Introduction
Durum wheat (Triticum durum) is a crop mainly cultivated for the production of pasta and semolina. A high level of proteins confers properties to the grains that are valued for the transformation process in the pastry industry (Bushuk, Reference Bushuk1997). The percentage of proteins in grains is enhanced by increased N fertilization (Daniel and Triboi, Reference Daniel and Triboi2000). On the other hand, farmers are concerned by the constraints inherent to environmental issues. In Europe, the Nitrates Directive aims at protecting ground and surface water from nitrate pollution (Monteny, Reference Monteny2001). To meet both industrial and environmental requirements, complementary processes have to be developed to better manage crop nutrition.
New strategies such as the use of biological molecules that act as biostimulants have been proposed. Yakhin et al. (Reference Yakhin, Lubyanov, Yakhin and Brown2017) defined biostimulants as ‘a formulated product of biological origin that improves plant productivity as a consequence of the novel or emergent properties of the complex of constituents, and not as a sole consequence of the presence of known essential plant nutrients, plant growth regulators, or plant protective compounds’. They are also able to increase the plant nutrient use efficiency and tolerance to abiotic and biotic stresses (Colla et al., Reference Colla, Nardi, Cardarelli, Ertani, Lucini, Canaguier and Rouphael2015a; Nardi et al., Reference Nardi, Pizzeghello, Schiavon, Ertani, Nardi, Pizzeghello, Schiavon and Ertani2016; Tanou et al., Reference Tanou, Ziogas and Molassiotis2017). In addition, they can enhance the effectiveness of conventional mineral fertilizers (Craigie, Reference Craigie2011; Bulgari et al., Reference Bulgari, Cocetta, Trivellini, Vernieri and Ferrante2015). Biostimulants are available in a variety of formulations and originating from different organic materials. They include humic substances, complex organic materials, beneficial chemical elements, peptides and amino acids, inorganic salts, seaweed extracts, chitin and chitosan derivatives, antiperspirants and other N-containing substances (Nardi et al., Reference Nardi, Pizzeghello, Schiavon, Ertani, Nardi, Pizzeghello, Schiavon and Ertani2016). Among these categories, substances extracted from seaweeds are the most frequently studied, and fungi extracts are receiving increasing attention (du Jardin, Reference Nardi, Pizzeghello, Schiavon, Ertani, Nardi, Pizzeghello, Schiavon and Ertani2015).
Studies on the effect of seaweed extracts have shown that they can improve growth in treated grapevine, strawberry, Arabidopsis thaliana and rapeseed (Mancuso et al., Reference Mancuso, Azzarello, Mugnai and Briand2006; Rayorath et al., Reference Rayorath, Jithesh, Farid, Khan, Palanisamy, Hankins, Critchley and Prithiviraj2008; Roussos et al., Reference Roussos, Denaxa and Damvakaris2009; Jannin, Reference Jannin2012). In the case of grapevine, marine bioactive substances induced a higher capacity to accumulate macronutrients, especially in leaves. Moreover, it helped plants to better resist water stress, maintaining a higher leaf water potential and stomatal conductance (Mancuso et al., Reference Mancuso, Azzarello, Mugnai and Briand2006). Enhanced root length and increased nitrate reductase activity have been reported in A. thaliana (Durand et al., Reference Durand, Briand and Meyer2003; Rayorath et al., Reference Rayorath, Jithesh, Farid, Khan, Palanisamy, Hankins, Critchley and Prithiviraj2008). Nitrogen uptake was stimulated by seaweed extract application on rapeseed (Jannin et al., Reference Jannin, Arkoun, Etienne, Laîné, Goux, Garnica, Fuentes, Francisco, Baigorri, Cruz, Houdusse, Garcia-Mina, Yvin and Ourry2013).
Fungal bioactive substances released by Trichoderma can supply nutrients to the host plant (Behie and Bidochka, Reference Behie and Bidochka2014). These substances also displayed biopesticidal and biocontrol capacities (Mukherjee et al., Reference Mukherjee, Horwitz, Herrera-Estrella, Schmoll and Kenerley2013; Nicolás et al., Reference Nicolás, Hermosa, Rubio, Mukherjee and Monte2014). Trichoderma application to vegetable crops increased tolerance to abiotic stress (Shoresh et al., Reference Shoresh, Harman and Mastouri2010), nutrient use efficiency and organ growth (Colla et al., Reference Colla, Rouphael, Di Mattia, El-Nakhel and Cardarelli2015b). The release of active metabolites by Trichoderma improved water and nutrient uptake capacity, thereby having an effect on abiotic stress tolerance, plant yield and growth (López-Bucio et al., Reference López-Bucio, Pelagio-Flores and Herrera-Estrella2015).
The effect of marine and fungal biostimulants depends on many factors such as the species or experimental conditions (Faessel et al., Reference Faessel, Gomy, Nassr, Tostivint, Hipper and Dechanteloup2014). Although marine and fungi biostimulants are widely studied for their effect on yield and plant growth (Khan et al., Reference Khan, Rayirath, Subramanian, Jithesh, Rayorath, Hodges, Critchley, Craigie, Norrie and Prithiviraj2009; Hermosa et al., Reference Hermosa, Viterbo, Chet and Monte2012; Latique et al., Reference Latique, Elouaer, Chernane, Hannachi and Elkaoua2014), very few studies refer to their specific effect on durum wheat crops. Moreover, mechanisms involved in the effects of biostimulants on N nutrition and fluxes within the plants are poorly understood (Calvo et al., Reference Calvo, Nelson and Kloepper2014).
Brown and Saa (Reference Brown and Saa2015) assumed that biostimulants would reduce negative plant response to stress by interacting with plant signalling processes. Indeed, algae and their extracts can be used in crop management to increase abiotic and biotic stress resistance (Sharma et al., Reference Sharma, Fleming, Selby, Rao and Martin2014). Moreover, Trichoderma induces plant defense responses under stress conditions (Mastouri et al., Reference Mastouri, Björkman and Harman2012; Contreras-Cornejo et al., Reference Contreras-Cornejo, López-Bucio, Méndez-Bravo, Macías-Rodríguez, Ramos-Vega, Guevara-García and López-Bucio2015).
The objective of the current study was to quantify the effect of two biostimulants (marine, DPI4913, and fungal, AF086) used in foliar application on durum wheat, on important agronomic traits including yield and grain N relationships. Impacts on protein composition of these biostimulants have recently been described by Pichereaux et al. (Reference Pichereaux, Laurent, Gargaros, Viudes, Durieu, Lamaze, Grieu and Burlet-Schiltz2019). The study, performed in greenhouse, has shown that DPI4913 and AF086 treatments promote grain yield while maintaining protein concentration in grains, and positively affect protein composition in terms of grain quality. The current study aims at determining if these biostimulants could be used to optimize durum wheat production in the field. We also attempt to elucidate the impact of the biostimulants on N absorption and N fluxes within the plant, especially towards grains in greenhouse experiments. To our knowledge, only a few studies have focused on the impact of biostimulants on N use efficiency at the whole plant scale.
Materials and methods
Experimental designs
A first field experiment (Experiment 1) was conducted from October 2014 to July 2015 in Saint-Sulpice, France (43°33′N, 1°27′E, 200 m a.s.l.). Durum wheat var. Miradoux was sown at a seed rate of 300 seeds/m2 in a silty, clayey and sandy soil (pH 8.0, 41.1% silt, 32.9% clay, 26.0% sand 19.5 g/kg organic matter). N fertilization was designed to follow conventional farming practices. A quantity of 180 kg N/ha was supplied in four applications: the first in February at the end of the tillering stage (granules of ammonium nitrate: 50 kg N/ha); the second at the first node stage (granulated nitrogen–sulphur fertilizer: 40 kg N/ha); the third at the second node stage (granules of ammonium nitrate: 60 kg N/ha) and the fourth at the fully-emerged flag-leaf stage (granules of ammonium nitrate: 30 kg N/ha). The experiment used a completely randomized design, and each treatment was replicated ten times.
Another field experiment (Experiment 2) was conducted from October 2015 to July 2016 in Mervilla, France (43°50′N, 1°47′E, 270 m a.s.l.). The durum wheat var. Anvergur was sown at the rate of 300 seeds/m2 in a silty, clayey and sandy soil (pH 8.2, 37.6% silt, 32.0% clay, 30.4% sand and 9.7 g/kg organic matter). N (200 kg N/ha) was supplied in three applications: the first at the start of stem elongation (granulated nitrogen–sulphur fertilizer: 65 kg N/ha); the second at the first node stage (granules of perlurea fertilizer: 85 kg N/ha) and the third at the visible flag-leaf stage (granules of ammonium nitrate: 50 kg N/ha). The experiment used a split-plot design in which biostimulant treatments were randomized on the plots and each treatment was replicated eight times. Plot dimensions were 2 by 4 m.
A greenhouse experiment was carried out from January to June 2016 in Toulouse, France (43 °52′N, 1 °50′E, 146 m a.s.l.). Seeds of the durum wheat var. Anvergur were germinated in plastic cups filled with sand for 1 week in a growth chamber (25 °C/20 °C day/night, light intensity of 200 μmol/m2/s PAR, photoperiod of 12 h) and then for 2 weeks in the greenhouse (20 °C > T > 10 °C, ambient light, fertigated with a modified Coïc-Lesaint solution). The nutrient solution composition was as follows: 9.03 mm NO3−, 1.25 mm NH4+, 0.88 mm PO43−, 3.49 mm K+, 2.70 mm Ca2+, 0.96 mm Mg2+ and 0.96 mm SO42−. Seedlings were transferred to 2-litres plastic pots containing 2.2 kg of sandy soil (pH 5.0, 86.4% sand, 10.6% silt, 3.0% clay, 51.7 g/kg organic matter). Each pot contained four one-tiller plants and received 50 ml of nutrient solution two to three times a week depending on plant needs.
The soil water retention capacity was determined as follows: five 2-litre pots were filled with soil saturated with water. After the complete percolation of free water, the soil water content reached field capacity. The soil samples were then weighed, placed in an oven at 105 °C for 48 h and then weighed again. The soil water content at field capacity was calculated as the difference between the two weights: 20.6%.
To investigate if biostimulants affect N nutrition under stressful as well as favourable environment, plants were subjected to two water treatments: standard irrigated conditions and water-stressed conditions. From the second node stage until harvest, pots were weighed three times a week and soil water content was adjusted to 75% of field capacity for the standard irrigated conditions and to 60% for the water-stressed conditions.
The greenhouse experiment used a randomized complete block design. Each block contained a complete set of treatments. The experiment was divided into eight blocks, each containing one replication of each treatment. One pot containing four plants is a replication.
Biostimulant treatments
The following products were tested: DPI4913 containing Ascophyllum nodosum extract and a mix of amino acids (5% weight/weight proteinogenic hydrophilic amino acids). Three treatments were compared: control (no foliar treatment), DPI4913 (foliar application at a rate of 1 litre/ha) and AF086 (foliar application at a rate of 5 litre/ha). Both DPI4913 and AF086 were provided by Agronutrition (nutritional supplements company, De Sangosse Group, Carbonne, France). Treatments were applied one time at the fully-emerged flag-leaf stage for the field experiment in Carbonne, and one time at the second node stage for the field experiment in Mervilla and the greenhouse experiment in Toulouse.
Plant labelling
In field Experiment 1, in order to estimate the potential of the plants to transfer soil mineral N to grains, the soil NH4+ and NO3− pool were labelled with 15NH4Cl and K15NO3, as previously described by Pornon et al. (Reference Pornon, Escaravage and Lamaze2007). Briefly, the labelling solution (2.4 litres, 2.38 mm NH4+ and 2.15 mm NO3−, 15N abundance of 99 atom%) was injected into the upper 15 cm of soil with a needle (24 injection points in 45 × 60 cm2 plots) at the fully-emerged flag-leaf stage. The amounts of 15N supplied to the plots were calculated so as to be able to detect the label after its dilution in the plant-soil system and were sufficiently low to avoid any meaningful modification of the total soil N (5% increase).
In the greenhouse, two plants per pot received 25 μl of Cl15NH4 solution (1.28 m abundance of 99 atom%) on the flag-leaf when it was fully emerged for one group of plants and at the flowering stage for another group of plants. The 15ClNH4 solution was deposited on the lower leaf surface using a micropipette. The deposit zone was gently rubbed with a brush beforehand to remove the hydrophobic cuticle, allowing for better absorption of the labelled solution in leaves. Tissues were sampled at harvest, which was performed at maturity.
Harvest
For both field experiments, harvest was performed at maturity with a plot combine-harvester (Delta plot combine, Wintersteiger, Austria). Grain yield was measured for each plot. Protein concentration was determined by spectroscopy on a sample of approximately 2 kg of grains using a grain analyzer (FOSS Infratec™1241, FOSS, Nanterre, France).
In the greenhouse, plants were harvested at maturity and divided into roots, grains, remaining ears (glumes and beards), flag leaves and remaining shoots (stems and leaves). They were then dried (60 °C for 48 h) for dry weight determination and subsequently ground into a fine powder for 15N and N analysis with a mass spectrometer (Isoprime™) coupled to an elemental auto-analyzer (EA 2000, EuroVector™, Manchester, UK). Natural 15N abundance was measured on four samples of each compartment from plants not exposed to 15N labelling. The amount of 15N (g) in samples was calculated as:

where Masssample is the dry mass of the sample (g dry weight); [N]sample is the N concentration (%) of the sample; A sample is the 15N abundance in the sample from 15N labelled plots and A natural is the 15N abundance in sample from unlabelled plants.
The flag-leaf of some plants was harvested when fully emerged, whereas for others, it was harvested at maturity. For both, the ears were harvested at maturity. Each plant organ (flag-leaf and ear) was dried (60°C for 48 h) for dry weight determination and then ground into a fine powder for N analysis (vario El cube elemental analyzer, Elementar, Langenselbold, Germany). N remobilization efficiency (NRE, proportion of N in the flag-leaf when fully emerged that is not present at harvest) was calculated using Eqn (1):

where N F is the amount of N in fully-emerged flag-leaf stage and N M is the amount of N in flag-leaf at maturity.
SPAD values of flag leaves were determined using a chlorophyll meter (Minolta SPAD-502) two to three times a week from flowering until complete senescence.
Statistical analysis
Data were analysed using R software (Free Software Foundation, Inc., Boston, MA, USA). Analysis of variances (ANOVAs) were followed by Tukey's tests.
Results
Field experiments
Grain yield and protein concentration
Both biostimulants lead to a small but non-significant increase in grain yield (DPI4913: +1.8 and +5.5%; AF086: +4.0 and +3.9%, in Experiments 1 and 2 (Table 1), respectively). Biostimulants had no effect on grain protein concentration (Table 1).
Table 1. Grain yield and protein concentration in wheat under different foliar treatments (field Experiment 1: FI1; field Experiment 2: FI2)
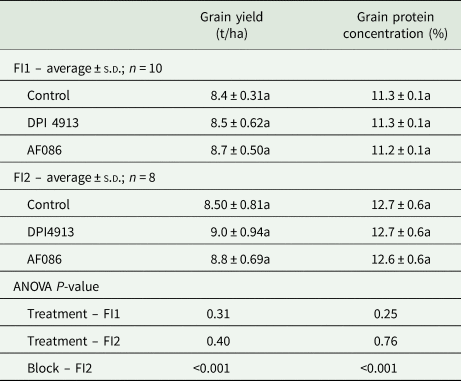
Note: Lower case letters indicate a statistical difference (P < 0.05) between treatments.
Accumulation in grains of 15N injected in soil as mineral N
Following injection of 15NH4+ and 15NO3− in the soil at the fully-emerged flag-leaf stage, the proportion of 15N recovered in grains, glumes and beards (GGB) at harvest was increased by 24.7% for the plants treated with DPI4913 (significant) and by 18.7% for the plants treated with AF086 (no significant) (Table 2).
Table 2. Proportion of 15N injected as 15NH4+ and 15NO3− into the soil at the fully-emerged flag leaf stage recovered in the ear at harvest (field experiment 1)

Lower case letters indicate a statistical difference (P < 0.05) between treatments.
Greenhouse experiment
Biomass in plant compartments
The plants bore only one ear each as they had only one tiller each. The water-restricted regime significantly decreased by 33.0% total dry biomass per plant (Table 3).
Table 3. Dry biomass of wheat compartments under different foliar treatments for two irrigation regimes (greenhouse experiment)

Note: Lower case letters indicate a statistical difference (P < 0.05) between treatments for a given irrigation level, whereas uppercase letters highlight a statistical difference between irrigation levels.
SI, standard irrigation; WL, water limitation.
For the standard irrigation regime, the total dry biomass per plant was significantly higher for plants treated with biostimulants (+19.7% for DPI4913 and +19.3% for AF086, Table 3) compared to the control. This was mainly due to significant effects on shoot dry biomass (+19.5% for DPI4913 and +17.8% for AF086), including significant higher grain dry biomass (+23.9% for DPI4913 and +20.4% for AF086). Although the effect was not significant, the mean values of root dry biomass were increased in the same proportion (+25.0% for DPI4913 and +32.1% for AF086).
Under water limitation, biostimulants had no significant effect on dry biomass. This treatment was not considered further.
Amount of nitrogen in the plant
The N amounts in plant compartments were considered in grains (G), in glumes and beards (GB), in ear (GGB), in shoots except ear (S) and in roots (R).
The DPI4913 treatment significantly increased the total amount of N in plants (+28.9%, Table 4) compared to the control. The increase for the AF086 treatment (+23.3%) was not significant. The proportion of N found in the ear (GGB) at harvest was significantly increased by 12.3% with DPI4913 and 8.0% with AF086. The proportion of N in roots was significantly lower for treated plants (DPI4913: −49.9%; AF086: −33.7%).
Table 4. Amount of nitrogen in plant and nitrogen distribution within plant compartments (greenhouse experiment)

Note: Lower case letters indicate a statistical difference (P < 0.05) between treatments.
G, grains; GB, glumes and beards; S, shoots except ear; R, roots.
Proportion of N in compartment ${\rm (\% )} = \;\displaystyle{{{\rm Amount}\;{\rm of}\;{\rm N}\;{\rm in}\;{\rm a}\;{\rm compartment}\;} \over {{\rm Total}\;{\rm amount}\;{\rm of}\;{\rm N}\;{\rm per}\;{\rm plant}}} \times 100$
For both biostimulants, the amount of N in grains per plant was significantly higher in treated plants than in the control (Table 5). The additional amount of N found in grains was 9.26 mg with DPI4913 (+25.1% compared to the control) and 9.50 mg with AF086 (+25.8% compared to the control). However, grain nitrogen concentration was not affected by the application of biostimulants.
Table 5. Nitrogen concentration and amount in grains per plant (greenhouse experiment)

Note: Lower case letters indicate a statistical difference (P < 0.05) between treatments.
Nitrogen remobilization
Dry biomass, N concentration and N amount in flag leaves showed a significant decrease between the fully-emerged flag-leaf stage and maturity: −9.9, −71.7 and −74.8%, respectively (Table 6).
Table 6. Flag leaf dry biomass, N concentration and N amount at two developmental stages: fully-emerged flag leaf and maturity (greenhouse experiment)

Note: Lower case letters indicate a statistical difference (P < 0.05) between treatments for a given sampling stage, whereas uppercase letters highlight a statistical difference between sampling stages.
FL, flag leaf.
At the fully-emerged flag-leaf stage, the flag-leaf dry biomass and amount of N were significantly increased in plants treated with DPI4913 (dry biomass: +22.7%; amount of N: +25.9%). Mean values were also increased by AF086 but this was not significant (dry biomass: +8.3%; amount of N: +5.7%). Flag-leaf N concentration was not affected by biostimulant application.
At maturity, flag-leaf dry biomass, N concentration and amount of N were not affected by biostimulants.
Flag-leaf NRE was calculated as the proportion of N in the flag-leaf at the fully-emerged stage that is not present in the flag-leaf at maturity (Eqn (1)). In control plants, this proportion was 68.2%. It was slightly higher for AF086 (+69.6%) and for DPI4913 (+79.6%) but this was not significant (P-value = 0.12).
Flag-leaf endogenous N was labelled with 15NH4+ at the fully-emerged stage. The proportion of 15N supplied to the flag-leaf and still present in this leaf at harvest was around 2%, and the proportion of 15N recovered in the rest of the plant was between 52.0 and 64.4%, depending on the treatment. This proportion was significantly enhanced by 23.9% for plants treated with DPI4913 (Table 7). The mean 15N value was increased by 15.6% with AF086 but the difference was not significant.
Table 7. Proportion of 15N applied on the flag leaf at the fully-emerged flag leaf stage recovered in total plant, in grains and remaining in the flag leaf at harvest (greenhouse experiment)

Note: Lower case letters indicate a statistical difference (P < 0.05) between treatments.
Following 15N labelling of the flag-leaf, 78.6% of the 15N recovered in the rest of the control plants at maturity was found in the grains. This proportion was increased by DPI4913 (+28.4%) and AF086 (+15.5%), but this was not significant (P-value = 0.09).
When 15N labelling of the flag-leaf was performed at flowering, the proportion of 15N retained by the flag-leaf at maturity was significantly enhanced compared to labelling performed at an earlier developmental stage (3.9 times higher). The labelling stage had a slight effect on the proportion of 15N recovered in grains at harvest (see below). Biostimulants had no effect on the proportion of 15N remaining in the flag-leaf (control: 7.06%; DPI4913: 7.46% and AF086: 6.75%) or on the proportion recovered in the grains (control: 41.09%; DPI4913: 44.04% and AF086: 34.44%) at harvest.
Flag-leaf senescence
DPI4913 and AF086 significantly reduced SPAD values in the flag leaves of treated plants compared to the control during the grain filling period (Fig. 1). For the control treatment, SPAD values declined from 24 days after anthesis until maturity. Decline began earlier for plants treated with DPI4913 (16 days after anthesis) and AF086 (9 days after anthesis). Photosynthesis was altered in leaves with SPAD values lower than 15 (data not shown), which occurred earlier for DPI4913 and AF086 than for the control. During the later filling stage, DPI4913 and AF086 treatments reduced SPAD values and shortened the duration of the photosynthetic function in flag leaves.

Fig. 1. Changes in SPAD values in flag leaves after anthesis under different treatments (greenhouse experiment). Biostimulants were applied at the second node stage.
Flag-leaf contribution towards grain filling
Flag-leaf removal at the fully-emerged stage significantly reduced by 16.0% grain dry biomass per ear (Table 8). The amount of N per ear was also significantly decreased by 15.3% (5.92 mg). Grain N concentration was not affected by flag-leaf ablation.
Table 8. Grain dry biomass and amount of N per plant, and N concentration at maturity, for two flag leaf removal stages: fully-emerged flag leaf and maturity (greenhouse experiment)

Note: Lower case letters indicate a statistical difference (P < 0.05) between treatments.
Discussion
Results on the improvement in growth, nutrient absorption, stress tolerance and crop quality by the application of biostimulants are inconsistent in the literature. The positive effects of biostimulants depend on many factors such as variety, environmental conditions, application conditions (quantity, foliar or root application), plant development stage, product formulation and storage conditions (Faessel et al., Reference Faessel, Gomy, Nassr, Tostivint, Hipper and Dechanteloup2014). To our knowledge, many studies have considered biostimulant effects on soft wheat but only a few on durum wheat.
In field experiments, no significant effects of biostimulants were observed on grain yield and protein concentration. However, mean values of grain yield were higher for plants treated by biostimulants (Experiment 1: +1.8% for DPI4913 and +4.0% for AF086, Experiment 2: +5.5% for DPI4913 and +3.9% for AF086). Variability between plots (soil and climatic conditions) may have had a greater impact than the use of biostimulants. In the same way, Al Majathoub (Reference Al Majathoub, Cantero-Martinez and Gabiña2004) observed that foliar application of four types of biostimulants (micronutrients, humic acid, seaweed extracts and amino acids) in a field experiment systematically affected plant growth and wheat yield, although this was only significant for the seaweed extract. Thereafter, only experiments in greenhouse are considered.
Biostimulants increased biomass and the amount of N in grains per plant but did not affect N grain concentration
In the greenhouse experiment under a standard irrigation regime, biostimulants significantly improved total dry biomass. This was mainly due to the increase in grain biomass. Indeed, a significant effect of biostimulants on grain yield was shown (+23.9% for DPI4913 and +20.4% for AF086). Grain biomass increase contributed to 50.9% of the biomass increase for plants treated with DPI4913 and to 44.2% for plants treated with AF086. This is consistent with the results observed by Rathore et al. (Reference Rathore, Chaudhary, Boricha, Ghosh, Bhatt, Zodape and Patolia2009) for soybean, by Jannin et al. (Reference Jannin, Arkoun, Etienne, Laîné, Goux, Garnica, Fuentes, Francisco, Baigorri, Cruz, Houdusse, Garcia-Mina, Yvin and Ourry2013) for rapeseed and by Polo and Mata (Reference Polo and Mata2018) for gold cherry tomato.
No significant effect was reported on grain N concentration. However, since the grain biomass was higher for plants treated with biostimulants, the amount of N in grains per plant increased (+25.1% for DPI4913 and +25.8% for AF086).
Biostimulants did not display any effect under water limitation
Although biostimulants had an effect on grain yield under standard water conditions (greenhouse experiment), no effect of biostimulant application appeared under water limitation. Our results do not agree with the prior suggestions that A. nodosum and Trichoderma extracts enhance stress tolerance in plants (Calvo et al., Reference Calvo, Nelson and Kloepper2014; López-Bucio et al., Reference López-Bucio, Pelagio-Flores and Herrera-Estrella2015).
After DPI4913 and AF086 treatment, the highest variations in grain protein abundances were found for proteins involved in grain technological properties but also in stress responses with the over-representation of proteins implied in biotic and abiotic stress defense (Pichereaux et al., Reference Pichereaux, Laurent, Gargaros, Viudes, Durieu, Lamaze, Grieu and Burlet-Schiltz2019). The surprising absence of effect of biostimulant application under water limitation may have been a result of the extent and timing of the stresses imposed in this experiment.
Effect of biostimulants on the total amount of N contained per plant (greenhouse experiment)
Because the total amount of N in plants at maturity was significantly improved (28.9% with DPI4913 and 23.3% with AF086), it is suggested that biostimulants have a positive effect on N net uptake by plants, as observed for the application of marine biostimulants on vines (Mugnai et al., Reference Mugnai, Azzarello, Pandolfi, Salamagne, Briand and Mancuso2007). This is strengthened by the results of our field experiment (Experiment 1) where 15NH4+ and 15NO3− ions were injected into the soil at the fully-emerged flag-leaf stage. The amount of 15N recovered in ears at maturity was significantly increased by 24.7% with DPI4913 and (non-significantly) increased by 18.7% with AF086. This revealed that DPI4913 and, to a lesser extent, AF086 increased the transfer to grains of soil N, suggesting improved root N uptake, at least from the fully-emerged flag-leaf stage to maturity. Billard et al. (Reference Billard, Etienne, Jannin, Garnica, Cruz, Garcia-Mina, Yvin and Ourry2014) also reported the positive effects of a biostimulant derived from algae on macronutrient uptake (N, S, K and P) for winter oilseed rape, as well as on root-to-shoot translocation of Fe and Zn. However, to our knowledge, little is known about biostimulant effects on N fluxes.
Effect of biostimulants on N allocation to the ear and on 15N remobilization from flag-leaf to grains
The proportion of N in the ear at maturity was higher for plants treated with biostimulants (+12.3% for DPI4913, +8.0% for AF086), whereas the proportion of N in other compartments was lower (shoots except for ear: −19.6% for DPI4913 and −12.4% for AF086; roots: −49.9% for DPI4913 and −33.7% for AF086). Nitrogen remobilization to the ear was thus improved by DPI4913 and AF086 application.
According to Gate (Reference Gate1995), the flag-leaf contributes to 24% of the N remobilization to the grains from flowering until harvest. To study the effect of biostimulants on N remobilization, the flag-leaf was labelled with 15NH4+ at the fully-emerged stage and at the flowering stage.
The labelling stage had a significant effect on the proportion of 15N remaining in the flag-leaf at maturity, but in both cases, most of the tracer supplied to the leaves was redistributed to other plant compartments at harvest. This indicates that foliar-applied N in flag leaves was very mobile.
Most of the 15N applied to the flag-leaf recovered in the rest of the plant was found in grains (79.6%). Biostimulants tend to increase the proportion of 15N applied to the fully-emerged flag-leaf that was recovered in the grains at harvest (+28.4% for DPI4913, +15.5% for AF086) and in the whole plant (+23.9% for DPI4913, +15.6% for AF086). When labelling was performed at the flowering stage, no biostimulant effect on 15N allocation to grains was observed, suggesting that biostimulants can enhance N mobility in the flag leaf at early stages of plant development but not at latter stage.
Remobilization
Senescence processes in wheat are associated with nutrient remobilization from senescing leaves to other organs (Gregersen et al., Reference Gregersen, Holm and Krupinska2008). The chlorophyll indexes obtained from anthesis to complete senescence have shown a premature loss of chlorophyll due to biostimulant application. In many instances, premature senescence is not a favourable outcome (Vilmus et al., Reference Vilmus, Ecarnot, Verzelen and Roumet2014). However, some authors suggest that accelerated senescence is correlated with an increased remobilization (Gaju et al., Reference Gaju, Allard, Martre, Le Gouis, Moreau, Bogard, Hubbart and Foulkes2014). Accordingly, we suggest that DPI4913 and AF086 could accelerate leaf senescence and thus remobilization, at least under our growing conditions (plants with a single tiller, a single ear). Whether this effect can appear in plants with more than a single tiller and ear must be considered.
Effect of removal of fully-emerged flag-leaf on the amount of N in grains at maturity
From the fully-emerged flag-leaf stage until harvest, the amount of N in the flag-leaf only decreased by 1.78 mg for the control, 2.50 mg for DPI4913 and 1.85 mg for AF086 treatments (Table 6). The amount of N in grains was approximately 37 mg and 54 mg per plant for the control and DPI4913, respectively. In terms of net N fluxes, flag-leaf N resorption cannot be a major source for N grain filling. The 15N labelling experiment suggests that the flag-leaf played a central role in plant N metabolism. In order to study the involvement of the flag-leaf in grain N filling other than through remobilization, flag-leaf ablation was performed when the leaf was fully emerged. This reduced the amount of N in grains per ear at maturity by 5.92 mg (Table 8). This demonstrated that flag leaves largely contribute to the mechanisms of grain N filling but not as a main N source, as observed by Harper et al. (Reference Harper, Sharpe, Langdale and Giddens1987). The increase in the amount of N in grains (and in the whole plant) resulting from biostimulant application was associated with enhanced labelled mineral N soil accumulated in the grains. On the one hand, biostimulants could have enhanced soil N uptake, resulting in enhanced plant growth but, on the other hand, biostimulants could have enhanced plant growth through increasing P or K or micronutrient uptake, resulting in enhanced soil N uptake.
Conclusion
This study provides clues about the mechanisms of action of DPI4913 and AF086 biostimulants on durum wheat, biostimulants that positively affect protein composition in terms of grain quality (Pichereaux et al., Reference Pichereaux, Laurent, Gargaros, Viudes, Durieu, Lamaze, Grieu and Burlet-Schiltz2019). Both biostimulants improved yield (grain biomass), and N recovery in whole plants at maturity was enhanced. However, the effect of the fungal biostimulant AF086 was less pronounced than that of the marine biostimulant DPI4913. A higher amount of N recovered in the crop would result in less N leaching if the same amount of N fertilizer was applied, positively impacting nitrate pollution issues. Because biostimulants increase the amount of N supplied to the flag-leaf recovered in grains at harvest, it would be relevant to evaluate the interest of the use of biostimulants in combination with foliar application of N on durum wheat to increase the protein concentration in the grain.
Acknowledgements
The authors acknowledge Pascal Tillard (BPMP) for 15N analysis, the Agronutrition experimentation team, Bernard Leguevaques, Denis Loubet, Michel Labarrère, Eric Lecloux, Sébastien Viudes, Bastien Dauphin and Diana Lezier for their technical assistance, as well as the Staphyt Company for harvesting the field. The authors thank Emilie Tierchant and Gail Wagman for proofreading the article in English.
Financial support
This study was part of the INNOPERF-BLE project, selected and supported by Pôle Agri-Sud-Ouest Innovation, and funded by the French FUI (Fond Unique Interministeriel), the Midi-Pyrénées Region and Bpifrance (Banque Publique d'Investissement). ANRT supported the PhD grant (CIFRE).
Conflict of interest
The authors declare that this research was conducted in the absence of any commercial or financial relationships that could be construed as a potential conflict of interest.
Ethical standards
Not applicable.