjacent mudflats and which may accumulate exogenous oestrogens. Coinciding with this removal of local direct sources, and the decline in intersex, there has been an overall national reduction in the dairy herd (Figure 5). Agriculture may therefore represent a potential source of feminization, compounding the influence of domestic and industrial wastes where these are prevalent.
Broader examination of populations of S. plana in SW England showed that disruption to the ‘normal’ gonadal development of male clams is widespread (17 out of 23 populations exhibited intersex with 60% of males from the Bristol Channel and Severn Estuary, the most impacted area surveyed, displaying the condition). Oocyte size was also higher in the Severn, notably at upstream sites (Langston et al., Reference Langston, Burt, Chesman and Vane2007a). Subsequently, an extensive survey of more than 100 sites along English and French Channel coastlines in 2009–2014 returned a similar range of frequency and severity figures (Pope et al., Reference Pollock, Dube and Schryer2015); intersex was present in 58% of populations studied and proportions of affected males ranged from 0–53% (average 9%). The severity of intersex in Channel clams varied across the entire classification range (stages 1–6, Figure 4), though the majority of impacted individuals show only low severity (68% stage 1, 13% stage 2 and above). The overall mean M:F ratio was almost unity (1.007), indicating equal numbers of males and females, though sex ratios were significantly skewed at some sites: Nine populations were significantly biased towards females, six biased towards males – the latter including sites known to be affected by TBT (Pope et al., Reference Pollock, Dube and Schryer2015). Intersex frequencies varying between 1.5% and 71% have also been reported in S. plana from other estuarine locations in Europe, including Ireland (Raleigh & Keegan, Reference Quinn, Gagné, Costello, McKenzie, Wilson and Mothersill2006), Portugal (Gomes et al., Reference Gomes, Gazo, Besnardeau, Hebras, McDougall and Dumollard2009) and NW France (Tankoua et al., Reference Sumpter and Jobling2012).
It is possible that low levels of intersex (up to 10%) are the ‘norm’ for this species. Establishing a true baseline for the species is an important priority for the future, but may prove difficult since there are likely to be few, if any, estuaries that are completely free from any human impact, as demonstrated by the Avon. However, clam populations sampled in the Fowey, Exe and Axe estuaries (SW England) and, notably, the undeveloped NW of Scotland were found to be completely free of intersex (none found in 186 clams examined from Scottish west coast mainland sites, Mull, Skye and Harris in the Outer Hebrides). There was no departure from unity in male to female sex ratios in any of these populations, implying that zero intersex (strict gonochorism) is probably the true reference condition in S. plana and that values above that reflect anthropogenic influences.
Some of the highest levels of intersex severity in S. plana have been observed in the Mersey Estuary (20% of clams exhibiting stage 6), consistent with elevated ED effects-levels in flounders from this highly industrialized and urbanized estuary (Lye et al., Reference Love1997; Kirby et al., Reference Kidd, Blanchfield, Mills, Palace, Evans, Lazorchak and Flick2004). This similarity strengthens the hypothesis linking intersex in S. plana with anthropogenic sources of EDC. Likewise, experimental exposures of S. plana to sediment spiked with mixtures of E2, EE2 (100 μg kg−1), octylphenol (OP) and nonylphenol (NP) (1000 μg kg−1), or individually to E2 or NP, indicate that oestrogens and xeno-oestrogens could be a contributory factor in the induction of intersex in male clams (up to 44% of exposed males exhibited ovotestis compared with 0–8% in controls; Langston et al., Reference Langston, Burt, Chesman and Vane2007a, Reference Langston, Burt and Chesman2007b). Furthermore, a link between intersex frequency in Scrobicularia populations and oestrogenicity in sediments, as determined by YES assay, has been observed (Figure 6) adding to the weight of evidence that this species is susceptible to EDC. If, as suspected, oestrogenic activity resides principally in sediment (Peck et al., Reference Pascoal, Carvalho, Vasieva, Hughes, Cossins, Fang, Ashelford, Olohan, Barroso, Mendo and Creer2004), as a deposit-feeder, S. plana might be considered sensitive to ensuing impacts from sediment-bound contaminants and appears to have ideal credentials as a sentinel species.

Fig. 6. Scrobicularia plana. Relationship between intersex and oestrogenic activity (YES assay) in sediments (SW estuaries): probability of the observed values was 0.027 suggesting that the E2 level might influence intersex. R = 0.588 (unpublished observations, Langston and Routledge).
Mechanisms of the inducible feminizing effects in S. plana have yet to be determined and it is not yet confirmed that these observations indicate endocrine involvement. However, the absence of size-related differences in the sex of individuals denote that protandry or protogyny are not reproductive strategies for this species, further supporting the view that ovotestis is linked to anthropogenic influences. Endocrine disruption in a range of species has now been linked to a range of sources including exposure to WWTW effluents, general estuarine pollution (Skelly et al., Reference Singh2010; Schultz et al., Reference Schulte-Oehlmann, Tillmann, Casey, Duft, Markert and Oehlmann2013), agriculture (McCoy et al., Reference McAllister and Kime2008; Orton & Routledge, Reference Oehlmann, Di Benedetto, Tillmann, Duft, Oetken and Schulte-Oehlmann2011), aquaculture (Ford et al., Reference Ford, Read, Jones, Michino, Pang and Fernandes2007), pharmaceutical manufacture (Sanchez et al., Reference Ruiz2011) and paper mill effluents (Pollock et al., Reference Peck, Labadie, Minier and Hill2010), or a combination thereof. Whilst the relative importance of different sources has yet to be determined, S. plana clearly offers potential for large-scale screening and investigation of reproductive effects in the estuarine environment, helping to focus remediatory strategy where it would be most beneficial.
Synergistic and antagonistic interactions between oestrogenic and androgenic EDC are feasible in nature and can cause complications in the interpretation of intersex levels and sex ratios – for example in S. plana collected at high TBT sites (discussed above), and in similarly exposed soft shell clams Mya arenaria (Linnaeus, 1758) (Gagné et al., Reference Gagné, Blaise, Pellerin, Pelletier and Strand2006) and mussels Mytilus galloprovincialis (Lamarck, 1819) (Ortiz-Zarragoitia & Cajaraville, Reference Orton and Routledge2010). Reproductive impairment (lower Gonadal Index), skewed sex ratios in favour of males, and elevated incidence of intersex (in both females and males) has also been observed in the venerid bivalve Gamphina veneriformis (Lamarck, 1818) as a result of prolonged exposure in the laboratory to elevated TBT levels (0.4–0.8 μg l−1) during early gonadal development (Park et al., Reference Osada, Takamura, Sato and Mori2015). Overall intersex frequencies in ‘control’ G. veneriformis were ~4%, rising to 31% at high TBT exposure (similar ranges to incidence levels in S. plana from UK estuaries). Measurement of steroid hormone levels in TBT-exposed gastropods and bivalves suggest an increase in testosterone and decrease in oestradiol (Horiguchi et al., Reference Hinck, Blazer, Schmitt, Papoulias and Tillitt1997; Morcillo & Porte, Reference Moore and Stevenson2000; Abidli et al., Reference Abidli, Santos, Lahbib, Castro, Reis-Henriques and El Menif2012); nevertheless, as with responses to other contaminants, the mode of action of TBT in many invertebrates is still tentative with regard to endocrine involvement. An alternative suggestion put forward by Hagger et al. (Reference Gross, Maycock, Thorndyke, Morritt and Crane2006) proposes a causal association between imposex and genotoxicity, though this relationship has yet to be confirmed extensively.
Low levels of intersex are known to occur in other gonochoristic bivalves, worldwide, including mussels Perumytilus purpuratus (Lamarck, 1819) from Argentina (Villalobos et al., Reference Varaksina, Varaksin and Maslennikova2010), razor clams Tagelus plebius (Lightfoot, 1786) and giant coquina Iphigenia brasiliana (Lamarck, 1818) from Brazil (Ceuta et al., Reference Ceuta, Boehs and Santos2007), Ruditapes decussatus (Linnaeus, 1758) from Galicia, Spain (Delgado & Camacho, Reference Delgado and Camacho2002) and blood cockles Anadara granosa (Linnaeus, 1758) and A. antiquata (Linnaeus, 1758) from Indonesia (Afiati, Reference Afiati2007) – though in the latter two examples low incidences of ovotestis are believed to signify protandric hermaphroditism as opposed to exogenous response. Nevertheless, surveys of R. decussatus and cockles Cerastoderma glaucum (Bruguière, 1789) from areas receiving effluent from waste water treatment plants in Tunisia imply more extensive frequencies of intersex due to pollution (Hamza-Chaffai, Reference Hagger, Depledge, Oehlmann, Jobling and Galloway2013). Experimentally, exposure of rock oysters Saccostrea glomerata Gould, 1850 to EE2 during early gonadogenesis has been shown to stimulate the transition of protandrous males to intersex and, eventually, females, though again the mechanism is uncertain (Andrew et al., Reference Andrew, O'Connor, Dunstan and Macfarlane2010).
Other observed reproductive effects in molluscs include enhanced embryo production at high dilutions of oestrogenic sewage effluent in freshwater mud-snails Potamopyrgus antipodarum (Gray, 1843), changing to inhibitory effects at low dilutions – a similar dose response to that following lab exposure of the same species to known oestrogenic chemicals (Jobling et al., Reference Jobling, Nolan, Tyler, Brighty and Sumpter2003). Enhanced oocyte production (along with proliferation of secondary sexual tissues) is also described in apple snails Marisa cornuarietis Linnaeus, 1758 and dogwhelks N. lapillus exposed to the xeno-oestrogens BPA and alkylphenol (Oehlmann et al., Reference Oberdörster and Cheek2000; Schulte-Oehlmann et al., Reference Schug, Johnson, Birnbaum, Colborn, Guillette, Crews, Collins, Soto, vom Saal, McLachlan, Sonnenschein and Heindel2001). Reduced penis, prostate and development were observed in male N. lapillus. Interestingly, the responses of Marisa to BPA could not be reproduced in studies by Forbes et al. (Reference Forbes, Warbritton, Aufderheide, Van Der Hoeven and Caspers2008), indicating that environmental conditions (tank sizes, design and configuration of animal exposures) can influence the outcomes of such experiments.
DDT exposure has been linked with altered germ cell development in freshwater mussels Dreissena polymorpha Pallas, 1771 in contaminated sections of Lake Maggiore: effects include oocyte degradation, and delayed sperm release, and may be symptomatic of neuroendocrine interference. The resultant asynchrony between sexes could compromise reproductive success (Binelli et al., Reference Binelli, Bacchetta, Vailati, Galassi and Provini2001, Reference Binelli, Bacchetta, Mantecca, Ricciardi, Provini and Vailati2004). In contrast, E2 (and other sex steroids – testosterone and progesterone) stimulate both oogenesis and spermatogenesis in scallops Mizuhopecten yessoensis (Jay, 1857) and Placopecten magellanicus (Gmelin, 1791) leading to increased gonad weight and larger oocyte diameter (Varaksina & Varaksin, 1991; Varaksina et al., Reference Varaksina and Varaksin1992; Wang & Croll, Reference Waldock and Thain2004). Significantly, injections of E2 at early stages of sexual maturation of the oyster C. gigas have been shown to induce sex reversal from male to female (Mori et al., Reference Morcillo, Ronis and Porte1969), illustrating the potential impact of non-endogenous sex steroids on bivalve sexuality.
Given this weight of evidence, not surprisingly, various measures of ‘endocrine disruption’ in molluscs, notably intersex, have been linked to sewage treatment works (WWTW) effluents (Moore & Stevenson, Reference Mintram, Brown, Maynard, Liu, Parker, Tyler and Thorbek1991; Chambers et al., Reference Chambers, Allard, Walker, Marsalek, Lawrence, Servos, Busnarda, Munger, Adare and Jefferson1997; Gagné et al., Reference Gagné, Blaise, Salazar, Salazar and Hansen2001; Gross et al., Reference Gomes, Gonzalez-Rey and Bebianno2001; Zulkosky et al., Reference Zhang, Zuo, Wang, Yu, Chen and Wang2002; Jobling et al., Reference Jobling, Nolan, Tyler, Brighty and Sumpter2003; Quinn et al., Reference Pope, Childs, Dang, Davey, O'Hara, Langston, Minier, Pascoe, Shortridge and Langston2004; Andrew-Priestley et al., Reference Andrew-Priestley, O'Connor, Dunstan, Van Zwieten, Tyler, Kumar and MacFarlane2012), or known areas of anthropogenic contamination (Moore & Stevenson, Reference Mintram, Brown, Maynard, Liu, Parker, Tyler and Thorbek1991; Horiguchi et al., Reference Horiguchi, Shiraishi, Shimizu and Morita2000; Gagné et al., Reference Gagné, Blaise, Pellerin and Gauthier-Clerc2002; Gauthier-Clerc et al., Reference Gauthier-Clerc, Pellerin, Blaise and Gagné2002; Barbeau & Grecian, Reference Barbeau and Grecian2003; Lye et al., Reference Lye, Frid, Gill and McCormick2005; Matozzo & Marin, Reference Marty, Blankinship, Chambers, Constantine, Kloas, Kumar, Lagadic, Meador, Pickford, Schwarz and Verslycke2007; Lee et al., Reference Lavicoli, Fontana and Bergamaschi2010).
There are additional changes in secondary sexual characteristics in marine arthropods (notably amphipods), which may be used to diagnose intersex. Intersex males possess rudimentary brood plates (female characteristics) and intersex females possess genital papillae (male characteristics) which may be used to assess the frequency and severity of intersexuality (number of papillae in females may denote degree of maleness). Intersexuality (and biased sex ratios) has been observed in the amphipod Echinogammarus marinus (Leach, 1815), frequency of which has been tentatively linked, partly, to feminizing microsporidian parasites. However, higher prevalence of intersex (affected males and females) was found at sites receiving industrial discharges in the Firth of Forth (~15%) when compared with reference sites (5–8%), suggesting parasitism is not the only cause of intersex and that pollution could be involved (Ford et al., Reference Ford, Fernandes, Robinson, Davies and Read2006). Analogous variability in intersex frequency (0.8–4.9%) is seen in freshwater Gammarus pulex (Linnaeus, 1758) from the Rivers Test and Itchen, with increasing levels found near WWTW, further implying a link to pollution (Love, Reference Lewis and Ford2017). Gammarus spp. have also been shown, experimentally, to be sensitive to EE2 and BPA, at least at comparatively high doses. Unlike fish, however, there is as yet no clear link in these amphipods to effects involving hormonal systems – though juvenile hormone mimics in crustaceans are known to have effects on vitellogenesis, intersex levels and sex ratios (EEA, 2012). Intersex frequency in E. marinus, as in S. plana, is seasonal and is lower in winter months, compared with warmer spring/summer months, as organisms mature. Other potential crustacean indicators include copepods (intersex levels found in sewage-exposed populations by Moore & Stevenson, Reference Mintram, Brown, Maynard, Liu, Parker, Tyler and Thorbek1991) and shore crab Carcinus maenas (Linnaeus, 1758), with male crabs displaying feminized abdomens and reduced claw depth in polluted UK estuaries (EDIT, 2003). Much of the information on endocrinology in crustaceans concerns the role of ecdysone and several peptide hormones in moulting and vitellogenesis, though the latter has yet to receive widespread attention as an ED biomarker in crustaceans. One exception is the induction of vitellogenin in grass shrimp Palaeomonetes pugio (Holthuis, 1949), following exposure to the PAH pyrene. In addition, the function of methyl farnesoate (a crustacean analogue of the insect growth hormone) may be compromised by insecticides designed to disrupt development (Oberdörster & Cheek, Reference Noel, Barrett-Lennard, Guinet, Dangerfield and Ross2001).
In annelids, effects on development and reproductive output have been described in worms exposed experimentally to the xeno-oestrogen nonylphenol (Depledge & Billinghurst, Reference Depledge and Billinghurst1999; Bettinetti et al., Reference Bettinetti, Cuccato, Galassi and Provini2002). Whilst an endocrine mode of action has not been established in these effects, various EDCs appear capable of agonistic and antagonistic activity, in vitro, on the annelid oestrogen receptor (Keay & Thornton, Reference Jones, Walker, Azizi, Tolbert, Williams and Snell2009). For these and the majority of invertebrate phyla however, our understanding of basic endocrinology, and hence true EDC-like effects, is sparse. For this level of analysis in aquatic environments we remain reliant on vertebrate responses, notably those described in fish.
Mechanisms
Vertebrates
The most obvious mechanism by which EDC can affect reproductive activity in vertebrates is through interference with the function of nuclear receptors (NR, such as the Oestrogen, Androgen and Retinoid- X Receptors – ER, AR, RXR, respectively), resulting in altered gene expression. NRs are able to interact with endogenous ligands (e.g. hormones) or exogenous equivalents, including pollutants, which can perform either as agonists or antagonists. The development of transgenic mouse models and advances in genomics and proteomics has led to the expansion of in vitro assays, based on ER/AR potencies (including YES and ER-calux described earlier). These assays allow widespread screening of chemicals for their hormone activity and mode of action and are often used to guide the requirements for further animal testing. Relevance to lower organisms sometimes remains unclear and, ultimately, determination of ED impact and risk may depend upon identification of responses in vivo (as in the example of intersex in S. plana; Figure 6) since these effects usually integrate key processes such as bioaccumulation, elimination and metabolism.
Invertebrates
The most intensive work on mode of action in compromised invertebrate reproductive systems relates to TBT-induced imposex in marine snails. It is likely that TBT interacts with the endocrine system in these gastropod molluscs, though there are several hypotheses as to the precise mechanism. Suggestions for the main drivers of gender disruption include neuropeptides such as APGWamide, secreted by various ganglia of the central nervous system (Oberdörster & Cheek, Reference Noel, Barrett-Lennard, Guinet, Dangerfield and Ross2001), and effects on steroid hormone titres. Methyltestosterone and the fungicide fenarimol have been shown to induce imposex in experimentally exposed snails, as does APGWamide, but in terms of the impacts of TBT in nature, the precise mode of action is still uncertain and may involve impeding conversion of testosterone to oestrogens in females (by inhibition of aromatase), targeting of nerves and ganglia associated with the neuro-endocrine system, or abnormal modulation of the retinoid-X receptor (Spooner et al., Reference Sonne, Leifsson, Dietz, Born, Letcher, Hyldstrup, Riget, Kirkegaard and Muir1991; Castro et al., Reference Castro, Lima, Machado, Melo, Hiromori, Nishikawa, Nakanishi, Reis-Henriques and Santos2007; see below).
Even less certain is the mode of action in bivalves, but the TBT-related declines in UK native oyster (Ostrea edulis) and clam (S. plana) populations were thought to be associated with masculinization or failure to differentiate, and by reduced larval production and recruitment (Thain & Waldock, Reference Tankoua, Amiard-Triquet, Denis, Minier, Mouneyrac and Berthet1986; Langston et al., Reference Langston, Chesman and Vane2015), which may involve similar mechanisms to those seen in gastropods (Matthiessen, Reference Matozzo and Marin2008).
In the freshwater prawn Macrobrachium rosenbergii (De Man, 1879), proteomic analysis has shown that proteins involved in the endocrine system and in the hormonal control of reproduction (including vitellogenin (VTG), farnesoic acid (FA), and o-methyltransferase (OMT)) may be deregulated by the organochlorine insecticide chlordecone (Lafontaine et al., Reference Lafont and Mathieu2017), which is thus suggested to be an EDC for decapods, as in vertebrates (where it binds to ER). However, although a number of vertebrate-like sex steroids occur in phyla such as molluscs and crustacea, their origins, targets and mode of action in controlling reproduction (and identities of chemicals which interfere in this process) are often ambiguous. It is unlikely, for example, that upregulation of VTG, FA or OMT in the prawn M. rosenbergii is explainable by the interaction of chlordecone with endogenous oestrogen and ER pathways, as in vertebrates; rather it may involve signalling pathways of other hormones and neuroendocrine organs – though may still be manifested in similar endpoints, such as altered oocyte/VTG production or sex differentiation (Sumpter & Jobling, Reference Sumpter1995; Lafontaine et al., Reference Lafont and Mathieu2017).
Nevertheless, based on work with the scallop M. yessoensis, Osada and co-workers (Osada et al., Reference Ortiz-Zarragoitia and Cajaraville2003; Thitiphuree et al., Reference Thain and Waldock2019) have led the alternative viewpoint that the biosynthetic pathway for sex steroids is similar to that of vertebrates and that vertebrate steroid-like receptors are functionally important in invertebrates, even if not classically similar to those in vertebrates, and the debate is still very much ongoing. A review of research on the identification and functioning of invertebrate receptors and pathways, in comparison to those in their vertebrate counterparts can be found in Lafont & Mathieu (Reference Kirby, Allen, Dyer, Feist, Katsiadaki, Matthiessen, Scott, Smith, Stentiford, Thain, Thomas K, Tolhurst and Waldock2007). More recently Blalock et al. (Reference Blalock, Robinson, Loguinov, Vulpe, Krick and Poynton2018) have reported evidence of a cholesterol side chain cleavage enzyme consistent with endogenous production of steroids and oestrogen signalling pathway, as a likely mechanism of action in mussels M. edulis exposed to 17-α-ethinylestradiol and 4-nonylphenol. A ligand-activated ER-like receptor has also been found in reproductive tissue of marine rotifers Brachionus manjavacas (Fontaneto, Giordani, Melone & Serra, 2007) which appears to bind human oestradiol and regulates reproductive output in females. This finding implies conservation of ER structure and function since the divergence of deuterostomes and protostomes 0.5 billion years ago (Jones et al., Reference Johnson, Misitano, Sol, Nelson, French, Ylitalo and Hom2017). However, among invertebrates active involvement of ER appears, on current evidence, to be the exception rather than the rule. Annelids may be another such exception, with ER apparently capable of binding oestradiol. In contrast, most invertebrate ER ligand-binding domains have occluded binding sites, preventing activation.
An orthologue of the vertebrate ER occurs in the molluscan genome but does not appear to bind to or be activated by vertebrate oestrogens (Bannister et al., Reference Bannister, Beresford, Granger, Pounds, Rand-Weaver, White, Jobling and Routledge2013) and no equivalent testosterone receptor has been identified. The term endocrine disruption is, therefore, ambiguous when applied to effects on such invertebrate reproductive systems. However, as indicated earlier, the involvement of the retinoid receptor RXR is implicated in molluscan imposex – in rock shell Thais clavigera (Küster, 1860) and N. lapillus – and activation and disruption of gene expression profiles has been demonstrated for both 9-Cis retinoic acid (a vitamin A derivative) and TBT (Castro et al., Reference Castro, Lima, Machado, Melo, Hiromori, Nishikawa, Nakanishi, Reis-Henriques and Santos2007; Horiguchi et al., Reference Horiguchi, Takiguchi, Cho, Kojima, Kaya, Shiraishi, Morita, Hirose and Shimizu2008). Isolation of TBT-responsive nuclear receptors has been performed in other marine molluscs (and humans); Pacific oysters, Crassostrea gigas – also susceptible to TBT – host genes for a retinoic acid receptor orthologue (RAR) and a peroxisome proliferator-activated receptor orthologue (PPAR), as well as RXR (Castro et al., Reference Castro, Lima, Machado, Melo, Hiromori, Nishikawa, Nakanishi, Reis-Henriques and Santos2007; Vogeler et al., Reference Villalobos, Paz and Gonzalez2017). Computer predictions confirm the potential for RXR to bind 9-cis retinoic acid and TBT (with, it is hypothesized, corresponding effects on development of oyster larvae) supporting the concept that these NRs could be an important part of the mode of action of some EDC in invertebrates. PPAR and Gonadotrophin Releasing Hormone (and its receptor) are amongst other contenders for a role in imposex development in snails along with inhibition, by TBT, of testosterone-transforming aromatase (Spooner et al., Reference Sonne, Leifsson, Dietz, Born, Letcher, Hyldstrup, Riget, Kirkegaard and Muir1991; Castro et al., Reference Castro, Lima, Machado, Melo, Hiromori, Nishikawa, Nakanishi, Reis-Henriques and Santos2007; Pascoal et al., Reference Park, Shin, Hung, Romano, Cheon and Kim2013).
Competitive interactions between EDCs add to the difficulty in interpreting modes of action. The anti-androgens cyproterone acetate and vinclozolin both appear capable of blocking the androgenic effects and activity of TBT in snails, whilst in M. cornuarietis it seems likely that activity of BPA (enhancing oocyte production) could be blocked by co-administration of other ER agonists (Oehlmann et al., Reference Oehlmann, Schulte-Oehlmann, Bachmann, Oetken, Lutz, Kloas and Ternes2007). Furthermore, in higher (chordate) invertebrates, neurodevelopment and pigmentation in developing embryos seems to be impaired by EDCs such as BPA, through action on NRs, in a mechanism analogous to ED (Gomes et al., Reference Gomes, Gazo, Besnardeau, Hebras, McDougall and Dumollard2019). This indicates susceptibility to EDCs, even though the mode of action does not directly involve ER or AR (which appear to have been lost in ascidians), nor does it appear to affect reproduction overtly. The PXR (Pregnane X Receptor) is a possible alternative candidate to bind EDCs in Ascidians.
Work done on invertebrates therefore suggests that EDCs can exert impact in a number of different ways not necessarily akin to ER/AR-based ED in vertebrates; as a result, effects of EDs, and susceptibility, may vary greatly among invertebrate species (Mintram et al., Reference Miller and Ankley2018).
Indigenous or exogenous hormones and their mimics? Bioaccumulation via dissolved and particulate pathways
Sex steroids may play a functional role in reproduction in some molluscs, as demonstrated by sex reversal and induction of vitellin-like protein in E2-injected bivalves (Mori et al., Reference Morcillo, Ronis and Porte1969; Blaise et al., Reference Blaise, Gagné, Pellerin and Hansen1999; Osada et al., Reference Ortiz-Zarragoitia and Cajaraville2003), correlations between steroid titres and gonad development in clams and mussels (Lafont & Mathieu, Reference Kirby, Allen, Dyer, Feist, Katsiadaki, Matthiessen, Scott, Smith, Stentiford, Thain, Thomas K, Tolhurst and Waldock2007), and penis development in testosterone-treated female snails (Bettin et al., Reference Bettin, Oehlmann and Stroben1996). Indeed, the presence of steroid hormones (and other non-steroidal EDCs) in invertebrate field samples now seems incontrovertible (Janer & Porte, Reference Hughes2007; Scott, Reference Schwarz, Katsiadaki, Maskrey and Scott2018), though evidence of an endogenous biosynthetic pathway, involvement in reproduction and their mode of action in terms of ED is equivocal: steroid receptors – present, for example, in molluscs – have still to be shown to be active in mediating steroid action (Matthiessen, Reference Matozzo and Marin2008). There is, however, growing evidence that marine invertebrates are capable of accumulating hormones and their mimics from their surrounding milieu. It is therefore important to consider exposure sources, distribution behaviour and bioaccumulation pathways as part of the risk assessment process.
Partitioning of EDCs and the importance of sediments for marine invertebrates
Dominant sources of EDCs to the marine environment include industrial discharges, consumer products, treated and untreated wastewater, urban and agricultural runoff (animal waste and fertilizer/pesticide application). Concentrations are likely to be highest near points of release into aquatic environments, but may be dispersed by currents and tides and partitioned between liquid and solid phases, with differing bioavailabilities.
A significant knowledge gap relates to the possibility that much of the oestrogenicity in aquatic environments may reside in benthic sediments and, if bioavailable, may be transferred to benthic biota (and hence through the food chain). Because sediments may concentrate contaminants perhaps hundreds to thousands of times higher than overlying water, biological availability of only a small proportion of this particulate loading could result in significant uptake in filter-feeders and other benthic invertebrates which are in direct content with sediments.
Previous observations regarding the bioavailability and effects of TBT have highlighted the role of sediments in the sequestration and concentration of EDCs in estuarine environments. TBT partition coefficients (Kd – the ratio of TBT in sediment relative to water) are relatively high – typically of the order of 105 in estuarine muds – with consequences for deposit-feeding organisms such as clams S. plana (Langston et al., Reference Langston, Pope, Arai, Harino, Ohji and Langston1990; Langston & Burt, Reference Lai, Johnson, Scrimshaw and Lester1991; Langston & Pope, Reference Langston and Burt1995). Investigations of sediment–water partitioning of other EDCs, including the hormone E2 and the alkylphenolic surfactant NP, show that sediments have an important role in their environmental behaviour also (Lai et al., Reference Lafontaine, Baiwir, Joaquim-Justo, De Pauw, Lemoine, Boulangé-Lecomte, Forget-Leray, Thomé and Gismondi2000; Bowman et al., Reference Bowman, Zhou and Readman2002; Langston et al., Reference Langston, Bryan, Burt and Gibbs2005, Reference Langston, Burt and Chesman2007b).
Batch experiments using natural sediments, and 14C-radiolabelled E2 and NP have been used to establish kinetic behaviour and Kd for these classes of EDC, enabling investigations into the influence of major estuarine variables (salinity, pH, suspended solid loading and sediment type) on sediment–water partitioning behaviour (Langston et al., Reference Langston, Burt and Chesman2007b). Equilibration of both E2 and NP with natural sediments is achieved rapidly – largely within one hour. The molecular structure of the two substrates has a major influence on binding characteristics however, with Kd values for NP (0.2–1 × 104) nearly two orders of magnitude higher than E2 (0.7–1.4 × 102). NP, with its long, linear non-polar (hydrophobic) ‘tail’ will pack around hydrophobic sites with greater density and strength than E2, which must present the hydrophobic regions of the ring structure as close to the sediment surface as possible and as a consequence the binding may not be particularly strong. These substrate binding results indicate, therefore, that E2 is much more hydrophilic, under typical estuarine conditions, than NP. Desorption behaviour for NP appears to be close to being completely reversible whilst a proportion of the sediment binding for E2 appears irreversible. The percentage binding to Avon Estuary surface sediment was 72% and 10% for NP and E2, respectively, though in other sediments proportions may vary (especially for E2), largely as a function of particulate organic matter content (Langston et al., Reference Langston, Burt and Chesman2007b).
Other estuarine variables have subtle implications for particle–water interactions of NP and E2. Of these variables, substrate concentrations appear to have a relatively minor influence on partitioning. In contrast, suspended solids loadings potentially have a significant effect over the range of concentrations of suspended sediment encountered in UK estuaries – characterized by a decrease in Kd values for E2 and NP with increased suspended sediment load. However, the proportion of both E2 and NP bound to the sediment phase increases as a function of the suspended solids concentration, rising to ~25% and ~80% of the total budget, respectively, in conditions typical of turbid estuaries. Based on this evidence the transport of NP is likely to be determined by sediment dynamics to a greater extent than the more hydrophilic E2. Experiments with TBT, likewise, demonstrate the importance of turbidity in determining the fate of TBT (Langston & Pope, Reference Langston and Burt1995).
Partitioning of EDCs is unlikely to be affected by pH in estuarine and coastal waters because of the buffering effect of seawater. Only near to industrial discharges would ambient pHs be likely to diverge significantly from circum-neutral and then differences in partitioning response may occur between classes of EDCs. For the alkylphenol NP an increase in binding to sediment occurs towards both ends of the pH range, implying reduced solubility. For E2, Kd rises gradually with increasing acidity (compared with that under neutral conditions) but decreases slightly with increasing alkalinity, due possibly to ionization of the phenol functional group at elevated pH. The more hydrophobic character of the NP molecule perhaps outweighs any such ionization effects at high pH. The effect of salinity on partitioning of both E2 and NP is typical of the salting-out effect on solubility common to many organic compounds. For NP, Kd values are lowest in fresh water and the binding of NP to the sediment is increased by even small amounts of ionic solutes in the water. E2 displays a more gradual overall trend towards increased Kd with rising salinity. The solubility of both compounds in the aqueous phase will clearly be reduced as a result of saline intrusion in estuaries, albeit to differing degrees.
Thus sediment type (particularly organic content), suspended solids loadings and salinity are likely to be major influences on partitioning behaviour of many EDCs, whilst pH and substrate concentration would not be expected to influence distributions in most estuaries. However, the chemical characteristics of the compounds themselves may account for the biggest variation in behaviour and distribution in estuaries: the alkylphenol NP is dominated by its association with particulates, unlike E2 which is preferentially associated with soluble fractions. This difference between compounds might intuitively be expected to be reflected in relative bioavailability to estuarine organisms and ultimately with their oestrogenic effects. However, whilst it is assumed that compounds which predominate in the dissolved phase, such as E2, are likely to have a stronger tendency for bioaccumulation this has rarely been put to the test. The potential for direct uptake from sediment has thus largely been overlooked, though as described below, it is potentially important in infaunal deposit-feeders.
Bioaccumulation
The relative importance of dissolved and particulate vectors of EDC bioaccumulation has been investigated in the clam S. plana, using water-sediment systems equilibrated (in combination and separately) with 14C-labelled E2 and NP (Langston et al., Reference Langston, Burt and Chesman2007b). Average concentrations in water and sediment were 15 ng l−1 and 69 ng g−1 (dw), respectively for NP and 4 ng l−1 and 7 ng g−1 for E2 in the experiment depicted in Figure 7. Bioaccumulation from water was rapid and the principal source for clams in the short term, with steady-state concentrations of E2 reached after 24 h and NP after 36 h. Bioconcentration factors, relative to water, were 1517 for E2 and 1819 for NP under these conditions. Over the longer term, however, though displaying slower bioaccumulation kinetics, sediments increased in relative importance as sustained sources, particularly for NP. After 12 days exposure in equilibrated systems only 2% of the body burden of NP was accounted for by uptake from water (10% in the case of E2) and there was no indication of steady state in the sediment-exposed clams over this timescale. Clearly, however, bioaccumulation of exogenous EDC in both dissolved and particulate form is to be expected in aquatic invertebrates exposed to contamination in nature.

Fig. 7. Scrobicularia plana. Bioaccumulation of (A) 14C-oestradiol (E2) and (B) 14C-nonylphenol (NP) in individuals exposed via water or labelled sediment.
Mechanistic studies with radiolabelled steroidal hormones (E2, testosterone and progesterone) suggest that the uptake in mussels Mytilus spp. involves metabolism and conjugation of the parent compound to fatty acids, prior to bioaccumulation as highly lipophilic esters (Schwarz et al., Reference Schwartz and Skafar2017a, Reference Schwarz, Katsiadaki, Maskrey and Scott2017b, Reference Schwarz, Katsiadaki, Maskrey and Scott2018). Though addressing the aqueous uptake route only, these results confirm the importance of exogenous sources of steroid hormones and the high accumulation potential of filter-feeding molluscs. As in clams, optimum uptake of dissolved E2 in mussels occurred within 24 h (Schwarz et al., Reference Schwartz and Skafar2017a) and was mostly (80%) present in tissues in lipophilic, esterified form (remainder divided between free- or sulphated- E2, the latter presumably released back to water). Estimated elimination rates for E2 varied between 16% (over 10 days) in static tanks and 63% (20 days) in running seawater, with the largest reduction in the first 5 days, typical of hyperbolic decay (half-life 12.5 days in flow-through conditions).
Given the high rates of uptake of exogenous E2 it is easy to concede that this source may mask any endogenous concentrations, if these exist (Schwarz et al., Reference Schwartz and Skafar2017a). Mussels exposed to tritiated testosterone also demonstrate a very high capacity for uptake, much of which becomes conjugated with fatty acid, notably as 5α-dihydrotestosterone (DHT) and 5α-androstane-3β,17β-diol, but with little (<5%) as the parent compound. Molluscs are known to exhibit 5α-reductase activity – which mediates transformation into DHT (a potent inducer of male secondary sexual characteristics in higher vertebrates). Despite evidence of DHT presence in bivalves in the field, however, it is still not clear if endogenous pathways are involved, or that altered sexual characteristics ensue (Schwarz et al., Reference Schwarz, Katsiadaki, Maskrey and Scott2017b).
The levels of bioaccumulation observed in experiments with clams and mussels (Peck et al., Reference Peck, Gibson, Kortenkamp and Hill2007; Langston et al., Reference Langston, Burt and Chesman2007b; Schwarz et al., Reference Schwarz, Katsiadaki, Maskrey and Scott2018) imply that benthic invertebrates could be susceptible to increases in body burdens of both steroidal and non-steroidal EDCs near sources, hence, could be important vectors to higher members of the food chain. Under typical estuarine conditions (chronic contamination) sediments may represent a particularly important source for bioaccumulation over the lifetime of benthic organisms, given that Kd range from hundreds to thousands for EDCs such as E2 and NP, respectively. Similar findings of sediment-dominated partitioning and bioaccumulation are found in clams exposed to TBT, where ~90% may be derived from the particulate phase in equilibrated systems (Langston & Burt, Reference Lai, Johnson, Scrimshaw and Lester1991). These observations add to the weight of evidence that both steroidal and non-steroidal EDCs derived from various environmental compartments could be linked to disturbances in reproductive processes and altered sex ratios in molluscs and other invertebrates.
Reviewing the evidence from various taxa, Scott (Reference Schwarz, Katsiadaki, Maskrey and Scott2018) concluded that the ability to bioaccumulate steroids (including E2, testosterone and progesterone) was a more likely explanation for their presence and impact in invertebrate tissues than endogenous hormonal involvement in control of reproduction – questioning the possibility of invertebrates acting as surrogates of vertebrate-style endocrine disruption. The significance of steroidal burdens in invertebrates is further confounded by the possibility of transformations within both the organism itself or by other aquatic fauna and flora in the diet, including sediment. Therefore, it is perhaps not surprising that cycling of steroid hormones has only occasionally been observed to track reproductive cycles in invertebrates and evidence of biological activity is at best tenuous (Schwarz et al., Reference Schwarz, Katsiadaki, Maskrey and Scott2018). Apparent absence of the steroid metabolizing enzyme aromatase (catalysing metabolism of T to E2) in invertebrates was cited by Scott (Reference Schwarz, Katsiadaki, Maskrey and Scott2018) as a further reason for uncertainty over biosynthesis and mode of action of ED in invertebrates. However, whilst such mechanistic evidence is sparse and tentative, there are a number of reports suggesting aromatase involvement, and that correlations between steroids and reproductive cycles are not purely coincidental. These include an indication that aromatase activity (transformation of T to E2) may be blocked by TBT in female dogwhelks, leading to the initiation of imposex (Spooner et al., Reference Sonne, Leifsson, Dietz, Born, Letcher, Hyldstrup, Riget, Kirkegaard and Muir1991). However, as already discussed, this is not conclusive, since other possible explanations for imposex – involving RXR receptor or neuropeptide pathways – have also been proposed (Oberdörster & Cheek, Reference Noel, Barrett-Lennard, Guinet, Dangerfield and Ross2001; Horiguchi et al., Reference Horiguchi, Takiguchi, Cho, Kojima, Kaya, Shiraishi, Morita, Hirose and Shimizu2008).
An additional factor in the debate over the nature and significance of the classic steroid–‘ED’ response model is illustrated by Schlenk (Reference Sanchez, Sremski, Piccini, Palluel, Maillot-Maréchal, Betoulle, Jaffal, Aït-Aïssa, Brion, Thybaud, Hinfray and Porcher2008), who showed that in vitro responses (ER-ligand based bioassay) may sometimes underestimate in vivo oestrogenic activity (fish VTG, normalized to oestrogen equivalents) particularly in complex waste situations. Application of TIE fractionation schemes using biological responses to these composite wastes failed to indicate any relationship to steroids (in contrast to simpler domestic waste), supporting the view that mechanisms other than direct ER binding may be important in the feminization of populations in nature, particularly those residing in habitats that receive mixed wastewaters or agricultural effluents. There would seem to be a case for additional mechanisms of disruption to be considered when determining causality of effects such as feminization. Thus, whilst screening assays for EDC based on interaction with ER/AR are rapid (and indicative of potential for hormone disruption), their relevance in terms of whole organism or population response may be uncertain, given the limited understanding of basic endocrinology in many invertebrates.
It is clearly plausible to put forward alternative hypotheses for the mode of action behind invertebrate ‘ED’ other than endogenous steroid pathways and control seen in most vertebrates. Nevertheless, the link between steroidal hormones and ED in invertebrates deserves further consideration (as do numerous other potential agonists and antagonists) in the search for appropriate explanations for reproductive disturbance. Moreover, the OECD has put forward strong ethical arguments recommending that invertebrate models should be sought to complement or replace vertebrate models in experimental testing systems. Invertebrates seem particularly well suited for testing issues such as the diversity and potency of EDC, synergism/antagonism, EDC metabolites and environmental transfer products, and no-effects levels –all of which involve testing with large numbers of individuals.
Experimental studies involving fish, molluscs and crustaceans have, to date, all contributed to our appreciation of the range of possible deleterious effects caused by EDCs. Ultimately, it is their ecological relevance which determines their value in impact assessment programmes. In this context, further examples where disruption to reproductive and development cycles permeate to the population level are discussed in the following section.
Consequences for populations
By definition the most useful models of ED ideally link an endocrine mode of action with a population-relevant deleterious effect. Impacts at the population level are often assumed, based on reproductive endpoints measured at the cellular, tissue or individual level, though in reality these endpoints may not relate directly to population success or result from an endocrine mode of action. The case of tributyltin impacts on neogastropods and, to a lesser extent, bivalves, represents one of the few exceptions in marine systems where field evidence provides the link between impacts at the lower level of biological organization (molecular, cellular, tissue) and population responses (though endocrine involvement remains uncertain). The most convincing evidence of TBT-induced impact on reproduction in marine organisms has involved sensitive molluscan indicators, though a range of other invertebrate populations and communities have undoubtedly been damaged (Matthiessen, Reference Matozzo and Marin2008). TBT has been shown to cause reproductive effects in fish, including testis histopathology, impaired embryo development and biased sex ratios, though at much higher concentrations (>100 ng l−1) than gastropods (Haubruge et al., Reference Hashimoto, Bessho, Hara, Nakamura, Iguchi and Fujita2000; McAllister & Kime, Reference Matthiessen, Wheeler and Weltje2003; Zhang et al., Reference Wang and Croll2009, Reference Zhang, Zuo, He, Cai, Wang, Chen and Wang2011). Reduced sensitivity in fish probably reflects the presence of efficient detoxifying systems in vertebrates and evidence of TBT-induced effects in native fish populations in the field has not emerged. However sex ratio in fish can be affected by a number of EDCs and is almost certainly a population-relevant endpoint (Hazlerigg et al., Reference Haubruge, Petit and Gage2014).
The implications of intersex (ovotestis) for the fecundity of invertebrates is unknown; however, the incidence of affected male S. plana in the field (up to 60%) is sometimes in excess of that observed in feminized fish populations whose reproductive success is compromised (Jobling et al., Reference Jobling and Tyler1998; Jobling & Tyler, Reference Janssen, Lambert, Vethaak and Goos2003; Langston et al., Reference Langston, Burt, Chesman and Vane2007a). It perhaps seems counterintuitive to anticipate population-level consequences in a broadcast spawner with planktotrophic larvae such as S. plana, nevertheless, although the clam is theoretically capable of substantial lateral migration, this may occur only rarely in nature (Casagranda & Boudouresque, Reference Casagranda and Boudouresque2005). Hence, faced with the prospect of elevated concentrations of endocrine disrupting chemicals and ensuing challenges to sexuality, failure to sustain clam populations, due to restriction in gamete/larval viability cannot be ruled out at some sites. Certainly, as depicted in Figure 3A, there is strong evidence that TBT has impacted populations of S. plana, through reductions in recruitment (Langston et al., Reference Langston, Chesman and Vane2015). Wider decline and disappearance of S. plana populations, from undetermined causes, has also been reported from areas in the Danish and German Wadden Sea and the western half of the Dutch Wadden Sea (Essink et al., Reference Essink, Beukema, Coosen, Craeymeersch, Ducrotoy, Michaelis, Robineau, Elliott and Ducrotoy1991). It is not unconceivable, therefore, that populations of deposit-feeding species such as S. plana may be vulnerable to the wider pressure of endocrine disruption – a risk exacerbated by bioaccumulation of EDCs from sediment and meriting further investigation.
In the absence of convincing field data such as that provided by the TBT–mollusc example, models that link laboratory dose-effect relationships with consequences for dynamics of populations are often the best surrogate for predicting ecotoxicological risk. Nevertheless, caution has to be applied in the interpretation of such models given that dose-response relationships used in their derivation may extend beyond ranges which are relevant to wild populations (Marty et al., Reference Lye, Bentley, Clare and Sefton2017). Life history traits and behaviour, and their density-dependence, may also need to be factored in, as indicated in the modelling of the anti-androgenic effects of the pesticide fenitrothion on population dynamics of the stickleback Gasterosteus aculeatus Linnaeus, 1758 (Mintram et al., Reference Miller and Ankley2018) which has freshwater and anadromous forms. Predictions of reductions in population size were parameterized from published data on disruption to male breeding behaviour (courtship, spiggin production and nest building) and suggested that effects were more marked following continuous, as opposed to intermittent, exposure and particularly influenced by altered sex-ratios. The value of these models lies in establishing endpoints which are likely to be most relevant at the population level, and targeting surveillance at the most effective markers.
Ecological hazards have been predicted for invertebrate and vertebrate populations following exposure, experimentally, to a variety of EDC. For example, trenbolone, a synthetic anabolic compound, acts as an androgen agonist and may cause alterations in sexual differentiation in fish, notably altered sex-ratio – favouring males. Treatment of undifferentiated catfish fry (Ictalurus punctatus, Rafinesque, 1818) results in an all-male population incapable of producing viable offspring (Davis et al., Reference Davis, Morrison and Galvez2000). The effect of trenbolone at the population level has also been modelled from laboratory exposures in fathead minnow P. promelas (Miller & Ankley, Reference McCoy, Bortnick, Campbell, Hamlin, Guillette and St Mary2004) and is an example of how such higher-level consequences of EDC can be simulated from robust experimental evidence. Given that it is not known whether ovotestis (and altered sex ratios) observed in crustaceans Echinogammarus marinus (Ford et al., Reference Ford, Fernandes, Robinson, Davies and Read2006) and molluscs S. plana (Langston et al., Reference Langston, Burt, Chesman and Vane2007a) has an effect at the higher level, there is a strong argument for ecological modelling of invertebrate populations exhibiting varying degrees of intersex and testing predicted outcomes against survey data.
The synthetic oestrogen EE2 induces population effects in fish, alongside the lower level responses of vitellogenin induction, altered gonad histology, fertilization success and larval growth. Kidd et al. (Reference Keay and Thornton2007) have shown reduced populations in fathead minnows P. promelas, as a result of feminization, in Canadian lake systems dosed with EE2 in the low ng l−1 range. The fungicide vinclozolin, an anti-androgen, has also been shown to alter male gonad histology and secondary sexual characteristics and to reduce fecundity in P. promelas (US EPA, 2013) though reproductive output was less affected in other species, including Japanese medaka Oryzias latipes (Temminck & Schlegel 1846). Correlative evidence of the effects of PCBs and pesticides (DDE) on fish reproduction is provided by Hansen et al. (Reference Hamza-Chaffai1985) who showed that reduced hatching success in Baltic herring Clupea harengus (Linnaeus, 1758) coincided with elevated levels of these organochlorines in ovaries.
The study of recovery of affected populations in the field, following removal/reduction of EDC is crucial. These studies help understanding of timescales and benefits of pollution control – illustrated most graphically by TBT: the cases of N. lapillus and S. plana confirm it is not necessarily commercial species, or popular test organisms which demonstrate the true ecological significance of EDCs in nature, but rather readily sampled, stationary/territorial invertebrates. Such studies also reveal the nature of the recovery process, whether it is reversal of effects in individuals or immigration from unaffected populations. The relevance of other environmental factors (other contaminants, physicochemical conditions, hydrography, mode of dispersal, proximity to recruitment reserves) may also become evident and be pertinent to the success of remediatory efforts.
Another significant aspect of marine invertebrates lies in their role in the food chain transfer of bioaccumulative and bioactive compounds, including EDCs, to predators. For example, PCB concentrations in a declining population of killer whales Orcina orcus (Linnaeus, 1758) were found to be comparable to, or higher than, those compromising immune activity in populations of other marine apex predators, harbour seals Phoca vitulina Linnaeus, 1758 (Noel et al., Reference Mori, Muramatsu and Nakamura2009). Though causation is difficult to prove, the implication is that bioaccumulating organic contaminants and changes in steroid metabolism represent a threat to endocrine systems in this declining orca population – as with reduced pupping rates in ringed seals Pusa hispida (Schreber, 1775) and other pinniped species (reviewed in Oberdorster & Cheek, Reference Noel, Barrett-Lennard, Guinet, Dangerfield and Ross2001; EEA, 2012), reduced gonadal size and productivity in polar bears Ursus maritimus Phipps, 1774 (Sonne et al., Reference Skelly, Bolden and Dion2006) and declines in otter Lutra lutra (Linnaeus, 1758) populations during the 1960s (attributed to PCBs, dieldrin and mercury accumulated in food; Simpson et al., Reference Segner, Caroll, Fenske, Janssen, Maack, Pascoe, Schäfers, Vandenbergh, Watts and Wenzel2000). Procurement of experimental evidence of ED is impractical in many of these protected species; however, reproductive failure (reduced progesterone-mediated embryo implantation) has been recorded in common seal Phoca vitulina fed PCB-enriched fish (Reijnders, Reference Ram and Sathyanesan1986) and is relevant in the case of other mammals.
Given that trends in marine species and populations at all trophic levels mirror recent declining trends in wildlife biodiversity and abundance in terrestrial ecosystems, there seems a powerful case to consider ED as a possible contributory stressor, alongside the threats from global climate trends, plastics and overfishing.
Conclusions
There is evidence, from the field and in laboratory experiments, to suggest that chemical-induced effects on reproductive systems may occur in marine invertebrates and may be manifested at very low doses. Whilst not many examples have been proven to be endocrine-mediated (unlike some fish species), they are sufficient to raise concerns for biota in contaminated estuarine and coastal areas and promote the case for wider research into health trends in marine life. Despite the lack of basic knowledge concerning the invertebrate endocrine system, the diverse taxonomic groups within this branch of the animal kingdom (particularly marine representatives) offer much in terms of understanding comparative and ecological perspectives surrounding reproductive impairment. Strictly, such damage may not be a direct result of hormone disruption and should, as proposed by Oberdörster & Cheek (Reference Noel, Barrett-Lennard, Guinet, Dangerfield and Ross2000) be ‘considered suggestive, but not conclusive, evidence of endocrine disruption’: in-depth investigation is still required to provide this evidence.
Given that many newly developed chemicals enter the environment with little evaluation at the whole organism level, and considering the physiological diversity of invertebrate taxa, the need for a range of rapid (high-throughput) screening tests, along the lines of those developed to investigate imposex and intersex in molluscs, is evident. These assessments should supplement existing in vitro and in vivo testing protocols adopted by the US EPA and OECD – including those based on interaction with vertebrate nuclear receptors: the latter are most useful in identifying the potential rather than actual consequences for ED (not all in vitro screens translate into in vivo effects). Though mechanisms may not be fully understood yet, invertebrate taxa provide for a series of diverse but relevant endpoints on which to focus more intensive cause-effect investigations into mode of action, severity, sensitive windows of development, reversibility and need for regulatory action. At present many invertebrate groups are under-represented in risk assessment programmes. Tests with invertebrates will help to reduce the number of higher animals currently sacrificed in laboratory testing protocols, but will of course still require close ethical scrutiny.
Wider appreciation of the frequency and severity of suitable responses in nature, coupled with investigation of the mode of action of different classes of chemicals, should involve the use of strategic indicator species such as Scrobicularia plana in order to understand and manage future contaminant threats to the marine environment. The characteristics of intersex (ovotestis) in populations of S. plana and induction following exposure to mixtures of (xeno-)oestrogens appear to be similar to those in fish, where endocrine disruption, and causes thereof, have been established definitively (Tyler & Routledge, Reference Thomas and Budiantara1998; Jobling & Tyler, Reference Janssen, Lambert, Vethaak and Goos2003). Intersex is a relatively widespread phenomenon in S. plana populations, with varying degrees of incidence and severity, which mirrors the extensive and variable nature of ovotestis in freshwater and estuarine fish from UK catchments – and implies that both may be symptomatic of reproductive modification caused by anthropogenic factors. It would be informative to compare the responses in vertebrate and invertebrate indicators more widely across a range of sites.
There is a need for further research on vulnerability and resilience across the lifespan of invertebrates (windows of ED sensitivity), trans-generational effects, comparison of influence on gene expression and epigenetic factors, and synergism/antagonism between EDCs. Field monitoring which links effects at the individual and population levels should be added to this challenging list. Outcomes need to be incorporated into robust procedures, models and regulatory guidelines for the protection of marine habitats which are subjected to mixtures of many potentially harmful chemicals (EEA, 2012). Screening of each may be prohibitive in terms of resources; however, through careful targeting, prevention of the release of deleterious EDCs into water bodies will ultimately be preferable and more cost-effective than subsequent remediation. Such an approach should take into consideration not only the broad spectrum of chemical threats but also the taxonomic diversity of marine invertebrates.
Significant instances of ED in wildlife cannot always be predicted from conventional testing with standard oganisms and may only become apparent after crucial field-based observations identify a problem –as in the case of TBT-impacted molluscs. Despite the limitations of established testing protocols, however, national and international action has proven to be effective in reducing risk from a number of EDCs including TBT, DDT, PCBs and other POPs over the last 20 years, and illustrates how reducing exposures can protect marine life from reproductive disorders. Interestingly, it is the study of recovery of populations – e.g. following the regulation of TBT inputs and reduction in sewage discharge to the marine environment – which is one of the most informative aspects of ED research and is one which deserves more attention in terms of learning how to handle future threats from EDCs. Improved spatial and temporal monitoring in relevant indicators is a particularly important requirement in this context. Given the global spread of contamination, an additional benefit from such surveillance will be the identification of ‘pristine’ locations which represent the true baseline condition – an essential conservation target for the protection of marine life.
Acknowledgements
Support from the Department for the Environment, Food and Rural Affairs (Defra) for research carried out under the EDAQ program (Project CTG0301) is gratefully acknowledged, and thanks are due to Dr Mike Roberts and Dr David Sheahan for their encouragement and helpful suggestions during that time.
Glossary
- Androgen
Male sex hormone that is produced in the testes and responsible for typical male sexual characteristics.
- Anti-androgen
Substance that mimics or blocks the natural male sex hormones.
- Anti-oestrogen
Substance that mimics or blocks the natural female sex hormones.
- Deposit feeder
Feeds on benthic sediment particles.
- Endocrine disrupting chemicals (EDs/EDCs)
Defined as (1) ‘exogenous substances that cause adverse health effects in an intact organism, or its progeny, consequent to changes in endocrine function’ (EU, 1997), or as (2) ‘exogenous agents that interfere with the production, release, transport, metabolism, binding and action or elimination of the natural hormones in the body responsible for the maintenance of homeostasis and regulation of developmental processes’ (US EPA, 1997).
- Gonochoristic
Pertaining to a unisexual individual; remaining as the same sex throughout the life cycle.
- Infauna
Category of organism whose natural habitat is within a soft substrate (sediment).
- Oestrogen
Any of various natural steroids (e.g. oestradiol) that are formed from androgen precursors, that are secreted chiefly by the ovaries, adipose tissue and testes, and that stimulate the development of female secondary sex characteristics and promote the growth and maintenance of the female reproductive system.
- Ovotestis
An ‘intersex’ condition whereby a gonad contains both testicular tubular elements and ovarian follicles.
- Phyto-oestrogen
Natural chemicals found in plants, notably whole grains, fibres and soy products (resorcylic acid, lactones, isoflavones, coumestans, lignans).
- Xeno-oestrogen
Any of various synthetic or semisynthetic steroids (such as ethinyl oestradiol) that mimic the physiological effect of natural oestrogens.