Introduction
Human strongyloidiasis is the infection produced mainly by the nematode Strongyloides stercoralis. It is generally an asymptomatic disease in immunocompetent patients. However, in the immunocompromised, life-threatening hyperinfection syndrome and disseminated infection may develop. A characteristic of this parasite is its ability to develop cycles of autoinfection, with the possibility of evolving into chronic infection for decades (Nuñes et al. Reference Nuñes, Pocaterra, Ferrara, Rojas, Pérez-Chacón, Hernán, Certan, Arenas and Goldstein2017; Dacal et al. Reference Dacal, Köster and Carmena2020).
S. stercoralis is a common parasite in tropical and subtropical areas. It is a geohelminth since it has the soil as a means of evolution. Its distribution is generally rural, but it can become urban due to human migrations (Eslahi et al. Reference Eslahi, Badri, Nahavandi, Houshmand, Dalvand, Riahi, Johkool, Asadi, Hoseini Ahangari, Taghipour, Zibaei and Khademvatan2021). The World Health Organization (WHO) reported that in the year 2022 approximately 1.5 billion people, or 24% of the world population, were infected with soil-transmitted helminthiasis throughout the world, of whom it was estimated that between 30 and 100 million were experiencing S. stercoralis infections (Eslahi et al. Reference Eslahi, Badri, Nahavandi, Houshmand, Dalvand, Riahi, Johkool, Asadi, Hoseini Ahangari, Taghipour, Zibaei and Khademvatan2021; WHO 2022).
S. stercoralis has a complex life cycle that includes free-living and parasitic forms. The infection occurs when the infective larva present in the soil penetrates the skin of those who walk barefoot. After their evolution in the intestinal wall of the human host into a parthenogenetic female adult worm, the ensuing larvae produced by these adult parasites pass into the faeces, and the case of inadequate excreta deposition, they can reach the soil (Keiser and Nutman Reference Keiser and Nutman2004).
The diagnosis of strongyloidiasis is difficult, because many cases are asymptomatic, the parasite loads are low, and eggs are not shed in the faeces, but only in larvae. In disseminated strongyloidiasis these larvae are not shed in faeces, giving false negative results. Currently, the most widely used method for the diagnosis of S. stercoralis, due to its ease and low cost, is the classic direct microscopic examination of faecal samples using the Baermann technique, in which the larval forms are sought. This method has a sensitivity of around 70% (Buonfrate et al. Reference Buonfrate, Formenti, Perandin and Bisoffi2015a, Reference Buonfrate, Mena, Angheben, Requena-Mendez, Muñoz, Gobbi and Bisoffi2015b; Dacal et al. Reference Dacal, Saugar, Soler, Azcárate, Jiménez, Merino and Rodríguez2018, Reference Dacal, Köster and Carmena2020). Other tests that allow the diagnosis of strongyloidiasis are the detection of larvae by culture on an agar plate and the Baermann method, with sensitivity between 60 and 85%. However, these methods are laborious, with limited practical use in clinical laboratories (Costa et al. Reference Costa, Bosqui, Corral, Costa-Cruz, Gryschek and de Paula2021). The immunological diagnosis allows for ELISA detection of the patient’s antibodies against the parasite, but the available tests are not yet very specific, due to cross-reactions with other helminths (Machado et al. Reference Machado, Teixeira, Goncalves, Loureiro, Araújo and Costa-Cruz2008; Hailu et al. Reference Hailu, Amor, Nibret, Munshea, Anegagrie, Flores-Chavez, Tang, Saugar and Benito2022).
An alternative for the diagnosis of S. stercoralis is polymerase chain reaction (PCR), which makes it possible to detect the parasite’s DNA in stool, blood, and serum samples (Robertson et al. Reference Robertson, Koehler, Gasser, Watts, Norton and Bradbury2017). Several PCR protocols have been described for the amplification of the different DNA sequences of S. stercoralis, among them: internal transcribed spacer 1 (ITS-1) (Nilforoushan et al. Reference Nilforoushan, Mirhendi, Rezaie, Rezaian, Meamar and Kia2007), 18S ribosomal RNA encoding gene (Verweij et al. Reference Verweij, Canales, Polman, Ziem, Brienen, Polderman and Van Lieshout2009), subunit 1 mitochondrial cytochrome C oxidase (COX1) (Sharifdini et al. Reference Sharifdini, Mirhendi, Ashrafi, Hosseini, Mohebali, Khodadadi and Kia2015), and the gene encoding ribosomal RNA 5.8S (Cunningham et al. Reference Cunningham, Stothard, Osei-Atweneboana, Armoo, Verweij and Adams2018).
Despite the importance of the infections produced by this parasite, the PCR technique has not yet been fully implemented in developing settings as a molecular diagnostic method for S. stercoralis. On the other hand, the reaction conditions of each PCR technique must be adapted to the laboratories where it will be used. In this work different PCR amplification targets for molecular detection of S. stercoralis were compared to standardize a diagnostic protocol with the target that produces the best results.
Materials and methods
Biological samples
S. stercoralis larvae isolated from stool samples of infected patients (according to coproparasitological diagnosis) were used. Each patient agreed to participate with prior authorization by means of informed consent. Similarly, they agreed that the samples would be used, in addition to their diagnosis, for research purposes.
Extraction of DNA
DNA extraction by saline precipitation was performed from larvae isolated from stool samples by the Baermann method. Larvae were transferred to a mortar in an ice bath and crushed. Subsequently, 500 μL of lysis buffer (50 mM Tris-HCL pH 8, 10 mM EDTA pH 8, 1% SDS) was added, and the liquid was collected in microvials. A total of 2.5 μL of proteinase K (20 mg/mL) was added, and the solution was incubated at 55 °C for 2 h. After this time, vials were centrifuged at 14,000 rpm for 10 min and 1 mL of ethanol (95%) and 50 μL of sodium acetate (3 M) were added to the supernatant, which was incubated overnight at -20 °C. The vials were centrifuged at 14,000 rpm for 30 min, and the ethanol was removed. The precipitate was then washed with ethanol (70%) and centrifuged at 14,000 rpm. Subsequently, the ethanol was removed. Finally, the DNA was resuspended in 100 μL of distilled water and stored at -20 °C until use.
DNA electrophoresis
DNA electrophoresis was performed according to the procedure described by Sambrook and Russell (Reference Sambrook and Russell2001). Electrophoresis was performed in 2% agarose gels, using TAE buffer (Tris-acetate-EDTA, 40 mM Tris Acetic Acid, 0.5 M EDTA pH 8.0) and a MINICELL* Primo EC 320 horizontal electrophoresis system (BIORAD Laboratories, Inc. CA, USA). The voltage used was 60–100V, according to the size of the gel. DNA bands were visualized with UV light using the BioRad Gel Doc 1000 system (BIORAD Laboratories, Inc. CA, USA). The size of the DNA bands was determined by comparing them with those of the DNA markers: 1 kb molecular size DNA Marker, Axigen Bioscienses® for DNA samples, and 100 bp plus DNA ladder molecular size marker, Promega®, for PCR products (Promega Corporation, Madison, USA).
Comparison of amplification targets for the detection of S. stercoralis DNA
The amplification targets of the 18S and 5.8S sequences of the DNA encoding the ribosomal RNA and the sequence of the mitochondrial cytochrome C oxidase subunit 1 (COX 1) of S. stercoralis were evaluated. The primers described in Table 1 were used.
Table 1. Characteristics of the primers for the different PCR DNA amplification targets for Strongyloides stercoralis
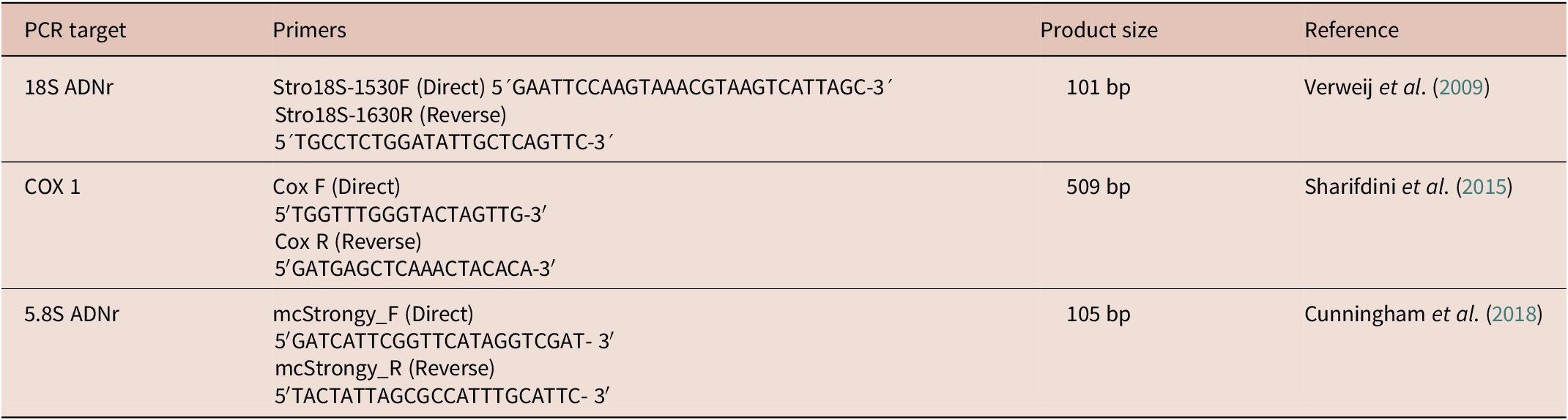
bp= base pairs
Conditions tested for PCR with the different amplification targets
PCR for the amplification of the S. stercoralis sequences studied was carried out using DNA extracted from parasite larvae. The amplification reactions were carried out according to the protocol described by Verweij et al. (Reference Verweij, Canales, Polman, Ziem, Brienen, Polderman and Van Lieshout2009) for the 18S rDNA gene, but by adapting qPCR to conventional PCR. In the case of COX 1, the protocol described by Sharifdini et al. (Reference Sharifdini, Mirhendi, Ashrafi, Hosseini, Mohebali, Khodadadi and Kia2015) was used, but by adapting nested PCR to conventional PCR. For the amplification of the 5.8S gene encoding the rRNA, the protocol described by Cunningham et al. (Reference Cunningham, Stothard, Osei-Atweneboana, Armoo, Verweij and Adams2018) was carried out, but by adapting qPCR to conventional PCR. In the three PCRs tested, various concentrations of reagents (dNTPs, primers, DNA polymerase, and magnesium chloride) and reaction conditions (temperature and number of cycles) were tested to obtain the best results.
Comparison of the amplification targets of the 18S and 5.8S sequences of the rDNA and COX 1 of S. stercoralis
The results of the different conditions tested for the three amplification targets were compared based on: the presence of the expected product, which was clear; the absence of secondary bands, with differences between positives and negatives; and reproducibility of the technique. Subsequently, the PCR technique was standardized with the target providing the best results.
Standardization of PCR protocols
For the standardization of the different PCR protocols, the following conditions were analysed: (i) template DNA concentration, from 100 nanograms (ng) to 1 attogram (ag). The curve was made using the DNA amounts of 100 ng, 10 ng, 1 ng, 100 pg, 10 pg, 1 pg, 100 fg, 10 fg, 1 fg, 100 ag, 10 ag, and 1 ag; (ii) concentration of the different reagents; magnesium chloride (MgCl2) from 1 to 2.5 mM (1; 1.5; 2, and 2.5 mM); deoxynucleotide triphosphates (dNTPs), from 50 to 200 μM (50; 100; 150, and 200 μM); primers, from 0.2 to 0.8 μM (0.2; 0.4; 0.6, and 0.8 μM); Taq DNA polymerase (Promega Corporation, Madison, USA), from 0.5 to 1.25 U (0.5, 0.75, 1.00, and 1.25 U); (iii) amplification program (annealing temperatures and number of cycles). The PCRs were carried out based on the programs described by the PCRs authors, varying the hybridization temperature (57, 60, and 63 ºC) and the number of cycles (35, 40, and 45 cycles). PCR products were visualized on 2% agarose gels and compared with molecular size markers.
Determination of analytical sensitivity and specificity of PCR
Sensitivity was determined by making titration curves with different amounts of DNA (100 ng to 1 ag) to identify the minimum amount of DNA that produced amplification. Specificity was determined using DNA from different parasites (Ascaris lumbricoides, Trichuris trichiura, Enterobius vermicularis, Necator americanus, Schistosoma mansoni, Fasciola hepatica, Taenia solium, Taenia saginata, Toxocara canis, and Leishmania infantum/chagasi) and human DNA from healthy patients, in which amplification should not occur.
Results and discussion
As most PCR targets are based on ribosomal or mitochondrial DNA sequences, we first chose two ribosomal (Verweij et al. Reference Verweij, Canales, Polman, Ziem, Brienen, Polderman and Van Lieshout2009; Cunningham et al. Reference Cunningham, Stothard, Osei-Atweneboana, Armoo, Verweij and Adams2018) and one mitochondrial (Sharifdini et al. Reference Sharifdini, Mirhendi, Ashrafi, Hosseini, Mohebali, Khodadadi and Kia2015) targets.
For the comparison of the targets, the original protocols described by Verweij et al. (Reference Verweij, Canales, Polman, Ziem, Brienen, Polderman and Van Lieshout2009), Sharifdini et al. (Reference Fadaei Tehrani, Sharifdini, Zahabiun, Latifi and Kia2015), and Cunningham et al. (2018) were used, but by adapting qPCR (Verweij et al. 2009; Cunningham et al. 2018) and nested PCR (Sharifdini et al. Reference Sharifdini, Mirhendi, Ashrafi, Hosseini, Mohebali, Khodadadi and Kia2015) formats to conventional PCR and also varying some conditions to best adjust the amplification reactions. The amplification results were compared, and a reaction protocol was standardized for the target that presented the best amplification and reproducibility, to adapt the PCR technique to laboratory conditions.
Conditions tested for amplification of S. stercoralis sequences by PCR
For the standardization of PCR amplification of 18S rDNA and 5.8S rDNA, different amounts of template DNA were evaluated. The optimal amount of DNA ranged between 100 picograms (pg) (10-12 g) and 1 femtogram (fg) (10-15 g). Consequently, 1 pg was taken as the amount of DNA to make subsequent determinations. With COX1 PCR no amplification was obtained at any DNA concentration tested.
Comparing the results obtained with the three amplification targets, it was possible to observe that in the case of 18S rDNA, the amplification bands were in many cases weak, and the results in each step were not reproducible. Using the COX 1 target, no amplification was obtained at any DNA dilution used, nor was it when the concentrations of reagents or reaction conditions were varied. Using the 5.8S rDNA target, DNA amplification was highly reproduced in a wide range of dilutions, so we decided to continue with the standardization of the PCR technique using this amplification target and the optimum reagent concentrations with good amplifications as can be observed in Table 2.
Table 2. Conditions used in the 5.8S-PCR technique for the amplification of S. stercoralis DNA

Once the PCR technique was standardized, the analytical sensitivity and specificity were determined. In the 2% agarose gel, it was possible to observe that the DNA was capable of producing amplification in a range of concentrations from 100 ng to 1 ag, with the analytical sensitivity of the technique being 1 ag (Figure 1a). To determine specificity, DNA from different parasites was used under the same reaction conditions, and it was shown that there was only amplification of the S. stercoralis DNA sample, indicating a specificity of 100% (Figure 1b).

Figure 1. Determination of the sensitivity and specificity of PCR technique for the amplification of the gene encoding ribosomal RNA 5.8S of S. stercoralis by electrophoresis in 2% agarose gel stained with Gel red. (1a) Sensitivity (M) 100 bp Promega® Marker (1–12) DNA Concentrations 100 ng, 10 ng, 1 ng, 100 pg, 10 pg, 1 pg, 100 fg, 10 fg, 1 fg, 100 ag, 10 ag, 1 ag. (13) Negative control. (1b) Specificity. (M) 100 bp DNA Promega® molecular size marker. (1) S. stercoralis DNA. (2–11) DNA of different parasites (2) Ascaris lumbricoides, (3) Trichuris trichiura, (4) Enterobius vermicularis, (5) Necator americanus, (6) Schistosoma mansoni, (7) Fasciola hepatica, (8) Taenia solium, (9) Taenia saginata, (10) Toxocara canis, (11) Leishmania infantum/chagasi, (12) human DNA, (13) negative control.
Comparison of the amplification targets of the 18S rDNA sequences, COX 1, and 5.8S rDNA sequences from S. stercoralis.
With the best conditions established in the afore mentioned protocols, other PCRs were performed to compare results of the different amplification targets, using the three pairs of primers under study, which allow the amplification of different S. stercoralis DNA segments, as described in Table 1. It was possible to demonstrate amplification with the primers for the 5.8S and 18S targets of the S. stercoralis rRNA whose products are 105 bp and 101 bp, respectively, while the COX1 target never produced amplification in any of the tested conditions (Figure 2).

Figure 2. Detection of S. stercoralis DNA using different primers, by gel red-stained 2% agarose gel electrophoresis of S. stercoralis PCR products. (M) Marker 100 bp Promega®, mcStrony 5.8S, Stro 18S, and COX 1.
Strongyloidiasis represents an important medical and veterinary helminthic disease. Human infection is caused mainly by S. stercoralis, and in some cases by S. f. fuelleborni and S. f. kellyi. S.f. fuelleborni probably represents an acquired zoonosis from non-human primates, whereas no animal reservoir for S. f. kellyi has been found. There is much controversy as to whether S. stercoralis represents a zoonosis acquired from dogs and cats. In the last two decades, various molecular techniques have been applied to genotyping Strongyloides spp., finding variability in different geographic areas (Bradbury et al. Reference Bradbury, Pafčo, Nosková and Hasegawa2021).
Patients infected with S. stercoralis may have low parasite loads, so the low sensitivity of parasitological methods and the low specificity of immunological methods, due to cross-reactions with other helminths, do not allow an adequate diagnosis of the disease (Buonfrate et al. Reference Buonfrate, Formenti, Perandin and Bisoffi2015a, Reference Buonfrate, Mena, Angheben, Requena-Mendez, Muñoz, Gobbi and Bisoffi2015b; Fernández-Rivas et al. Reference Fernández-Rivas, Rivaya, Romani, Jun Hao Wang Alcaide and Matas2016).
Some researchers have used molecular biology methods for the diagnosis of strongyloidiasis, as they offer high sensitivity and specificity (Robertson et al. Reference Robertson, Koehler, Gasser, Watts, Norton and Bradbury2017; Bosqui et al. Reference Bosqui, Marquez, Melo, Rosario, Malta, Pavanelli, Conchon, Costa- Cruz and Costa2018). All PCR techniques require prior standardization, adaptation, and evaluation of the reaction conditions for their use and must be validated in each laboratory (Ferrer et al. Reference Ferrer2015). On the other hand, variability of S. stercoralis can lead to primers failing because the primer binding regions were designed in variable regions. In Venezuela, there is no published work on the use of the PCR technique for the diagnosis of strongyloidiasis, so it was unknown which amplification targets could give results for the molecular detection of circulating parasites in this setting. Consequently, the main objectives of this work were to compare different PCR targets for the molecular detection of S. stercoralis and to standardize the PCR technique that would provide the best diagnostic results.
Multiple PCR assays were performed to assess reaction conditions using three primer pairs for molecular diagnosis of S. stercoralis. First, the amplification of the gene encoding the 18S ribosomal RNA of S. stercoralis was performed, varying the concentrations of the different reagents according to the protocol described by Verweij et al. (Reference Verweij, Canales, Polman, Ziem, Brienen, Polderman and Van Lieshout2009).
Compared with the concentrations of reagents used by Verweij et al. (Reference Verweij, Canales, Polman, Ziem, Brienen, Polderman and Van Lieshout2009), in the present work better results were achieved when using lower concentrations of them (MgCl2, dNTPs, primers, Taq polymerase); however, with the use of these primers (for 18S), results could not be reproduced Therefore, it was not possible to finalize the standardization of PCR for the amplification of this target (18S). This could be due to genetic variability of S. stercoralis in the sequence of the primers with no complete complementarity, and this could generate weak and unstable junctions to the DNA template, resulting in no detectable products or indistinct bands. The genetic variability of the 18S rDNA of S. stercoralis has been widely reported (Hasegawa et al. Reference Hasegawa, Hayashida, Ikeda and Sato2009; Bae et al. Reference Bae, Jeong, Shin, Kim, Ahn, Choi and Yu2020; Bradbury et al. Reference Bradbury, Pafčo, Nosková and Hasegawa2021).
Regarding the amplification of COX 1, PCR was performed based on the protocol described by Sharifdini et al. (Reference Sharifdini, Mirhendi, Ashrafi, Hosseini, Mohebali, Khodadadi and Kia2015). No amplification was observed at any of the DNA dilutions used, nor by varying concentrations of reagents. This suggests that in this case the genetic variability could be greater than in the previous case (18S), which could cause the primers to never bind to the DNA template, as they do not find a complementary sequence. Wide genetic variability has also been reported in COX 1, which has allowed the identification of five different groups according to hosts and geographic regions, in a study of 47 isolates of S. stercoralis (28 from humans and 19 from canids) from Asian countries (Bae et al. Reference Bae, Jeong, Shin, Kim, Ahn, Choi and Yu2020; Fadaei Tehrani et al. Reference Fadaei Tehrani, Sharifdini, Zahabiun, Latifi and Kia2019).
In another work analysed, the genetic variability of S. stercoralis based on COX1 from Latin American samples, in a clinical context, 10 haplotypes organized into two groups were found. The most frequent variants have also been described on the Asian continent in humans and canine samples. Regarding symptoms, the presence of group 1 haplotypes increased the risk of reactivation of the infection (Repetto et al. Reference Repetto, Quarroz Braghini, Risso, Argüello, Batalla, Stecher, Sierra, Burgos, Radisic, González Cappa and Ruybal2022).
The results obtained in the amplifications of the two targets described above suggest that due to the genetic variability of S. stercoralis, amplification does not occur or there is no reproducibility of results with the samples found in Venezuela. This was found despite the fact that other authors have used these targets in other countries for the diagnosis of this parasite, obtaining good results. Such was the case for Saugar et al. (Reference Saugar, Merino, Martin-Rabadán, Fernández, Ortega, Garate and Rodríguez2015), who evaluated a real-time PCR for the amplification of the gene encoding the 18S rRNA of Strongyloides spp. and compared it with routine parasitological methods in Spain. The sensitivity and specificity of the PCR were 93.8% and 86.5%, respectively. On the other hand, it may be that the adaptation of qPCR and nested PCR to conventional PCR did not work in those two cases.
Due to the amplification difficulties encountered with the subunits 18S and COX1, the gene encoding ribosomal RNA 5.8S of S. stercoralis was selected for standardization, since it was the target in which amplification was always obtained. The standardization of this PCR was carried out based on the protocol described by Cunningham et al. (Reference Cunningham, Stothard, Osei-Atweneboana, Armoo, Verweij and Adams2018). We found variations in the concentrations of the different reagents, since the latter authors used higher concentrations of dNTPs, primers, and Taq polymerase compared with the lower concentrations used in this work, which represents a major saving of reagents. The same occurred in the number of cycles used, which could be reduced, while no variation was found in the hybridization temperature.
With respect to the sensitivity of the PCR technique for amplification of the RNA 5.8S of S. stercoralis, an amplification range that goes from 100 ng to 1 ag was obtained, indicating that the minimum amount of DNA that is amplified by the PCR technique is the equivalent of one millionth of a larva, since it has been estimated that a larva has between 0.18 and 1.04 pg of DNA (Pillote et al. 2016). Such high sensitivity means traces of a larva might be detected in the stool sample, an important factor for the diagnosis of a disease in which coprological methods show sensitivity problems because infected patients have low parasitic loads (Dacal et al. Reference Dacal, Saugar, Soler, Azcárate, Jiménez, Merino and Rodríguez2018, Reference Dacal, Köster and Carmena2020).
On the other hand, it was determined that the PCR technique for the amplification of the gene encoding the 5.8S rRNA of S. stercoralis did not amplify DNA from other parasites tested or human DNA, thus demonstrating that the technique has 100% specificity. Although this has been demonstrated by other authors (Cunningham et al. Reference Cunningham, Stothard, Osei-Atweneboana, Armoo, Verweij and Adams2018), it should always be evaluated in the area where the PCR will be used due to the possibility of genetic variability of S. stercoralis and also because of the presence of other circulating parasites in the area that could cause interference in the technique.
As mentioned above, other authors have demonstrated genetic variability in both 18S rDNA (Bae et al. Reference Bae, Jeong, Shin, Kim, Ahn, Choi and Yu2020; Bradbury et al. Reference Bradbury, Pafčo, Nosková and Hasegawa2021; Hasegawa et al. Reference Hasegawa, Hayashida, Ikeda and Sato2009) and COX1 (Bae et al. Reference Bae, Jeong, Shin, Kim, Ahn, Choi and Yu2020; Hasegawa et al. Reference Hasegawa, Hayashida, Ikeda and Sato2009; Fadaei Tehrani et al. Reference Fadaei Tehrani, Sharifdini, Zahabiun, Latifi and Kia2019; Repetto et al. Reference Repetto, Quarroz Braghini, Risso, Argüello, Batalla, Stecher, Sierra, Burgos, Radisic, González Cappa and Ruybal2022). For this reason, representative sequences from localities around the world should be used when designing primers. In the work carried out by Barratt et al. (Reference Barratt, Lane, Talundzic, Richins, Robertson, Formenti, Pritt, Verocai, Nascimento de Souza, Mato Soares, Traub, Buonfrate and Bradbury2019), more than 1000 18S rDNA and COX1 sequences of Strongyloides spp. from different hosts and geographic regions were found, demonstrating that Strongyloides spp. varies globally. On the other hand, Fadaei-Therani et al. (2019) mentioned that the COX 1 gene mutates faster than 18S rDNA. Perhaps, for this reason, COX1 has a greater difference with the sequences of the parasites that are circulating in Venezuela and therefore, it is not adequate for the diagnosis of strongyloidiasis in this country.
It is striking that the two targets that produced amplification were evaluated and used by others mainly with samples from Africa, while COX1, which never produced amplification in our experiments, has been mainly evaluated with samples from Asia. In other models of parasitic helminths, for example, in Taenia solium, it has been possible to differentiate two genotypes, one American/African and the other Asian (Yamasaki et al. Reference Yamasaki, Nakao, Sako, Nakaya, Sato, Mamuti, Okamoto and Ito2002), and genetic variability has also been observed in the recognition of different antigenic epitopes. This probably means that some immunological diagnostic techniques for Asian cysticercosis do not work in Venezuela, because Asian antigens are not recognized by samples from Venezuelan patients (Ferrer et al. Reference Ferrer, Sánchez, Milano, Álvarez, La Rosa, Lares, González, Cortéz, Dávila, Harrison, Parkhouse and Gárate2012).
It is very important to carry out sequencing studies of the ribosomal and mitochondrial DNA of S. stercoralis to clarify these suppositions and to identify the haplotypes/genotypes/groups and even species of Strongyloides that are circulating in Venezuela. This is the first molecular study in this regard in Venezuela, and the results obtained show that the PCR technique for the amplification of the 5.8 S ribosomal RNA of S. stercoralis showed high sensitivity and specificity, which could allow early diagnosis of the disease and treatment follow-up, especially in cases of hyperinfection syndrome and disseminated infection. In addition, this work demonstrates the importance of evaluating the amplification targets to be used and of adequate standardization so that the PCR technique becomes reliable and reproducible and can be applied in individual diagnosis as well as a support in epidemiological studies.
Financial support
This work was supported by Universidad de Carabobo, Project DIPISA-PG-2017-004
Competing interest
None.
Ethical standard
The project was approved by the Committee of Bioethics of the Institute of Biomedical Research (University of Carabobo, BIOMED-UC). An informed consent agreement was signed by the individuals who supplied the faeces samples.