Introduction
The excessive growth of some green macroalgae is termed ‘green tide’ (Fletcher, Reference Fletcher, Schramm and Nienhuis1996). In recent years, coastal China has been widely threatened by green tides. The first report came from the Ulva prolifera green tides in the Yellow Sea (YS), which occurred consecutively for 16 years (2007–2022) and ranked first on scale in the world (Ye et al., Reference Ye, Zhang, Mao, Liang, Xu, Zou, Zhuang and Wang2011; Liu and Zhou, Reference Liu, Zhou, Glibert, Berdalet, Burford, Pitcher and Zhou2018; Hao et al., Reference Hao, Qu, Guan, Zhao, Hou, Tang and Wang2020; Wang et al., Reference Wang, Zhao, Wang, Zhang, Li and Zhang2022). Moreover, it was suggested that the drifting U. prolifera from the YS may expand to the East China Sea, resulting in the green tides in the Gouqi Island since 2011 (Zhang et al., Reference Zhang, Liu, Yang, Song, Ji, Huo, Yu, Xu and He2015a). In the Bohai Sea, U. prolifera has also been reported to cause summer green tides since 2015 (Song et al., Reference Song, Han, Wang and Li2019). The unique features of U. prolifera green tides in the YS, including huge biomass, wide distribution, long-distance transport, have caused severe ecological consequences and wide concerns (Yu et al., Reference Yu, Sun, Yan and Zhou2018; Zhang et al., Reference Zhang, He, Li, Li, Liu, Jiao, Zhang, Huo, Shi and Su2019; Song et al., Reference Song, Kong, Li, Zhao, Yu, Zhou, Jiang and Yan2022; Zheng et al., Reference Zheng, Wu, Cui, Tian, Yang, Zhao, Xue and Liu2022).
The U. prolifera green tide in the YS is a trans-regional disaster. According to satellite monitoring and homology analysis, it was considered that U. prolifera green tides in the YS originated from Subei shoal of Jiangsu province and then floated to Qingdao Sea area. In every spring, small-scale free-floating green algae drifted northwards by seasonal monsoons and surface currents in mid-April to early May. During the migration process, the biomass of U. prolifera increased fast because of the appropriate temperature and nutrient enrichment. In June and July, a large amount of U. prolifera (biomass could reach 106–107 tonnes) drifted northern YS, covering a large area of the Shandong peninsula. After that, green tides declined gradually, with great U. prolifera sink down to the bottom of the YS in August (Keesing et al., Reference Keesing, Liu, Fearns and Garcia2011; Liu et al., Reference Liu, Keesing, He, Wang, Shi and Wang2013; Zhang et al., Reference Zhang, He, Li, Li, Liu, Jiao, Zhang, Huo, Shi and Su2019).
During the formation process of green tides in the YS, with invisible microscopic propagules as well as visible thalli occurring in U. prolifera life cycles (Liu et al., Reference Liu, Keesing, He, Wang, Shi and Wang2013; Li et al., Reference Li, Song, Xiao, Wang, Fu, Zhu, Li, Zhang and Wang2014), U. prolifera has complicated life histories and multiple reproduction modes (Lin et al., Reference Lin, Shen, Wang and Yan2008; Liu et al., Reference Liu, Yu, Yan, Zhang and Zhou2015b). During their life cycles, various life forms at different development stages were observed including microscopic propagules, germlings, mature thalli and decayed thalli. A large quantity of microscopic propagules, including spores, gametes and zygotes are regarded as the simplest stage of U. prolifera (Clayton, Reference Clayton1992; Li et al., Reference Li, Song, Xiao, Wang, Fu, Zhu, Li, Zhang and Wang2014). They inevitably undergo two basic processes, settlement and germination, before developing into multicellular seedlings. With the growing of germinated seedlings, their branch gradually increased and developed into mature thalli. After releasing germ cells, thalli turned white and enter the decay stage. Every stage is critical for the dynamic adjustment of the whole macroalgal life histories, even the occurrence of green tides (Hoffmann and Santelices, Reference Hoffmann and Santelices1991; Lotze et al., Reference Lotze, Schramm, Schories and Worm1999; Santelices et al., Reference Santelices, Aedo and Hoffmann2002).
Green macroalgae including Ulva spp. and microalgae in seawater have a similar habitat, which gives them a chance to interact with each other. In view of the competition between microalgae and U. prolifera, it is well known that green macroalgal inhibit unicellular microalgae, and the effect of microalgae on macroalgae is rarely reported (Jensen, Reference Jensen, Faulkner and Fenical1977; Wang et al., Reference Wang, Wang, Zhou, Sun and Tang2013; Liu et al., Reference Liu, Yan, Yu, Zhang and Zhou2017). Most recent studies have pointed that Ulva thalli could inhibit the growth and reproduction of microalgae in multiple ways, and reduce the diversity and stability of the phytoplankton community, even threaten the formation and succession of microalgal blooms (Tang and Gobler, Reference Tang and Gobler2011; Wang et al., Reference Wang, Yu and Zhou2012; Sun et al., Reference Sun, Wang, Guo, Pu, Yan and Wang2016; Gao et al., Reference Gao, Zhou, Tang, Shi and Su2018). However, both Schonbeck and Norton (Reference Schonbeck and Norton1979) and Huang and Boney (Reference Huang and Boney1983) believed that microalgae can also change the development process of macroalgae by affecting their early microscopic stage. Our previous research also found some microalgae could inhibit settlement of U. prolifera gametes, and subsequent development will be impacted (Liu et al., Reference Liu, Yan, Yu, Zhang and Zhou2017). Since every life stage is critical for the population dynamics of U. prolifera, any variation may eventually influence the population development and the magnitude of green tides. Moreover, U. prolifera is an opportunistic macroalgae, their growth characteristics gradually change during life stages (Clayton, Reference Clayton1992; Lotze et al., Reference Lotze, Schramm, Schories and Worm1999). So what are the competition relations between U. prolifera and microalgae at different life stages? What is the role of these interactions in the occurrence and influence of green tides in the YS? These questions are still poorly understood and need to be answered.
This is the first study to examine the interactions between microalgae and U. prolifera at different life stages. We used five separate co-culture assays to investigate the competition relationship between two microalgae and U. prolifera at settlement, germination, seedling, adult and decay stages under laboratory conditions. Two chosen microalgae Alexandrium tamarense and Prorocentrum donghaiense were all harmful strains, commonly occurring in the coastal waters of China (China Marine Environmental Bulletin, 2001–2021). A. tamarense was bloom-forming microalgae which have caused severe red tides in the YS, Bohai Sea and the East China Sea. It was known for producing paralytic shellfish toxins (PST) in many coastal regions. Although P. donghaiense only trigged large-scale red tides in the East China Sea every year, their special threat to marine environment made us interested in. The main objectives of this study were to provide evidence of any competitive relations between U. prolifera and microalgae, and to evaluate all potential factors affecting the formation and consequences of green tides in detail.
Materials and methods
Sample identification and preparation
The macroalgae U. prolifera were collected from Qingdao Huiquan Bay in July 2014. All strains were identified as U. prolifera by both morphological and molecular analyses. Thalli were washed several times with sterile seawater, gently cleaned with soft brushes and checked under a microscope to ensure that they were free of epiphytes. The treated thalli were controlled in a laboratory environment for several days prior to experiments.
Two microalgae were selected for studying the interactions with U. prolifera. Axenic strains of A. tamarense was isolated from the South China Sea and provided by Jinan University. P. donghaiense was isolated from the East China Sea and provided by the Second Institute of Oceanography. The detailed descriptions of the classification status, particle sizes, bloom densities and toxin-producing features are presented in Table 1. Two strains were cultured to the exponential phase before inoculation in the following experiments.
Table 1. List of microalgal species used in the experiment

PST, paralytic shellfish toxins.
Microalgae and U. prolifera were cultured in f/2 medium, and maintained at 20 ± 1°C, under an irradiance of 50 μmol photons m−2 s−1, with a light:dark cycle of 14:10. Natural seawater from Huiquan Bay, Qingdao was sand-filtered prior to utilization. Prior to each experiment, the seawater was filtered through a 0.45 μm membrane and autoclaved to prepare the culture medium. The salinity of the seawater was 30 ± 1. The average concentrations of NO3−-N and PO43−-P in the seawater were 24.9 ± 2.5 and 0.5 ± 0.08 μmol l−1, respectively.
Obtaining different life forms of U. prolifera at five development stages
According to our laboratory observed results (Liu et al., Reference Liu, Yan, Zhou, Zhang and Lin2015a, Reference Liu, Yan, Yu, Zhang and Zhou2017), the whole life cycle of U. prolifera was divided into five different stages, including the settlement stage (from free-swimming zoids to settled on substratum), germination stage (from settled unicellular zoids to two germinating cells), seedling stage (multicellular germling length of 1 mm or more), adult stage (mature thalli had abundant branches and length reached more than 5 cm) and decay stage (mature thalli turned white after releasing zoids).
To obtain different life forms of U. prolifera for the experiments, released gametes were collected to culture until they developed into different life forms. Gamete formation of U. prolifera was induced by a modified punching method (Dan et al., Reference Dan, Hiraoka, Ohno and Critchley2002). The thalli were cut into 1–2 cm small fragments, and incubated in a Petri dish containing f/2 medium. The medium was replaced every day for 2–3 days until the gametes were released. The initial concentration of U. prolifera gametes used in the experiments was set as 1.0 × 106 cells l–1, same as the microalgae.
Interactions between microalgae and U. prolifera at five different stages
We conducted time-course experiments of U. prolifera in five different stages. At the first day of these five stages, exponentially growing microalgae were inoculated into the beakers and used as treatment groups. Meanwhile, monocultures of U. prolifera and microalgae were used as controls, respectively. Before the experiments, one coverslip (10 × 10 mm2) was placed at the bottom of each beaker to facilitate the counting of attached, germinated gamete numbers and the growth rate of U. prolifera thalli. Each experiment was conducted separately in three beakers, with triplicate samples taken from each beaker for the counts. Only at the settlement stage, the experiments were under dark conditions for 1 day to ensure gametes’ settlement randomly. For the other four stages, experiments were conducted under light conditions for 7 days. To avoid nutrition limitation, 40 μl of f/2 nutrition solution was added at the beginning of the experiment. The concentration of f/2 nutrition solution was 882 μmol l−1 NO3−-N and 36.2 μmol l−1 PO43−-P.
At the settlement stage, numbers of attached gametes and growth inhibition rate of microalgae were used for statistical analysis. After 24 h, attached gametes on the coverslips were removed and counted in 20 fields under a microscope (400× magnification). The fields were taken at approximately 1 mm intervals across the surface of each coverslip from left to right, and the averages were used to calculate the number of settled gametes. Meanwhile, 200 μl of samples in each beaker were pipetted, fixed in Lugol's solution and the density of microalgae counted under a microscope. The growth inhibition rate (%) = (1 − N/N 0) × 100% (where N 0, the cell density of microalgae in the control groups; and N, the cell density of microalgae in the treatment groups).
At the germination stage, the germination rate of U. prolifera and growth inhibition rate of microalgae in each beaker were used for statistical analysis. Every day, the coverslip in each beaker was taken out to count the germination rates (a gamete that divided into two cells was considered the germination standard) and then put back. Meanwhile, 200 μl of samples were pipetted, fixed in Lugol's solution and counted under a microscope. All beakers were shaken twice every day to avoid the microalgae growth adhering to the wall. All samples were preserved in Lugol's solutions for calculating the microalgal cell densities. The germination rate (%) = N/N 0 × 100% (where N 0, the total number of gametes in one field; and N, the germinated gamete numbers in one field).
At the seedling, adult and decay stages, the growth rate of U. prolifera and growth inhibition rate of microalgae were used for statistical analysis. The growth rate (%) = (ln N t − ln N 0)/t × 100% (where N t is the wet weight of U. prolifera at the end of the experiment; N 0 is the wet weight of U. prolifera at the beginning of the experiment and T is the experimental time).
In addition, the nutrients, dissolved oxygen (DO) and pH were measured after every experiment. All samples for nutrient analyses were filtered on GF/F filters, and then an auto analyzer (Quattro, Germany) was used to test the concentrations of NO3−-N, NH4+-N and PO43−-P. The DO and pH of incubated samples were measured with a portable dissolved oxygen meter (JENCO 901, America) and a handheld pH meter (pH211, Italy).
Statistical analysis
The data were analysed using origin 8.5 and SPSS 16.0 software. The data were calculated as the means ± standard deviations (SD) from the different replicated beakers per treatment (n = 3). Statistical differences between the treatments and controls were considered significant at the 0.05 level.
Two statistical methods were used in our experiments. First, the data from settled gametes, germination and growth rates of U. prolifera were analysed using one-way analysis of variance (ANOVA) after testing for normal distribution and homogeneity of variance. For some data, heterogeneity of variances between groups was detected and not corrected by transformation, which were then analysed using non-parametric tests (Kruskal–Wallis one-way ANOVA). If significant differences of the overall ANOVA were found, the post-hoc test (Tukey's test or Dunn's test) were performed to test among the experimental groups. The data from the growth of microalgae at five different stages were examined using independent-samples t test because there were only two experimental groups in each microalgal species.
Results
Effects of microalgae on the settlement, germination and growth of U. prolifera
Figure 1 shows the different effects of two microalgal species on the settlement of U. prolifera gametes. Compared with the controls, the tested microalgae A. tamarense significantly decreased the settled number of gametes (one-way ANOVA, P < 0.05), while P. donghaiense had no significant effects on the settlement of gametes (one-way ANOVA, P > 0.05).

Figure 1. Effects of A. tamarense and P. donghaiense on the settlement of U. prolifera gametes. Data points are means ± SD (n = 3). *P < 0.05 as compared to control.
Gametes in these tested groups were germinated completely within 7 days, and the final germination rates of gametes co-cultured with A. tamarense and P. donghaiense were not significantly different (Figure 2). Although the germination process of gametes seems slightly accelerated in the groups co-cultured with two microalgae from 2 to 5 days, the differences between control and treatments were not significant (one-way ANOVA, P > 0.05).

Figure 2. Effects of A. tamarense and P. donghaiense on the germination of U. prolifera gametes. Data points are means ± SD (n = 3). *P < 0.05 as compared to control.
Figure 3 shows the growth of U. prolifera co-cultured with two microalgae at three different stages. The growth rates of co-culture U. prolifera were less than that of monocultures, but there was no significant difference at these three stages (one-way ANOVA, P > 0.05). In the co-culture groups of A. tamarense, the growth rates of U. prolifera were 94, 98 and 81% at the seedling, adult and decay stages on the 7th day, compared with the control groups. For P. donghaiense, the growth rates of U. prolifera were 97, 93 and 81%, also had no significant growth inhibition relative to the controls.

Figure 3. Effects of A. tamarense and P. donghaiense on the growth of U. prolifera thalli at the seedling, adult and decay stages. Data points are means ± SD (n = 3). *P < 0.05 as compared to control.
For the monocultures of U. prolifera control groups, there were significant differences for U. prolifera incubated at these three stages (including seedling, adult and decay stages) (one-way ANOVA, P < 0.05). In the control groups, the growth rate of U. prolifera at the seedling stage reached 82%, significantly higher than that for the adult stage (10%). In addition, the growth rate of U. prolifera at the decay stage was less than 0, for the decomposition state of thalli.
Effects of U. prolifera on the growth of microalgae at five different stages
Figure 4 shows the growth of A. tamarense and P. donghaiense co-cultured with U. prolifera gametes at the settlement stage. Compared with the control groups, no significant inhibitory or lethal effects of gametes were observed on these two microalgae strains at this stage (t test, P > 0.05). The results suggested that U. prolifera gametes had no significant inhibitory effects on microalgae.
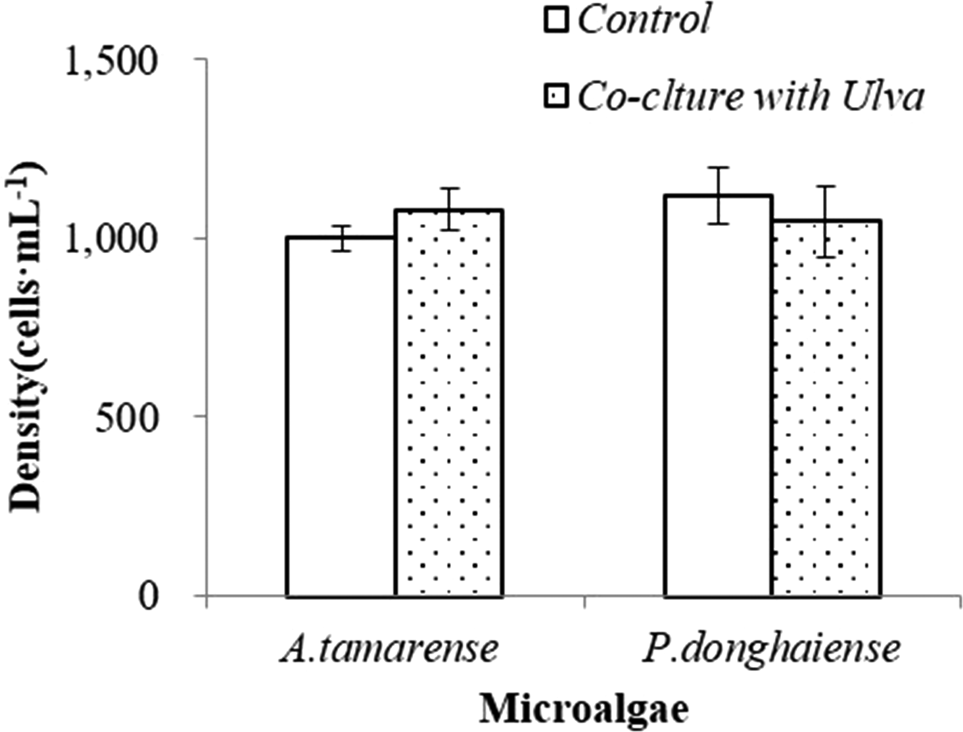
Figure 4. Growth-inhibition effects on microalgae by U. prolifera gametes at the settlement stage. Data points are means ± SD (n = 3). *P < 0.05 as compared to control.
The effects of U. prolifera on the growth of A. tamarense and P. donghaiense at the other stages are shown in Figure 5. Among the four stages of U. prolifera, all treatment groups showed significant inhibitory activity against tested two red tide microalgae, as the strongest inhibition was observed on the decay stage. For A. tamarense, their growths were significantly inhibited when co-cultured with U. prolifera, and the growth inhibition rates reached 19, 30, 78 and 93%, respectively, at the germination, seedling, adult and decay stages (Figure 5a, c, e, g) (t test, P < 0.05). For P. donghaiense, similar negative effects were displayed at these four stages, while growth inhibition exhibited by U. prolifera reached 28, 31, 61 and 100%, respectively (Figure 5b, d, f, h). The cell densities of treatment groups were significantly lower than control groups (t test, P < 0.05).

Figure 5. Growth-inhibition effects on microalgae by U. prolifera at the germination, seedling, adult and decay stages. Data points are means ± SD (n = 3). *P < 0.05 as compared to control.
Changes of environmental factors at five different stages
Changes of DO, pH and nutrients (NO3−-N and PO43−-P) in co-culture systems of A. tamarense and P. donghaiense at five stages are shown in Figure 6. For A. tamarense (Figure 6a), first, DO and pH had similar variation trends. The lowest DO concentration and pH in the seawater both appeared at the decay stage, which have been in the state of low oxygen and acidification. When compared to the normal seawater quality (DO: 8.5 mg l−1, pH: 8.6), the DO and pH decreased by 54 and 10%, respectively. At the other four stages, the DO concentration was higher than the normal level, which is in the saturated state, and pH was also in the normal level. The results indicate that at the decay stage the seawater quality was greatly influenced by algae and even caused hypoxia and acidification. In addition, the nutrient concentrations (NO3−-N and PO43−-P) of A. tamarense co-culture systems all showed the declining trends at five different stages, compared to the initial nutrient levels. Especially at the adult stage, NO3−-N and PO43−-P decreased quickly in the co-culture groups, the uptake rate of NO3−-N by U. prolifera and A. tamarense reached 96%, and PO43−-P were almost depleted on day 7. At the first three stages and the last decay stage, there were still some nutrients that remained throughout the experiment, although the consumption rate of nutrients during the experiments varied with different stages. The uptake rates of NO3−-N were 28, 52, 88 and 14% by day 7, and 35, 49, 91 and 20% for the PO43−-P, respectively, have been removed. Among these four stages, the nutrient consumption was the highest at the seedling stage. The results imply that nutrients were the limiting factors for the growth of algae at the adult stage.

Figure 6. Changes of DO, pH and nutrients (NO3−-N and PO43−-P) in co-culture systems of A. tamarense and P. donghaiense at five stages. Data points are means ± SD (n = 3). *P < 0.05 as compared to control.
In the P. donghaiense treatment groups (Figure 6b), the changes of DO and pH were also consistent with A. tamarense. Only at the decay stage, the concentration of DO and pH decreased by 62 and 9%, obviously below the normal seawater levels. At the settlement, seedling, adult and decay stages, the DO and pH in the co-culture systems all present the normal states. Moreover, the declining trends of nutrient levels (NO3−-N and PO43−-P) were also similar to A. tamarense. Only at the adult stage, the nutrients were almost taken up completely by U. prolifera and the microalgae. The remaining NO3−-N concentrations are extremely low (only 1%), and PO43−-P was fully removed after the 7 day incubation. In other four stages, there are still different degrees of residual nutrients in all incubation groups, so nutrient was not the key factor to interrupt the growth of microalgae among these stages.
Discussion
Possible causes of the observed interactions between microalgae and green algae U. prolifera
This study was the first to analyse the competition relationship between microalgae and bloom-forming U. prolifera at five different growth stages through co-culture experiments. The results showed that the interactions were complex and different at five different stages (Table 2). Only at the settlement stage, one experimental microalgae A. tamarense showed significant inhibition on U. prolifera gametes, while the gametes had no apparent effects on microalgae. However, at the subsequent four stages, the microalgae did not affect the germination and growth of U. prolifera, while the thalli showed significant inhibitory effects on the growth of microalgae. The above results demonstrated that microalgae could only inhibit the naked U. prolifera propagules at the early settlement stage. Since U. prolifera propagules germinated, they began to show strong and non-selective inhibitory effects on microalgae.
Table 2. Competition relationship between microalgae and U. prolifera at five different development stages

‘–’ represents no effect; ‘/’ represents negative effect.
It is well known that the interactions between macroalgae and microalgae are common in the marine environment. Multiple mechanisms were responsible for these negative interactions, mainly including resource competition, allelopathy and alteration of seawater environment (Tang and Gobler, Reference Tang and Gobler2011; Wang et al., Reference Wang, Yu and Zhou2012, Reference Wang, Wang, Zhou, Sun and Tang2013; Sun et al., Reference Sun, Wang, Guo, Pu, Yan and Wang2016). In our study, an interesting finding is that the competition mechanisms between microalgae and U. prolifera at different stages were different.
At the settlement stage, resource competition was first excluded to account for the interactions, because enough nutrients were added before the experiments to avoid the possible nutrient competition. Moreover, other environmental factors (e.g. light, temperature, DO and pH) would not be the limiting factor. Because the experiment at this stage was performed under dark conditions and temperature was controlled in our laboratory. Additionally, DO and pH were still well suited for the growth of algae when they were measured after the experiments. Through the settlement experiment, we found that microalgae A. tamarense significantly impaired Ulva gametes’ settlement. Although harmful algal bloom species A. tamarense could produce PST, the PST did not play a major role in affecting gametes, because there are no differences between the inhibitory effects on gametes by the PST-producing A. tamarense and the non-PST-producing Alexandrium affine under the same experimental conditions. When non-toxic A. affine (at the density of 1.0 × 106 cells l–1) was co-cultured with the gametes, the settled number of gametes was less than 1 cell mm–2, very close to the co-culture with toxic A. tamarense (0 cell mm–2) (Liu et al., Reference Liu, Yan, Zhou, Zhang and Lin2015a, Reference Liu, Yan, Yu, Zhang and Zhou2017). Other chemicals like bioactive compounds may be involved in the interactions. However, we did not test the specific compounds produced by A. tamarense in inhibiting the gametes, for which further elucidation is needed.
The initial inhibitory effects of U. prolifera on microalgae started from the early germination stage. Resource competition was also unlikely to cause this phenomenon. Nutrients, DO and pH were also measured throughout the experiments and were unlikely to become limiting factors. Light competition was also excluded, because the propagules of U. prolifera would hardly reach extreme high densities to cause the shading effects (Amsler et al., Reference Amsler, Reed and Neushul1992). All containers were shaken twice every day to ensure all phytoplankton were suspended in the culture medium to fully utilize the light as much as possible. It seemed that certain metabolites, produced by microscopic propagules of U. prolifera, may be the main factor inhibiting the microalgae. The metabolites were generally thought to cause negative impacts on microalgae by changing the microenvironment (Jensen, Reference Jensen, Faulkner and Fenical1977; Huang and Boney, Reference Huang and Boney1983).
At the seedling stage, U. prolifera also showed significant inhibition on two microalgae, even the toxin-producing species. Chemicals produced by U. prolifera seedlings, instead of resource competition, were responsible for this phenomenon, because the environmental factors like nutrients, DO, pH and light were also suitable for the growth of algae at this stage. In addition, we analysed the relationship between the biomass of U. prolifera and the growth inhibition rate of microalgae at the germination and seedling stages. The average length of U. prolifera was 0.5 and 50 mm, respectively, at these two stages, while the growth inhibition rate on A. tamarense reached 19 and 30%. The inhibition rates of microalgae only increased by 1.5 times, while the total biomass of U. prolifera increased by approximately 100 times. Therefore, the potential threats by U. prolifera propagules cannot be ignored and should be carefully considered.
At the adult stage, U. prolifera thalli displayed stronger inhibition on microalgae, while microalgae still showed no significant negative effect on U. prolifera. By analysing the environmental data, we found that the nutrient uptake characteristics of U. prolifera could be an important factor for reducing the microalgae density. It is well known that U. prolifera exhibit high rates of nutrient uptake; they can rapidly assimilate the organic and inorganic nutrients for growth. This characteristic provided a competitive advantage to U. prolifera, relative to other planktonic algae (Li et al., Reference Li, Zhang, Han, Shi, Rivkin and Legendre2016; Wang et al., Reference Wang, Su, Guo, Yang, Zhang, Zhang, Xu, Shi and Wei2019; Zhang et al., Reference Zhang, He, Li, Li, Liu, Jiao, Zhang, Huo, Shi and Su2019). However, we compared the inhibitory effects of A. tamarense and P. donghaiense with daily addition of enough nutrients under the same experimental conditions, and found the growth of microalgae was just alleviated but still inhibited. Under the sufficient nutrient conditions, the growth inhibition rates of A. tamarense and P. donghaiense were 42 and 44%, obviously lower than the rates under limited nutrient conditions (78 and 61%) (Figure S1). Therefore, we conclude that except for nutrient competition, other mechanisms may be responsible for the adverse role. Recent research studies have shown that mature thalli of U. prolifera could produce some chemicals that reduce the growth of microalgae (Tang and Gobler, Reference Tang and Gobler2011; Wang et al., Reference Wang, Wang, Zhou, Sun and Tang2013; Sun et al., Reference Sun, Wang, Guo, Pu, Yan and Wang2016; Gao et al., Reference Gao, Zhou, Tang, Shi and Su2018). Certain metabolites like polyunsaturated fatty acids were considered to cause negative effects on microalgae, and some other antialgal active substances from macroalgae Ulva were isolated and identified (Sun et al., Reference Sun, Wang, Guo, Pu, Yan and Wang2016). However, whether the mature thalli of U. prolifera secreted the same chemicals as the germlings and seedlings is still unclear. It is necessary to compare and analyse the antialgal activity substances at different stages of U. prolifera in future research.
At the decay stage, U. prolifera displayed the strongest inhibition on microalgae, and the tested red tide microalgae were nearly completely suppressed in the co-cultured environment. The bad seawater quality (hypoxia and acidification) caused by U. prolifera played the main adverse role in microalgae. The uncomfortable environment makes microalgae hardly grow even under the sufficient nutrient conditions. Moreover, our results revealed that the decomposition of U. prolifera release abundant NH4+, which is likely to inhibit the growth of microalgae species. The negative effect of ammonium cannot be ignored, which could cause regional eutrophication and threaten the microalgae (Nelson et al., Reference Nelson, Lee and Smith2003; Wang et al., Reference Wang, Yu and Zhou2012). In addition, substantial amounts of microbial organisms, mainly bacteria, are released after U. prolifera decomposition, which are well known to have negative effects on microalgae (Hardison et al., Reference Hardison, Canuel, Anderson and Veuger2010; Zhang et al., Reference Zhang, Song, Liu, Keesing and Gong2015b).
Potential ecological influences of the interactions in the YS
Our findings may provide some theoretical references for the outbreak reasons of green tides and their following consequences on phytoplankton. The Porphyra culture rafts in Subei shoal are considered to be the initial origin of green tide (Keesing et al., Reference Keesing, Liu, Fearns and Garcia2011; Liu et al., Reference Liu, Keesing, He, Wang, Shi and Wang2013; Hao et al., Reference Hao, Qu, Guan, Zhao, Hou, Tang and Wang2020). Due to the highly turbid seawater, the biomass of phytoplankton is low and few reports on microalgal blooms are available (Kang et al., Reference Kang, Sun, Sun, Xu and Que2013). In addition, large amounts of attached green macroalgae on the rafts are observed, which also inhibit the growth of microalgae based on our research and other reports (Wang et al., Reference Wang, Wang, Zhou, Sun and Tang2013; Sun et al., Reference Sun, Wang, Guo, Pu, Yan and Wang2016). Therefore, the inhibition effect of microalgae on the settlement of U. prolifera is unlikely to exist in Subei shoal, which is beneficial for the growth of U. prolifera. It is a protective mechanism for the early development of green tides, and even promotes the occurrence of green tides. If these ‘seed banks’ of micro-propagules in Subei shoal are not inhibited by microalgae at the initial settlement stage, they will gradually show negative effects on microalgae through various ways. First, the germinated micropropagules in Subei shoal could inhibit or even kill microalgae by secreting certain metabolites. Elimination of competitors would accelerate the growth and development of U. prolifera, which is beneficial for developing into mature thalli. During the subsequent drift process, the biomass of U. prolifera increased rapidly under appropriate environmental conditions, including light, temperature and nutrients. The inhibition effects on microalgae can be further increased by blocking sunlight and absorbing large amounts of nutrients. These results are consistent with reports of dramatic decreases in chlorophyll-a concentrations during the blooming period of U. prolifera green tides (Xing et al., Reference Xing, Hu, Tang, Tian, Tang, Wang, Lou and Gao2015; Sun et al., Reference Sun, Wu, Xing, Song, Zhao, Han and Zhang2018). Even though the environmental conditions were not suitable for U. prolifera in August, decayed U. prolifera thalli still showed strong inhibitory effects on microalgae by altering the seawater environment, such as hypoxia and acidification (Wang et al., Reference Wang, Yu and Zhou2012; Zhang et al., Reference Zhang, He, Li, Li, Liu, Jiao, Zhang, Huo, Shi and Su2019).
In addition, interactions between macroalgae and microalgae play an important role in the succession process of primary producers and even change the occurrence of harmful algal blooms (Smith and Horne, Reference Smith and Horne1988; Sfrifo and Pavoni, Reference Sfrifo and Pavoni1994; Schramm, Reference Schramm1999). Our results are consistent with this conclusion. Large-scale U. prolifera green tides have successively occurred in the YS for 16 years (2007–2022), while the occurrence of red tides is relatively infrequent. The outbreak periods of these harmful algal blooms coincides well with the green tides in the YS (from May to August). According to China Marine Environmental Bulletin (2001–2021), the records of red tides decreased rapidly over the past few years, from nine times in 2001–2005 to four times in 2008–2012. Our experimental results demonstrated U. prolifera can affect the growth of some red tide species in various ways at multiple development stages, which is likely to account for this phenomenon. Similar situations have also been reported that accumulated Ulva decreased the occurrence of microalgal blooms (Smith and Horne, Reference Smith and Horne1988; Sfrifo and Pavoni, Reference Sfrifo and Pavoni1994; Qin et al., Reference Qin, Ji, Song and Xu2011). Therefore, the negative impacts on microalgae by green tides in the YS, may have positive significance in the biological control of red tides in the future.
Conclusion
This paper discussed the competition relationships between U. prolifera and microalgae by five co-culture experiments, and also discussed the possible feedback between green tide and phytoplankton in the YS, China. The main findings are as follows:
(1) One harmful microalgae A. tamarense, only inhibited the naked U. prolifera gametes at the settlement stage. However, PST produced by A. tamarense did not play a major role.
(2) U. prolifera show strong and non-selective inhibitory effects on microalgae early from the germination stage in multiple ways. The negative impacts and influence mechanisms are different for each stage.
(3) The competition relationship may influence the formation of green tides and cause potential ecological influence on phytoplankton in the YS.
Supplementary material
The supplementary material for this article can be found at https://doi.org/10.1017/S0025315423000310
Data availability
Data are available on request from the corresponding author.
Author contributions
Q. L., J. N. L., Z. J. K. and X. J. Z. conceived and planned the experiments. Q. L. and R. F. C. conducted experiments, analysed experimental and statistical data and wrote the manuscripts. J. N. L., Z. J. K. and X. J. Z. supervised and contributed to the final version of the manuscript.
Financial support
This research was financially supported by the Open-end Fund of Jiangsu Key Laboratory of Marine Bioresources and Environment (SH20211206), and the Open Fund of Guangxi Key Laboratory of Marine Disaster in the Beibu Gulf (2020KF03).
Competing interest
The authors declare no conflict of interests.