Introduction
Milne Fiord on the north coast of Ellesmere Island contains the 300 km2 Milne Ice Shelf. At the head of the fiord (lat. 82°31′N., long. 80°40′W.), contiguous with the ice shelf, lies Milne Glacier (Figs 1 and 2) which drains from a large inland ice cap. The glacier is 4 to 5 km wide, about 55 km long and flows from an elevation of 1000 m to sea-level in a valley fringed with mountains and nunataks. These characteristics are similar to those of Otto Glacier, 150 km to the south-west. Otto Glacier surged 5 to 6 km between 1950 and 1964, and was noted to be one of a number of possible surging glaciers in the region (Reference Hattersley-SmithHattersley-Smith, 1964, Reference Hattersley-Smith1969). Observations made in spring 1983 indicate that Milne Glacier has advanced rapidly during the past 15–25 years. Evidence of surge behavior is presented in this paper.

Fig. 1. Milne Glacier and Milne Ice Shelf, 1959 and 1983. The glacier advance has halved the area of the inner unit of Milne Ice Shelf. The area immediately in front on the glacier had a very rough irregular surface in 1983 whilst the remainder of the inner unit was essentially flat. Stratified water (fresh water overlying sea-water) in the small inlet, indicates that much of the Milne Glacier tongue is floating upon sea-water.
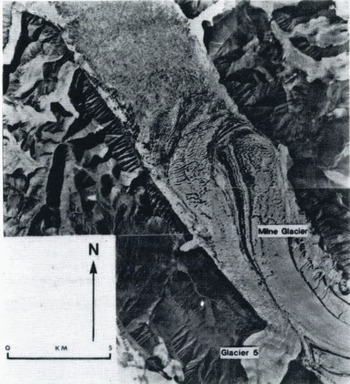
Fig. 2. 1959 air-photomosaic of Milne Glacier and the inner unit of Milne Ice Shelf. Note the corrugated surface topography of the inner ice shelf unit. Unlike the outer ice shelf there is no preferred orientation to this undulating surface. The mature developments of a "rolling" topography has probably been inhibited by periodic surges of Milne Glacier. The west glacier tongue has an undulating surface of parallel ridges and troughs similar to other ice shelves whilst the east tongue has an irregular surface drainage pattern.
Milne Ice Shelf and Milne Glacier, 1959 to 1983
A Canadian National Topographic Survey map (340E & 340H) of Milne Ice Shelf and Milne Glacier (Fig. 1) was produced with the aid of air photographs taken in July 1959 (Fig. 2). At that time the glacier front adjoined the ice shelf although ice wasting and disintegration had created two areas of lake ice along the east and west shores (Fig. 1). The lower 13 km of Milne Glacier consists of three ice streams. The east tongue is composed of ice from the main valley glacier plus that of a tributary glacier which flows into the north side of the valley 19 km from the ice front. Ice issuing from Glacier 5 creates a west tongue. A number of features on the surface of Milne Glacier are suggestive of past surge behavior: 1) contorted moraines, 2) moraine-covered stagnant ice at the terminus, and 3) irregular surface drainage, especially on the east tongue. Ice thickness measurements were undertaken by radio echo-sounding in April 1966 (Reference Hattersley-Smith, Hattersley-Smith, Fuzesy and EvansHattersley-Smith and others, 1969). At the glacier front a sharp transition in thickness from 60 m to 130 m was interpreted as a surface step between the two ice tongues. From there to the grounding line, about 8.5 km up-glacier, the ice thickness was between 120 m and 130 m. Thereafter the ice thickened to about 800 m at 55 km.
During a flight along Milne Fiord in late April 1983 a conspicuous pressure ridge was observed across the south end of the ice shelf. The pressure ridge, and by implication the glacier front, was a considerable distance beyond the 1959 glacier front suggesting Milne Glacier had recently advanced. Ground observations of the inner unit of Milne Ice Shelf and Milne Glacier were made in May 1983. The advance of the glacier had fractured and buckled the relatively thin shelf ice (≤ 20 m, personal communication from B. Prager), and it was not possible to approach the pressure ridge. Similar ridges up to 5 m high existed along the west shore. Beyond the shoreline pressure ridges there were numerous large, conical mounds of moraine upon the glacier.
Discussion
During the 24 year period 1959 to 1983 Milne Glacier advanced an estimated 4.25 km; a mean annual rate of advance of 177 m a−1. Since Reference Hattersley-Smith, Hattersley-Smith, Fuzesy and EvansHattersley-Smith and others (1969) reported no signs of recent glacier activity it is assumed that the glacier surged during the 17 year period 1966 to 1983; a mean annual rate of 250 m a−1. Such velocities are quite remarkable for a High Arctic glacier. If, as Reference Meier and PostMeier and Post (1969) suggest, the active surge phase lasts two to three years, Milne Glacier perhaps advanced at a mean rate of > 2 km a−1 over an unidentified two-year period.
The moraines on the surface of Milne Glacier (Fig. 2) do not appear as smooth lines more or less parallel to the valley walls (Reference PatersonPaterson, 1981, p. 282). Although these moraines are not as looped and folded as those of Tikke and Susitna Glaciers (Reference Meier and PostMeier and Post, 1969) they are certainly deformed. The deformation must be due to the flow of ice from Glacier 5 (Figs 1 and 2) forming loops when the main valley glacier is quiescent. When the latter surges the loop is carried forward some distance. A new loop forms after the surge. Reference Robin and BarnesRobin and Barnes (1969) proposed that the surge of one glacier stream can trigger a tributary glacier surge due to a type of stress instability. It is suggested that a surge of the main valley glacier induces surges of Glacier 5. It is noted that the moraines have been forced over to the east side of the fiord by the Glacier 5 ice stream. Furthermore, as the Glacier 5 ice has come up against Milne Ice Shelf it has been deflected across the east ice tongue so that the moraines have been rotated through 90°. The deflection of the west tongue across the front of the east tongue would explain the surface step mentioned previously. There are at least three moraine loops at the glacier front. This has also been noted as diagnostic of surging glaciers (Reference Meier and PostMeier and Post, 1969). A little to the east of Glacier 5 is a very angular moraine loop (Fig. 2) indicating a possible surge of another tributary glacier.
It has already been noted that the moraines are underlain by stagnant ice. At the time of the surge the ice was probably heavily crevassed and broken but since then it has become smooth and subdued as a result of heavy ablation. Ward Hunt Ice Shelf (Fig. 1), 80 km north-north-east of Milne Fiord, has undergone a long period of net ablation since at least 1906 (Reference Hattersley-Smith and SersonHattersley-Smith and Serson, 1970) thus it is likely that Milne Ice Shelf and Milne Glacier have also. The smooth appearance of the surface of Milne Glacier in 1983 suggests that the surge occurred some years previously; in the late 1960s or early 1970s.
Reference KoernerKoerner (1979) has shown the coast of northern Ellesmere Island to have higher accumulation rates than the higher inland ice caps. However, although the ice shelves and near-coast glaciers have relatively high accumulation rates there is a net loss of ice each year. Conversely, the lower accumulation inland is preserved as firn or superimposed ice. Though the accumulation rates are low there will be a gradual increase of ice volume in the “reservoir area” of a surging glacier. Although the formation of superimposed ice with consequent release of heat will affect the ice temperature distribution to some extent, it is believed that Milne Glacier, like Otto Glacier, is a cold glacier. At an elevation of 1040 m on Gilman Glacier (180 km south-east of Milne Glacier) the 10 m ice temperature was −18.5°C. (Reference Hattersley-SmithHattersley-Smith, 1960). Thermocouples installed in bore holes on Ward Hunt Ice Shelf in 1982 and 1983 revealed 10 m ice temperatures of −15°C. Surging in cold glaciers has been explained by creep instability warming the basal ice to the melting point allowing the glacier to slide (Reference RobinRobin, 1955). For a glacier to have a cold surface and near-temperate bed, the ice thickness, ice temperature, and geothermal flux must be fortuitously related (Reference ClarkeClarke, 1976). Such a situation would explain the thermal regulation of Milne Glacier surges. It is further believed that the glacier has a long surge-cycle period; as accumulation rates are low it will take some considerable time to attain a critical condition in the “reservoir area”.
Acknowledgements
I wish to thank the following for their generous financial and logistic support of my work on the north coast of Ellesmere Island; Polar Continental Shelf Project, Dome Petroleum Ltd., Gulf Canada, Arctic Acoustics Section of the Defence Research Establishment Pacific, the Arctic Institute of North America, and the University of Calgary. Dr G.K.C. Clarke, Dr G. Holdsworth, and Dr S.A. Harris kindly read the manuscript. Harold Serson provided invaluable assistance and companionship in the field.