1. INTRODUCTION
The European Union (EU) Horizon2020 project Standardisation of Global Navigation Satellite System (GNSS) Threat Reporting and Receiver Testing through International Knowledge Exchange, Experimentation and Exploitation (STRIKE3) (EU H2020 project STRIKE3, 2017; Dumville et al., Reference Dumville, Pattinson, Ying, Bhuiyan, Gabrielsson, Waern, Pölöskey, Hill, Shivaramaiah, Kibe, Manikundalam, Lee and Reyes Gonzalez2016) is a new European initiative to support the increasing use of GNSS within safety, security, governmental and regulated applications. One of the objectives of STRIKE3 is the deployment and operation of an international GNSS interference monitoring network (based on the threat monitoring and reporting system described here) to capture the scale and dynamics of the problem, and to work with international GNSS partners to develop, negotiate, promote and implement standards for threat reporting and receiver testing. Both standards are missing across all civil application domains and are considered a barrier to the wider adoption and success of GNSS in the higher value markets.
The STRIKE3 Consortium brings together competences from GNSS research and development technology, GNSS within transportation, GNSS testing, and GNSS interference. The partners include Nottingham Scientific Limited (UK), the Swedish Defence Research Agency (Sweden), the Finnish Geospatial Research Institute of the National Land Survey (Finland), the Automotive and Rail Innovation Center (Germany), GNSS Labs (India), and the Electronics and Telecommunications Research Institute (South Korea). The project duration is 36 months (February 2016 to February 2019).
The problem of radio frequency interference in the GNSS frequency bands affects diverse applications, from the more traditional such as truck tolling, determining road tax, maritime vessel monitoring, and offender tracking, to the more recent such as automated vehicle navigation, integrity-intensive airplane landing procedures, and Pokemon Go! Likely causes of threats to GNSS include unintentional threats, intentional (incidental and/or malicious) threats, and threats due to natural disturbances. To understand the level of threats, and to develop effective countermeasures, it is highly desirable to monitor for interference in a systematic way and to share the results with interested stakeholders.
The drive towards GNSS threat information standardisation was initiated by the International Committee on GNSS of the United Nations (Zhen, Reference Zhen2012). It recommended standardising, sharing and disseminating interference information through standardisation of user information, interference event information and interference source information and by forming regional and national interference databases reporting finally to a global database after filtering and validation. In Giraud et al. (Reference Giraud, Mathieu, Boyero Garrido and Fernandez Hernandez2013) the European Telecommunications Standards Institute's (ETSI's) Technical Committee on Satellite Earth Stations and Systems (TC SES) elaborates a firm and common standard for GNSS-based location systems. (ETSI, 2012) discusses more closely the GNSS-based applications and standardisation needs. The Resilient Navigation and Timing Foundation (RNTF, 2017) urges the development of standards for jam-resistant receivers to include Advanced Receiver Autonomous Integrity Monitoring (ARAIM) and RAIM to protect, toughen and augment GNSS. Additionally, it discusses the ‘PTA’ (Protect, Toughen and Augment) approach which also underpins the case for reporting and receiver standards. Lastly, Section 10 (Open Service performance standards) of the International Committee on GNSS's (ICG's) Report of the Systems, Signals and Services Working Group (ICG, 2015) discusses the compatibility and spectrum protection issues of GNSS. It recommends the development of standards for interference reports submitted to GNSS Civil Service National Centres and establish routine communications among the centres and to develop standards for interference detection module capabilities to be implemented by national governments and industry.
There are a number of different types of detection equipment that can be used to detect GNSS interference, and there are previous and existing projects and monitoring campaigns to try to detect interference. In general, the different threat monitoring systems can be divided into two categories; fixed and portable. Fixed monitoring systems are installed at a site or a platform. After installation, the system is intended to operate over a long time period. This type of a monitoring system could also be expanded into a monitoring programme consisting of several nodes that are interconnected to a central server. Portable monitoring systems are typically handheld devices. These devices do not need to be installed, and are available ‘off-the-shelf’. Their size is smaller than the fixed systems and they can be placed, for example, in a moving vehicle.
However, although these types of local monitoring efforts can be effective at monitoring and protecting a specific site or local area, the ability to combine results from different detection equipment and monitoring networks and gain a wider understanding of the level of threat is limited for several reasons. Firstly, different detection equipment and monitoring networks report different values and statistics about interference events and so it is not always easy to combine results. Secondly, different types of detection equipment have different detection algorithms and thresholds as they are designed for different purposes, and so different types of detection equipment installed at the same site may report completely different numbers of events.
The goal of this paper is to propose a system architecture and draft reporting standard that can enable the results from different types of detection equipment and monitoring networks to be reported in a common format and combined in common analysis. Such a system could be very valuable in monitoring the level of threat posed by GNSS interference over large areas and to see how the threat changes over time by combining data from many different types of monitoring networks. This paper is further motivated by the fact that recent publications seldom provide an overall big-picture of the diverse deployed and commercially available solutions for GNSS threat monitoring and reporting and their associated reporting messages. Therefore, this paper hopes to consolidate existing literature and to provide an authoritative reference guide to future researchers regarding the evolution of this domain.
The paper is arranged as follows: Section 2 presents a comparative analysis of the existing threat monitoring systems. A similar comparative study of existing standardised threat reporting messages is presented in Section 3. Building on the results of these studies, the STRIKE3 project has developed proposals for a standardised reporting message and an integrated threat monitoring demonstration system, which are described in Sections 4 and 5 respectively. The paper concludes with a recapitulation of the main issues and outlook within the STRIKE3 project.
2. ANALYSIS OF EXISTING GNSS THREAT MONITORING SYSTEMS
This section presents a comparative study of some of the identified existing systems, including initiatives at national and international levels, for detection, characterisation, and monitoring of GNSS threats. Table 1 shows a summary of this comparison which is based on the capabilities of the threat monitoring systems; especially if they are also capable of classifying the interference type, if they support detection of signal spoofing, and if they are capable of geo-localisation of the interference source.
Table 1. Overview of existing GNSS threat monitoring systems.
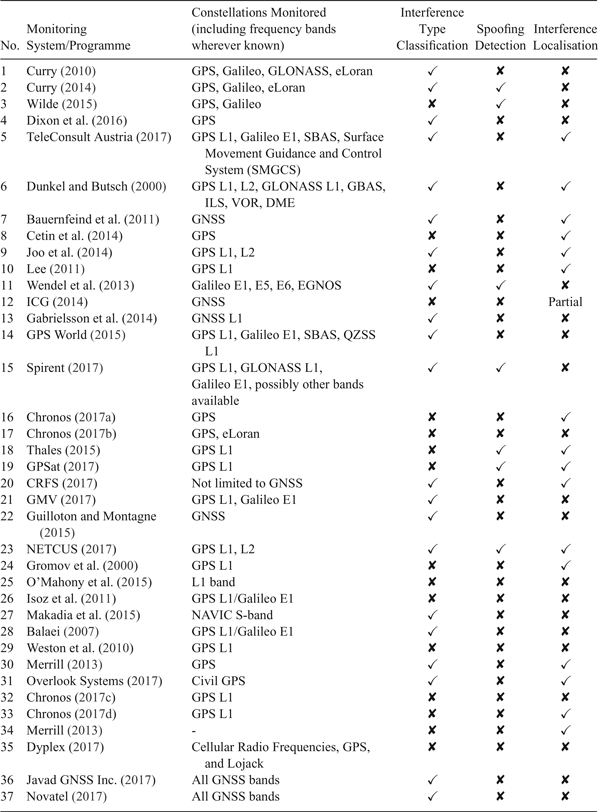
2.1. Summary of Findings from Study of Deployed Threat Monitoring Systems
This summary is based on the in-depth study of the deployed threat monitoring systems listed in Table 1. It provides further information about some of the defining characteristics of these systems. Special emphasis is placed on highlighting aspects which are common to a number of systems (possibly indicating shared best practices) or aspects which are unique to certain systems (possibly indicating an innovation worth replicating).
A number of traditional schemes adopt familiar network architecture - essentially a set of distributed sensor nodes installed at critical infrastructures, and connected to a central operational/processing/command unit via a communication network. The sensor nodes sense the local environment to determine if a radio interference event is currently underway. They do not necessarily contain substantial local storage and some nodes can operate on battery power. Communication with the central unit is usually via one or more of the following diverse schemes: 3G/4G, Very Small Aperture Terminal (VSAT), Ethernet, Virtual Private Network (VPN), Short Messages (SMS), Wireless Local Area Network (WLAN), etc. The central unit usually has a graphical user interface for operator interaction which can generate alerts if an interference event is identified. The control unit is also capable of remotely controlling the sensor nodes.
Various parameters related to the receiver performance and the interference are monitored at the sensor nodes: interference type/frequency spectrum shape, time domain signal structure of the interferer, direction of origin of the interference source, coarse geo-location of the interference source, power profile, carrier to noise ratio, noise signal profile, receiver quality of service, receiver signal integrity, reliability, continuity, accuracy, receiver trustworthiness in presence of jamming, etc. Sensors can monitor different frequency bands from L1 only to Global Positioning System (GPS)/Galileo L1, L2, L5, E6, GLONASS L1, Navigation with Indian Constellation (NAVIC) S-band, eLoran, Instrument Landing System (ILS), VHF Omni Directional Radio Range (VOR), Distance Measuring Equipment (DME), with narrowband and wideband analysis.
Algorithms for interference detection include Signal to Noise Ratio (SNR) mask/ threshold (for example, Curry (Reference Curry2014), Dunkel and Butsch (Reference Dunkel and Butsch2000)), Fast Fourier Transform (FFT)-based (for example, Curry (Reference Curry2014)), and Automatic Gain Control (AGC)-based (Isoz et al., Reference Isoz, Akos, Lindgren, Sun and Jan2011; Bauernfeind et al., Reference Bauernfeind, Ayaz and Eissfeller2011) algorithms.
Most monitoring systems focus on low cost commercially available jammers. In general, the monitoring programs and systems can detect the presence of any interference type in the frequency band of interest. The following is a list of the different interference regimes that have been detected: unintentional interferences such as harmonic and spectrum leakages from adjacent channels, Amplitude Modulation (AM)/Frequency Modulation (FM) channels, Orthogonal Frequency-Division Multiplexing (OFDM) channels, LightSquared satellite communications transmissions, atmospheric (ionospheric and tropospheric) effects, and interference from leaked signals originating from pseudolites installed inside built-up areas. Types of intentional interference from jammers include coherent continuous wave, chirp signals with sinusoidal, triangular, and saw-tooth patterns, pulsed coherent continuous wave format, noise signals with low and wide bandwidth and pulsed noise format with low and wide bandwidths.
A number of monitoring systems use commercially available off-the-shelf components and software and firmware which are freely available or open-source. The overall cost can be maintained at less than €100. Such systems are easy to assemble without any particular technical expertise, no special additional equipment is necessary and the installation is straightforward with no requirement for pre-survey or on-site calibration. Increasingly, software defined radio receivers and digital signal processor platforms are being used in such monitoring systems.
The optimum location for installation of the sensor nodes depends on the objective of the monitoring network and the critical infrastructure under surveillance. A sensor node can also be made mobile by installation inside a van with multiple antennae to monitor diverse signal bands and direction-finding for locating interference sources. Mobile sensors can also be mounted in airborne vehicles linked to ground stations via Radio Frequency (RF) data links.
The spatial range of threat monitoring activities can extend from small areas to large, even nation-wide regions. A wide spatial range threat monitoring system can be developed using national Continuously Operated Reference Station (CORS) networks. The number of CORS stations witnessing the loss of lock helps to estimate the geographical extent of the interference event. Crowdsourcing techniques, which allow for mass market personal navigation devices to sense the environment and relay information autonomously and anonymously to authorities are becoming increasingly attractive due to the possibility of implementing a denser interference monitoring network over a flexible area without the need for separate investment in deployed infrastructure.
3. ANALYSIS OF EXISTING GNSS THREAT REPORTING MESSAGES AND STANDARDISATION ACTIVITIES
3.1. Existing Threat Reporting Standards
Table 2 presents a comparison of the interference reporting formats recommended by four national and international organisations: the US Coast Guard (Navigation Center, 2017), the International Telecommunication Union (ITU, 2017), the Chinese delegation to the ICG Working Group A meeting (Zhen and Zhao, Reference Zhen and Zhao2013), and by Korean researchers (reference withheld due to security considerations) engaged with the study of GNSS interference. The first column lists all possible information categories and information fields as found in the different references. This includes information of the GNSS user, GNSS anomaly that was experienced, interference source, and analysis of interference and actions. The ✓/✘ under each reference denotes if that particular information field is available in the format recommended by that reference.
Table 2. Comparison of existing threat reporting standards.

3.2. Summary of Findings from Review of Standardisation Activities Related to GNSS Threat Reporting
GNSS interference can be classed as a type of-cyber security event and parallels can be drawn between it and more ‘conventional’ security threats afflicting computer systems. Therefore, there is wide support for mitigating actions to be taken to counter this threat. Central to these efforts is the development of a truly global standard for the reporting of interference events, which also directly supports receiver testing standards. The future course of action therefore, should be focused in the following directions:
• To develop a common and efficient reporting standard through evolution of existing regional standards,
• To ensure flexibility in standards to handle evolving threats,
• To implement reporting datasets which directly support receiver testing standards,
• To develop testing standards that evaluate in a standardised manner receiver performance during marginal and denied GNSS conditions,
• To identifying key performance indices related to GNSS threats which translate readily into user requirements.
4. PROPOSED STANDARD THREAT REPORTING MESSAGE
The purpose of the proposed reporting message is to share information about the detected jamming events within an interference monitoring network to a centralised server in near-real time or in periodic batches, for example, once a month. Sharing some estimated metrics or detailed information about the interference event within a wider community might be sensitive for the contributing organisation and possibly raise costs. Therefore, privacy, data security aspects and motivational factors to convince them to contribute have been duly considered.
With regards to incentives for network operators to contribute to the proposed system using the standard threat reporting message, several possibilities exist. One incentive is the opportunity to pool knowledge and increase understanding about threats to ultimately help mitigate them. For example, if several different airports with their own monitoring networks shared information through standardised reporting it might help to identify common types of events and give further clues as to the likely cause. Also, the sharing of selected information in this way provides a good opportunity for monitoring network operators and/or monitoring equipment manufacturers to advertise their other services. Sharing limited information through these reporting standards is useful in itself but also acts to advertise an organisations’ capabilities, and gives the opportunity for users to contact the data providers to procure additional data and/or services, thus helping to offset the additional costs of standardisation.
The reporting message consists of two types of data; mandatory information and optional information. Mandatory information is designed to be non-sensitive information that can be shared by all contributors. Information that can potentially be sensitive is left in the optional section of the reporting message. Each contributing monitoring network will most likely have its own definition of what constitutes an interference event. Therefore, it is necessary to standardise this definition before interference event data can be shared with the central server. This will also enable the performance of reliable statistical and trend analysis on the shared data.
4.1. Event Message Definition
The contents of the proposed threat reporting message are described in Table 3. The fields of the mandatory section are so designed to allow the contributor to decide on the level of detail that it is comfortable sharing with the wider user community. In the optional part of the message, more detailed information about the detected event may be provided. This would eventually make it possible to have a deeper analysis of the interference event.
Table 3. Description of the information shared for each detected event.

4.2. Event Definition
To be able to compare results and statistics from different interference monitoring networks, it is important to have a common definition of what an interference event is. However, even if the criteria for an event are well defined, it is at the end the sensitivity of the detection system that defines when the event is detected. Table 4 provides descriptions on two standard event definitions proposed by the STRIKE3 project. Event ‘Type a’ is intended for interference detection equipment that is capable of measuring received power or GNSS-receivers that provide Automatic Gain Control (AGC) information. Event ‘Type b’ is intended to be used by detection equipment that is based on the carrier-to-noise ratio measurement by the internal GNSS receivers, e.g. in CORS networks.
Table 4. Description of two standard event definitions.

4.3. Justification for the Proposed Approach
The threshold for events ‘Type a’ and ‘Type b’ are chosen so that the reported event most likely will affect the performance of the internal GNSS receiver negatively. This does not exclude other possible event definitions. This is especially true in a situation where the distance to the threat source is so great that the energy reaching the monitoring system falls below the threshold stated in the event definition. To generalise, this situation will arise each time the victim receiver and the monitoring system are not co-located.
Both event definitions have the drawback that they are relative to the noise power at the corresponding site and that their performance is dependent on the type of GNSS receiver used in the monitoring station. The advantage is that they are quite straightforward to implement in diverse types of detection equipment. A more sophisticated definition could in the future be based on correlation of received signals to a threat database built from collating prior experiences of threat signatures. In this case, the received waveform characteristics are correlated with characteristics of known interference sources pre-recorded in the database. This will allow for a more realistic definition of a standard event definition. As the capabilities and performance of threat monitoring equipment evolve, additional event definitions can be integrated into the reporting standards.
5. PROPOSED INTEGRATED THREAT MONITORING SYSTEM
5.1. Overview
The purpose of the standardised threat report message and event definition is to facilitate the integration of existing threat monitoring systems into an international threat monitoring network with a central database to collate the threats that are encountered by the constituent systems. The overall system concept to implement this approach consists of two main elements:
• Sensors (for detecting interference and reporting events),
• Centralised server (for collating reports from the different sensors in a centralised database and providing access to the results for end-users).
As shown in Figure 1, the sensors are operated independently of the centralised server. It is the intention to allow different types of detection equipment from different manufacturers to be used for interference monitoring, and to enable already deployed sensors and monitoring networks to contribute to the centralised database, as well as new installations. The centralised server will act as a central hub to collect results from different sensors deployed in a variety of monitoring networks, and allow end-users to view information about the events and generate statistics.
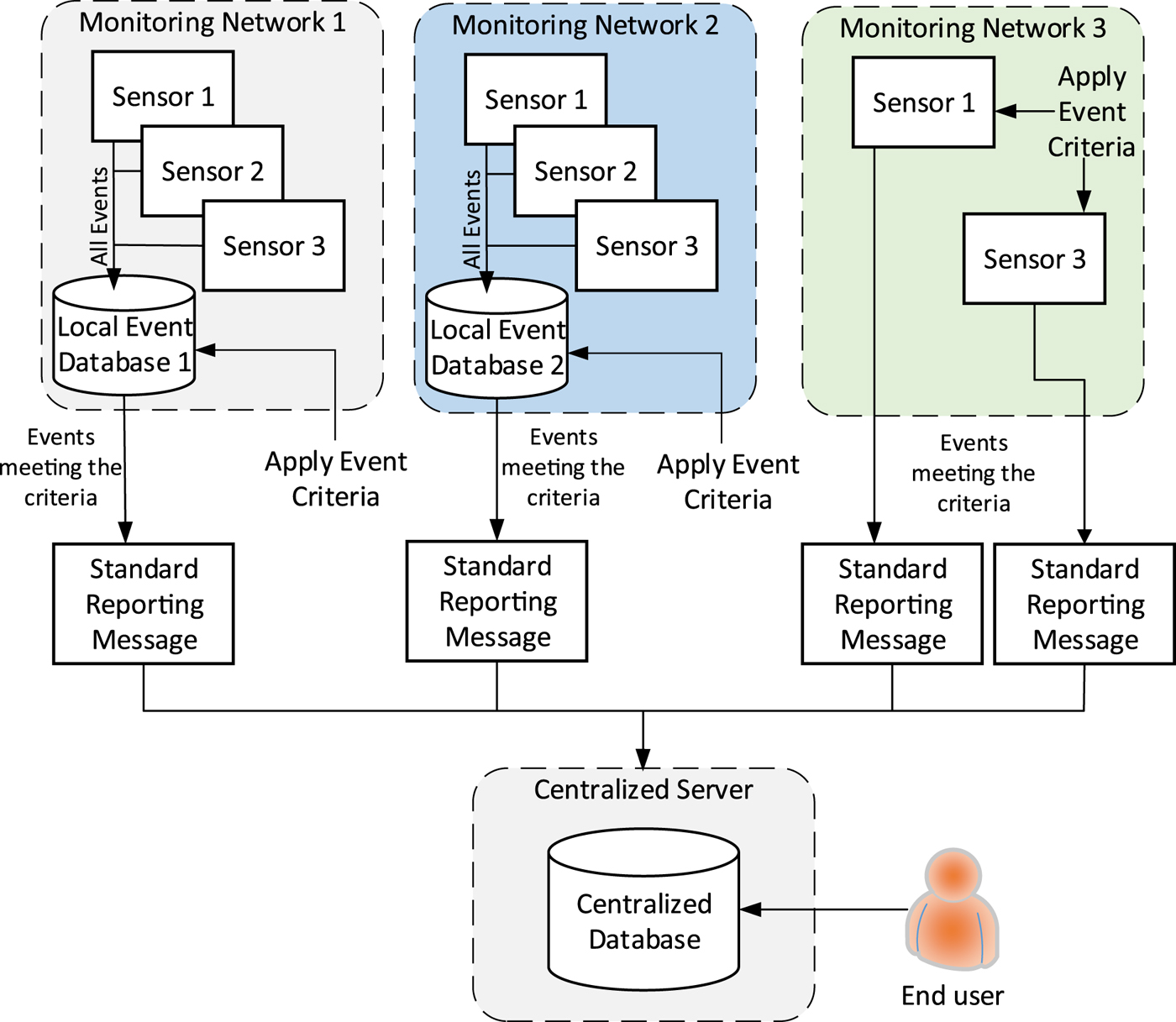
Figure 1. Overview of STRIKE3 threat monitoring and reporting system concept.
The logic of this approach is as follows:
• Sensors will be used to detect interference events. The sensors may be deployed in a monitoring network where they report to their own local event database or the sensors may store data locally at the sensor;
• Only high-level information about interference events that are detected by the sensors will be provided to the centralised server for storage in the centralised database following the proposed standards.
• The events detected by the detection equipment at the sensors must be verified against standard event criteria as a pre-filtering step. This pre-filtering can be done either at a local network database (as in ‘Monitoring Network 1’) or at the sensor itself (as in ‘Monitoring Network 3’);
• Those events that meet the event definition criteria must be formatted according to the reporting standard and provided to the centralised server;
• A minimum set of mandatory information is defined for all events;
• Optional fields are also available to allow organisations to provide additional information that is interesting for more detailed analysis if so desired;
• It is foreseen that contributing organisations will need to register before they can contribute to the centralised database.
• An interface will be available to allow end-users to access the information in the centralised database in order to view the information about events and perform some simple analysis.
• Possible analysis will allow an overview of the global threat situation and change of threat level over time;
• This provides a mechanism for end-users to obtain additional detailed information about certain events from the organisation that owns the data.
5.2. Justification for the Proposed Approach
When defining the proposed reporting standards and system architecture there were a number of elements to consider, many of which are conflicting. For example, adding more detailed information about events to the test standards increases the level of analysis that is available at the centralised server and makes this more attractive to end-users, but on the other hand having more detailed information in the event messages may raise sensitivity and security issues in terms of the data, which may increase the requirements on the centralised server and may also discourage monitoring network operators from wanting to contribute data in the first place. Similarly, imposing more constraints on the detection equipment at the sensors can help to ensure that events reported by different sensors and monitoring networks are compatible, but if too proscriptive may reduce the available pool of sensors and networks that are able (and willing) to report according to the standards. The proposed approach therefore, is a compromise between these conflicting aspects. An overview of the efforts towards improving the overall data security and access control are described in the design of the STRIKE3 central server, below.
5.3. High Level Design of STRIKE3 Centralised Server
Within the STRIKE3 project a test system will be implemented to demonstrate the system concept and show the benefits of different types of monitoring equipment in a wide network reporting to a centralised server for analysis. Within the STRIKE3 demonstrator, the centralised server will consist mainly of a series of Simple Object Access Protocol (SOAP)-based web services that handle GNSS interference report uploads from a contributor's central hub (and/or from their equipment itself) as well as external end user interference data requests. A database server module is also part of the system and facilitates data storage of all the incoming and outgoing messages. The initial group of web services compiled under the STRIKE3 gateway is:
• Account Management Services.
• Interference Monitoring Data Management Services.
Figure 2 shows how the web services and the rest of the modules are linked together on the server and how the flow of data is running between them. Contributors of interference reports and end users exchange data with the server using the SOAP protocol.

Figure 2. Overview of STRIKE3 threat monitoring and reporting system concept.
The STRIKE3 Gateway is a secure Hypertext Transfer Protocol (HTTP) web server (with Secure Sockets Layer (SSL) or Transport Layer Security (TLS)) that hosts the SOAP-based web services that will be used to handle GNSS interference report requests from either contributors or end user clients.
The Interference Monitoring Data Management Services group includes web services for handling the upload of event information from contributors, as well as requests for data from end users. The services included in this group are:
• Report Upload Service: This service is available to data providers (contributors) only. Its purpose is to allow data providers to upload detection reports to the system. The service will store the reports to the STRIKE3 centralised database and send a negative or positive response back to the client.
• Data Mining Service: This service is available to end users only. Its main purpose is to interrogate the SQL database on request and provide analysis and statistics of the interference reports uploaded by the data providers. Data pattern discoveries and data relationships are also features provided by this service.
• Advanced Data Request Service: This service is available to end users only. Its purpose is to make available extra/advanced information about a report to a user such as RF data, spectrum or spectrogram values, etc., by proving the necessary communication information required to retrieve these extra data (e.g. ftp accounts, email addresses etc.).
The Account Management Services will handle registration of new contributors and end users by issuing a unique digital key, which is necessary before they can contribute to or access data from the centralised server.
It is also noted that the minimum reporting standards have been defined in part to minimise the sensitivity of data that needs to be stored in the centralised servers. Data providers do not have to provide detailed location or time information in reports, and no I/Q sample data is stored in the centralised server - any such data is maintained by the original data provider.
6. TRANSITIONAL STEPS TOWARDS THE FINAL IMPLEMENTATION
Here, the series of future steps to be undertaken towards full implementation of the proposed integrated threat monitoring system are briefly discussed. A demonstration system to showcase the reporting standards and integration of diverse monitoring systems will be developed within the STRIKE3 project. The system architecture (centralised server) is currently being developed, and existing monitoring systems from the project partners are being adapted to provide reports in the standard format. The demonstration system will be ready in October 2017 and a long-term monitoring campaign for one year will collect event reports from a global monitoring network of different types of equipment. The intention of this long-term campaign is to assess the success of the reporting standards, both in terms of ensuring consistent results between systems and in providing a useful set of minimum data for assessing the level of interference activity.
7. CONCLUSIONS
In conclusion, disruptions to GNSS-enabled positioning and navigation have become a global phenomenon. Systems to monitor and report the presence of threats to GNSS signals are increasingly being deployed at locations providing critical public and private services. However, to tackle a global problem the GNSS community requires a global solution.
A comprehensive and simultaneous study of the threat levels and threat categories occurring throughout the world at any given moment will help to define the magnitude of the problem, understand the diversity of threat sources, and study the evolution of this problem over time and space. However, a precursor to launching this wide investigation is the necessity to develop a mechanism whereby disparate monitoring systems with diverse equipment and goals will be capable of and, will be motivated to contribute to a common entity, at least basic information about the threat scenarios they experience.
The STRIKE3 project attempts to lay the foundation and framework for just such a mechanism. The initial steps have been described in this manuscript. First, a thorough background study of the possible causes of intentional and unintentional interference to GNSS signals has been conducted. Second, a state-of-the-art literature survey of existing threat monitoring and reporting systems was conducted. The systems were compared based on their technical specifications, capabilities, and features regarding threat detection, classification, localisation, and spoofing detection. Third, a similar literature survey was conducted regarding threat reporting message formats used by different monitoring networks and standardisation activities related to threat reporting in general.
Based on this background study, the STRIKE3 project proposes a standard for threat reporting messages which will allow disparate monitoring systems to share information with the proposed integrated system. Such standardisation is essential to provide a level ground for comparison of threats, and to allow end-users to map the extent and evolution of the GNSS threat landscape. In parallel, the project also proposed an integrated threat monitoring demonstration system capable of collating threat reports from multiple yet diverse monitoring systems and networks already deployed in the world. The paper discusses the technical architecture and constituent modules of the proposed integrated monitoring system and justifications for the design choices.
ACKNOWLEDGMENTS
This work has been co-funded under the H2020 programme through the European GNSS Agency (GSA).