Introduction
The prevalence of improvised explosive devices (IEDs) during Operations Enduring Freedom, Iraqi Freedom, and New Dawn (OEF/OIF/OND) exposed US military service members to blast injury at a rate not experienced in previous conflicts (Owens et al., Reference Owens, Kragh, Wenke, Macaitis, Wade and Holcomb2008). In theory, blast has the potential to result in brain injury when energy emanating from the explosion is transferred into compressed pressure waves that can pass through tissues within the cranium at a rapid speed (DePalma et al., Reference DePalma, Burris, Champion and Hodgson2005; Ling et al., Reference Ling, Bandak, Armonda, Grant and Ecklund2009). Waves induce particle motion as they pass through tissues with varying densities, such as air-fluid or fluid-solid interfaces, making them particularly vulnerable to blast injury (DePalma et al., Reference DePalma, Burris, Champion and Hodgson2005; Taber et al., Reference Taber, Warden and Hurley2006). Blast waves can also reflect off objects, such as walls, to produce complex wave fields. It is unknown exactly how rapid pressure gradients injure brain tissue, but blast within the context of combat is almost always accompanied by secondary, tertiary, or quaternary effects (Bryden et al., Reference Bryden, Tilghman and Hinds2019). Secondary and tertiary effects involve mechanisms similar to blunt trauma to the brain in that objects and debris thrown by explosions can hit and penetrate the head (secondary effect), or the service member can be thrown a distance by the energy from the explosion to hit hard surfaces (tertiary effect). Quaternary effects include burns, toxic fumes, or effects of other explosive products.
There is some evidence of subtle neural compromise following blast-related traumatic brain injury (TBI). For example, a history of mild TBI (mTBI) sustained within a blast context has been found to be associated with abnormal activation of neural systems involved in response selection and inhibition (Fischer et al., Reference Fischer, Parsons, Durgerian, Reece, Mourany, Lowe, Beall, Koenig, Jones, Newsome, Scheibel, Wilde, Troyanskaya, Merkley, Walker, Levin and Rao2014; Scheibel et al., Reference Scheibel, Newsome, Troyanskaya, Lin, Steinberg, Radaideh and Levin2012) and with resting state connectivity (Gilmore et al., Reference Gilmore, Camchong, Davenport, Nelson, Kardon, Lim and Sponheim2016; Han et al., Reference Han, Mac Donald, Johnson, Barnes, Wierzechowski, Zonies, Oh, Flaherty, Fang, Raichle and Brody2014; Huang et al., Reference Huang, Harrington, Robb Swan, Angeles Quinto, Nichols, Drake, Song, Diwakar, Huang, Risbrough, Dale, Bartsch, Matthews, Huang, Lee and Baker2017; Robinson et al., Reference Robinson, Lindemer, Fonda, Milberg, McGlinchey and Salat2015). Blast has also been associated with white matter microstructural abnormalities (Davenport et al., Reference Davenport, Lim, Armstrong and Sponheim2012; Mac Donald et al., Reference Mac Donald, Barber, Andre, Evans, Panks, Sun, Zalewski, Elizabeth Sanders and Temkin2017; Mac Donald et al., Reference Mac Donald, Johnson, Cooper, Nelson, Werner, Shimony, Snyder, Raichle, Witherow, Fang, Flaherty and Brody2011; Taber et al., Reference Taber, Hurley, Haswell, Rowland, Hurt, Lamar and Morey2015; Yeh et al., Reference Yeh, Wang, Oakes, French, Pan, Graner and Riedy2014) and with cortical thinning (Clark et al., Reference Clark, Merritt, Bigler, Bangen, Werhane, Sorg, Bondi, Schiehser and Delano-Wood2018; Eierud et al., Reference Eierud, Nathan, Bonavia, Ollinger and Riedy2019; Tate et al., Reference Tate, York, Reid, Cooper, Jones, Robin, Kennedy and Lewis2014). Blast intensity specifically has been associated with the number of white matter hyperintensities (Martindale et al., Reference Martindale, Rowland, Shura and Taber2018), though not all studies have found support for structural abnormalities (Davenport et al., Reference Davenport, Lim and Sponheim2015; Levin et al., Reference Levin, Wilde, Troyanskaya, Petersen, Scheibel, Newsome, Radaideh, Wu, Yallampalli, Chu and Li2010). Neuroimaging findings within the cortical region raise the possibility that blast may have physiological effects on the brain, due to varying tissue density, even when other more vulnerable bodily regions escape injury (e.g. lungs, ears).
Despite evidence supporting some physiological sequelae from mTBI within a context of blast, studies have not reliably demonstrated that these sequelae have long-term cognitive consequences. Processing speed and verbal memory deficits have been observed during the acute stage up to seven days after mTBI sustained within a blast context (Kontos et al., Reference Kontos, Elbin, Kotwal, Lutz, Kane, Benson, Forsten and Collins2015; Luethcke et al., Reference Luethcke, Bryan, Morrow and Isler2011), but most studies do not find cognitive deficits after three months of injury (Frencham et al., Reference Frencham, Fox and Maybery2005; Iverson, Reference Iverson2005; Vasterling et al., Reference Vasterling, Brailey, Proctor, Kane, Heeren and Franz2012). A majority of the studies that compare mTBI within a blast context to a non-blast context do not find differences due to the mechanism of injury (Belanger et al., Reference Belanger, Kretzmer, Yoash-Gantz, Pickett and Tupler2009; Cooper et al., Reference Cooper, Chau, Armistead-Jehle, Vanderploeg and Bowles2012; Greer et al., Reference Greer, Sayer, Koeller, Velasquez and Wilt2017; Storzbach et al., Reference Storzbach, O’Neil, Roost, Kowalski, Iverson, Binder, Fann and Huckans2015). Some exceptions have been reported though. For example, Lange and colleagues found that mTBI within a blast context was associated with better cognitive performance compared to mTBI from mechanisms not associated with blast; however, effects were ameliorated after controlling for psychological factors (Lange et al., Reference Lange, Pancholi, Brickell, Sakura, Bhagwat, Merritt and French2012). Martindale and colleagues reported that blast pressure severity exacerbated the effects of mTBI on attention (Martindale et al., Reference Martindale, Ord and Rowland2020).
Several factors may contribute to the heterogeneity of findings following mTBI sustained during OEF/OIF/OND. First, while the majority of these injuries are associated with blast, some involve blunt-force trauma, and studies typically do not separate these mechanisms of injury. Second, service members deployed to OEF/OIF/OND may also engage in activities associated with low-level blast, such as explosive ordnance disposal or the firing of large weapons. Controlled blast may have different effects than blast experienced in an uncontrolled context due to precautions taken during controlled blasts, such as the use of cover to shield service members from the most deleterious effects. It is yet unknown which aspects of blast context are associated with physiological or cognitive sequelae. Early animal studies suggested peak pressure as important (Ling et al., Reference Ling, Bandak, Armonda, Grant and Ecklund2009). Because peak pressure dissipates over distance, some assessment instruments, such as the Boston Assessment of Traumatic Brain Injury Lifetime interview, inquire about distance to blast as a proxy for severity of blast exposure (Fortier et al., Reference Fortier, Amick, Grande, McGlynn, Kenna, Morra, Clark, Milberg and McGlinchey2014). Others suggest that blast pressure, as felt by service members, may be a better measure of severity of TBI sustained in a context of blast during deployment (Martindale et al., Reference Martindale, Ord and Rowland2020). Yet others have proposed estimates of lifetime blast exposure based on a history of use of weapons that create blast pressure (Modica et al., Reference Modica, Egnoto, Statz, Carr and Ahlers2021). Grande and colleagues reported that being within 10 meters of blast was associated with decreased verbal memory, regardless of the presence of mTBI (Grande et al., Reference Grande, Robinson, Radigan, Levin, Fortier, Milberg and McGlinchey2018). It is possible that some characterization of blast can be reliably associated with cognitive sequelae, but the medical community has not yet documented such a relationship.
Another factor that likely contributes to the heterogeneity of cognitive sequelae is the psychological trauma that frequently accompanies combat blast events, including the witnessing of severe injury or death (Hoge et al., Reference Hoge, McGurk, Thomas, Cox, Engel and Castro2008). There is evidence that blast-related mTBI is associated with greater posttraumatic stress symptoms than non-blast mTBI (Kennedy et al., Reference Kennedy, Leal, Lewis, Cullen and Amador2010; Ryan-Gonzalez et al., Reference Ryan-Gonzalez, Kimbrel, Meyer, Gordon, DeBeer, Gulliver, Elliott and Morissette2019) and one meta-analysis found that executive dysfunction is related to posttraumatic stress symptoms (Woon et al., Reference Woon, Farrer, Braman, Mabey and Hedges2017). Other studies have shown that posttraumatic stress disorder (PTSD) explains cognitive deficits in veterans better than mTBI (Hantke et al., Reference Hantke, Adamson, Noda, Lazzeroni, Beaudreau, Yutsis, Fairchild, Kinoshita, Kong, Sheng, Waltzman, Ashford and Yesavage2021; Vasterling et al., Reference Vasterling, Brailey, Proctor, Kane, Heeren and Franz2012). Thus, controlling or accounting for the effects of posttraumatic stress is important in understanding effects of blast exposure on cognitive functioning.
Whether there is a reliable and valid measure of blast overpressure with a long-lasting relationship with cognitive performance is still an open question. This study contributes to this effort by evaluating whether distance to blast has a long-term influence on cognitive outcome following OEF/OIF/OND deployment. Of various estimates of the severity of blast exposure, this study chose distance to blast because there has been at least two reports that blast in close range may have long-term sequelae (Grande et al., Reference Grande, Robinson, Radigan, Levin, Fortier, Milberg and McGlinchey2018; Robinson et al., Reference Robinson, Lindemer, Fonda, Milberg, McGlinchey and Salat2015) and it is a measure available within our dataset. Because the goal is to evaluate effects of distance to blast, we did not limit our analyses to individuals who reported symptoms of mTBI. We seek to replicate Grande and colleagues’ findings (Grande et al., Reference Grande, Robinson, Radigan, Levin, Fortier, Milberg and McGlinchey2018), and therefore hypothesized that those within close proximity of blast would demonstrate decreased verbal memory compared to those not within close proximity of blast. Because deployment to a combat zone can be associated with intense stress that can itself be associated with cognitive inefficiencies, we statistically control for the severity of post-traumatic stress symptoms that may mediate the cognitive effects of blast.
Method
This cross-sectional study included three groups of participants based on a self-reported proximity to the nearest primary blast: 1) close blast group: close proximity to blast with or without symptoms of mTBI; 2) non-close blast group: non-close proximity to blast with or without symptoms of mTBI; and 3) comparison group: no exposure to blast and no symptoms of mTBI.
Participants
Service members and veterans from 18 to 65 years of age with a history of deployment to OEF/OIF/OND were eligible to participate in this study. Participants were recruited from military and Veterans Administration (VA) hospitals in the southern part of the United States using flyers placed in various clinics, clinical referral, and by word-of-mouth. Exclusionary criteria were as follows: history of pre- or post-deployment concussion that required hospitalization; pre-existing neurological disorder associated with cerebral dysfunction and/or cognitive deficit; or pre-existing severe psychiatric disorder including alcohol or drug misuse. The study recruited three groups of participants, a mTBI group, a blast-exposed group without mTBI, and a comparison group. mTBI was defined using VA/Department of Defense (DoD) criteria of: Loss of consciousness less than or equal to 30 min, alteration of consciousness less than or equal to 24 h, or posttraumatic amnesia (PTA) less than or equal to 24 h; and no positive neuroimaging findings. For the mTBI group, the injury must have been sustained on deployment and occurred at least 3 months prior to evaluation. Additionally, they could not have had a history of moderate or severe TBI, and if the only criterion met for mTBI was alteration of consciousness, the duration may not have been less than five minutes. For the blast-exposed group without mTBI, participants must have had a history of exposure to blast without symptoms that meet criteria for mTBI; nor could they have had a history of impairment of consciousness, PTA, or other evidence of intracranial injury. For the comparison group, if they had a non-cranial injury, they must have been more than 3 months post-injury at evaluation; they may not have had a history of impairment of consciousness, PTA, or other evidence of intracranial injury; and they may not have had a history of blast exposure. Our previous work showed no difference between mTBI and the control group (no history of mTBI or exposure to blast) on cognitive outcome (Troyanskaya et al., Reference Troyanskaya, Pastorek, Scheibel, Petersen, McCulloch, Wilde, Henson and Levin2015).
For the purpose of this particular blast-related hypothesis, participants were re-grouped according to their proximity to blast. There are no empirical guidelines on what constitutes close proximity to blast. Grande and colleagues defined close proximity as within 10 meters of blast (Grande et al., Reference Grande, Robinson, Radigan, Levin, Fortier, Milberg and McGlinchey2018). They used the Boston Assessment of Traumatic Brain Injury interview (Fortier et al., Reference Fortier, Amick, Grande, McGlynn, Kenna, Morra, Clark, Milberg and McGlinchey2014), which asked for self-reported distance to blast in the following categories: 0–10, 11–25, 26–100 meters. Our polytrauma interview used the following distance categories: less than 10 feet, 10–30, 30–50, more than 50 feet. To compare our findings to Grande and colleagues’, we defined the close blast group as within 30 feet of a blast (approximately 9 meters) regardless of whether they met criteria for mTBI (n = 47; 39 (83%) met mTBI criteria). Participants who reported having experienced blast that occurred beyond 30 feet away were categorized into the non-close blast group (n = 14; 9 (64%) met mTBI criteria). Those who had never been exposed to blast and had no mTBI were categorized into the comparison group (n = 39). Those who had blunt mTBI with no blast exposure were not included (n = 7). Correspondence between recruited and present group membership is presented in Table 1.
Table 1. Correspondence between present group membership and membership targeted by recruitment
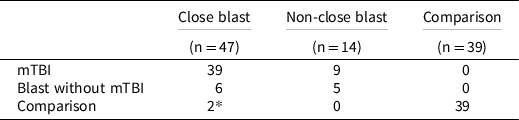
Note:
* Participants were recruited and enrolled into the Comparison group but were later revealed to have had blast exposure.
Injury characteristics and deployment-related information
A semi-structured polytrauma interview was used to assess lifetime history of TBI (including presence and duration of loss of consciousness, alteration of consciousness, and PTA for most severe and most recent TBI) and any extra-cranial deployment-related injuries, number of combat deployments, number of blasts, proximity of the nearest blast, and type of explosive devices (Belanger et al., Reference Belanger, Uomoto and Vanderploeg2009; Troyanskaya et al., Reference Troyanskaya, Pastorek, Scheibel, Petersen, McCulloch, Wilde, Henson and Levin2015). A masters-level study coordinator conducted this interview, and when multiple TBI or blast events were reported, the exposure during deployment that the participant considered most severe was queried as the index event. To corroborate participants’ reports, the study coordinator reviewed available medical records; however, in many instances documentation of the reported injury/event could not be found due to many events occurring during combat and away from medical care. Combat experience was measured using the Combat Exposure Scale, a 7-item self-report measure of wartime stressors rated on a 5-point Likert-like scale (Keane et al., Reference Keane, Fairbank, Caddell, Zimering, Taylor and Mora1989). Scores range from 0 to 41 with higher scores indicative of greater combat stress. Posttraumatic stress symptom severity was measured using the PTSD Checklist, Civilian version (PCL-C), a 17-item self-report measure of symptom severity within the last month that corresponds to the Diagnostic and Statistical Manual of Mental Disorders, Fourth Edition criteria for PTSD (Dobie et al., Reference Dobie, Kivlahan, Maynard, Bush, McFall, Epler and Bradley2002; Weathers et al., Reference Weathers, Litz, Herman, Huska and Keane1993). Each item was rated from 1 to 5 (total score 17–85), with higher scores indicating greater severity of posttraumatic stress symptoms. Post-concussive symptoms from the past two weeks were measured using the Neurobehavioral Symptom Inventory (NSI), a 22-item self-report measure endorsed by the DoD and VA to track neurocognitive complaints after TBI (Cicerone & Kalma, Reference Cicerone and Kalma1995; King et al., Reference King, Donnelly, Donnelly, Dunnam, Warner, Kittleson, Bradshaw, Alt and Meier2012). Each item is rated from 0 to 4 (total score 0–88) with higher scores indicating greater symptom burden. Additionally, depressive symptom severity was measured using the Center for Epidemiological Studies, Depression (CES-D), a 20-item self-report of depressive symptoms in the past week rated on a 4-point Likert-like scale (Radloff, Reference Radloff1977), with the total score ranging 0–60 and higher scores indicating greater symptom severity. Headache impact in the past four weeks was measured using the Headache Impact Test, 6 Items Version (HIT6) (Ware et al., Reference Ware, Bjorner and Kosinski2000). Scores range from 6 to 78, with higher scores indicating greater functional limitation from headache.
Performance and symptom validity
Screening for suboptimal effort was performed using the Word Memory Test (Green, Reference Green2005). The NSI Validity-10 was used to screen for symptom over-reporting (Bodapati et al., Reference Bodapati, Combs, Pastorek, Miller, Troyanskaya, Romesser, Sim and Linck2019; Vanderploeg et al., Reference Vanderploeg, Cooper, Belanger, Donnell, Kennedy, Hopewell and Scott2014). The recommended cut-off scores for both measures were applied (Bodapati et al., Reference Bodapati, Combs, Pastorek, Miller, Troyanskaya, Romesser, Sim and Linck2019; Green, Reference Green2005; Vanderploeg et al., Reference Vanderploeg, Cooper, Belanger, Donnell, Kennedy, Hopewell and Scott2014).
Cognitive measures
Test of Nonverbal Intelligence, 4 th Edition (TONI-4) was used to estimate the level of intelligence pre-injury. On each trial, participants saw a grid with a missing part and chose the most logical missing part from four or six options. Raw scores were converted to standard scores that have a mean of 100 and standard deviation of 15 based on age norms.
Four cognitive domains were evaluated using standardized objective neuropsychological tests, all of which have acceptable to good psychometric properties (Mitrushina et al., Reference Mitrushina, Boone, Razani and D’Elia2005a, Reference Mitrushina, Boone, Razani and D’Elia2005b; Strauss et al., Reference Strauss, Sherman and Spreen2006a, Reference Strauss, Sherman and Spreen2006b, Reference Strauss, Sherman and Spreen2006c; Tombaugh, Reference Tombaugh2006):
Working memory
Auditory consonant trigrams (ACT)
During each trial, the participant heard three letters and a number, counted backwards by counts of three from that number until told to stop, then recalled the three letters heard at the start of the trial. The duration of counting backwards was 9, 18, or 36 seconds, which made up the three delay conditions. The numbers of letters correctly recalled was tallied across five trials for 0–15 points per condition.
Paced Auditory Serial Addition Test (PASAT)
This is a serial addition test where participants heard pre-recorded series of single digit numbers and summed up the two most recent digits prior to the presentation of the next digit. We administered two trials, one at a rate of 3 s per digit and another at a rate of 2 s per digit (Diehr et al., Reference Diehr, Cherner, Wolfson, Miller, Grant, Heaton and Group2003). There were 50 digits on each trial, yielding 0–49 points per trial.
Processing speed
Symbol Digit Modality Test (SDMT)
This test employs a page with rows of symbols with a blank box underneath each symbol. The participant completed the page as quickly as possible by filling in the blank box with the correct number as identified by a row of keys at the top where each unique symbol was associated with a single digit number. The participant wrote on the page during the written condition and called out numbers for the research staff to record during the oral condition. The score was the number of correct responses in 90 s.
Trail Making Test (TMT), Part A
Participants drew a line to connect numbers one through 25 in sequential order. The numbers were randomly placed on an 8.5 × 11-inch piece of paper. If an error was made, staff gave feedback immediately by pointing to the last correct location and asked the participant to continue from that location. The score was the number of seconds required to complete this task.
Verbal learning and memory
Verbal Selective Reminding Test (VSRT)
This list learning test consisted of 12 words presented at a rate of one word every two seconds. During the first trial, participants recalled as many words as possible. Staff then reminded the participant of other words on that list that were not recalled. Then the participant recalled the entire list again (second trial), and the same procedure repeated for a maximum of six trials or until the participant successfully recalled all 12 words on two consecutive trials, at which point any trials not administered were scored as correctly recalled. The Total Recall score was the number of correctly recalled words across six learning trials (0–72). The participant recalled the list again after a 30-minute delay for the Delay Recall score (0–12). Participants were not given notice that there would be a delayed recall.
Cognitive flexibility
Trail Making Test (TMT), Part B
Participants drew a line starting at the number one, connected to the letter A, and alternated between numbers and letters in sequential order through number 13 and letter L, which were all randomly placed on an 8.5 × 11-inch piece of paper. If an error was made, staff gave feedback immediately by pointing to the last correct location and asked the participant to continue from that location. The score was the number of seconds required to complete this task.
Procedures
Procedures were completed in compliance with standards for human research in accordance with the Helsinki Declaration. Participants were enrolled in the study using written informed consent procedures approved by the Institutional Review Board at each recruitment site. They then attended an individual screening visit in which they received a study-specific semi-structured polytrauma interview in which they provided a history of TBI, demographic information, and medical history. Participants also completed performance validity testing at this visit. Those who met all inclusionary and no exclusionary criteria were invited back to complete the remaining procedures. Veterans recruited at the VA hospital were compensated for their time and effort. Service members recruited at the military hospital were not compensated in accordance with the Department of Defense policy against payment for off-duty participation from a Federal source (Kendall, Reference Kendall2011).
Statistical analyses
Background demographic, military, and injury variables were analyzed for differences between the two sites and the three participant groups. Analyses of variance (ANOVA) compared the continuous background variables between the three participant groups, independent samples t-tests compared the continuous background variables between the two sites and chi-square tests compared categorical background variables between groups and sites.
Raw scores from cognitive domains were used as dependent variables instead of standard scores. Standard scores are generally calculated in reference to some demographic characteristics; those who enter military service tend to be men and may not match the civilian sample from which standard scores are based (McGrath et al., Reference McGrath, Linder, Koop, Zimmerman, Ballantyne, Ahrendt and Alberts2020). Thus, raw scores were considered more appropriate dependent variables given that there was a control group against which to interpret findings. Bonferroni correction was applied within each cognitive domain to achieve a family-wise error rate set at p-value of 0.05. For example, a corrected p-value of 0.01 (p of 0.05/5 variables = 0.01) was used to determine significance for the five dependent variables in the working memory category. Background demographic, military, and behavioral health variables that differed between groups (p < 0.05) were entered as covariates when evaluating cognitive differences using analysis of covariance (ANCOVA).
Results
Of the 118 participants recruited, those who did not have documentation of having passed performance validity (n = 10), symptom validity (n = 1), and those who had blunt mTBI without blast exposure (n = 7) were excluded. The remaining 100 participants from the two sites differed in age, marital status and education. The military sample was older (t(98) = 2.15, p = .03), had proportionately more married participants (χ 2(3) = 22.55, p < .01) and proportionately more with postgraduate education (χ 2(7) = 23.75, p < .01) compared to the veteran sample. Demographic characteristics of the participants at each site are shown in the Supplemental Table.
Table 2 shows the three study groups’ demographic and military characteristics. Groups differed on branch of service, gender distribution, education, and estimated preinjury intelligence. These variables were entered as covariates in subsequent analyses. The close blast and the non-close blast groups did not differ on injury characteristics (see Table 2). Notably, all three groups experienced their last deployment an average of seven years prior to evaluation, yielding cognitive functioning data associated with a remote history of deployment experience.
Table 2. Demographic and military characteristics according to group status
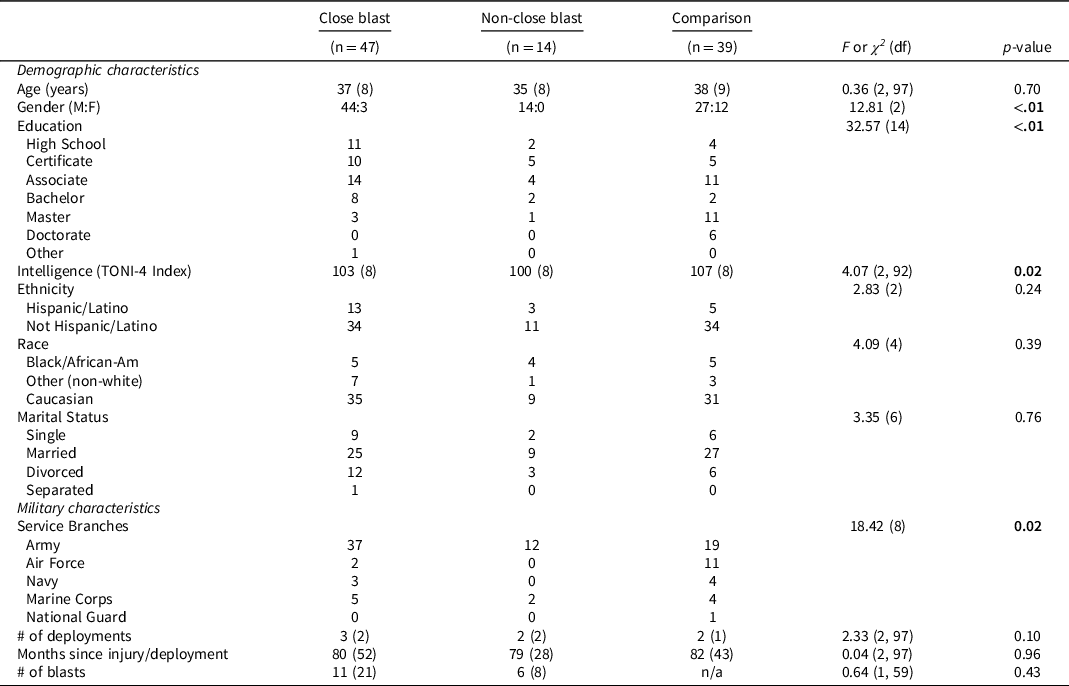
Note: M = male; F = female; TONI-4 = Test of Nonverbal Intelligence, 4th Edition.
Table 3 shows group differences on self-reported symptom and behavioral health measures. Both close blast and non-close blast groups reported more post-concussive symptoms (NSI), traumatic stress symptoms (PCL-C), depressive symptoms (CES-D), and combat exposure relative to the comparison group. The close blast and non-close blast groups did not differ from each other on these measures (p > .05). A different pattern emerged for headaches, in which the close blast group reported more significant impact of headaches than the comparison group only (p < .01). Table 4 shows that the NSI, PCL-C, and CES-D were highly correlated with each other and moderately but significantly correlated with combat exposure and the HIT-6. In analyses evaluating the influence of psychological distress, the PCL-C was chosen as the covariate to control for posttraumatic stress symptom severity; other measures of symptoms and experiences were not included due to these measures’ multicollinearity with the PCL-C.
Table 3. Self-reported symptom and behavioral health questionnaires

Note: NSI = Neurobehavioral Symptom Inventory. PCL-C = PTSD Checklist, Civilian version. CES-D = Center for Epidemiological Studies, Depression. HIT6 = Headache Impact Test, 6 Items Version.
Table 4. Self-report questionnaires (N = 100). Pearson’s correlation coefficients are above the diagonal. P-values are below the diagonal

Group differences on cognitive measures
Figure 1 depicts group differences, and Table 5 shows group differences with and without covariates (branch of service, gender distribution, education, estimated preinjury intelligence, and PCL-C). There are no differences between close blast, non-close blast, and comparison groups on working memory, processing speed, verbal learning, and cognitive flexibility measures that survived Bonferroni correction for family-wise error rate. Findings are the same with and without controlling covariates that differed between groups. Effect sizes of ANOVA models tested, η p 2 , are also reported. For measures that trend towards statistical significance, their corresponding effect sizes are very small.
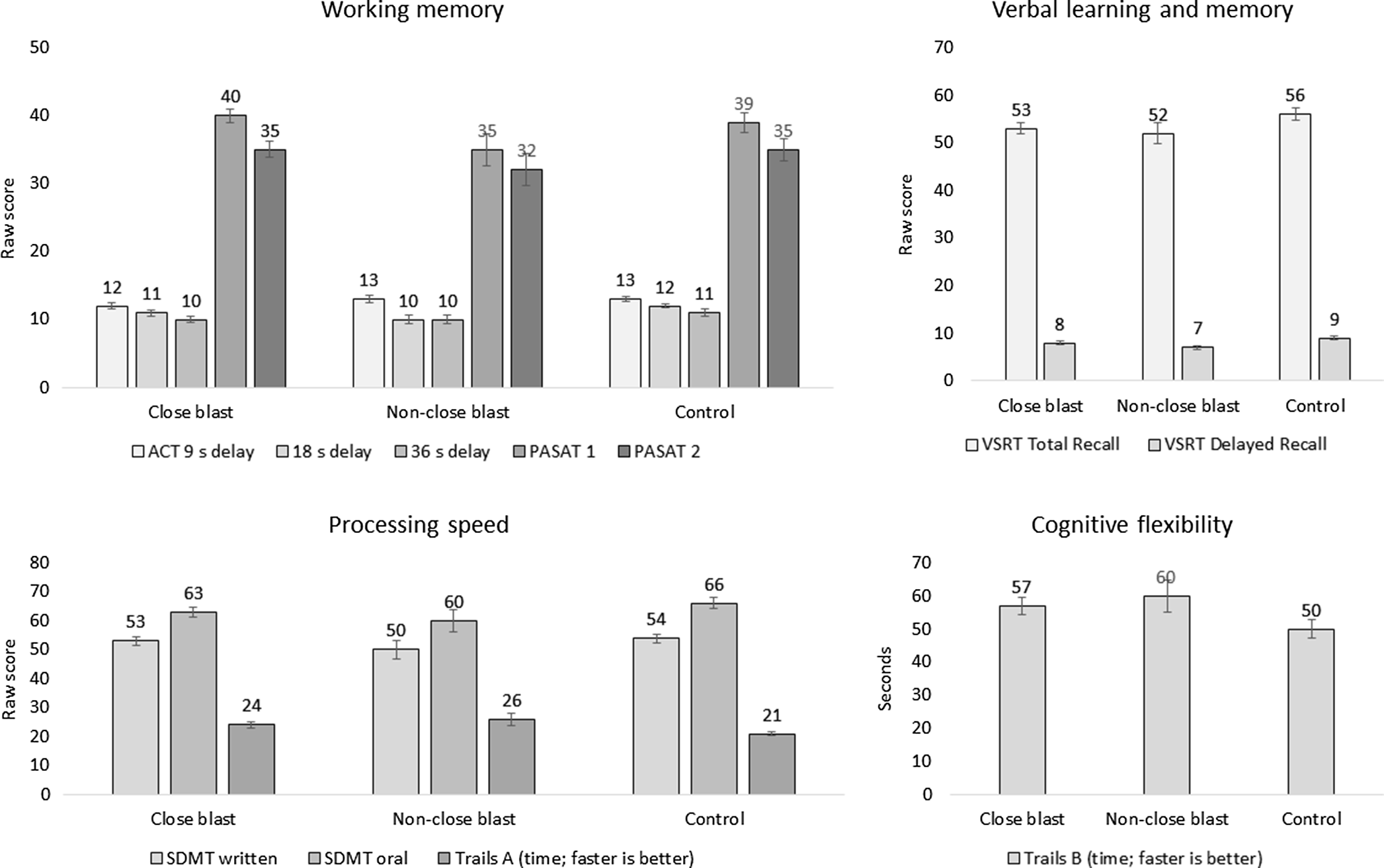
Figure 1. Group differences on measures of cognitive performance. No group difference was significant after controlling for branch of service, gender distribution, education, estimated preinjury intelligence, and PCL-C.
Table 5. Test of group differences on cognitive measures: close-blast vs. non-close-blast vs. control
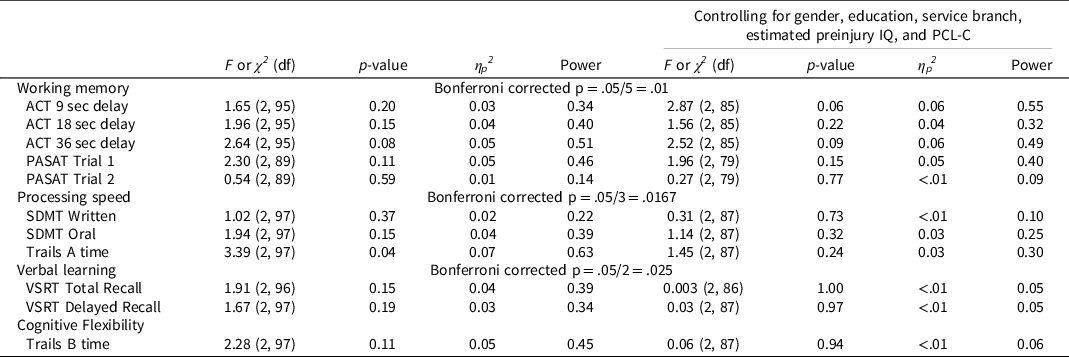
Note: IQ = Test of Nonverbal Intelligence, 4th Edition. PCL-C = PTSD Checklist, Civilian version. ACT = Auditory Consonant Trigrams. PASAT = Paced Auditory Serial Addition Test. SDMT = Symbol Digit Modality Test. Trails = Trail Making Test. VSRT = Verbal Selective Reminding Test.
Discussion
Close proximity to blast, defined as within 30 feet, in a deployed setting was not associated with lower scores within working memory, processing speed, verbal learning, and cognitive flexibility domains. This was also the case after controlling for posttraumatic stress severity and other demographic variables that may have contributed to findings. On measures where there were trends towards significance, effect sizes were very small. Figure 1 shows that performance of the close blast and non-close blast groups was comparable to that of the comparison group and was within the average range.
Previous meta-analyses, which did not account for distance to blast, reported small to moderate effect sizes acutely after injury between mTBI and comparison groups on cognitive domains including working memory, processing speed, executive functioning, and memory (Belanger et al., Reference Belanger, Curtiss, Demery, Lebowitz and Vanderploeg2005; Frencham et al., Reference Frencham, Fox and Maybery2005; Rohling et al., Reference Rohling, Binder, Demakis, Larrabee, Ploetz and Langhinrichsen-Rohling2011; Schretlen & Shapiro, Reference Schretlen and Shapiro2003) that decreased to small or negligible effect sizes beyond three months post injury (Belanger et al., Reference Belanger, Curtiss, Demery, Lebowitz and Vanderploeg2005; Dougan et al., Reference Dougan, Horswill and Geffen2014; Frencham et al., Reference Frencham, Fox and Maybery2005; Rohling et al., Reference Rohling, Binder, Demakis, Larrabee, Ploetz and Langhinrichsen-Rohling2011; Schretlen & Shapiro, Reference Schretlen and Shapiro2003). Our findings, which are based upon a sample approximately 7 years post deployment, are generally consistent with what others have reported in the literature.
In contrast to the study that prompted analyses of our data (Grande et al., Reference Grande, Robinson, Radigan, Levin, Fortier, Milberg and McGlinchey2018), we did not find lower scores in verbal memory associated with exposure to blast at close range. The effect size reported by Grande and colleagues was very small, with approximately 2% of variance in memory performance accounted for by distance to blast exposure after controlling for education (Grande et al., Reference Grande, Robinson, Radigan, Levin, Fortier, Milberg and McGlinchey2018). We did find a comparable effect size (3–4% that decreased to <1% after controlling for posttraumatic stress symptom severity and demographic confounders) albeit statistical significance was not reached, possibly due to a smaller sample size and thus limited statistical power (0.34–0.39) in our study.
Similar to the Grande and colleague’s study, this project examined effects of distance to blast regardless of mTBI status. Most participants in our close blast and non-close blast groups had presented with mTBI, but some did not. Due to the heterogeneity of presentations associated with blast exposure in the military, this classification of cases allowed for examination of blast effects in a way that is not as skewed by sampling bias as might be present in a mTBI sample. Presence of mTBI depends on self-report of symptoms, which may be influenced by multiple factors. For example, in a 2007 sample of National Guard deployed to Afghanistan, 9% self-reported mTBI one month before returning from combat zone but 22% of the same sample self-reported mTBI one year after returning from deployment (Polusny et al., Reference Polusny, Kehle, Nelson, Erbes, Arbisi and Thuras2011). It is unknown whether this discrepancy in self-reported rates reflects ambiguity regarding symptoms, attribution of sources of symptoms, and/or a desire to present oneself as consistent with a warrior ethos. A mTBI sample may reflect biased sampling. Our decision to classify groups irrespective of mTBI symptoms represents an effort to glean a more objective examination of effects of blast.
The experience of a blast event may be associated with traumatic stress if it occurred within a combat environment, but it may not have such an association if the blast occurred within controlled training environments. Both the close blast and non-close blast groups reported significantly more severe posttraumatic symptoms and combat exposure than the control group (Table 3). The general reduction in effect sizes after controlling for posttraumatic symptom severity (Table 5) is consistent with other studies that evaluated the influence of both PTSD and TBI and showed that PTSD seems to have more impact on cognitive performance than TBI (Hantke et al., Reference Hantke, Adamson, Noda, Lazzeroni, Beaudreau, Yutsis, Fairchild, Kinoshita, Kong, Sheng, Waltzman, Ashford and Yesavage2021; Pagulayan et al., Reference Pagulayan, Rau, Madathil, Werhane, Millard, Petrie, Parmenter, Peterson, Sorg, Hendrickson, Mayer, Meabon, Huber, Raskind, Cook and Peskind2018; Vasterling et al., Reference Vasterling, Aslan, Lee, Proctor, Ko, Jacob and Concato2018; Vasterling et al., Reference Vasterling, Brailey, Proctor, Kane, Heeren and Franz2012). Nelson and colleagues used structural equation modeling to elucidate the relationship between blast concussion, PTSD severity, and cognitive performance, and found that only PTSD severity directly impacted cognitive performance (Mattson et al., Reference Mattson, Nelson, Sponheim and Disner2019; Nelson et al., Reference Nelson, Disner, Anderson, Doane, McGuire, Lamberty, Hoelzle and Sponheim2020). Postconcussive blast indirectly impacted cognitive performance, and its effect was mediated by PTSD severity (Nelson et al., Reference Nelson, Disner, Anderson, Doane, McGuire, Lamberty, Hoelzle and Sponheim2020). Thus far this evidence suggests that blast exposure influences cognitive performance through accompanying traumatic stress, and in the absence of traumatic stress, proximity to blast may not convey risk to cognitive sequelae. From the perspective of intervention, these findings suggest that addressing PTSD symptoms may lead to a decrease in cognitive complaints.
This study has several limitations. First, our measure of blast proximity is based on self-report. Recall of events occurring an average of seven years prior may be influenced by factors that affect reconstruction of memory, such as accompanying traumatic events. Second, blast effects on the nervous system may be complex and varied depending on multiple factors, including peak pressure wave severity, accompanying infrasounds, complexity of waves associated with enclosed versus open fields, etc. Distance to blast also does not consider important factors such as whether the context of the blast was controlled, during combat, or whether protective measures were in place. Although we attempted to account for a potential confound by statistically controlling for posttraumatic stress, it remains to be determined by future research what aspects of blast influence cognition and which should be measured and controlled. Third, heterogeneity of our groups (mix of individuals with and without mTBI symptoms after exposure; close blast group had proportionately more participants who met criteria for mTBI than the non-close blast group) presumably increased within-group variability in the outcome measures, which may have contributed to null group effects. Ideally a longitudinal design that measures cognitive performance before injury and tracks service members through deployment and/or blast exposure would be necessary to answer whether blast characteristics are associated with cognitive change. Finally, the non-close blast group had a very small sample size, which contributed to low statistical power. Despite these limitations, our sample characteristics reflected the cohort of service members who were deployed to OEF/OIF/OND, and for this cohort, close exposure to blast rendered no greater remote cognitive risk than farther blast exposure.
Supplementary material
To view supplementary material for this article, please visit https://doi.org/10.1017/S1355617722000558
Funding statement
The views expressed in this manuscript are those of the authors and do not necessarily represent the official policy or position of the Defense Health Agency, Department of Defense, Department of Veterans Affairs, or any other U.S. government agency. This material is based upon work supported in part by United States (U.S.) Department of Veterans Affairs Merit Review Awards Numbers [I01 CX001820] (PI Scheibel, RS) and [O1062-I] (PI-Scheibel RS). It was prepared under Contract HT0014-21-C-0012 with DHA Contracting Office (CO-NCR) HT0014 and, therefore, is defined as U.S. Government work under Title 17 U.S.C.§101. For more information, please contact [email protected]. UNCLASSIFIED.
Conflicts of interest
None.