Introduction
According to the Food and Agriculture Organization of the United Nations (Reference Fioravanti, Gustinelli, Rigos, Buchmann, Caffara, Pascual and Pardo2022), fisheries and aquaculture – whose production totalled 214 million tonnes in 2020 – will play an increasingly important role in providing food and nutrition to a growing world population in the future. Recent Food and Agriculture Organization of the United Nations statistics show significant increases in the consumption of aquatic foods worldwide. As aquaculture is a prominent actor in this growth, we must face the challenge and secure sustainability in future developments. On the other hand, fishery resources are continuing to decline due to overfishing, pollution, inadequate management and other factors, although the number of landings of biologically sustainable stocks is rising. Therefore, the expansion of the paramount contribution of fisheries and aquaculture to global food security and nutrition will require ‘transformative changes in policy, management, innovation, and investment to achieve sustainable, inclusive and equitable global fisheries and aquaculture’ (Food and Agriculture Organization of the United Nations, Reference Fioravanti, Gustinelli, Rigos, Buchmann, Caffara, Pascual and Pardo2022).
Parasites in fish represent a high diversity of taxa, are ubiquitous components of biological systems and establish obligate interactions with their hosts. They affect the physiology, morphology, reproduction and behaviour of their hosts, and their presence illustrates the host distribution and population structure (Cantatore & Timi, Reference Cantatore and Timi2015). Therefore, these factors may play a prominent role in the changes needed in the near future for aquaculture and fisheries. These roles, as played over the past century, are reviewed and updated in this review.
When the Journal of Helminthology was launched a century ago, fish parasitology was a growing field, but had a predominantly descriptive perspective (i.e. Hesse, Reference Hesse1923; Khalil, Reference Khalil1923; Kobayashi, Reference Kobayashi1923). Yet its relationship to fisheries and aquaculture remained minor or unexplored. At present, parasitism is increasingly recognized as having significant impacts on host individuals, populations, communities and even ecosystems resulting from the tight host–parasite reciprocal adaptations that allow parasites to exploit the specific biological features of their hosts to ensure their transmission and survival and the maintenance of viable populations (Timi & Poulin, Reference Timi and Poulin2020). These characteristics make parasites suitable tools as biological markers to provide information on the diet, movements, population and stock structure of their hosts (Williams et al., Reference Williams, MacKenzie and McCarthy1992; MacKenzie & Abaunza, Reference MacKenzie, Abaunza, Cadrin, Kerr and Mariani2014), a methodology increasingly applicable to fishery management and monitoring (Timi & MacKenzie, Reference Timi and Mackenzie2015; Pita et al., Reference Pita, Casey and Hawkins2016). The historic development of the use of parasites’ tags in fish stock studies, as well as its limitations, benefits, advantages and disadvantages have been comprehensively reviewed in many articles, especially recently (Timi & MacKenzie, Reference Timi and Mackenzie2015; Pascual et al., Reference Pascual, Abollo and González2016). Thus, only recent advances will be discussed here, along with the value of parasite genetics in assessing the host population structure and as indicators of the success of protective measures to restore or preserve aquatic resources. The past century has also presented strong scientific evidence that fish parasites in wild fish stocks challenge fish quality (Mohamed et al., Reference Mohamed, Zuo, Karami, Marnis, Setyawan, Mehrdana, Kirkeby, Kania and Buchmann2020) and may constitute a human health hazard (Strøm et al., Reference Strøm, Haarder, Korbut, Mejer, Thamsborg, Kania and Buchmann2015; Nordholm et al., Reference Nordholm, Kurtzhals, Karami, Kania and Buchmann2020). The occurrence of zoonotic nematodes (Mattiucci et al., Reference Mattiucci, Abaunza, Ramadori and Nascetti2004, Reference Mattiucci, Giulietti and Paoletti2018; Buchmann & Mehrdana, Reference Buchmann and Mehrdana2016; Gay et al., Reference Gay, Bao and MacKenzie2018) and trematodes (Skov et al., Reference Skov, Kania, Dalsgaard, Jørgensen and Buchmann2009; Madsen et al., Reference Madsen, Nguyen, Lanza and Stauffer2022) poses a major problem for the fish processing industry, as the removal of worms before they reach the consumer is labour-intensive and undermines profitability. However, the medical importance and influence on human health will not be discussed further in this work. In contrast, the impact of parasites on fish health in aquaculture will be mentioned, due to the potentially pathogenic effect of many parasite taxa. Protozoans, myxozoans, monogeneans, cestodes, digeneans and crustacean parasites have a major impact on global finfish and shellfish aquaculture and constitute a key constraint to production, sustainability and economic viability (Shinn et al., Reference Shinn, Pratoomyot, Bron, Paladini, Brooker and Brooker2015). Selected problem parasites, which have attracted special attention in the aquaculture industry over the past century, will be treated and discussed in the following sections. Therefore, in this review, our aim is to synthesize the major developments and trends in parasitological research on fisheries and aquaculture carried out over the past century.
Parasites in fisheries
Parasites as natural tags in fisheries, an update
One of the most frequent overlaps between fishery and parasitology is the use of parasite tags for identifying the population structure or stock composition of exploited resources. This science may contribute significantly to fish biology, considering that only about 25% of the global catch currently comes from numerically assessed stocks (Food and Agriculture Organization of the United Nations, Reference Ferrer-Castelló, Raga and Aznar2020). Fisheries lacking formal assessment account for more than 80% of the global catch (Costello et al., Reference Costello, Ovando, Hilborn, Gaines, Deschenes and Lester2012), which indicates an increasing need for alternative and complementary methods of detailed stock assessment as a prerequisite for rebuilding plans and effective management of most resources.
At present, parasite tags are one of the variety of approaches used to identify and classify stocks, which also include catch data, life-history characteristics, mark–recapture, otolith microchemistry, morphology and genetics (Begg & Waldman, Reference Begg and Waldman1999; Cadrin et al., Reference Cadrin, Friedland and Waldman2005). A growing number of studies use parasites as indicators (Timi & MacKenzie, Reference Timi and Mackenzie2015), as they may identify variations and differentiating parameters of parasite populations and communities.
Nearly a century has passed since Herrington et al. (Reference Herrington, Bearse and Firth1939) used parasites to investigate the stock structure of a marine fish for the first time. Since then, the use of parasite biological approaches has become a reliable, well-established subdiscipline of parasitology (Poulin & Kamiya, Reference Poulin and Kamiya2015) that has deserved the publication of two special issues of specialized journals devoted to the subject (Lewis, Reference Lewis2007; Timi & MacKenzie, Reference Timi and Mackenzie2015).
At present there is a consensus on a series of methodological guidelines, which are periodically reviewed and adjusted, although with a variable degree of application at global scale (Timi & MacKenzie, Reference Timi and Mackenzie2015). These reviews discuss and update the criteria for the selection of suitable tags, their advantages and disadvantages, the biological target systems and the perspectives of the study (population structure, migrations and recruitment) for different groups of parasites’ geographical regions (Kabata, Reference Kabata1963; Sindermann, Reference Sindermann1983; MacKenzie, Reference MacKenzie1983, Reference MacKenzie1987, Reference MacKenzie1993, Reference MacKenzie1999, Reference MacKenzie2002; Lester, Reference Lester1990; Moser, Reference Moser1991; Williams et al., Reference Williams, MacKenzie and McCarthy1992; MacKenzie & Abaunza, Reference MacKenzie and Abaunza1998, Reference MacKenzie, Abaunza, Cadrin, Kerr and Mariani2014; Mosquera et al., Reference Mosquera, De Castro and Gómez-Gesteira2003; Timi, Reference Timi2007; Abaunza et al., Reference Abaunza, Murta and Campbell2008a; Lester & MacKenzie, Reference Lester and MacKenzie2009; Baldwin et al., Reference Baldwin, Banks and Jacobson2012; Catalano et al, Reference Catalano, Whittington, Donnellan and Gillanders2014; Cantatore & Timi, Reference Cantatore and Timi2015; George-Nascimento & Oliva, Reference George-Nascimento and Oliva2015; Lester & Moore, Reference Lester and Moore2015; MacKenzie & Hemmingsen, Reference MacKenzie and Hemmingsen2015; Marcogliese & Jacobson, Reference Marcogliese and Jacobson2015; Reed, Reference Reed2015; Timi & MacKenzie, Reference Timi and Mackenzie2015; Pascual et al., Reference Pascual, Abollo and González2016). This compilation of literature provides an ‘evolutionary view’ of the development undergone by the use of parasites as biological markers for the study of fish populations or stocks that resulted in an increasingly efficient and reliable methodology. Therefore, there is no need to repeat their concepts and case studies here. Instead, this review will summarize some of the main recent findings of that research derived from the use of parasite tags and which aims to improve, reinforce or complement this methodology. These topics include: (a) the extension of the use of parasite indicators to host groups other than bony fishes; (b) the increasing interest in selecting those parasites’ groups of guilds, suitable for each kind and scale of study; (c) the host traits driving the structure and composition of parasite assemblages and their effects on their use as natural tags; and (d) the increasingly recognized need for integrative, multidisciplinary studies.
Parasite tags in studies of non-teleost hosts
Parasites can be used as indicators of diverse ecological features even for fragile or difficult to mark species, such as many invertebrates (Williams et al., Reference Williams, MacKenzie and McCarthy1992). The exploitation of aquatic invertebrates largely relies on extractive activities in natural populations, which require strict management measures to ensure sustainability. However, despite the economic relevance of many of these fisheries, that is, squids, scallops, prawns, lobsters, oysters and the knowledge about their parasites, few studies have used parasitological evidence for stock or population assessment. According to Timi & MacKenzie (Reference Timi and Mackenzie2015), the vast majority of published papers on parasite tags deal with teleost fish as hosts, with only a minor proportion of studies on elasmobranchs and invertebrates of commercial interest. This situation has not changed in recent years, with only four new publications on elasmobranchs (Isbert et al., Reference Isbert, Rodríguez-Cabello, Frutos, Preciado, Montero and Pérez-del-Olmo2015; Irigoitia et al., Reference Irigoitia, Incorvaia and Timi2017, Reference Irigoitia, Levy, Canel and Timi2022; Gérard et al., Reference Gérard, Hervé, Hamel, Gay, Barbier and Barreau2022) being issued since that revision. Yet no new research has been conducted on exploited invertebrates, despite the value of natural tags having been recognized for many years (Williams et al., Reference Williams, MacKenzie and McCarthy1992; Pascual & Hochberg, Reference Pascual and Hochberg1996). The present development and acceptance of this methodology may promote research on natural tags of many groups of exploited invertebrates to assist in ensuring a suitable management and sustainable exploitation of these resources.
Improvements in the selection of parasite tags
A series of criteria for selecting ideal parasite tags have been articulated and refined over the years (Kabata, Reference Kabata1963; MacKenzie, Reference MacKenzie1983, Reference MacKenzie1987; Sindermann, Reference Sindermann1983; Williams et al., Reference Williams, MacKenzie and McCarthy1992), although parasites that meet all of them are not frequently found (MacKenzie & Abaunza, Reference MacKenzie, Abaunza, Cadrin, Kerr and Mariani2014). Among the suitable characteristics, the residence time of a parasite in the subject host is one of the key features worth considering (Lester & MacKenzie, Reference Lester and MacKenzie2009). Indeed, it is suggested that parasites should persist in the host for a long period, that is, at least one year for stock identification and recruitment studies, whereas species with life spans of less than one year are acceptable for studies of seasonal migrations (MacKenzie & Abaunza, Reference MacKenzie, Abaunza, Cadrin, Kerr and Mariani2014). Some recent studies have tested these recommendations by comparing long-lived vs. short-lived parasite guilds, with results confirming the validity of these criteria. For example, long-lived larval endoparasites of red porgy Pagrus pagrus were able to discriminate among stocks along the Atlantic coast of South America, whereas short-lived guilds (ectoparasites and gastrointestinal endoparasites) exhibited significant differences even among samples belonging to a single stock (Soares et al., Reference Soares, Lanfranchi, Luque, Haimovici and Timi2018). Similar results were obtained for the Sympterigia bonapartii, a seasonally migratory skate from the same region. However, in that case, variations of transient parasites were related to the migratory behaviour of the fish, constituting, therefore, suitable markers for fish migration (Irigoitia et al., Reference Irigoitia, Incorvaia and Timi2017). In addition, temporal and or short-scale spatial variations in parasitism have been subjected to methodological analyses to avoid the possible occurrence of pseudoreplication problems (Ferrer-Castelló et al., 2007; Espinola-Novelo & Oliva, 2016).
Effect of host traits on the application of parasite tags
The role of host traits in the value of fish parasites as tags has been also under methodological scrutiny. Since long-lived parasites are recommended as biological tags for most studies, and cumulative patterns with fish age and/or size are common to them (Timi et al., Reference Timi, Luque and Poulin2010, Reference Timi, Rossin, Alarcos, Braicovich, Cantatore and Lanfranchi2011), prevalence and abundance tend to increase with host traits such as size, mass, age and even sex. Consequently, there is a need to evaluate their value as descriptors of the host population structure of stocks. In this context, Braicovich et al. (Reference Braicovich, Ieno, Sáez, Despos and Timi2016) conducted a comparative analysis of the effect of host variables on the parasite abundance and species richness in Percophis brasiliensis caught in the Argentine Sea. Length and sex consistently appeared in the most parsimonious models, suggesting that fish length seems to be a slightly better predictor than age or mass. Thus, these authors confirmed that fish size is a suitable measure of growth and recommended restricting the comparisons to fish of similar length or incorporating length as covariate when comparing parasite burdens. Host sex should be also considered for sexually dimorphic fish in terms of morphology, behaviour or growth rates.
Beyond the effect of characteristics of individual fish on their parasite burdens, evolutionary and ecological traits may exert considerable influence on comparative analyses. For example, Levy et al. (Reference Levy, Canel, Rossin, Hernández-Orts, González-Castro and Timi2019) demonstrated that parasites can be excellent models for comparative research in assessments of fine-scale population structure when site fidelity and strong adaptations to local conditions prevail and/or where physical heterogeneity needs to be revealed (Levy et al., Reference Levy, Canel, Rossin, Hernández-Orts, González-Castro and Timi2019).
The interaction between host traits (such as size or age) and ecology must be considered in some studies. For example, for migratory species whose foraging and spawning habitats alternate seasonally during their life cycles, ontogenetic changes in the structure of parasite assemblages must be taken into account since, due to cumulative patterns, host-length differences can lead to a misinterpretation of the patterns, especially when migrations are asynchronous among cohorts. This was observed for Umbrina canosai migrating between the coastal waters of southern Brazil and northern Argentina (Canel et al., Reference Canel, Levy, Braicovich, Haimovici and Timi2021). Thus, differences in parasite assemblages were more clearly observed in young fish, indicating possible variations in migratory routes, distance travelled and/or latitude reached, depending on environmental conditions and age. Consequently, it is advisable to consider the differences among fish length classes when using parasite tags for resources with temporally and spatially variable migratory patterns, especially when different cohorts are compared.
Need for integrative multidisciplinary studies
Among the most recent literature, the need for integrative studies applying simultaneously different, but complementary techniques for stock identification has been a recurrent topic for improving fishery management tools (McClelland et al., Reference McClelland, Melendy, Osborne, Reid and Douglas2005; Abaunza et al., Reference Abaunza, Murta and Campbell2008b; Niklitschek et al., Reference Niklitschek, Secor, Toledo, Lafon and George-Nascimento2010; Baldwin et al., Reference Baldwin, Banks and Jacobson2012; Mattiucci et al., Reference Mattiucci, Cimmaruta, Cipriani, Abaunza, Bellisario and Nascetti2015; Van der Lingen et al., Reference Van der Lingen, Weston, Ssempa and Reed2015; Welch et al., Reference Welch, Newman and Buckworth2015; de Moor et al., Reference de Moor, Butterworth and van der Lingen2017; Brickle et al., Reference Brickle, Randhawa, Reid, Lee, Shcherbich and Arkhipkin2021; Zhang et al., Reference Zhang, Zhu, Xu and Chen2021). In general, higher discriminatory power and increased accuracy of stock assignment may result from combining different sources of information, including host genetics, otolith microchemistry and parasites (Baldwin et al., Reference Baldwin, Banks and Jacobson2012; Brickle et al., Reference Brickle, Randhawa, Reid, Lee, Shcherbich and Arkhipkin2021; Zhang et al., Reference Zhang, Zhu, Xu and Chen2021). These advantages also include the elucidation through the complementarity of connectivity patterns of host populations across different spatial and temporal scales (Taillebois et al., Reference Taillebois, Barton and Crook2017).
Parasite genetic data and fisheries
Genetic markers are used for complementing and resolving taxonomic issues, thus helping to achieve higher taxonomic resolutions in ecological studies. Poor taxonomic resolutions may mask undetected biodiversity, probably leading to biases in ecological analyses where accurate estimates of species richness or species co-occurrences matter (Poulin & Leung, Reference Poulin and Leung2010). For example, the genetic identification of several species of larval Anisakis spp. has contributed to the identification of stocks of the European hake (Merluccius merluccius) in Atlantic and Mediterranean waters (Mattiucci et al., Reference Mattiucci, Abaunza, Ramadori and Nascetti2004), of Atlantic horse mackerel, Trachurus trachurus (Mattiucci et al., Reference Mattiucci, Farina, Campbell, MacKenzie, Ramos, Pinto, Abaunza and Nascetti2008) and of the skipjack tuna Katsuwonus pelamis in the north-west Pacific (Takano et al., Reference Takano, Iwaki, Waki, Murata, Suzuki, Kodo, Kobayashi and Ogawa2021). Similarly, the genetic identification of larval Anisakis spp. confirmed the relative contribution of oceanographically contrasting masses of waters to the parasite fauna of the silvery John dory Zenopsis conchifer, living in a transitional zone (Lanfranchi et al., Reference Lanfranchi, Braicovich, Cantatore, Alarcos, Luque and Timi2016, Reference Lanfranchi, Braicovich, Cantatore, Irigoitia, Farber, Taglioretti and Timi2018).
Beyond the value for parasite systematics, the development of molecular and computational tools for population genetics and phylogenetics has fuelled an enormous advancement of parasite ecology, including the use of molecular data on parasites to infer diverse aspects of the ecology of their hosts and reconstruct their evolutionary and demographic histories (Nieberding & Olivieri, Reference Nieberding and Olivieri2007; Archie et al., Reference Archie, Luikart and Ezenwa2009). For example, the use of genetic markers to assess population structures has been applied to parasites relevant for aquaculture, such as the sea louse Lepeophtheirus salmonis, and has improved the understanding of its dispersal capabilities and control in farmed salmonids (Glover et al., Reference Glover, Stølen, Messmer, Koop, Torrissen and Nilsen2011; Messmer et al., Reference Messmer, Rondeau, Jantzen, Lubieniecki, Davidson and Koop2011).
Selected genetic markers comprise innate tags whose advantages are that all members of a population are inherently marked and do not affect the behaviour or survival of the organisms. Furthermore, information may be obtained on conservation issues, the identification of catch origins and migratory routes (Antoniou & Magoulas, Reference Antoniou, Magoulas, Cadrin, Kerr and Mariani2014). The use of genetic information to ascertain population membership of individuals includes a variety of approaches known as assignment methods, also used to identify the number of populations coexisting in a given area, mixed-stock analysis or the origin of migrant individuals (Manel et al., Reference Manel, Gaggiotti and Waples2005; Criscione et al., Reference Criscione, Cooper and Blouin2006).
In fisheries, both mitochondrial and nuclear genetic markers are commonly used for such purposes (Antoniou & Magoulas, Reference Antoniou, Magoulas, Cadrin, Kerr and Mariani2014), but unfortunately such assignments are often inaccurate when there is little or no neutral genetic differentiation among source populations, outlining the need for alternative methods (Criscione et al., Reference Criscione, Cooper and Blouin2006). Independently of techniques utilized for the study of the geographical distribution of genetic lineages, phylogeographic patterns between species linked by a parasitic relationship may be congruent in time and space for specific, obligate parasites (Nieberding et al., Reference Nieberding, Morand, Libois and Michaux2004). The usefulness of genetic data on parasites species to assign fish to source populations was initially proposed by Beverley-Burton (Reference Beverley-Burton1978) after comparing the frequencies of allozymes in Anisakis simplex parasitizing Atlantic salmon. After this and early in the 21st century, the use of parasite genetic signatures to assess host populations and regions of origin was recommended and viewed as a promissory perspective (Manel et al., Reference Manel, Berthier and Luikart2002). Nevertheless, despite the potential of parasite genetics to identify many aspects of host phylogeography and population structure, molecular methodologies have not been widely applied to fish parasites relevant to fisheries in general (Criscione et al., Reference Criscione, Poulin and Blouin2005) and as markers of fish stocks (Pascual et al., Reference Pascual, Abollo and González2016).
The underlying idea of this methodology is that if a parasite is genetically subdivided more finely than its host, then the genotypes of the parasite in question could potentially be used to assign hosts to their population of origin with higher probabilities than by using the host genotypes (Criscione et al., Reference Criscione, Poulin and Blouin2005). Support for this concept is the fact that the rate of molecular evolution is faster in parasite DNA and RNA, relative to that within the homologous loci of their hosts, which yields genetic sequences that are comparatively better sources of data (Whiteman & Parker, Reference Whiteman and Parker2005). Furthermore, in addition to differences in mutation rates, the shorter generation time of most parasites relative to their hosts accelerates the evolutionary processes, allowing favourable genotypes of the population obtained by mutation, recombination or migration, to rapidly increase in frequency, rendering powerful inferential tools (Gandon & Michalakis, Reference Gandon and Michalakis2002; Whiteman & Parker, Reference Whiteman and Parker2005). Additionally, a parasite can be more genetically structured than its host due to the different rates of gene flow of effective population sizes between them, because not all hosts are infected and because parasites may be locally adapted at a higher level compared to their hosts (Criscione et al., Reference Criscione, Cooper and Blouin2006).
The first study to test the hypothesis that parasite genotypes are more accurate in assigning hosts to source populations than the host genotypes themselves was carried out using microsatellite markers to compare the accuracy of assignment back to known source populations between steelhead trout Oncorhynchus mykiss and its strictly freshwater parasitic trematode Plagioporus shawi (Criscione et al., Reference Criscione, Cooper and Blouin2006). These authors showed that the genotypes of the parasite had fourfold greater odds of correct assignment than host genotypes. This highlighted how the genotypes of the parasites are more accurate than host genotypes when assigning individuals to their population of origin. They may also be useful for identifying individuals from protected areas, and for tracing dispersal patterns or feeding grounds for migratory species.
More recently, several authors have studied the population genetic structure of a parasite species to infer that of its fish host along a geographical range, using mitochondrial genetic markers (table 1). Most studies have been carried out for larval Anisakis spp. on fish, with the exception of a study on cetaceans (Marigo et al., Reference Marigo, Cunha and Bertozzi2013). The success in identifying the population structure varied with the identity of both host and parasite species, the host ecology, the region of origin and the geographical scale. Indeed, this success relied on the selection of an appropriate scale of sampling to account for the different life histories and geographical distribution of the species being studied (Cross et al., Reference Cross, Collins, Campbell, Watts, Chubb, Cunningham, HatWeld and MacKenzie2007). According to Baldwin et al. (Reference Baldwin, Banks and Jacobson2012), the features and approaches necessary for selecting parasite molecular markers include: (a) ease of recovery and identification; (b) the use of more than one genetic marker to verify that a parasite species is cryptic; (c) an assessment of mutation rates for specific genetic marker types; and (d) temporal and geographical stability in a parasite population to enable long-term monitoring.
Table 1. Studies using genetic markers (mitochondrial genes) to assess population structure of marine parasites of relevance in fisheries.

In addition to those factors driving the population structure of parasites – such as evolutionary and ecological history, mode of reproduction and transmission, host dispersal, and life-cycle complexity – anthropogenic factors, such as biological invasions, environmental perturbation, global warming and extractive activities, may affect the parasite faunas (Zarlenga et al., Reference Zarlenga, Hoberg, Rosenthal, Mattiucci and Nascetti2014). In particular, stressors such as overfishing can reduce the host population size (Wood et al., Reference Wood, Lafferty and Micheli2010), with a concomitant reduction in parasite populations and the genetic diversity within them. Thus, a minimal genetic structure among geographically isolated populations may be interpreted as a hallmark of human activity (Zarlenga et al., Reference Zarlenga, Hoberg, Rosenthal, Mattiucci and Nascetti2014). Nevertheless, little has been done to correlate habitat degradation with genetic diversity among parasites’ populations, although clear-cut examples are available about the effects of habitat disturbance on the genetic diversity of anisakid nematode populations (Mattiucci & Nascetti, Reference Mattiucci and Nascetti2007, Reference Mattiucci and Nascetti2008). The values of genetic variability of different anisakid genera (i.e. Anisakis, Contracaecum and Pseudoterranova) were significantly higher in Austral populations than in Boreal regions in coincidence with a lower degree of habitat disturbance in southern populations (Mattiucci et al., Reference Mattiucci, Paoletti, Cipriani, Webb, Timi, Nascetti, Klimpel, Kuhn and Melhorn2017). Increased overfishing, the by-catch of cetaceans, the hunting and disease mortality of seals, sea water pollution and acidification in Boreal regions reduce host population sizes, which reduces the population of lower anisakid and heightens the probability of genetic drift phenomena in the parasite gene pools (Mattiucci et al., Reference Mattiucci, Cimmaruta, Cipriani, Abaunza, Bellisario and Nascetti2015). Therefore, comparisons of genetic variability among parasite populations inhabiting ecosystems with a different degree of disruption may be applied to an evaluation of the effect of overfishing or, alternatively, of protection measures for many fisheries.
Phylogeographic analysis of parasites, as well as those variations in their genetic diversity resulting from the effect of anthropogenic stressors, represent valuable and reliable sources of information to be integrated into multidisciplinary studies dealing with a holistic approach to fisheries management and stock identification (Begg & Waldman, Reference Begg and Waldman1999), aiming for the long-term maintenance and sustainability of fishery resources.
Phylogeographic analysis of both parasites and their fish host, performed on the same genes, are promissory tools to be included in multidisciplinary studies on stock structure (Mattiucci et al., Reference Mattiucci, Cimmaruta, Cipriani, Abaunza, Bellisario and Nascetti2015). Such co-phylogeographic studies, under the ‘magnifying glass hypothesis’ (the increased chance of tracking the genealogical history of the host with genetic data of the parasite) (Huyse et al., Reference Huyse, Oeyen, Larmuseau and Volckaert2017; Geraerts et al., Reference Geraerts, Huyse and Barson2022), along with the recent advances in next-generation DNA sequencing technologies and the genome-wide genotyping applications to fish parasites, will undoubtedly be of paramount relevance to fisheries and aquaculture in the near future.
Parasites as indicators of the success of Marine Protected Areas (MPAs)
Fishing practices and aquaculture, in combination with many other anthropogenic factors, have led to a rapid and radical degradation of marine ecosystems. This may drive considerable and complex changes in the physical structure, chemistry, biology and ecological functioning of oceans (Lubchenco et al., Reference Lubchenco, Palumbi, Gaines and Andelman2003). Some of the signs of such alterations, related to fisheries, include abrupt changes in species composition, habitat degradation, epidemics, mass mortalities and the collapse of fisheries (Lubchenco et al., Reference Lubchenco, Palumbi, Gaines and Andelman2003).
An effective mechanism for preventing or reversing these widespread declines and for protecting, maintaining and restoring ecosystems is the implementation of MPAs, a key management tool employed worldwide to conserve biodiversity and sustain fisheries (Pelletier et al., Reference Pelletier, Claudet, Ferraris, Benedetti-Cecchi and Garcìa-Charton2008; Watson et al., Reference Watson, Dudley, Segan and Hockings2014). Indeed, MPAs allow for the recovery of depleted stocks of exploited species and provide a source of individuals for fished areas (Lubchenco et al., Reference Lubchenco, Palumbi, Gaines and Andelman2003).
Although most MPAs allow some extractive activities such as fishing, by protecting both biota and their biophysical environments, they offer an ecosystem-based approach to conservation or fisheries management (Lubchenco et al., Reference Lubchenco, Palumbi, Gaines and Andelman2003). An efficient management of MPAs requires continuous feedback on the objectives achieved and the suitable indicators for assessing the efficacy of using them (Pomeroy et al., Reference Pomeroy, Watson, Parks and Cid2005). Numerous studies have provided a broad dataset on the biological effects of reserve protection for a wide range of geographical locations and organisms (Lester et al., Reference Lester, Halpern, Grorud-Colvert, Lubchenco, Ruttenberg, Gaines, Airamé and Warner2009). On average, positive effects of reserve protection on the biomass have been reported, such as increases in density, species richness and the size of organisms within their boundaries (Lester et al., Reference Lester, Halpern, Grorud-Colvert, Lubchenco, Ruttenberg, Gaines, Airamé and Warner2009). However, there is considerable variation in the responses documented across reserves (Lester et al., Reference Lester, Halpern, Grorud-Colvert, Lubchenco, Ruttenberg, Gaines, Airamé and Warner2009), which requires further and permanent improvement of the evaluation of their ecological effects (Claudet & Guidetti, Reference Claudet and Guidetti2010).
Recent analyses (Sala & Giakoumi, Reference Sala and Giakoumi2018) showed that the biomass of whole fish assemblages in marine reserves is, on average, 670% greater than in adjacent unprotected areas, and 343% greater than in partly protected MPAs. This suggests that reserves contribute to the restoration of the complexity of ecosystems through trophic cascades once the abundance of large animals recovers sufficiently. Given the dependence of parasites on the population density of all hosts involved in their life cycles, as well as on the complexity of food webs for those trophically transmitted species (Wood et al., Reference Wood, Lafferty and Micheli2010), differences in free living communities due to protective measures are expected to have a correlate in parasite communities.
An example was provided by Huspeni & Lafferty (Reference Huspeni and Lafferty2004), who evaluated the success of an ecological restoration project at a degraded site, the Carpinteria Salt Marsh in California, United States, in a before–after, control–impact study using larval digeneans infecting the California horn snail, Cerithidea californica. Over a period of six years, trematode prevalence nearly quadrupled and species richness doubled at restored sites, whereas both factors remained unchanged at control sites. These authors attributed these changes to the use of the restored habitats by birds.
At present, several studies have evaluated the success of MPAs using fish parasites as biological indicators (table 2), most of which recorded increased abundance, species richness and/or diversity of different parasite taxa, resulting from the protection measures and varying with their characteristics. Only a few exceptions were unable to detect changes in parasite loads (Loot et al., Reference Loot, Aldana and Navarrete2005; Ternengo et al., Reference Ternengo, Levron, Mouillot and Marchand2009). Most of these studies have also evaluated the effect of MPAs on fish parasites by comparing protected and unprotected areas, with the exception of Braicovich et al. (Reference Braicovich, Irigoitia, Bovcon and Timi2021), who compared temporal changes in the composition and structure of parasite communities of P. brasiliensis. The hosts were caught at the beginning of the implementation of protection measures (temporal and spatial closures to fishery) and after a period of 13 years in a coastal region of northern Argentina (table 2).
Table 2. Studies on fish parasites as indicators of success of Marine Protected Areas (MPAs).
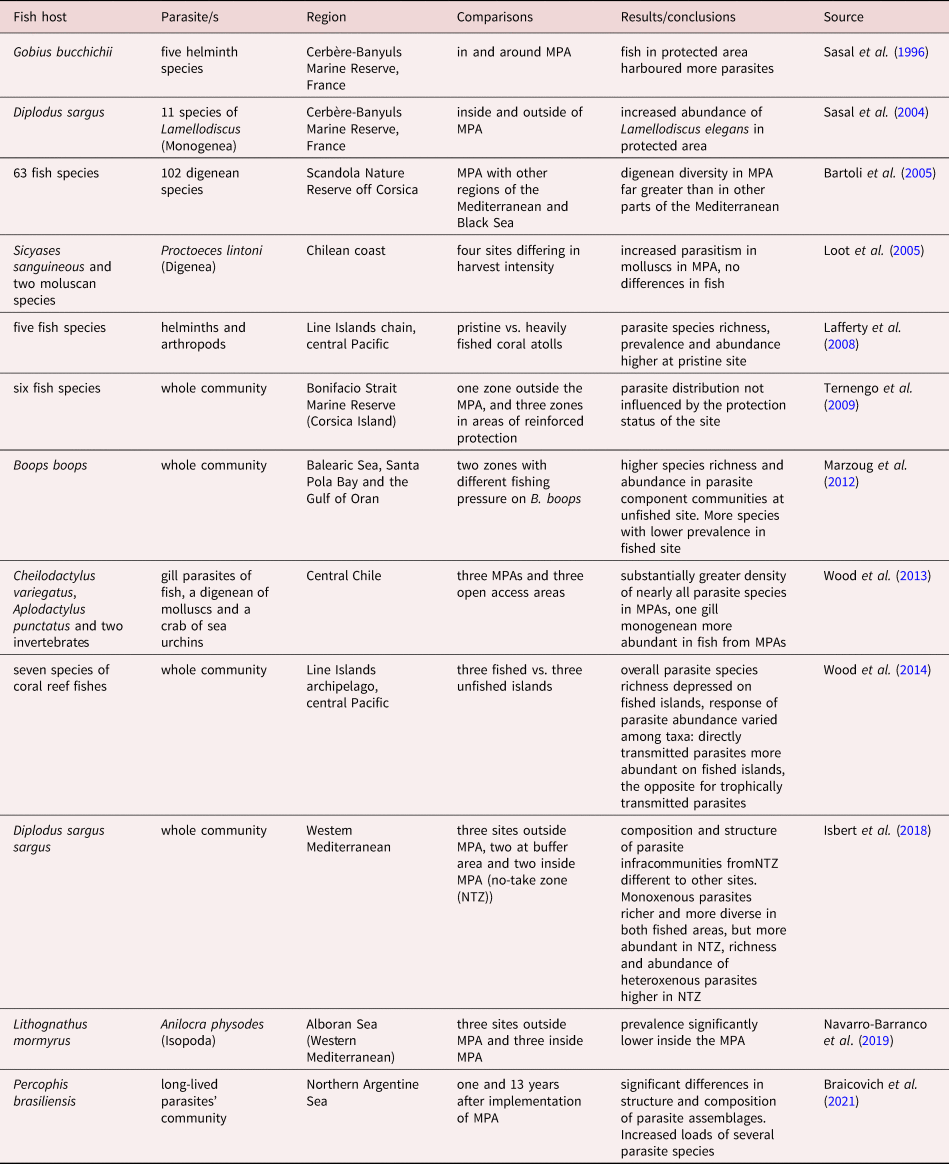
Temporal evaluation of changes due to MPAs should be complementary to that of spatial evaluation, since either biotic or abiotic factors can affect the structure of parasite populations and assemblages, even at an exceedingly small scale (Levy et al., Reference Levy, Canel, Rossin, Hernández-Orts, González-Castro and Timi2019). Therefore, the most reliable results are expected when using a sampling scheme comparing ‘before and after’. When complemented by simultaneous sampling ‘inside and outside’ the MPAs, this will permit the identification of any possible regional change from local variations occurring as a consequence of the protection measures.
As fishing activities reduce the density of fish and other hosts and may affect the fish populations (selectively removing large fish), they may reduce food web complexity and drive a decline in parasite burdens (Wood et al, Reference Wood, Lafferty and Micheli2010). The protection of these environments, therefore, is expected to restore the systems to their original state or at least return them as closely as possible to their original conditions, explaining the majority of the observed increases in the levels of parasitism in the studies listed in table 2. However, under certain circumstances, reserves can pose threats to conservation by increasing the density and, thus, the transmission of parasites, especially through enhanced contact rates in small, overcrowded or highly diverse reserves where organisms may be more vulnerable to infection (Ezenwa, Reference Ezenwa2004; McCallum et al., Reference McCallum, Gerber and Jani2005; Lebarbenchon et al., Reference Lebarbenchon, Poulin, Gauthier-Clerc and Thomas2007). For example, for parasites with heteroxenous life cycles, the diversity and abundance in intermediate and/or paratenic hosts should consequently increase with the diversity and abundance of definitive hosts (Hechinger & Lafferty, Reference Hechinger and Lafferty2005).
Beyond the use of parasites as indicators of the success of MPAs, their possibly detrimental effects, together with that of other pathogens, have received virtually no attention (McCallum et al., Reference McCallum, Gerber and Jani2005; Wootton et al., Reference Wootton, Woolmer, Vogan, Pope, Hamilton and Rowley2012) and should be included in any cost–benefit analysis of marine reserves to improve their efficacy and subsequent management strategies (Wootton et al., Reference Wootton, Woolmer, Vogan, Pope, Hamilton and Rowley2012).
Parasites in aquaculture
Historical reports on parasites and aquaculture
Although the production of plants and animals in the aquatic environment (aquaculture) has greatly expanded over the past century in general and the last fifty years in particular (Food and Agriculture Organization of the United Nations, Reference Fioravanti, Gustinelli, Rigos, Buchmann, Caffara, Pascual and Pardo2022), this food production method is not novel. Chinese reports have described the production of various cyprinid fish species in freshwater pond culture systems since the early days, at least 8000 years ago (Costa-Pierce, Reference Costa-Pierce2022). It is also from these ancient aquacultures that the first reports of fish parasites appeared (Lom & Dykova, Reference Lom and Dykova1992). Asia is still the main producer of aquacultured fish (Food and Agriculture Organization of the United Nations, Reference Fioravanti, Gustinelli, Rigos, Buchmann, Caffara, Pascual and Pardo2022), and the other main players in this region, historically and currently, are Indonesia (Rimmer et al., Reference Rimmer, Sugama, Rakhmawati, Rofiq and Habgood2013), India (Kumar et al., Reference Kumar, Khar, Dwivedi, Sharma and Himabindu2015) and Vietnam (De Silva & Phuong, Reference De Silva and Phuong2011). In Africa, archaeological evidence points to early fish-keeping in small ponds in ancient Egypt (Costa-Pierce, Reference Costa-Pierce2022), associated with exceedingly early reports of the occurrence of fish pathogens (Snieszko, Reference Snieszko1975), whereas large-scale farming developed much later in East Africa (Dadzie, Reference Dadzie1992; Mwainge et al., Reference Mwainge, Ogwai, Aura, Mutie, Ombwa, Nyaboke, Oyier and Nyyaudi2021). In South America, Brazilian aquaculture dates back to the 17th century (Valenti et al., Reference Valenti, Barros, Moraes-Valenti, Bueno and Cavalli2021), but the main focus of South American aquaculture parasitology developed much later in Chile with the advent of salmonid mariculture necessitating a focus on the ectoparasitic crustacean Caligus rogercressei. United States and Canadian mariculture industries similarly urged fish parasitologists to target L. salmonis in their research (Burka et al., Reference Burka, Fast, Revie, Woo and Buchmann2012). The North American catfish farming industry (Engle et al., Reference Espínola-Novelo and Oliva2022) was also a cradle for aquaculture parasitology. Russian aquaculture dates back several centuries. Although the culture of cyprinids has been in focus, major technological steps have been taken within sturgeon production since the 1860s (Vasilyeva et al., Reference Vasilyeva, Elhetawy, Sudakova and Astafyeva2019). In Europe, archaeological evidence points to the existence of primitive fish-keeping facilities already 6000 years ago (Costa-Pierce, Reference Costa-Pierce2022), but more organized production systems appeared later on when carp aquaculture was organized in the Roman Empire from 100 BC to AD 500. The artificial reproduction of trout was developed during the 18th century, and production facilities were subsequently established in several European countries and in North America. Despite the ancient history of aquaculture, large scale production was established far later. The intensification of production systems mainly arose over the past century, a period in which aquacultured fish from both freshwater and marine systems have gained increasingly greater economic importance (Food and Agriculture Organization of the United Nations, Reference Fioravanti, Gustinelli, Rigos, Buchmann, Caffara, Pascual and Pardo2022).
Intensification of fish parasitology with the development of aquaculture
Along with the aquaculture intensification process, the study of parasitic diseases became indispensable due to the role of the diseases as a production-limiting factor. Ancient reports on diseases, although not strongly documented, date back to 330 BC. (Snieszko, Reference Snieszko1975), and classical zoologists with an interest in parasites, such as Müller (Reference Müller1776) and Abildgaard (Reference Abildgaard1794a, Reference Abildgaardb), described a range of parasites in fish. Although the stringency and level of detail in their descriptions lag behind modern systematics, their contributions remain valid. The impact of parasites on productivity was not a major issue until commercial aquaculture developed, however.
The spread of natural infections (caused by viral, bacterial, protozoan and metazoan pathogens) of wild fish to domesticated fish species was largely uncharacterized and unknown before the intensification process. The impact of infections on cultured fish kept in high densities became increasingly visible. The pathogens could be detected, isolated and described, and with the increasing focus on fish production seen during the past century, important parasitic pathogens have been the subject of increasing interest and research efforts. In the early 20th century, Hofer (Reference Hofer1904) and later Plehn (Reference Plehn1924) published their treatises on parasitic diseases in fish. In the following years, a wide series of publications, research papers and textbooks emerged and now constitute a valuable basis for the control of parasitic diseases in aquaculture.
The number of parasites in wild fish is extremely high, which is a consequence of the vast number of fish species (33,000) described. The high specificity of fish parasites will result in a correspondingly high number of parasites. In contrast, there are 369 species of aquacultured fish (Food and Agriculture Organization of the United Nations, Reference Fioravanti, Gustinelli, Rigos, Buchmann, Caffara, Pascual and Pardo2022), meaning that the number of parasite species is correspondingly lower. This is reflected in the publications on fish parasites published over the past century. Papers on wild fish issues are concerned with a wide diversity of parasites, whereas papers on aquaculture parasites target a limited number of species. On the other hand, the number of publications on the individual aquaculture parasite species may be extreme due to the massive problems caused by individual species such as Ichthyophthirius multifiliis and L. salmonis.
Differing impacts of different parasite types
The different parasite types also differ with regard to their role as pathogens in aquaculture settings. Nematodes with complex life cycles, such as anisakids, are highly prevalent in wild fish stocks (Gay et al., Reference Gay, Bao and MacKenzie2018; Mattiucci et al., Reference Mattiucci, Giulietti and Paoletti2018), but are generally not found in aquacultured fish (Fioravanti et al., 2021). The swim bladder nematode Anguillicoloides crassus, which uses copepods as intermediate hosts (and fish as transport hosts), may occur in eel cultures following the introduction of wild-caught infected elvers, because stocking is still based on wild fish. When subsequently established in fish cultures, these can have a devastating impact on productivity, but these nematodes exert their main impact on wild stocks (Lefebvre et al., Reference Lefebvre, Fazio, Crivelli, Woo and Buchmann2012).
Likewise, there are reports of acanthocephalans occurring in aquacultured fish, but these are primarily associated with pond cultures connected to natural waters from where intermediate hosts (amphipods and isopods) may enter the production system. Their impact on fish in the most intensive aquaculture production systems is limited, however, because the water inlet is controlled and transmission eliminated. In contrast, other parasites with less complex life cycles may flourish. Below, selected examples of protozoans and metazoans (monogeneans, cestodes and crustaceans) in aquaculture settings are presented, focusing on their development over the past century.
Problematic protozoans
Over the past century, protozoans have caused serious disease in aquacultured fish, which has fostered a vast amount of literature focused on amoebae (Nowak, Reference Nowak, Woo and Buchmann2012; Nowak et al., Reference Nowak, Valdenegro-Vega, Crosbie and Bridle2014), haemoflagellates Cryptobia (Woo & Li, Reference Woo and Li1990), Trypanoplasma (Wiegertjes et al., Reference Wiegertjes, Groeneveld and van Muiswiunkel1995), bodonid flagellates (Ichthyobodo) (Chettri et al., Reference Chettri, Kuhn, Jafaar, Kania, Møller and Buchmann2014), diplomonadid flagellates (Spironucleus) (Sterud et al., Reference Sterud, Mo and Poppe1998), scuticociliates (Philasterides) (Lamas et al., Reference Lamas, Sanmartin, Parama, Castro and Cabaleiro2008) and hymenostomatid ciliates such as Ichthyophthirius (Dickerson et al., Reference Dickerson, Clark and Leff1993).
Finds of I. multifiliis in cultured fish, macroscopically visible as epidermal white spots, were detected in China as early as the Sung dynasty (starting from AD 926) (Lom & Dykova, Reference Lom and Dykova1992). This protozoan was scientifically described later on in France by Fouquet (Reference Fouquet1876), as it was frequently found in trout in nearby freshwater ponds. During the 20th century, the parasite was the subject of numerous investigations due to its high pathogenicity and commercial importance. It was especially interesting that fish developed a level of resistance to reinfection indicating the development of an immune response (Buschkiel, Reference Buschkiel1910), as this could imply that immunoprophylaxis, including vaccination, would be a possible control strategy. Russian fish parasitologists investigated this in more depth and noted that the antiparasitic response was positively correlated to the size of the primary infection (Bauer, Reference Bauer1953). It was later shown that carp activated a series of humoral and cellular immune factors while infected (Hines & Spira, Reference Hines and Spira1973, Reference Hines and Spira1974), and these authors could confirm a protective response established in the fish host. The accumulation of host lymphocytes, macrophages and neutrophils around the feeding stage of the parasite (the trophont) in the host epidermis was further characterized by Cross & Matthews (Reference Cross and Matthews1993). The lymphocytes surrounding the trophonts were later demonstrated to stain positively for B-cell and T-cell markers (Olsen et al., Reference Olsen, Heinecke, Skjødt, Rasmussen, Kania and Buchmann2011). The humoral reactions were shown to include antibodies with high specificity in some cases (Dickerson et al., Reference Dickerson, Clark and Leff1993; Clark et al., Reference Clark, Lin and Dickerson1996; Sigh & Buchmann, Reference Sigh and Buchmann2001; Alishahi & Buchmann, Reference Alishahi and Buchmann2006) and complementary factors (Gonzales et al., Reference Gonzales, Nielsen and Buchmann2007).
Following the development of new immunological tools, it was shown that not only did the fish host produce immunoglobulin M (IgM) (Dickerson et al., Reference Dickerson, Clark and Leff1993; Sigh & Buchmann, Reference Sigh and Buchmann2001), but also another immunoglobulin class that was recently detected and termed immunoglobulin T (IgT). These antibodies were secreted both systemically and locally on mucosal surfaces against the invading parasite (Jørgensen et al., Reference Jørgensen, Heinecke, Skjoedt, Rasmussen and Buchmann2011; Olsen et al., Reference Olsen, Heinecke, Skjødt, Rasmussen, Kania and Buchmann2011; Xu et al., Reference Xu, Parra and Gomez2013). Whereas genes encoding immunoglobulin classes IgM and IgT are found upregulated during the course of infection, a third class, immunoglobulin D was downregulated (Jaafar et al., Reference Jaafar, Ødegård and Mathiessen2020). Furthermore, transcriptomic data subsequently indicated that the number of genes involved in the rainbow trout response towards I. multifiliis infection counted more than 1796 up-regulated and 1556 down-regulated gene sequences (Syahputra et al., Reference Syahputra, Kania, Al-Jubury, Jafaar, Dirks and Buchmann2019). Despite the well documented development of an adaptive immune response in fish towards I. multifiliis, and several attempts to produce vaccines against the infection, experimental vaccines (based on proteins or DNA) (Jørgensen et al., Reference Jørgensen, Sigh, Kania, Holten-Andersen, Buchmann, Clark, Rasmussen, Einer-Jensen and Lorenzen2012, Reference Jørgensen, Kania, Rasmussen, Mattsson, Schmidt, Al-Jubury, Sander, Salanti and Buchmann2017) have only exhibited a partial protection, and commercial vaccines are still not available at present.
Investigations conducted over the past century have described various ways to control the infection by use of chemotherapeutants and medicines. The organic dye malachite green was applied for years in facilities producing both ornamental and production fish (Alderman, Reference Alderman1985). Its toxicity and carcinogenicity, and subsequent ban of its usage (European Food Safety Authority, Reference Engle, Hanson and Kumar2016), prompted farmers to search for alternatives (Rintamäki-Kinnunen et al., Reference Rintamäki-Kinnunen, Rahkonen, Mannermaa-Keränen, Suomalainen, Mykrä and Valtonen2005; Picon-Camacho et al., Reference Picon-Camacho, Marcos-Lopez and Shinn2012). Biocides used in water baths – such as formalin, hydrogen peroxide (Rach et al., Reference Rach, Gaikowski and Ramsay2000), peracetic acid (Meinelt et al., Reference Meinelt, Matzke, Stüber, Pietrock, Wienke, Mitchell and Straus2009; Mathiessen et al., Reference Mathiessen, Marana, Korbut, Wu, Al-Jubury, Karami, Kania and Buchmann2021b), sodium percarbonate (Heinecke & Buchmann, Reference Heinecke and Buchmann2009), copper sulphate (Straus, Reference Straus1993) and potassium permanganate (Straus & Griffin, Reference Straus and Griffin2001) – were then investigated for a description of their efficacy and environmental impact. The biocides releasing hydrogen peroxide generally induce an inflammatory response in the skin of the fish host (Mathiessen et al., Reference Mathiessen, Marana, Korbut, Wu, Al-Jubury, Karami, Kania and Buchmann2021b), but are considered relatively safe from an environmental point of view, because the reaction products from the interaction with organic material are carbon dioxide, oxygen and water. These oxidizing agents are being applied in increasing amounts, but the search for alternative products is still ongoing. Herbal extracts have exhibited strong effects on different stages of the parasite (Lin et al., Reference Lin, Hua, Zhang, Xu, Fu, Liu and Zhou2016; Mathiessen et al., Reference Mathiessen, Jaafar, Al-Jubury, Jørgensen, Kania and Buchmann2021a), but before licensing, marketing and possible application at farm level, further investigations into the environmental impact, toxicity towards the host and any effect on human health are needed. Correspondingly, a lipopeptide (surfactant) isolated from the bacterium Pseudomonas H6 has lethal effects on the theronts, tomonts and tomocysts of Ichthyophthirius (Al-Jubury et al., Reference Al-Jubury, Lu, Kania, Jørgensen, Liu, de Bruijn, Raaijmakers and Buchmann2018; Li et al., Reference Li, He, Jaafar, Kania and Buchmann2022). Subsequent studies of this microbial compound demonstrated a low level of host effects (skin inflammation in rainbow trout) (Mathiessen et al., Reference Mathiessen, Marana, Korbut, Wu, Al-Jubury, Karami, Kania and Buchmann2021b) and limited adverse effects on cyanobacteria, green algae, Daphnia and zebrafish (Korbut et al., Reference Korbut, Skjolding, Mathiessen, Jaafar, Li, Jørgensen, Kania, Wu and Buchmann2022). These initial ecotoxicological steps suggest a potential future use of the compound in aquaculture enterprises, but the road to licensing must be completed before its usage. This will include future research into the toxicological and environmental effects.
Due to the questionable use of chemotherapy, research efforts targeting alternative methods have been increasingly prominent. One approach has highlighted the use of ultraviolet irradiation (Gratzek et al., Reference Gratzek, Gilbert, Lohr, Shotts and Brown1983), and another the application of mechanical control techniques by removing key life-cycle stages. Continuous filtration of water to remove tomonts, thus preventing tomocyst formation with the release of infective stages, was demonstrated by Heinecke & Buchmann (Reference Heinecke and Buchmann2009). In line with this approach, Shinn et al. (Reference Shinn, Picon-Camacho, Bawden and Taylor2009) launched a strategy for removal of tomocysts by coating fish tanks with a material to prevent tomocyst attachment. This enabled the loose parasite stages to be removed by a modified vacuum-cleaning technique.
A more recent approach to Ichthyophthirius control in fish farms is based on the observed differential susceptibility of fish to infection, which suggests that it is possible to breed fish with a higher natural resistance to infection. When exposing an outbred population of rainbow trout to infection, using infective theronts, a serious infection develops but with a different time span from disease to death (Jaafar et al., Reference Jaafar, Ødegård and Mathiessen2020). By genotyping individual fish, both susceptible fish and those that stay healthy, it was possible to identify genetic markers (single nucleotide polymorphisms (SNPs)) in the host genome associated with relative resistance. This information was then applied by the breeder to select spawners carrying the beneficial SNPs. By using these selected fish as parent fish to produce the next generation, it is possible to establish strains with improved resistance (Buchmann et al., Reference Buchmann, Nielsen, Mathiessen, Marana, Duan, Jørgensen, Zuo, Karami and Kania2022).
Problematic myxozoans
Over the past century, myxozoan biology research has expanded markedly. The number of described species in both wild and cultured fish populations has increased dramatically, but for aquaculturists the main target was to develop control methods. The impact of Myxobolus cerebralis, eliciting whirling disease in trout cultures, attracted the interest of various research groups. With the, at the time, surprising results by Wolf & Markiw (Reference Wolf and Markiw1984), showing that oligochaetes were obligate elements of the life cycle, a brand-new stage for biological and life-cycle studies opened up in the field of myxozoan biology. The past four decades have seen prominent research and the surprising impact of myxozoans on aquaculture production (Sitjà-Bobadilla et al., Reference Sitjà-Bobadilla, Diamant, Palenzuela and Alvarez-Pellitero2007). The elevated exposure of this specific field has paved the way for numerous descriptions of life cycles (Székely et al., Reference Székely, Borkhanuddin, Cech, Kelemen and Molnár2014) and new insights into immune responses in the host towards myxozoans (Bartholomew et al., Reference Bartholomew, Lorz, Sollid and Stevens2003; Sitjà-Bobadilla et al., Reference Sitjà-Bobadilla, Diamant, Palenzuela and Alvarez-Pellitero2007; Holzer et al., Reference Holzer, Carla Piazzon, Barrett, Bartholomew and Sitjà-Bobadilla2021).
Problematic monogeneans
Monogeneans have a direct life cycle and pathogenic potential, which is illustrated by events occurring in both natural waters and aquaculture settings. A series of fish-parasitizing monogeneans were already described by Müller (Reference Müller1776), among these, large species such as the capsalid Entobdella hippoglossi from halibut, which in aquacultural settings may suffer from infection (Bergh et al., Reference Bergh, Nilsen and Samuelsen2001). In the same era, the monogeneans Nitschia sturionis from the sturgeon and Axine belones, from the garfish Belone belone, were described by Abildgaard (Reference Abildgaard1794a, Reference Abildgaardb). The latter monogenean was later shown to be highly pathogenic to Acipenser nudiventris, an endemic sturgeon species in the Aral Sea. The different susceptibility of different sturgeon species became evident following the anthropogenic introduction of the parasite with Acipenser stellatus from the Caspian Sea. The latter host species seemed to support a balanced relationship with the parasite in its original habitat (the Caspian Sea). However, when N. sturionis was introduced to a new location, the parasite spread to the endemic sturgeon species, which appeared highly susceptible. Consequently, the introduced monogenean caused an epidemic resulting in mass mortality among the local sturgeon population (Petrushevski & Shulman, Reference Petrushevski, Shulman, Dogiel and Petrushevski1961). The interest in monogeneans and their peculiarities emerged in the 20th century, and Russian (Bychowky, Reference Bychowsky1957), Pacific (Yamaguti, Reference Yamaguti1968; Ogawa, Reference Ogawa, Woo and Buchmann2012; Whittington, Reference Whittington, Woo and Buchmann2012), European (Llewellyn, Reference Llewellyn1965; Justine, Reference Justine1993) and American (Kritsky & Boeger, Reference Kritsky and Boeger2002) schools laid the groundwork for extensive, global monogenean research. However, the description of the genus Gyrodactylus by Nordmann (Reference Nordmann1832) had already initiated the description of several hundred species within this genus, many of which showed high pathogenic potential. A classic case is the epidemic caused by Gyrodactylus salaris, which spread through more than fifty salmon rivers in Norway. The parasite species was originally isolated and described by Malmberg (Reference Malmberg1957). The specimens were obtained from Atlantic salmon (Salmo salar) in a Swedish salmon hatchery producing juvenile fish from a local strain of Baltic salmon. The species was subsequently introduced in Norway during the 1970s with infected salmon smolts (Mo, Reference Mo, Woo, Leong and Buchmann2020). In Baltic rivers (draining Swedish, Finnish and Russian catchment areas), the infection level was generally low due to a well-documented lower susceptibility/higher resistance in the strains of Baltic salmon compared to the east Atlantic salmon stocks. These Atlantic strains, occurring in Norway, Scotland and Denmark, all showed a high susceptibility and vulnerability to G. salaris infection (Bakke et al., Reference Bakke, Jansen and Hansen1990; Dalgaard et al., Reference Dalgaard, Nielsen and Buchmann2003; Lindenstrøm et al., Reference Lindenstrøm, Sigh, Dalgaard and Buchmann2006). This encouraged genetic research to determine genetic markers for resistance against infection in the Atlantic salmon genome (Gilbey et al., Reference Gilbey, Verspoor, Mo, Sterud, Olstad and Hytterød2006), suggesting that breeding could improve the survival rate of exposed fish.
Severe disease is often associated with monogeneans infecting fish hosts in confined environments, such as public aquaria. This was documented by Jahn & Kuhn (Reference Jahn and Kuhn1932) and Nigrelli & Breder (Reference Nigrelli and Breder1934), both of which described massive infections by Neobenedenia melleni across a broad spectrum of host fishes. In the same period, freshwater aquaculture became increasingly popular, and particular attention was given to monogeneans recognized as pathogens in cyprinid aquaculture. The fish were suffering from monogenean infections comprising species within the genera Dactylogyrus and Gyrodactylus. Common carp cultures were found to be infected with Dactylogyrus vastator, Dactylogyrus anchoratus and Dactylogyrus extensus (Wunder, Reference Wunder1929; Prost, Reference Prost1963; Paperna, Reference Paperna1964; Buchmann et al., Reference Buchmann, Slotved and Dana1993) and tench cultures with Gyrodactylus macracanthus (Wilde, Reference Wilde1937). The extensive introduction of cyprinid species from East Asia to Europe further stimulated the research into parasites of aquacultured species. Thus, Molnar (Reference Molnar1984) could add several introduced dactylogyrids, including Dactylogyrus lamellatus imported with grass carp Ctenopharyngodon idella, to the list of new species in Europe. Various chemicals and drugs have been used for treatment, and one of the anthelmintics, praziquantel, showed an effect (Schmahl & Mehlhorn, Reference Schmahl and Mehlhorn1985). The farming of Japanese eels has a long history in the Far East including Japan, but recirculating aquaculture systems were constructed in the 1970s and stocked with glass-eels caught in natural waters. The congeneric species Pseudodactylogyrus anguillae and Pseudodactylogyrus bini, originally described in China and Japan, respectively, were thereby introduced into European farms and showed a tremendous ability to propagate under these confined conditions (Buchmann, Reference Buchmann, Woo and Buchmann2012). The anthelmintic mebendazole was shown to eliminate the parasites (Szekely & Molnar, Reference Szekely and Molnar1987), which initiated the frequent use of the compound at farm level. However, reduced sensitivity to the drug was documented in the monogeneans after repeated treatments (Buchmann et al., Reference Buchmann, Roepstorff and Waller1992), supporting previous reports outlining the risk of anthelmintic resistance in monogeneans (Goven et al., Reference Goven, Gilbert and Gratzek1980).
With the development of mariculture facilities, introducing a range of new species with a high market value, the natural parasites of the new production species demonstrated their pathogenic potential. One of the maricultured species, Seriola quinqueradiata, carries the monogenean Benedenia seriolae, a large capsalid that colonizes the head, flanks, eyes and fins of the fish. The continuous browsing of epidermal tissue will cause skin erosion and require treatment (Whittington, Reference Whittington, Woo and Buchmann2012). Evidence points to the potential for breeding strains with a higher natural resistance to infection. Thus, quantitative trait loci for resistance were determined through a genome-wide analysis (Ozaki et al., Reference Ozaki, Yoshida, Fuji, Kubota, Kai and Aoki2013), which paves the way for future breeding programmes. Puffer fish (Takifugu rubripes) are a major commodity in Japanese aquaculture, and the monogenean Heterobothrium okamotoi, occupying the branchial cavity of the fish, has proved a major pathogen (Ogawa, Reference Ogawa, Woo and Buchmann2012). It is haematophagous, and severe infection levels cause anaemia in the host, which has made control methods a major research target (Ogawa & Yokoyama, Reference Ogawa and Yokoyama1998; Kimura et al., Reference Kimura, Nomura, Kawakami, Itano, Iwasaki, Morita and Enomoto2009). The maricultured rockfish Sebastes schlegeli is infected with Microcotyle sebastis, creating corresponding pathological challenges due to the haematophagous feeding habit of the parasite. Research pointed to anthelmintic treatments using praziquantel as the most promising method (Kim & Cho, Reference Kim and Cho2000). A corresponding polyopisthocotylean monogenean Sparicotyle chrysophrii, causing similar problems in Mediterranean mariculture of gilthead seabream, may be controlled by this anthelmintic as well (Sitjà-Bobadilla et al., Reference Sitjà-Bobadilla, Felipe and Alvarez-Pellitero2006). The possibility of taking an immunoprophylactic approach (vaccination) to reduce the impact of parasitism was presented by Kim et al. (Reference Kim, Hwang, Cho and Park2000).
Problematic cestodes
Some species of cestodes, such as the so-called Asian tapeworm Bothriocephalus acheilognathi, play a role in some fish productions. The parasite is associated with cyprinid production, and over the past century, reports have shown that carp fry in particular suffer due to high loads of cestodes (Scholz & Di Cave, Reference Scholz and Di Cave1993; Scholz et al., Reference Scholz, Kuchta, Williams, Woo and Buchmann2012). As Asian carp production dominates global aquaculture (Food and Agriculture Organization of the United Nations, Reference Fioravanti, Gustinelli, Rigos, Buchmann, Caffara, Pascual and Pardo2022), the overall impact of this cestode should not be disregarded. Another bothriocephalidean cestode Eubothrium crassum occurs in the pyloric caeca of salmonids and may decrease the growth rate in mariculture at certain sites (Saksvik et al., Reference Saksvik, Nilsen, Nylund and Berland2001). As fish also may serve as intermediate hosts of certain cestodes with a human pathogenic potential, such as Dibothriocephalus (syn. Diphyllobothrium) latum and Dibothriocephalus dendriticum, a series of studies in this field has been published over the past century.
Problematic digeneans
Most digenean life cycles depend on an intermediate molluscan host. Various snail species have shown to be able to colonize aquaculture ponds if these are connected to natural aquatic systems. Over the past century, numerous studies have documented how these molluscs play this key role in the transmission of digeneans to aquacultured fish. Several of these digeneans have demonstrated their pathogenic potential in fish. A classic example is the group of eye flukes using birds as a final host, snails as the initial intermediate hosts and the fish host as an intermediate host harbouring metacercariae in their eyes (Karvonen & Marcogliese, Reference Karvonen, Marcogliese, Woo, Leong and Buchmann2020). Representative genera are Diplostomum and Tylodelphys, parasites occupying the lens and corpus vitreum of the fish host, respectively. These eye flukes may severely affect vision (Duan et al., Reference Duan, Jørgensen, Kania, Al-Jubury, Karami and Buchmann2021), whereas other species reach maturity in mammals including humans and are thus regarded as serious zoonotic pathogens. Classic examples are Clonorchis, Opisthorchis, Metagonimus, Haplorchis, Centrocestus and Pseudamphistomum where the most effective control methods established rely on snail control in ponds and the control of infective stages in inlet water (Skov et al., Reference Skov, Kania, Jørgensen and Buchmann2008, Reference Skov, Kania, Dalsgaard, Jørgensen and Buchmann2009; Madsen et al., Reference Madsen, Nguyen, Lanza and Stauffer2022). A prominent digenean group are blood flukes with a non-molluscan host, a polychaete, as the intermediate host. These parasites infect tuna in their natural environment but due to the practice of stocking wild juvenile tuna in net pens, these blood worms have become aquacultural parasites (Power et al., Reference Power, Evenden, Rough, Webber, Widdicombe, Nowak and Bott2021).
Problematic parasitic crustaceans
Crustacean parasites comprise a richness of species, and the literature encompassing species descriptions, life cycles and general biology has expanded over the past century (Kabata, Reference Kabata1979). With the advent of new aquaculture, species-specific interest has been concentrated on those exhibiting pathogenic potential. This applies to branchiurans (Møller, Reference Møller, Woo and Buchmann2012), isopods such as Cerathotoa in gilthead seabream farming (Mladineo et al., Reference Mladineo, Hrabar and Vidjak2020), copepods such as lernaeids (Avenant-Oldewage, Reference Avenant-Oldewage, Woo and Buchmann2012) and, not least, caligids (L. salmonis and C. rogercrosseyi) (Burka et al., Reference Burka, Fast, Revie, Woo and Buchmann2012). Mariculture based on net pens stocked with salmonids developed during the 1960s (Gjedrem, Reference Gjedrem and Gjedrem1986). With an increasing number of fish in confined environments exposed to natural environmental parameters, including various pathogens, parasitic problems rapidly emerged. Lepeophtheirus salmonis showed the potential to propagate rapidly in mariculture settings, and it is now considered the main parasitic problem in the net-pen based production of salmonids (Torrissen et al., Reference Torrissen, Jones, Asche, Guttormsen, Skilbrei, Nilsen, Horsberg and Jackson2013). This causative pathogen has been known about for centuries from observations of wild Atlantic salmon returning from their sea migration. Berland & Margolis (Reference Berland and Margolis1983) found written evidence that the bishop Erik L. Pontoppidan (1698–1764) observed Atlantic salmon (S. salar) with heavy infestations of lice, probably salmon lice, when they returned to the rivers of Western Norway. The first scientific description was done by Krøyer (Reference Krøyer1838), who reported that this crustacean parasite was common in Danish salmon in summer. Krøyer termed the species Caligus salmonis, but its lack of lunules places it in the genus Lepeophtheirus erected by Nordmann (Reference Nordmann1832). In high-intensity occurrences, the parasite may cause massive damage to the host's skin. Erosion of the epidermis and dermis may expose underlying muscle layers, which was also described in wild Atlantic salmon returning from sea migration to the Moser river in Nova Scotia decades before mariculture was established (White, Reference White1940). Since the early reports of salmon lice problems in mariculture during the 1970s, the interest in research has increased exponentially. The number of peer reviewed scientific publications about L. salmonis found at SCI (Science Citation Index, Web of Science) has reached 1469 in mid-2022 and close to 100 papers on salmon lice are being published annually. Accordingly, the total number of citations may exceed 5000 per year. Due to the marked disease problem caused by the lice, a main part of the publications has been concerned with control methods, including chemical and medical treatments. A series of compounds and drugs have been tested and various drugs and compounds such as hydrogen peroxide (Helgesen et al., Reference Helgesen, Aaen, Romstad and Horsberg2015), organophosphates (Kaur et al., Reference Kaur, Jansen, Aspehaug and Horsberg2016), pyrethroids (Bakke et al., Reference Bakke, Agusti, Bruusgaard, Sundaram and Horsberg2018), emamectin benzoate (Stone et al., Reference Stone, Roy, Sutherland, Ferguson, Sommerville and Endris2002; Lees et al., Reference Lees, Baillie, Gettinby and Revie2008; Poley et al., Reference Poley, Purcell, Igboeli, Donkin, Wotton and Fast2013) and chitin synthesis inhibitors (Harðardottir et al., Reference Harðardottir, Male, Nilsen and Dalvin2019) have been investigated and subsequently used. However, due to the rapid development of resistance to treatment (Helgesen et al., Reference Helgesen, Aaen, Romstad and Horsberg2015; Kaur et al., Reference Kaur, Jansen, Aspehaug and Horsberg2016; Bakke et al., Reference Bakke, Agusti, Bruusgaard, Sundaram and Horsberg2018), researchers have been looking for other control strategies. These may include the use of cleaner fish (labrids and lumpfish) (Bjordal, Reference Bjordal1991; Groner et al., Reference Groner, Cox, Gettinby and Revie2013; Imsland et al., Reference Imsland, Reynolds, Eliassen, Hangstad, Foss, Vikingstad and Elvegård2014). Removal of infective larvae using filtration by bivalves was shown to work experimentally (Bartsch et al., Reference Bartsch, Robinson, Liutkus, Ang, Webb and Pearce2013). Direct high-tech combatting of lice on salmon by radiating the lice with laser beams was tested, but found less effective (Bui et al., Reference Bui, Geitung, Oppedal and Barrett2020). Mechanical removal by freshwater flushing has been widely implemented in the industry (Østevik et al., Reference Østevik, Stormoen and Evensen2022) and may, despite its adverse effects on the host, lead to a reduction of the lice count. More intricate farm constructions have used the knowledge about host-finding behaviour by the infective salmon louse copepodids. These seek the upper layers of water when attaching to the host, an observation that was exploited by Geitung et al. (Reference Geitung, Oppedal, Stien, Dempster, Karlsbakk, Nola and Wright2019) when constructing snorkel cages to prevent copepodids from attacking salmon kept at lower water depths. Still other ideas were developed that included the use of herbal repellents to jam the parasite's host finding (O'Shea et al., Reference O'Shea, Wadsworth, Pino-Marambio, Birkett, Pickett and Mordue-Luntz2016). Genetic markers for susceptibility have been identified (Gharbi et al., Reference Gharbi, Glover, Stone, MacDonald, Matthews and Grimholt2009), and quantitative trait loci for resistance in salmon have been described (Robledo et al., Reference Robledo, Guitierrez, Barria, Lhorente, Houston and Yanez2019), which may lead to development of breeding programmes to obtain salmon with a higher natural resistance to infection. Several research groups have focused on the immune response in fish against lice infestations (Dalvin et al., Reference Dalvin, Jørgensen, Kania, Grotmol, Buchmann and Øvergård2020) and have documented the responses. This has encouraged others to invest in the development of vaccines to control the salmon lice problem at salmon farms, and recently Tartor et al. (Reference Tartor, Karlsen and Skern-Mauritzen2022) reported a partial reduction of infection in Atlantic salmon vaccinated with a recombinant louse gut protein. Although the research efforts in this field must be characterized as impressive and extensive, they have yet to result in a control of the problem, but serve as a sound basis for future research.
Future perspectives
According to the United Nations, it is estimated that nearly eleven billion people will inhabit the Earth by the end of the 21st century (Adam, Reference Adam2021), and the global food-producing sector, including fisheries and aquaculture, faces the challenge of increasing its levels of production to ensure food and nutrition for this growing population. Marine fish stocks, however, are in decline in many parts of the oceans due to overfishing and climate change (Sumaila & Tai, Reference Sumaila and Tai2020), and their assessment and the basis for management are preferably achieved through integrated analysis (or integrated population modelling) methods, including the use of parasites and other biological markers (Punt et al., Reference Punt, Dunn, Elvarsson, Hampton, Hoyle, Maunder, Methot and Nielsen2020).
For such purposes, parasitologists must provide reliable tools that can be integrated into multidisciplinary studies. Therefore, further research is required to clarify the selection criteria for biological indicators, adjusting them to each kind of study, host–parasite system and environment. The role of different host traits on parasite populations must be also determined and their effects controlled in order to achieve reliable comparisons. Finally, the use of molecular studies of parasites – both to increase the taxonomic resolution of quantitative studies and to use the parasite population structure as an additional tool for fish stock assessment – is one of the most promising avenues for new research on parasite tags.
The studies on parasites in aquaculture settings have increased exponentially over the past century due to the massive development of the aquaculture business and the need to safeguard the health of production fish suffering from parasitic infections. The potential for further expanding the industry is high (Gentry et al., Reference Gentry, Froehlich, Grimm, Kareiva, Parke, Rust, Gaines and Halpern2017) and may surpass the expected continued expansion of aquaculture productions in coming decades (Food and Agriculture Organization of the United Nations, Reference Fioravanti, Gustinelli, Rigos, Buchmann, Caffara, Pascual and Pardo2022), which will further emphasize the need for parasitological research. As the massive investment, due to the economic importance of aquaculture, already seen in parasitological research has resulted in an enormous expansion of the overall knowledge about fish parasites and their biology, it is likely that we will enjoy a further development of the literature in the field of aquaculture parasites in the years ahead.
Acknowledgement
The authors thank Dr Robert Poulin for kindly inviting us to write this contribution to this Special Issue celebrating the 100th anniversary of the Journal of Helminthology.
Financial support
Financial support was provided by grants from Fondo para la Investigación Científica y Tecnológica, ANPCyT (PICT 2018 No. 1981, PICT 2018 No. 2359) and Universidad Nacional de Mar del Plata (15/E1062; EXA1104/22).
Conflicts of interest
None.
Ethics statement
Since this is a review work, no ethical statement is needed regarding use of animals for scientific purposes.