Introduction
Agricultural production on existing land must feed the world's population in a sustainable manner (Robertson and Swinton, Reference Robertson and Swinton2005; Fedoroff et al., Reference Fedoroff, Battisti, Beachy, Cooper, Fischhoff, Hodges, Knauf, Lobell, Mazur, Molden, Reynolds, Ronald, Rosegrant, Sanchez, Vonshak and Zhu2010). Ongoing research on integrated crop-livestock systems (ICLSs) throughout the world has shown promise for providing synergy between agricultural production practices and environmental quality (Herrero et al., Reference Herrero, Thornton, Notenbaert, Wood, Msangi, Freeman, Bossio, Dixon, Peters, van de Steeg, Lynam, Parthasarathy Rao, Macmillan, Gerard, McDermott, Seré and Rosegrant2010; Lemaire et al., Reference Lemaire, Franzluebbers, de Faccio Carvalho and Dedieu2014).
Weeds remain a major constraint to productivity and the increasing prevalence of herbicide-resistant weeds worldwide is alarming in terms of global food security, as the majority of agricultural systems rely heavily on herbicides for weed control (Busi et al., Reference Busi, Vila-Aiub, Beckie, Gaines, Goggin, Kaundun, Lacoste, Neve, Nissen, Norsworthy, Renton, Shaner, Tranel, Wright, Yu and Powles2013). Research is being conducted on a variety of cropping systems to develop more effective integrated weed management methods that are ultimately sustainable and reduce reliance on herbicides (Lechenet et al., Reference Lechenet, Deytieux, Antichi, Aubertot, Bàrberi, Bertrand, Cellier, Charles, Colnenne-David, Dachbrodt-Saaydeh, Debaeke, Doré, Farcy, Fernandez-Quintanilla, Grandeau, Hawes, Jouy, Justes, Kierzek, Kudsk, Lamichhane, Lescourret, Mazzoncini, Melander, Messéan, Moonen, Newton, Nolot, Panozzo, Retaureau, Sattin, Schwarz, Toqué, Vasileiadis and Munier-Jolain2017). Investigations into the effects of including livestock grazing in crop-based systems have demonstrated that, compared with conventional cropping systems, ICLSs can result in lower of weed infestation as well as lower costs and risks associated with herbicide usage (Tracy and Davis, Reference Tracy and Davis2009; Miller et al., Reference Miller, Menalled, Sainju, Lenssen and Hatfield2015; Lehnhoff et al., Reference Lehnhoff, Miller, Miller, Johnson, Scott, Hatfield and Menalled2017). However, these investigations revealed that, in some years, livestock led to an increase in weed infestation in arable lands (Miller et al., Reference Miller, Menalled, Sainju, Lenssen and Hatfield2015).
The effectiveness of grazing as a weed control method depends on animal forage selection, pasture type, grazing duration and herd size (Lacey and Sheley, Reference Lacey and Sheley1996; De Bruijn and Bork, Reference De Bruijn and Bork2006; Renne and Tracy, Reference Renne and Tracy2013; Lustosa et al., Reference Lustosa, Schuster, Martinichen, Pelissari and Gazziero2016). Additionally, many studies have demonstrated the benefits of diversified crop rotation for reducing weed infestation in arable crops (Cardina et al., Reference Cardina, Herms and Doohan2002; Bellinder et al., Reference Bellinder, Dillard and Shah2004; Hosseini et al., Reference Hosseini, Karimi, Babaei, Mashhadi and Oveisi2014). In ICLSs, these factors (i.e. grazing management and crop rotation) can occur over the same area at different spatial-temporal scales within a year. For example, the practice of using a field for grazing a grass cover crop in the winter and producing row crops the following summer is widespread in sub-tropical and temperate regions of the USA (Sulc and Franzluebbers, Reference Sulc and Franzluebbers2014), Brazil (De Moraes et al., Reference De Moraes, Carvalho, Anghinoni, Lustosa, Costa and Kunrath2014) and Australia (Nie et al., Reference Nie, McLean, Clough, Tocker, Christy, Harris, Riffkin, Clark and McCaskill2016); however, how grazing management and crop rotation collectively impact weed population dynamics (i.e. if their effects interact, are additive or neutralize one another) and affect weed outcomes (i.e. synergistically or antagonistically) in ICLSs is still unknown. The current study objectives were to assess the effects of crop rotation, forage allowance and animal stocking method on the seedbank and emerged weed flora in an ICLS. In addition, the ways in which these management practices differ in their effects on weed community composition were analysed. It was hypothesized that crop rotation and grazing management would have interactive effects on weed seedbank sizes and weed flora emergence in an ICLS and high forage allowance, rotational grazing and crop rotation collectively should result in lower weed flora density.
Materials and methods
Site and treatment descriptions
The current study was conducted in a long-term ICLS experiment under no-tillage management, located at the research farm of the Federal University of Rio Grande do Sul in Rio Grande do Sul State, Brazil (30°05′S, 51°39′W, 63 m a.s.l.). The study area is characterized by a marked seasonality of temperature and a homogeneous distribution of precipitation throughout the year (Neto et al., Reference Neto, Savian, Schons, Bonnet, do Canto, de Moraes, Lemaire and de Faccio Carvalho2014), and the summers are warm and humid (Cfa according to the Köppen classification system). The soil is classified as a Typic Paleudult (Soil Survey Staff, 1999); the soil within the 0–20 cm layer contained 15% clay and 20 g/kg organic matter and the pH was 4.87.
The experimental site covers a total area of 4.8 ha, and before 2003, native pasture (pampa rangeland) covered the experimental area. Beginning in 2003, the area was converted into no-tillage cropland and an ICLS experimental protocol was established that consisted of two growing seasons per year: (1) a winter season with cover crop pasture grazed by sheep from May until November and (2) a summer season with row crop production from November until April.
In March of 2003 and 2004, glyphosate was applied at 2400 g acid equivalent (ae)/ha to eliminate all vegetation prior to seeding Italian ryegrass (Lolium multiflorum Lam. cv. ‘Common’ at 45 kg seed/ha) for the winter grazing season. Since 2005, naturally reseeded Italian ryegrass has formed the winter pasture and the pasture establishment phase has occurred from late May until late June, which is the common practice for ICLSs in the region (Neto et al., Reference Neto, Savian, Schons, Bonnet, do Canto, de Moraes, Lemaire and de Faccio Carvalho2014). In early July, the stocking phase was initiated with the introduction of sheep for the winter grazing period. The ryegrass becomes reproductive and sheds seed at the end of each winter grazing period from late October through November. Treatment factors included stocking method (continuous or rotational), forage allowance (moderate or high) and summer cropping (continuous soybean or soybean-maize rotation). Treatments consisted of a 2 × 2 × 2 factorial in a randomized complete block design; four replicates were included, totalling 32 experimental units. The plot size was 1400/m2 (20 × 70 m2). The forage allowance treatments were defined as 2.5 times (moderate forage allowance) and 5 times (high forage allowance) the potential daily dry matter intake of lambs in accordance with the National Research Council (NRC) (1985), resulting in forage allowances values (Sollenberger et al., Reference Sollenberger, Moore, Allen and Pedreira2005) of 10 and 20 kg of forage dry matter per 100 kg animal live weight for the moderate and high forage allowance treatments, respectively. Calculations were made every 15 days to adjust the animal stocking and ensure 10 and 20 kg of forage dry matter per 100 kg animal live weight was maintained. For the continuous stocking treatment, the entire plot received three tester animals (animals that remained permanent throughout the grazing period) plus a variable number of animals adjusted periodically with put-and-take animals that were added or removed from the plot as required to maintain the desired forage allowance (biomass was approximately 1.8 ± 0.32 and 1.2 ± 0.38 t/ha for the high and moderate forage allowances, respectively). For rotational stocking, plots were divided into successive grazing tracts with an electric fence adjusted to maintain a minimum of three animals in each plot for the desired forage allowance. The length of the grazing cycle in each grazing tract was defined previously as a function of Italian ryegrass leaf lifespan (500 and 410 growing degree days [GDDs] in August and September–October, respectively; Pontes et al., Reference Pontes, Nabinger, de Faccio Carvalho, Da Trindade, Montardo and dos Santos2003) to ensure that leaf senescence was minimized in the grazing tract before the animals returned.
At the end of the winter season in late November, the pasture vegetation was treated with glyphosate (2400 g ae/ha), and the residual biomass was approximately 3.2 ± 0.37 and 1.8 ± 0.21 t/ha for the high and moderate forage allowance, respectively. The row crop treatments were sown at an inter-row spacing of 0.4 m in December of each year. Conventional non-transgenic soybeans were sown in the first 2 years of the experiment and a transgenic glyphosate-resistant cultivar was sown in subsequent years. A conventional non-transgenic maize hybrid was sown in the first 10 years of the experiment and a transgenic glyphosate-resistant hybrid was sown in subsequent years. In the first 2 years, the conventional soybeans received a post-emergence application of imazethapyr (150 g active ingredient (ai)/ha) and tepraloxydim (100 g ai/ha) in mid-January, and the conventional maize received a post-emergence application of tembotrione (100 g ai/ha) or nicosulfuron (60 g ai/ha) during the first 10 years. As a post-emergence herbicide, glyphosate at 2400 g ae/ha was applied to the transgenic glyphosate-resistant soybean and maize in mid-January. The application volume was 200 ± 50 l/ha and adjuvants were not used. Insecticides and fungicides were applied as needed in accordance with agronomic recommendations. Row crops were harvested at the end of May each year.
Seedbank sampling
Soil seedbanks were sampled before summer crop sowing in November 2014. A total of 28 soil cores were taken within the central 4 × 52 m2 area of each plot. The soil samples were collected manually at the intersections of a 4 × 4 m2 grid using a steel 4.2-cm-diameter probe inserted to a depth of 10 cm. Each core was divided into 0–5-cm- and 5–10-cm-depth segments.
The 28 soil cores for each sampling depth within each plot were bulked and mixed. The bulked samples for each plot (minus large stones and root fragments) were spread out in 48 × 38 cm2 plastic trays to record seedling emergence. The plastic trays were kept for 12 months in a greenhouse and the seedling emergence method (Thompson et al., Reference Thompson, Bakker and Bekker1997) was used to quantify readily (active) germinating seeds from the seedbanks. The measured active seedbank reflected the total viable seedbank closely, as confirmed by squeezing the non-germinated seeds recovered from two randomly chosen test trays; only 2% of the larger seeds remained firm when squeezed with forceps. Optimum soil moisture conditions in the trays were maintained by regular sub-irrigation (i.e. three times per week). In the winter, the lowest night-time temperature in the greenhouse was 10 °C and the maximum daytime temperature was 30 °C. Emerged seedlings were identified, counted and removed from the plastic trays weekly. A 2-week drought period was imposed in March 2015 to break seed dormancy (de Cauwer et al., Reference de Cauwer, van den Berge, Cougnon, Bulcke and Reheul2010). At the end of the drought period, the contents of the trays were stirred and sub-irrigation was reactivated. After seed emergence ceased, the samples were stirred and placed in a 4 °C cold room for 3 weeks followed by 1 week of alternating temperatures (15 and 4 °C) before being returned to the greenhouse (Cardina et al., Reference Cardina, Herms and Doohan2002). This process was repeated until no additional seedlings emerged.
Sampling of weed flora
In each field plot, emerged weed flora was determined at three times during each year: at the end of the grazing season (November 2014 and 2015), before herbicide applications for soybean or maize cultivation (mid-January 2015 and 2016) and after crop harvest (May 2015 and 2016). Emerged weed flora was determined just before the point when herbicides are typically applied or when other interventions for weed control occur in this type of ICLS (in this protocol, only herbicides were used to control weeds). At the end of the winter grazing season, the area was treated with glyphosate to introduce grain crops. Weeds were controlled during the summer crop growing season using herbicides to avoid weed interference and herbicides were also applied after harvest to ensure pasture establishment. In the central area (4 × 52 m2) of each plot, the emerged weed flora within 50 × 50 cm2 quadrats located at the intersections of a 4 × 10 m2 grid were counted and identified, resulting in ten sub-samples per plot. The weeds were identified in accordance with the methods of Kissmann and Groth (Reference Kissmann and Groth1997) and Lorenzi (Reference Lorenzi2006). The means of the ten sub-samples were used for statistical analysis in accordance with the suggestion of Onofri et al. (Reference Onofri, Carbonell, Piepho, Mortimer and Cousens2010) and the data were reported as the population density (weeds/m2) for each species.
Data analyses
Weed density was evaluated first using a global analysis of variance (ANOVA) with a generalized linear model; year, block, treatment (stocking method, forage allowance and crop rotation), sampling time (grazing, crop and harvest) and previous crop (maize or soybean) were designated as main effects. All interactions between the previous crop, year and sampling time with treatments were also tested. The previous crop was included because, in the maize-soybean rotation, weeds were sampled in both maize and soybean in two separate years. In the soybean-soybean rotation, weeds were sampled in the same crop in both years. Therefore, if the previous crop affected weed emergence, the analysis needed to control for crop effects v. rotational effects since they can be confounded. No significant interactions among treatment and year or the previous crop were detected in any of the cases, and a stepwise backward elimination of terms was used based on the Akaike Information Criterion (AIC) to confirm that the year and previous crop did not influence the weed density analysis. Sampling time exhibited considerable interactive effects with treatment, and the data were analysed separately for each time. Thus, for weed density at each time (i.e. grazing, crop and harvest) and for the weed seedbank size at each depth, ANOVA was conducted using linear mixed-effects models that out-performed other models; blocks were designated as random effects, whereas crop rotation, stocking method and forage allowance were designated as fixed effects. The statistical models were structured to include factorial interaction effects of a given order and all lower order effects and main effects contained within those interaction effects were also included (Piepho and Edmondson, Reference Piepho and Edmondson2018).
Analysis of species data introduces issues of non-independence and inference (species response may be due to direct effects of treatment or indirect effects of treatments mediated through effects on other species). Thus, treatment effects at the species level were detected using multivariate analysis of variance (MANOVA); the models included terms for a year and the previous crop (the reason for including the previous crop was explained previously) as well as their interactions with treatments. No significant interactions among treatments or between treatments and year or the previous crop were detected and multiple ANOVAs with a single treatment factor were performed for each species. The total weed density and species density data were log transformed to normalize the variances, and the normality and homogeneity were tested by using the Shapiro–Wilk test and the Bartlett test, respectively. Due to the large number of species (Table 1), the results presented at the species level in the tables are focused on the most prevalent species (relative density >0.5 plants/m2 for the emerged weed flora and >100 seeds/m2 for the seedbank) for which significant treatment effects were observed.
Table 1. Average density of seedbank and emerged flora (seeds at a 0–10-cm depth or plants/m2) of weed species present in an integrated crop-livestock system in southern Brazil
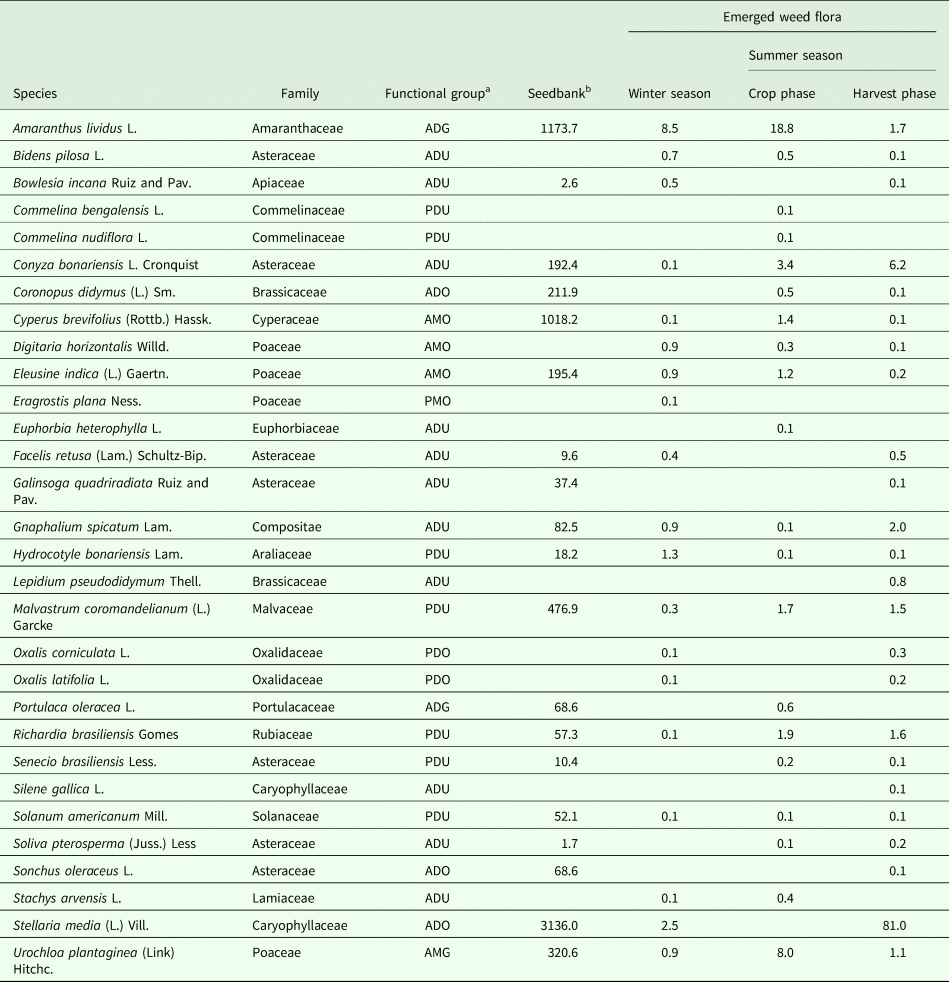
a A, annual; P, perennial; D, dicotyledonous; M, monocotyledonous; U, unpalatable by sheep; G, grazed by sheep; O, occasionally grazed by sheep.
b Blank fields indicate that no species were found.
The weed community composition and structure were calculated by considering all emerged weed flora (summing all sampling times of emerged weed flora, i.e. winter-grazed cover crop + summer crop and harvest phase) in order to represent the total weed community of the systems (rather than for each season). To test the effects of grazing management (forage allowance and stocking) and crop rotation on weed community composition, non-metric multidimensional scaling (NMDS) ordination was used for the visual representation of community differences. Bray–Curtis dissimilarity indices (Bray and Curtis, Reference Bray and Curtis1957) were calculated based on the following equation:

where BCjk is the dissimilarity between sites j and k; a ij and a ik are the relative species densities of species i at sites j and k, respectively; and S is the combined total density of the species in both communities. The data were log-transformed to de-emphasize the effect of dominant species using the following equation:

where T ij is the log-transformed density of species i in community j and M ij is the raw density of species i in community j (McKenzie et al., Reference McKenzie, Goosey, O'Neill and Menalled2016). Bray–Curtis dissimilarities were subjected to permutation-based multivariate analysis of variance (PerMANOVA), which included terms for blocks to test for grazing management (allowance and stocking) and crop rotation effects on the weed seedbank and emerged weed flora community composition (McCune et al., Reference McCune, Grace and Urban2002). The Shannon diversity index (H) of the weed seedbanks and emerged weed flora for each treatment were estimated using the following equation:

where N is the total number of individuals per plot, ni refers to the number of individuals per species per plot and S describes the total number of species. The evenness (J) of the species in each treatment was also calculated using the Shannon diversity index, where J = H/log2(s), as described by Hosseini et al. (Reference Hosseini, Karimi, Babaei, Mashhadi and Oveisi2014). The species richness, Shannon diversity and evenness data were subjected to ANOVA using linear mixed-effects models; blocks were designated as random effects, whereas crop rotation, stocking method, forage allowance and years were designated as fixed effects. Model selection was based on the AIC. The normality and homogeneity were tested by using the Shapiro–Wilk test and the Bartlett test, respectively. All analyses were conducted using R Version 3.1.0 (R Core Team, 2017).
Results
Weed seedbank size and vertical distribution
The weed seedbanks near the soil surface were affected by forage allowance but were similar among crop rotation and stocking treatments. Forage allowance affected the overall weed seedbank density at the 0–5-cm and 0–10-cm soil depths significantly (P < 0.05), while no significant interactions with crop rotation or stocking treatments were observed. However, no significant treatment differences were observed in the seedbank at the 5–10-cm soil depth. Compared with the moderate forage allowance (high stocking rates), high forage allowance (lower stocking rates) reduced the weed seed density. Furthermore, compared with moderate forage allowance, high forage allowance led to a 48% lower overall seedbank density in the top 5 cm of the soil (Fig. 1). No significant differences in the overall weed seedbank size were found for crop rotation or stocking treatments. At the species level, the total amount of seeds of some species was affected significantly by forage allowances at the 0–5-cm soil depth (P < 0.05; Table 2), but not at the 5–10-cm soil depth. The effects of forage allowance on the total amount of weed seeds appear to be driven by species-specific responses. An approximately ten-fold greater seed density for both Conyza bonariensis and Stellaria media was observed under moderate forage allowance than under high forage allowance. Other seedbank species (Table 1) were not affected by treatments.
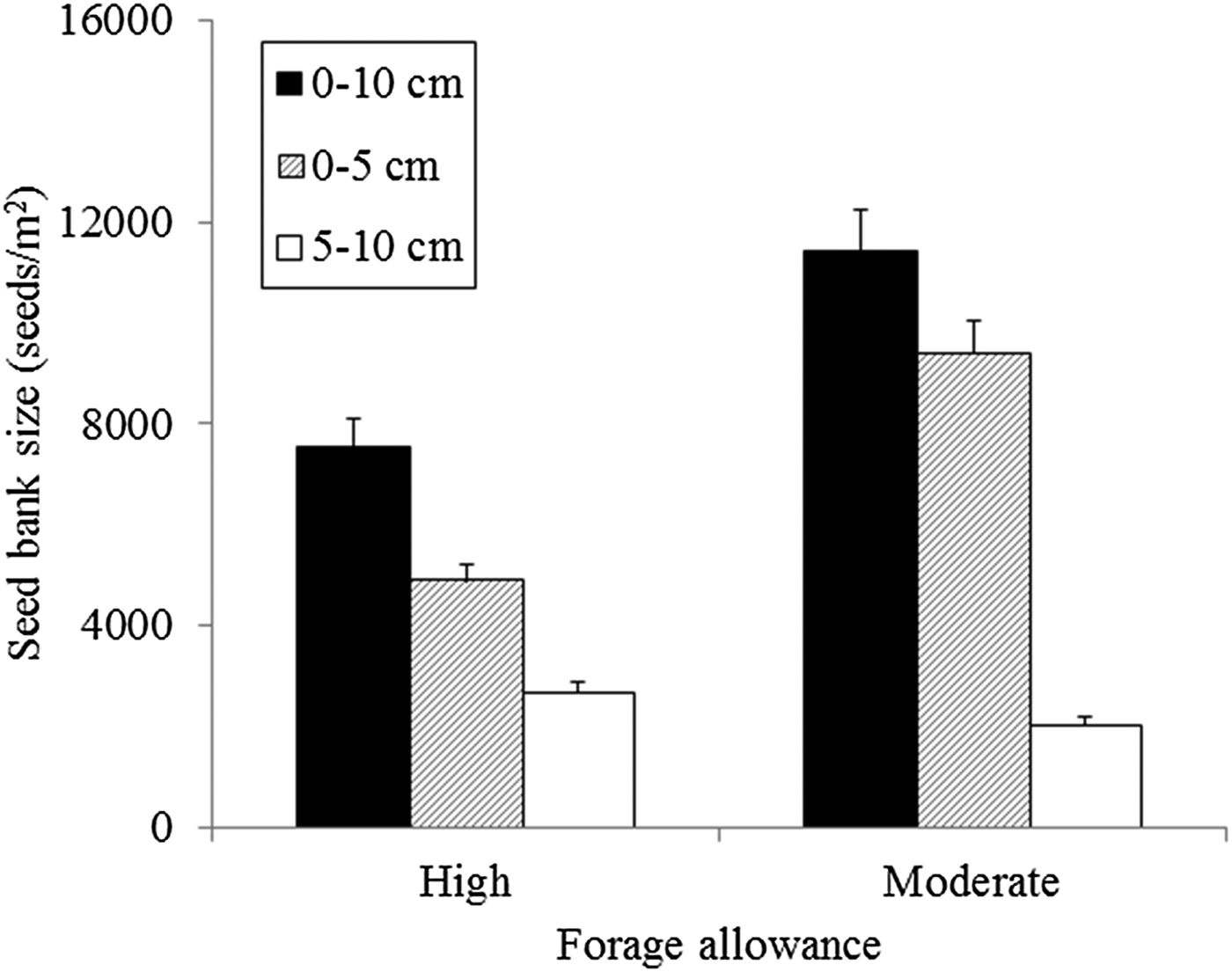
Fig. 1. Effects of forage allowance on changes in the total weed seedbank size (seeds/m2) in the 0–10-cm, 0–5-cm- and 5–10-cm soil layers after 12 years in an integrated crop-livestock system in southern Brazil. The vertical error bars represent the standard errors.
Table 2. Forage allowance effect on the species-level weed seedbanks (seeds/m2 ± s.e. at a depth of 0–5 cm) of an integrated crop-livestock system in southern Brazil

Winter season emerged weed flora
Forage allowance and stocking method affected the density of emerged weed flora in the winter grazing season (P < 0.001 and 0.01, respectively), but the summer crop rotation did not affect the emerged weed flora density in the grazing season. Significant interactive effects between forage allowance and stocking method (P < 0.05) indicated that continuous stocking with moderate forage allowance resulted in greater emerged weed flora than did the other treatments, whereas the emerged weed flora density under rotational stocking did not differ between high and moderate forage allowance (Fig. 2). Digitaria horizontalis and S. media plant densities were significantly greater (P < 0.05) under continuous stocking than under rotational stocking (Table 3). The weed species that emerged in the winter grazing season and that were significantly affected by forage allowance are shown in Table 3. For the majority of the weed species, high forage allowance resulted in lower emergence than did moderate forage allowance. However, the emergence of Amaranthus lividus showed the opposite effect of forage allowance.

Fig. 2. Effects of forage allowance and stocking method on emerged weed flora density (plants/m2) in the winter-grazed cover crop of an integrated crop-livestock system in southern Brazil. The vertical error bars represent the standard errors. CS, continuous stocking; RS, rotational stocking.
Table 3. Effects of forage allowance and stocking method on emerged weed flora (plants/m2 ± s.e.) in the winter season (grazing phase) of an integrated crop-livestock system in southern Brazil

Summer season emerged weed flora
During the summer (crop) phase, significant differences in total emerged weed flora densities were found only between forage allowance treatments (P < 0.01; Fig. 3); the differences were lower under the high forage allowance than under moderate forage allowance treatment. The species whose emerged weed flora densities were higher under high forage allowance than under moderate forage allowance included Malvastrum coromandelianum, Richardia brasiliensis and C. bonariensis (Table 4). Urochloa plantaginea plant densities were significantly greater (P < 0.01) under the soybean-maize rotation treatment than the continuous soybean cultivation treatment (Table 4).

Fig. 3. Effect of forage allowance on emerged weed flora density (plants/m2) in the summer season during the cropping phase of an integrated crop-livestock system in southern Brazil. The vertical error bars represent the standard errors.
Table 4. Effects of forage allowance and crop rotation on emerged weed flora (plants/m2 ± s.e.) in the summer season (crop growth phase) of an integrated crop-livestock system in southern Brazil

At the harvest phase of the summer season, the emerged weed flora density was significantly affected by all treatments (forage allowance, P < 0.001; stocking method, P < 0.001; and crop rotation, P < 0.05), and the forage allowance, stocking method and crop rotation treatments exhibited a triple interaction (P < 0.01; Fig. 4). Compared with moderate forage allowance, high forage allowance resulted in a lower emerged weed flora density. In addition, the moderate forage allowance resulted in higher emerged weed flora densities under rotational stocking than under continuous stocking, as well as under a maize-soybean summer crop rotation than under a soybean monoculture. The species affected by each treatment are shown in Table 5.
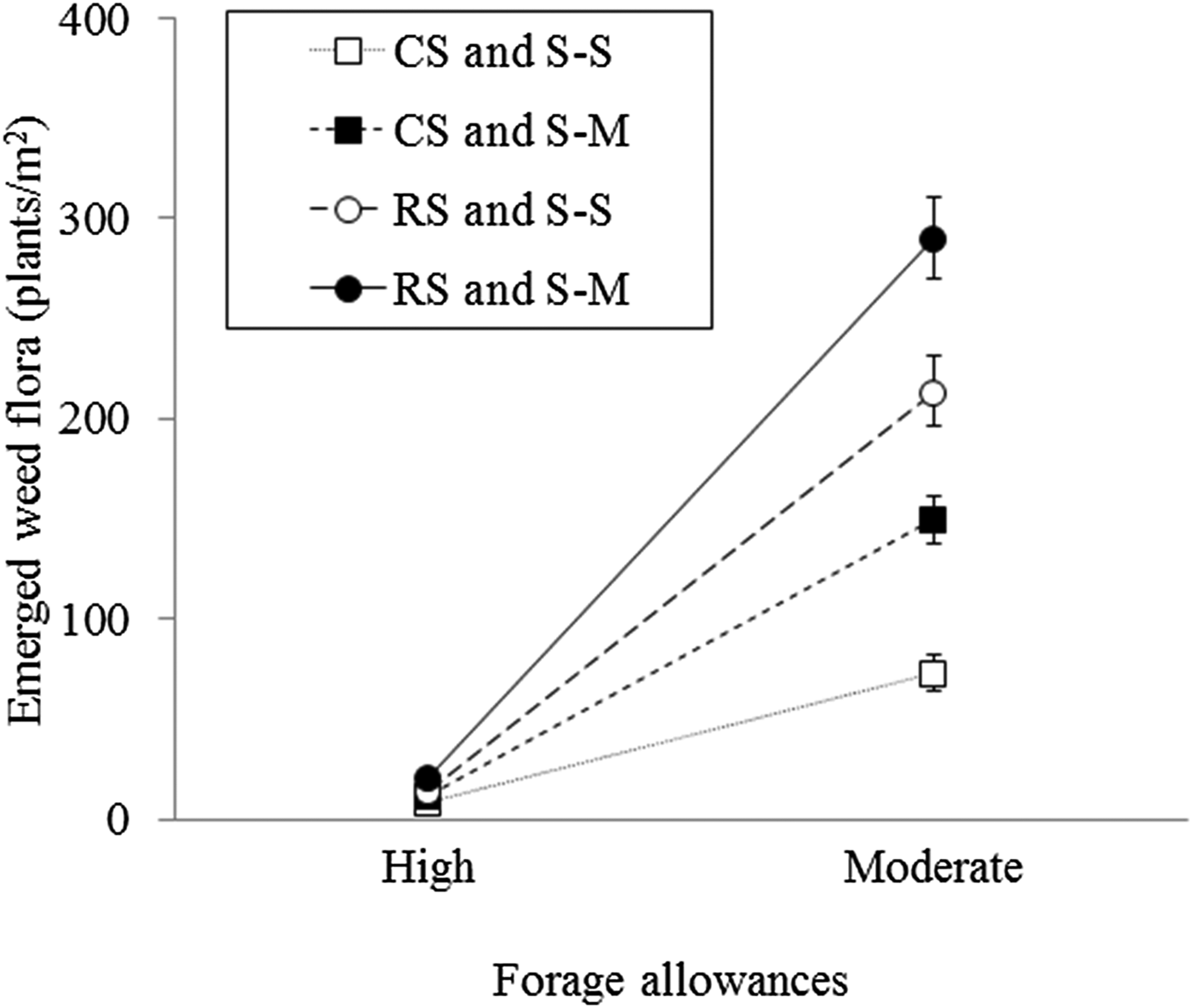
Fig. 4. Effects of stocking method, crop rotation and forage allowance on emerged weed flora density (plants/m2) in the summer season at the crop harvest phase of an integrated crop-livestock system in southern Brazil. The vertical error bars represent the standard errors. CS, continuous stocking; RS, rotational stocking; S–S, soybean–soybean; S–M, soybean–maize rotation.
Table 5. Effects of forage allowance, stocking method and crop rotation on emerged weed flora (plants/m2 ± s.e.) in the summer season (crop harvest phase) of an integrated crop-livestock system in southern Brazil

Weed community composition and structure in an integrated crop-livestock system
A total of 30 weed species representing 16 families (25 dicotyledonous and 5 monocotyledonous as well as 19 annuals and 11 perennials) were identified in the soil seedbank and in the emerged weed flora (Table 1). Amaranthus lividus L., Cyperus brevifolius (Rottb.) Hassk., and S. media (L.) Vill. dominated the soil seedbank and together accounted for 74.6% of the total. The dominant emerged weed flora included A. lividus during the winter and summer in the crop phase (45.9 and 47.4% of the emerged weed flora, respectively), and S. media was dominant during the summer in the harvest phase and comprised 82.3% of the total emerged weed flora (Table 2).
The results of the NMDS (Fig. 5) showed that a shift in the species composition of both the weed seedbank and emerged weed flora (winter + summer emerged weed flora) occurred in response to only the forage allowance factor via the dissimilarity matrix that was subjected to the PerMANOVA test (P < 0.001). No differences were found by the PerMANOVA test of the Bray–Curtis dissimilarity matrix regarding the weed seedbank and emerged weed flora species composition for crop rotation or stocking method.
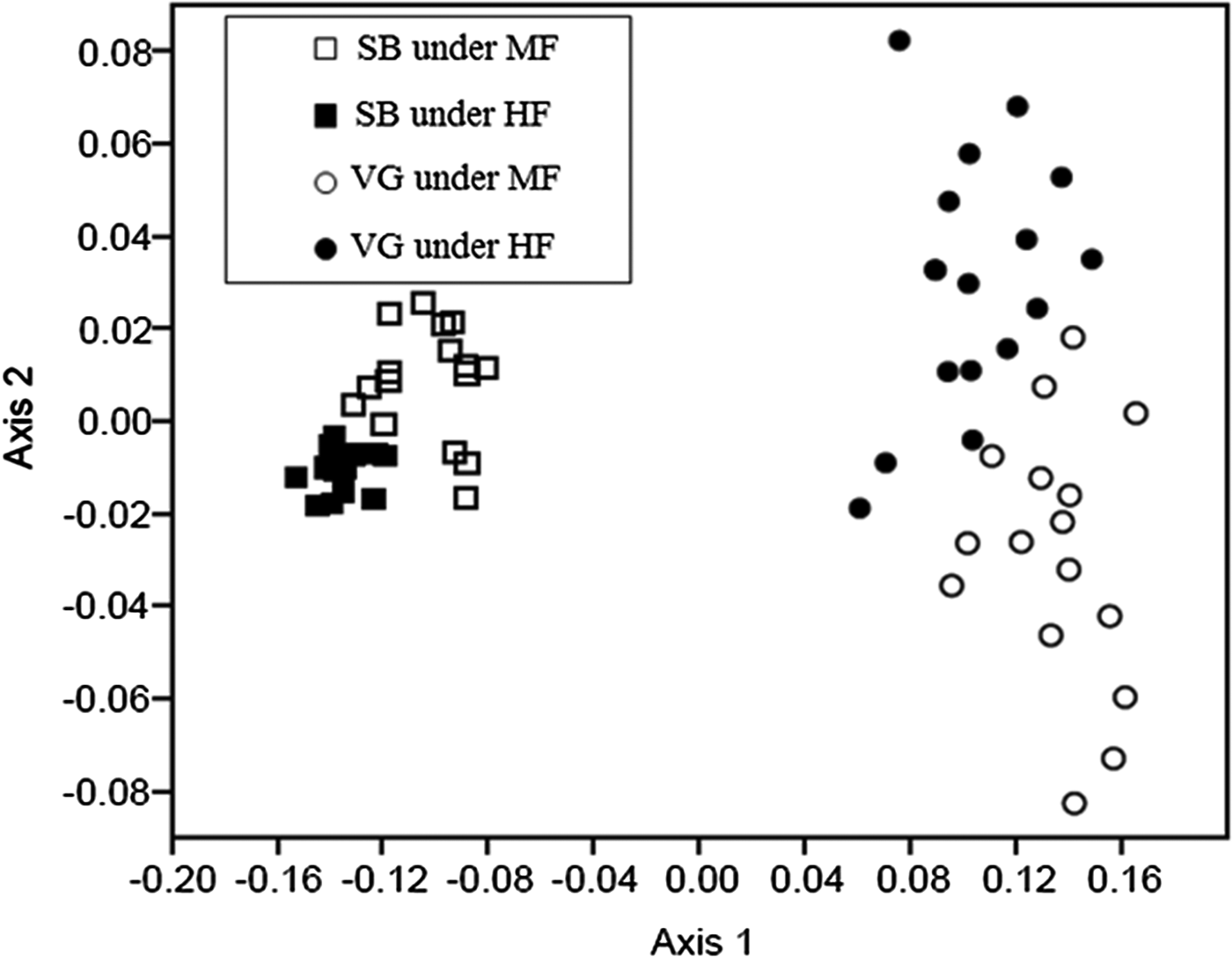
Fig. 5. Non-metric multidimensional scaling ordination plots based on the species composition of emerged weed flora (winter + summer plants/m2) and the seedbank (seeds/m2) in an integrated crop-livestock system in southern Brazil. The stress value was 0.1127. The ordinations are based on the Bray–Curtis dissimilarity matrix for relative abundance. MF, moderate forage allowance; HF, high forage allowance; VG, weed vegetation (winter + summer emerged weed flora); SB, weed seedbank.
The analysis of the community structure revealed that crop rotation and grazing management (forage allowance and stocking method) did not affect species richness, the Shannon diversity index, or evenness in the weed seedbank. For the emerged weed flora (winter + summer), forage allowance significantly (P < 0.01) affected the species richness (Table 6) and evenness of the emerged weed flora (Table 6). Moderate forage allowance resulted in higher species richness and lower evenness than did high forage allowance. Overall, the low evenness values indicate that the species community was dominated by a single species (Hosseini et al., Reference Hosseini, Karimi, Babaei, Mashhadi and Oveisi2014). Compared with that under rotational stocking, the emerged weed flora under continuous stocking had a significantly higher Shannon diversity index value (Table 6), indicating a more diverse weed community.
Table 6. Effects of forage allowance and stocking method on weed species richness (number of species), Shannon diversity and evenness index with ± s.e. of an integrated crop-livestock system in southern Brazil

Discussion
Studies conducted in other regions of the world have demonstrated that integrating livestock grazing into diversified crop-based systems can reduce weed infestations in arable lands (Tracy and Davis, Reference Tracy and Davis2009; Miller et al., Reference Miller, Menalled, Sainju, Lenssen and Hatfield2015; Lehnhoff et al., Reference Lehnhoff, Miller, Miller, Johnson, Scott, Hatfield and Menalled2017). In the present study, it has demonstrated that the magnitude of weed infestation in an ICLS depends on grazing management (i.e. forage allowance and stocking method) and its interaction with crop rotation. Furthermore, the current research was performed under no-tillage management, whereas other studies have been conducted in tilled management systems (Tracy and Davis, Reference Tracy and Davis2009; Miller et al., Reference Miller, Menalled, Sainju, Lenssen and Hatfield2015). The current results also provide new insight with robust evidence that forage allowance, along with the standard management of no-tillage ICLS (i.e. crop rotation and grazing management), is the major factor that determines weed outcomes. Competition for light, forage regrowth and both defoliation and possible species selection by grazing animals are direct impacts that can occur during the grazing phase in winter, whereas residue cover during the summer row-cropping phase likely results in a physical barrier to weed emergence. All of these impacts may potentially be affected by forage allowance.
Changes in the weed seedbank in response to grazing management and crop rotation in an integrated crop-livestock system
The soil seedbank is the primary source of weed infestation in agricultural fields and management practices that reduce weed seedbanks are valuable for integrated weed control methods (Davis et al., Reference Davis, Anderson, Hallett and Renner2006). The results of the present study indicate that high forage allowance in an ICLS under no-tillage management with a sheep-grazed winter cover crop reduced the weed seedbank size significantly and specifically reduced the number of seeds of two particularly problematic species: C. bonariensis and S. media. These weeds produced seed during the harvest season, as incomplete control by herbicides and moderate forage allowance allowed relatively more plants of these species to produce seed each year. These results agree with those of Schuster et al. (Reference Schuster, Pelissari, de Moraes, Harrison, Sulc, Lustosa, Anghinoni and Carvalho2016), who reported that reducing the grazing intensity of cattle (i.e. increasing the forage allowance) under no-tillage management reduced the seedbank size in an ICLS. Therefore, high forage allowance may be required to reduce the weed seedbank in an ICLS under no-tillage management independently of the nature of the grazing species, as the same response has now been observed between sheep and cattle grazing. It is possible that the high forage allowance led to greater competitive pressure on these species, reducing their development and fecundity and, as a consequence, reducing their seedbank size and recruitment (Schuster et al., Reference Schuster, Pelissari, de Moraes, Harrison, Sulc, Lustosa, Anghinoni and Carvalho2016).
In the current study, >0.70 of the weed seedbank was found in the 0–5-cm soil layer and no treatment effects were found on the seedbank size at the 5–10-cm soil depth. Under no-tillage management, weed seeds accumulate primarily in the top layer of the soil (Cardina et al., Reference Cardina, Herms and Doohan2002), although some seeds can infiltrate soil over time via cracks, transport by fauna and thermohydric cycles (Dorado et al., Reference Dorado, Del Monte and Lopez-Fando1999). The results of the present study indicate that the treatments had no great influence on the factors that drive the vertical distribution of seeds in the no-tillage ICLS despite the treatments having been in place for a 12-year period.
Changes in emerged weed flora in response to crop rotation and grazing management in an integrated crop-livestock system
Winter-grazed cover crop phase
In the present study, higher forage allowance resulted in 44% more crop biomass and higher vegetative cover than did moderate forage allowance during winter-grazed cover crop phase. The higher vegetative cover exerted greater competition, resulting in the suppression of many weeds during the winter pasture phase of the ICLS. Thus, high forage allowance could potentially be used as a management tool in an ICLS. However, A. lividus showed the opposite response to forage allowance (i.e. lower emerged weed flora density in the moderate forage allowance treatment than under high forage allowance), probably because plants of this genus are grazed selectively by sheep (Ramos et al., Reference Ramos, Ferrer, García, Fernández and Loste2005). Decreased forage allowance reduces animal feed selection and this reduction could result in increased intake of this species by sheep under moderate forage allowance. Furthermore, annual dicotyledonous plants, in general, are more vulnerable to frequent defoliation than are perennials and grasses (Meiss et al., Reference Meiss, Munier-Jolain, Henriot and Caneill2008). Moreover, De Bruijn and Bork (Reference De Bruijn and Bork2006) reported that rotational stocking depends on high grazing intensities for effective weed control in perennial pastures. In accordance with this, the current results demonstrated that weed suppression by increased grazing intensity (i.e. the use of moderate forage allowance instead of high forage allowance) was greater under rotational management than under continuous stocking management, and specifically, rotational stocking reduced the number of plants of D. horizontalis and S. media. Rotational stocking can reduce selective grazing by animals, thus increasing animal intake of these weed species when compared with continuous stocking management, which tends to enable animals to be more selective in what they graze.
The current findings revealed that during the winter-grazed cover crop phase, two distinct methods occur for weed suppression, each with different trade-offs. First, relatively low grazing intensity (high forage allowance) results in more cover crop biomass and increased weed suppression by competitive exclusion for some weeds; however, these actions reduce the system efficiency because less grazing can result in reduced gains or increased need for supplemental feed for animals, reducing overall profits from livestock production (Neto et al., Reference Neto, Savian, Schons, Bonnet, do Canto, de Moraes, Lemaire and de Faccio Carvalho2014). Second, relatively high grazing intensity (moderate forage allowance) and the use of rotational stocking potentially reduces animal feed selection, which could result in increased weed intake and a subsequent reduction in the density of some weeds. However, increased grazing intensity can reduce overall residual biomass, which can reduce soil cover and thus weed suppression during summer row cropping (Schuster et al., Reference Schuster, Pelissari, de Moraes, Harrison, Sulc, Lustosa, Anghinoni and Carvalho2016).
Summer crop phase
Winter forage allowance for grazing animals determines the amount of pasture residue that remains when summer row crops are seeded in an ICLS under no-tillage management (Schuster et al., Reference Schuster, Pelissari, de Moraes, Harrison, Sulc, Lustosa, Anghinoni and Carvalho2016). A high forage allowance increases residual straw (Kunrath et al., Reference Kunrath, Cadenazzi, Brambilla, Anghinoni, de Moraes, Barro and de Faccio Carvalho2014), which provides a physical barrier that can reduce emerged weed flora (Webster et al., Reference Webster, Simmons, Culpepper, Grey, Bridges and Scully2016), corroborating the current results. For example, the current results showed lower C. bonariensis emergence under high forage allowance than under moderate forage allowance. Wu et al. (Reference Wu, Walker, Rollin, Tan, Robinson and Werth2007) reported that C. bonariensis seeds are positively photoblastic, so increased pasture residues that better cover the soil during the summer crop phase could restrict light penetration into the soil during a fallow situation sufficiently to reduce C. bonariensis germination.
After crop harvest, producers often allow weeds to grow uncontrolled, but controlling these weeds is important to avert new seed deposits into the seedbank, which is the main source of future weed infestations in arable lands. In the present study, high forage allowance reduced weed population densities after harvest in ICLS, possibly because residual straw had the same suppressive effects as those reported above during the crop growing season. Furthermore, as Italian ryegrass begins to establish before crop harvest, it could compete with any weed seedling emergence; the number of emerged Italian ryegrass seedlings is higher under high forage allowances when compared with moderate forage allowances (Neto et al., Reference Neto, Savian, Schons, Bonnet, do Canto, de Moraes, Lemaire and de Faccio Carvalho2014), indicating that the high emergence of Italian ryegrass under high forage scenarios can result in lower weed emergence. Moreover, several studies have demonstrated that, compared with crop monocultures, crop rotations reduce weed populations (Liebman and Dyck, Reference Liebman and Dyck1993). Nevertheless, the current study showed contrasting results in the harvest phase; weed populations in the soybean-maize rotation were higher than those in the soybean monoculture. However, this effect was closely linked to the effects of significant increases in the seedling emergence of specific weeds (i.e. S. media) in the crop rotation treatments S. media is among the 12 most successful opportunistic species to colonize fields (Holm et al., Reference Holm, Pancho, Herberger and Plucknett1991). This species can grow and reproduce in a very short time and is adapted to a wide range of habitats; these attributes partially explain why this species was most abundant among the emerged weed in the current study. Additional research is needed to determine how management factors in ICLSs, especially summer crop rotations and stocking method under moderate forage allowance, affect S. media in an ICLS.
Weed community composition and structure in an integrated crop-livestock system subjected to long-term crop rotation and grazing management
The current ICLS results with a winter-grazed grass cover crop under no-tillage management demonstrated that forage allowance is a more important long-term ecological filter for determining weed outcomes than are stocking method and summer crop rotation. In agricultural landscapes, weeds behave as a metacommunity and different types of management practices act as filters (i.e. preventing or facilitating weed invasion or seedbank expression) that ultimately affect the weed community at the field level. Species richness in the seedbank was not affected by forage allowance and was higher in the emerged weed flora under moderate forage allowance than under high forage allowance. This finding provides evidence that high forage allowance filters the weed seedbank expression better than a moderate forage allowance both in terms of the density of emerged weed flora as well as the number of species present. The increased similarity between the weed seedbanks and emerged weed flora resulting from reduced forage allowance was previously reported by Schuster et al. (Reference Schuster, Pelissari, de Moraes, Harrison, Sulc, Lustosa, Anghinoni and Carvalho2016) in a similar ICLS experiment.
In the past century, weed diversity has drastically decreased in arable lands (Robinson and Sutherland, Reference Robinson and Sutherland2002) and several studies have drawn attention to the need to conserve weed diversity in croplands (Gaba et al., Reference Gaba, Reboud and Fried2016). The importance of weed diversity can be viewed as a trade-off between (1) the benefits of providing ecosystem services (e.g. trophic offerings for herbivores and pollinators) and (2) the harmful effects on crop production (e.g. alternate hosts for pathogens and insect pests, crop-weed competition losses and harvesting difficulties) (Mézière et al., Reference Mézière, Colbach, Dessaint and Granger2015). The current results showed that continuous stocking in the winter-grazed grass cover crop under no-tillage management promoted greater weed diversity than did rotational stocking at the plot scale, which is adequate for evaluating how biotic factors can affect species community structure (Perronne et al., Reference Perronne, Munoz, Borgy, Reboud and Gaba2017). However, for a functional analysis of both ecosystem services and the harmful effects of weeds, it is necessary to use data from a regional scale to assess the entire species pool. Other environmental advantages of continuous stocking have been reported previously. For example, Savian et al. (Reference Savian, Neto, De David, Bremm, Schons, Genro, do Amaral, Gere, McManus, Bayer and de Faccio Carvalho2014) demonstrated that, compared with rotational stocking, continuous stocking reduced methane emissions per unit animal.
Limits of the present results
Production systems that have increasingly diverse and dynamic components, such as ICLSs, create complexity that shifts the living plant and soil communities further along the continuum of spatial-temporal grassland-cropping succession. In the present study, the ICLS was characterized by bi-annual no-tillage crop-pasture rotation; these characteristics differ from those of other ICLS types used in temperate regions, which are mostly based on multiannual tillage pasture-crop rotations. Under the latter type, the impact of crop-pasture management on weed dynamics could differ from that resulting from the current data. Moreover, due to the characteristics of the present investigation, the data set tends to produce a descriptive picture of an ICLS under a specific pedoclimate and weed species pool conditions. Therefore, these findings are not applicable to all production systems, particularly because the current results showed that different weed species reacted differently to grazing management. Thus, for a deep, mechanistic understanding of the reasons for effective weed control in ICLSs, future studies are needed to disentangle animal–weed interactions and identify weed traits and livestock grazing management practices that can be mutually beneficial for weed control, livestock grazing management and the environment. Modelling approaches have been used to provide substantial insight into the interactions between weeds, management practices and the environment (Colbach et al., Reference Colbach, Biju-Duval, Gardarin, Granger, Guyot, Mézière, Munier-Jolain and Petit2014), and these models could represent means for achieving a deeper understanding of weed dynamics in an ICLS.
Summary and concluding remarks
The current study adds to the existing knowledge of cropping systems–weed interactions demonstrating that the effects of crop rotation or grazing management on weeds in an ICLS with a winter-grazed grass cover crop under no-tillage management, with or without interactive effects between them, depended on the weed species pool and the time at which those weed species emerged. Furthermore, the data also indicate that, in this type of ICLS, whole-system weed management benefits from maintaining a high forage allowance during the grazing phase. In addition, the data specifically showed that high forage allowance neutralizes negative weed outcomes (i.e. increased total emerged weed flora density) that occurred in conjunction with moderate forage allowance both from continuous stocking during the winter grazing season and from crop rotation and rotational stocking during the summer in the harvest phase. Therefore, managers should generally account for the occurrence of complex assemblies of weeds in the agricultural landscape and farms should adopt high forage allowances to reduce emerged weed flora density and seedbanks in this type of ICLS. However, if managers want to prioritize livestock production via increased grazing intensity (i.e., lower forage allowance), they should use alternatives management practices that avoid the trade-off between livestock efficiency and residual biomass that covers the soil during the row crop phase, such as delaying winter-grazed cover crop desiccation after the grazing period to allow more cover crop residue.
Acknowledgements
The authors are grateful for the help provided by Arthur Weiser and Kakai Shinmi during field sampling.
Financial support
The financial support for this work provided by CAPES Foundation (Ministry of Education of Brazil) via process no 88881.135724/2016-01 is greatly appreciated.
Conflict of interest
None.
Ethical standards
Not applicable.