No CrossRef data available.
Article contents
Accepted manuscript
Unveiling the Scented Spectrum: A Mini Review of Objective Olfactory Assessment and Event-Related Potentials
Published online by Cambridge University Press: 21 October 2024
Abstract
An abstract is not available for this content so a preview has been provided. As you have access to this content, a full PDF is available via the ‘Save PDF’ action button.
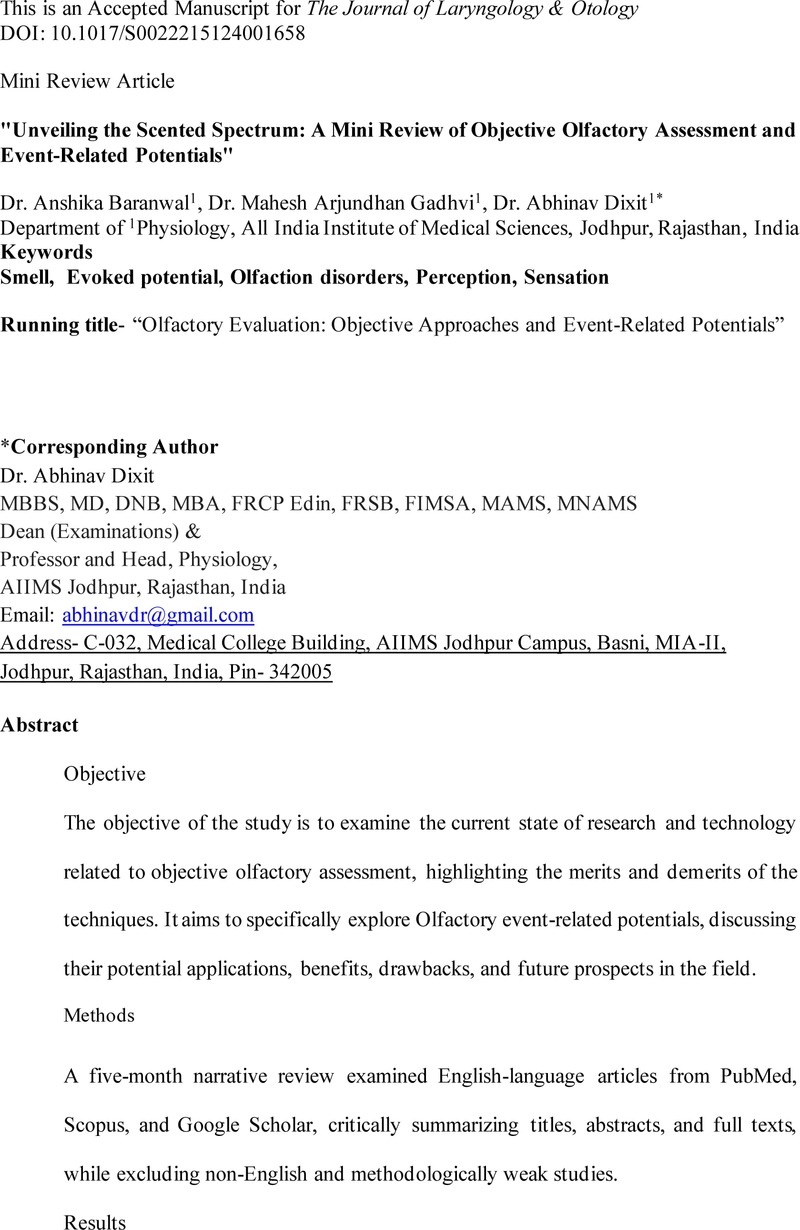
- Type
- Review Article
- Information
- Copyright
- Copyright © The Author(s), 2024. Published by Cambridge University Press on behalf of J.L.O. (1984) LIMITED