Introduction
New fieldwork focusing on the paleoenvironment and past channel systems was carried out during 2016–2017 in southern Iraq. This work focused on gaining a better understanding of long-term palaeochannel and environmental development during prehistoric and historic periods. Boreholes were drilled in the Dalmaj and Nahrawan regions south of Baghdad (Fig. 1) in order to obtain sedimentary samples for analyses.Footnote 1 The new samples were combined with previously recovered sediments from Nippur's ancient channel (Shatt en-Nil) that ran through the city and with sediments recovered from a borehole near Uruk. Sediments were analysed for their structure, petrography, mineralogy, and microfossil remains, with phytoliths being a particular focus, and absolute dates were obtained using Accelerator Mass Spectrometry (AMS) methods where possible. New results also include palaeoclimate proxy data obtained from a speleothem (stalagmite) sample from Shalaii cave in the Kurdistan region of Iraq, dated using Uranium-Thorium dating. These combined data provide a long-term, environmental and hydrologic history in southern Mesopotamia that can now be juxtaposed to archaeological and historical data. Results provide insights into the plant ecology and human-environmental interactions that shaped southern Mesopotamia in different millennia.
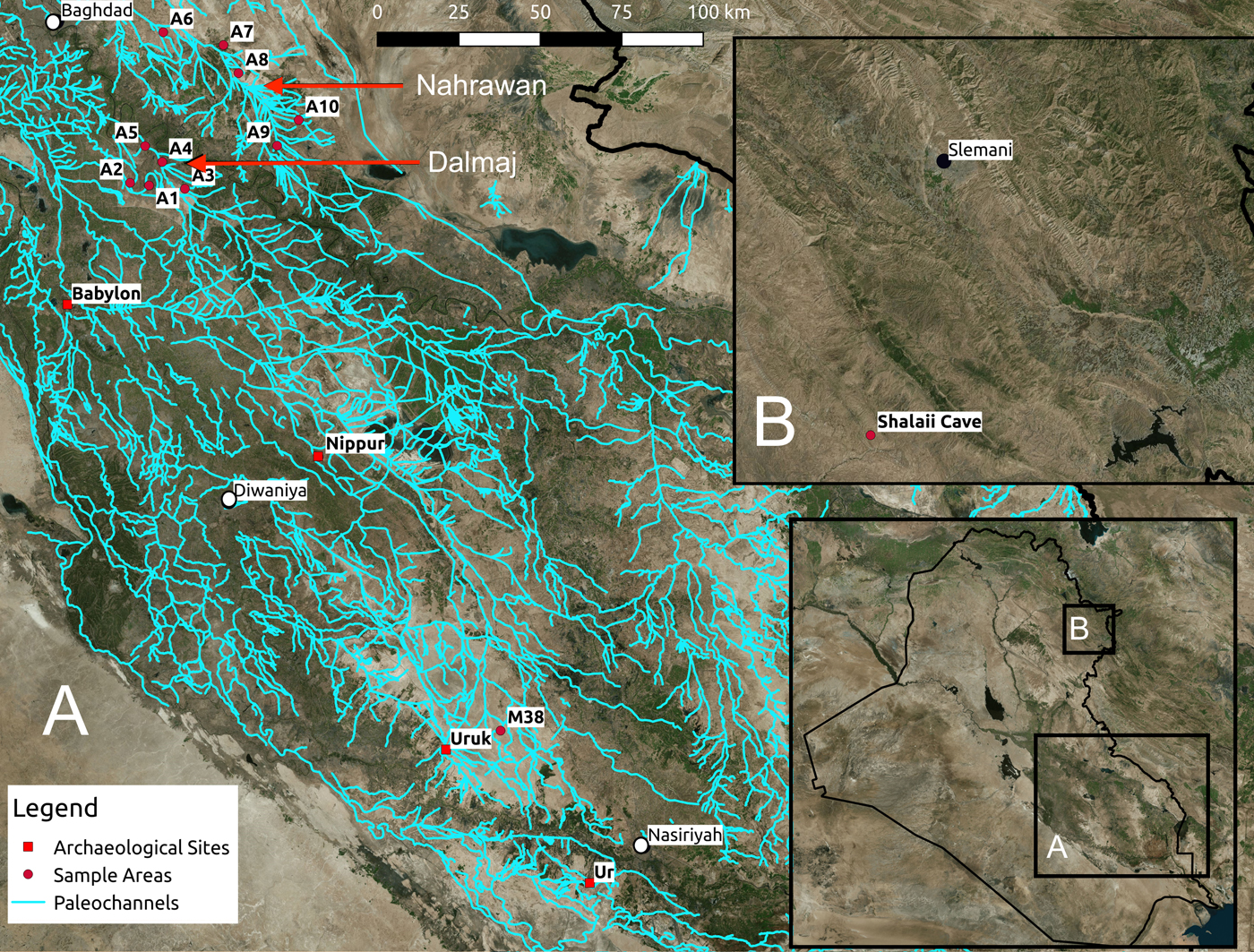
Fig. 1 Locations of fieldwork areas in southern Iraq. Inset A: numbers prefixed with A are sites of boreholes on the Naharwan and Dalmaj channels. Nippur and borehole M38 near Uruk are also indicated. Inset B: location of Shalaii cave in the Kurdish region of Iraq
In this article, we summarize the new results while also focusing on particular periods and instances where novel insights are gained. Up to this point, much of southern Mesopotamia's environmental history has been determined through evident surface remains, such as ancient river courses or canals dated by spatial association with archaeological sites, or geomorphological techniques that assess sedimentary remains. Furthermore, there is a lack of knowledge of Iraq's ancient botanical remains, including prehistoric and historic periods. For the first time in the study of ancient southern Mesopotamia, we focus on microfossil and sedimentary data and combine terrestrial proxy climate data from cave speleothems to gain novel insight into wider environmental and landscape change.
This article begins by presenting existing research on the palaeolandscapes and environmental history of southern Mesopotamia. This includes geoarchaeological, archaeological, remote sensing, and some textual analysis. Our methods are then presented, demonstrating where our work builds from these earlier efforts, and our new results are described. Finally, a discussion on the novel insights obtained and benefits of this type of research for understanding Mesopotamian environmental history is given, in light of previous archaeological and historical data.
Archaeology, Geoarchaeology and History
Beginning in the 1950s and continuing nearly unabated until 1990, archaeologists endeavoured to document and understand the settlement and environmental history of southern Mesopotamia. Work initially focused on excavation of large mounded sites (tells) that mark the landscape and represent the accumulated material remains of long-term occupation (Adams Reference Adams1981; Gibson Reference Gibson1972; Hritz Reference Hritz2010; Wright Reference Wright1980). While these features reflect the importance of place in the long history of southern Mesopotamia, archaeologists also recognized the important role of landscape and environment, specifically the ancient rivers and canals, in shaping settlement history. The guiding premise in these earlier works was that durable human settlement in ancient Mesopotamia was only possible along river channels and canals, and that changes in watercourses would be accompanied by changes in settlement locations.
The first attempts to associate tells with relict river channels, thereby providing dates of visible relict channels by association with dated tell sites and demonstrating the linkages between channels, canals, and settlement history, were made by the Diyala Basin Archaeological project begun in 1937 and led by Thorkild Jacobsen (Reference Jacobsen1982) and Robert McC. Adams (Reference Adams1965). Adams and Jacobsen postulated that by systematically mapping all mounded sites in the region, dating them by examining visible pottery on the surface, associating them with locations mentioned in textual records, and plotting them on period maps, any linear patterns that emerged would represent the major routes of ancient water courses. In other words, spatial distributions of tells were used to infer the location of channels and canals that were no longer visible or only partially visible on the ground. Using this method, archaeologists surveyed around one third of the entire alluvial plain, plotting and postulating key hydrologic events, including avulsion, siltation, and salinisation, while associating these changes to changes in the fortunes of settlements (Adams Reference Adams1981; Gibson Reference Gibson1972; Jacobsen Reference Jacobsen1960; Jacobsen and Adams Reference Jacobsen and Adams1958). Work by Adams (Reference Adams1965) in the Diyala used archaeological survey and historical data together to understand periods of environmental stress affecting settlements, where channel abandonment, including that of canals, was seen as related to settlement abandonment.
Another frequently cited environmental reason explaining the abandonment of settlements and even the collapse of major early states is salinisation induced by irrigation (Jacobsen Reference Jacobsen1982; Jacobsen and Adams Reference Jacobsen and Adams1958). The primary evidence for salinisation comes from the analysis of ancient texts. The term ki-mun (‘saline ground’) was used to classify the fertility of soils from 2500 B.C. onwards. Surveys of agricultural ground delineate parcels as saline ground that remained uncultivated due to salinisation (Jacobsen Reference Jacobsen1982: 8–9). Scholars have also compared texts recording crop yield and yield projections and used changing amounts to suggest cycles of progressive salinisation, including impact on the health of early states’ economies (Algaze Reference Algaze1993: 4–5, 104–106; Civil Reference Civil, Gibson and Biggs1987: 39–44; Gibson Reference Gibson, Downing and Gibson1974; Maekawa Reference Maekawa1974; Sallaberger Reference Sallaberger, Sallaberger and Westenholz1999: 175–177). The most comprehensive study of ancient crop yields and projections (third millennium B.C. through first millennium A.D.) was made by Jacobsen (Reference Jacobsen1982). He argued that progressive salinisation promoted the switch at the beginning of the Akkadian Period (2335-2120 B.C.) from cultivating primarily emmer wheat to farming almost exclusively more salt tolerant barley in the alluvial plain (Jacobsen Reference Jacobsen1982: 15–17; see also Helbaek Reference Helbaek, Braidwood and Howe1960: 195). This conclusion has been questioned by Powell (Reference Powell1985), who criticized not only the translation of key elements of the textual data but also noted that the preference for barley in southern Mesopotamia might have been related to its greater productivity, better adaptability and greater tolerance to seasonal variation (see also Helbaek Reference Helbaek1959: 370).
Building on the earlier extant approaches to understanding environmental and settlement pattern changes over time and space, a few local-scale survey and excavation projects demonstrated the importance of diverse micro-topography and environment within the alluvial plains. For example, field studies by Mahringer, Lintner and Brandt undertaken as part of the Nippur Expedition in the 1970s-80s (Gibson Reference Gibson and Brinkman1976, Reference Gibson and Brinkman1977 and Reference Gibson and Brinkman1978) focused on examination of historical and contemporary environmental processes in the central alluvial plain landscape, on both a local and regional level, and the relationship of these processes to the city, including how they might have influenced the course of the ancient Euphrates in relation to the urban (Brandt Reference Brandt1990). Work around Abu Salabikh also focused on channel change, as well as settlement and landscape change, demonstrating the important interplay between processes and cycles of erosion, plain scour, sedimentation, and marsh development in a seemingly homogeneous agricultural landscape (Wilkinson Reference Wilkinson1990). Collaborative geoarchaeological, archaeological, and historical analysis around Tell ed-Dēr in the 1970s focused on reconstructing and better understanding the relationship of the Euphrates and Tigris-Euphrates belt around the site (Paepe Reference Paepe1971; Paepe and Baeteman Reference Paepe and Baeteman1978), demonstrating the presence of layers of contemporaneous and diachronic relict river levees, identified through multiple datasets. Later efforts combined textual and archaeological sources with continued geoarchaeological work (Heyvaert et al. Reference Heyvaert and Baeteman2008; Cole and Gasche Reference Cole, Gasche, Gasche and Tanret1998; Morozova Reference Morozova2005; Verhoeven Reference Verhoeven, Gasche and Tanret1998). Taken together, the interdisciplinary work at Nippur, Tell ed-Dēr and Abu Salabikh, along with the shift to closer analysis of sediments to understand channel change, demonstrated the value of landscape and environmental proxy records for micro-topographic analysis of natural and ecological data. The outcome of these efforts is the broad recognition that the Mesopotamian landscape is a palimpsest, where the premise that linear site alignments always demarcate canals provides only a small portion of palaeochannel and settlement history.
During the late 1990s, although foreign work had largely stopped in southern Iraq, new access to high resolution satellite imagery, in particular CORONA imagery, allowed palaeochannels to be more easily identified and encouraged archaeologists to analyse areas beyond survey boundaries. CORONA satellites were in operation from the 1950s to 1970s, giving researchers the opportunity to map palaeochannels across Mesopotamia before the large landscape altering land reclamation projects of the 1970s. Later satellite data included higher resolution imagery, including QuickBird, and relatively easy access to digital elevation models (DEM) provided by different satellite and radar systems, such as the Shuttle Radar Topography Mission (SRTM). For the first time, these datasets provided archaeologists with a broad and encompassing view of the alluvial plains, including its micro-topography, enabling base images for time-series comparisons. Work by Pournelle (Reference Pournelle2003), Hritz (Reference Hritz2005) and al-Hamdani (Reference al-Hamdani2014) focused on areas previously unsurveyed by archaeologists, including the former Haur al-Hawiza and Hammar marshes, the Shatt al-Gharraf, and Tigris and Euphrates levees. Using remote datasets and applying geoarchaeological principles of landscape change (Wilkinson Reference Wilkinson2003), these studies identified possible new archaeological sites and landscape features such as irrigation works or habitable regions.
Beginning in 2010, Hritz et al. (Reference Hritz, Pournelle and Smith2013) began ground truthing features detected on imagery, both validating the methodological approach and illustrating the complexity of landscape evolution in southern Mesopotamia. Collecting targeted samples from archaeological sites and relict visible canals, this work demonstrated that (1) preservation in southern Iraq is such that sections exposed in historical excavations may still yield reliable AMS dates, and (2) proxy records such as shell and sediment cores can shed light on long-standing questions about landscape, such as the evolution and extent of marsh formation and canal development in antiquity (Hritz et al. Reference Hritz, Pournelle, Smith, Albadran, Issa and al-Handal2012: 77). Jotheri (Reference Jotheri2016) has greatly added to our knowledge about palaeochannels, including relict rivers and canals, through imagery analysis and subsequent ground truthing, including dating these features using augering, structural remains in sediments, mineralogy, and AMS dating.
On the historical side, considerable progress has been made in gathering textual sources to reconstruct ancient environments and historical geographies (Blaschke Reference Blaschke2018; Cancik-Kirschbaum and Ziegler Reference Cancik-Kirschbaum and Ziegler2009; |Reculeau Reference Reculeau, d'Alfonso, Cohen and Sürenhagen2008, Reference Reculeau and Düring2015, Reference Reculeau2011; Rost Reference Rost2015; Schrakamp Reference Schrakamp, Sallaberger and Schrakamp2015, Reference Schrakamp2017; Vanderroost Reference Vanderroost2012). This progress is largely due to the recent availability of online databases of ancient texts (e.g., Database of Neo-Sumerian Texts [BDTNS], Cuneiform Digital Library Initiative [CDLI], Ebla Digital Archives, Archives Babyloniennes [ARCHIBAB], etc.), which allow for the digital sourcing and more systematic analysis of entire text corpora. The success of integrating textual and archaeological data in the reconstruction of ancient environments has been demonstrated by Cole and Gasche (Reference Cole, Gasche, Gasche and Tanret1998), and currently there are several ongoing projects adopting such a combined approach (e.g., Historische Geographie Obermesopotamiens [HIGEOMES]; Cancik-Kirschbaum and Hess Reference Cancik-Kirschbaum and Hess2016; Fink Reference Fink2016; Ziegler and Langlois Reference Ziegler and Langlois2016).
These studies demonstrate the value of environmental data collection and analysis. With renewed foreign access to southern Iraq and the emergence of different techniques to collect, integrate, and analyse data, a large, regional-scale sampling program is possible, to reconstruct a general picture of long-term social, environmental, and historical change in southern Mesopotamia.
Methods
Most previous work in southern Mesopotamia focused on macro-remains, visible to the naked eye. Our work, while using satellite imagery (i.e., CORONA and QuickBird imagery) to identify palaeochannels, has also focused on combing microfossil, petrographic, and heavy mineral composition. Furthermore, new proxy data on the paleoclimate from cave speleothems now allow us to contextualize some results with climate trends, including their relation to the hydrologic cycle. Table 1 summarizes the dated sediments and samples discussed below, showing sample numbers, sediment types, dates, coordinates (in UTM 38N), and materials used for absolute dating.
Table 1 Summary of remains, key contents, locations (UTM 38N), and dated levels for sediments and speleothem samples. The Sample column indicates the sample number (e.g., A3) and depth (e.g., A3-4, with ‘4’ being depth in metres), where present. Not all samples are given in detail here; the list mostly provides dated samples and samples discussed in the text. Calibration for AMS dates employs IntCal13 (Reimer et al. Reference Reimer, Bard, Bayliss, Beck, Blackwell, Ramsey and Buck2013) and was conducted by Beta Analytic.

Geoarchaeology and Satellite Imagery
The primary goal of fieldwork was identifying and sampling from palaeochannels across southern Mesopotamia, as these channels act as environmental traps that allow dating and also contain microfossils that reflect the surrounding paleoenvironment. Palaeochannels were identified and selected using CORONA and QuickBird images, where channels can be seen as sinuous linear features that cast shadows on imagery. Identified paleochannels were then selected for fieldwork based on their visibility in the floodplain as well as possible historical relevance. Figure 1 shows the palaeochannels identified and the areas where fieldwork was conducted. This includes areas that were sampled using hand augering in the Dalmaj (A1-5) and Nahrawan (A6-10) regions. Figure 2 shows the sedimentary results from fieldwork. Boreholes A1-A10 were taken to a depth of six meters, with the exception of A2 and A3 that were shallower (three and four meters respectively), due to difficulty in penetrating deeper levels. For the samples, elevations were obtained using a level and theodolite during field surveys. The design of the augers used meant that each 0.5 m in depth resulted in a single sample being recovered. While this method is generally not enough accurate to develop a fine-scale reconstruction of deposition, it is possible to recognize the main depositional environments. Since the Dalmaj and Nahrawan areas are part of the Mesopotamian floodplain, riverine are the main type of deposits. Based on the sediments’ grain sizes, colours, microfossils, and organic matter, this environment can be divided into channel, floodplain, crevasse splay, and marsh sub-environments (Jotheri et al. Reference Jotheri, Altaweel, Tuji, Anma, Pennington, Rost and Watanabe2017). The channel deposits consist of coarse grain sediments that are mainly grey-fine sand; floodplain deposits consist of massive blocks of clay and sandy to silty clay; crevasse splay deposits are characterized by very light grey fine sand, silty sand to fine silt; marsh deposits are of clay to silty clay. Deposits were dated using AMS, where possible, using shells, charcoal, or gyttja. Problems with dating accuracy can occur in all of these materials, although shells and gyttja can be particularly problematic, where introduced carbon may not give a date of deposition (Lougheed et al. Reference Lougheed, Obrochta, Lenz, Mellström, Metcalfe, Muscheler, Reinholdsson, Snowball and Zillén2017). While acknowledging such issues, we have minimized this by presenting results where we feel there is some relative accuracy given by the fact that dates follow a chronological order (e.g., bottom layers are older) or there is good context, such as ceramics being found between dates obtained that suggest a general accuracy of dates (e.g., see Nippur below).

Fig. 2 Boreholes from the Dalmaj (A1-5) and Nahrawan (A6-10) regions. Elevation (mean sea level; MSL) is provided for the boreholes
The samples from the Shatt en-Nil, the large channel running north to south that divides Nippur into massive western and eastern tells, were recovered from excavations during Seasons 13–14 (1975–1976) of the Nippur Expedition, although the results presented here are new. The channel is believed to be the remains of an ancient Euphrates channel or canal; it is famously known from a Kassite map of Nippur and very likely existed for most of the history of the site (Kramer Reference Kramer1956: 271–275; Gibson Reference Gibson and Johnson1985). In order to examine historic environmental conditions in the vicinity of this feature, a trench was excavated with heavy mechanical equipment normally used for construction and maintenance of large irrigation canals and drainage structures. The excavation, “Trench 2,” was approximately 200 by 2 meters, with a maximum depth of 5.5 meters (Fig. 3). In some locations at the bottom of the trench, hand-excavated pits were used to allow sampling at a greater depth.

Fig. 3 Trench 2, excavated across the Shatt en-Nil at Nippur. Sediment characteristics were generally homogeneous
Petrography
Analyses of sediments included grain size and structural analysis, including composition and morphology. Samples from Dalmaj and Nahrawan were sent to the Department of Earth and Environmental Sciences, University of Milano-Bicocca in Italy for bulk petrography and heavy mineral analysis. The intent was to determine the likely origin of the sampled area's palaeochannels, specifically if the samples derived from one of the major rivers such as the Tigris or Euphrates, using mineralogy from given regions. Samples A1-5, A2-3, and A4-4 were analysed from the Dalmaj area; the Nahrawan samples A9-3 and A10-4 were also used. Mineral compositions of the samples were compared with samples from the Tigris, Euphrates and Diyala rivers. Quantitative petrographic analysis of sand within samples was carried out by point-counting in thin section (Gazzi-Dickinson method; Ingersoll et al. Reference Ingersoll, Bullard, Ford, Grimm, Pickle and Sares1984). Sands were classified according to the relative proportions of quartz, feldspars, and lithic fragments (Garzanti Reference Garzanti2016). Heavy mineral analyses were carried out using a 32-500 μm size window obtained by dry sieving. Detrital grains denser than 2.90 g/cm3 were separated by centrifuging in sodium polytungstate and recovered by partial freezing with liquid nitrogen. On grain mounts, ~250 transparent heavy mineral grains were point-counted at suitable regular spacing to obtain real volume percentages using Galehouse's (Reference Galehouse and Carver1971) method. Compositional data of sediments carried by the Euphrates, Tigris, and Diyala Rivers used for comparison are from Garzanti et al. (Reference Garzanti, Al-Juboury, Zoleikhaei, Vermeesch, Jotheri, Akkoca and Vezzoli2016). Relative sediment contributions from these rivers were assessed by forward mixing models based on integrated bulk petrography and heavy mineral data (Garzanti et al. Reference Garzanti, Resentini, Vezzoli, Andó, Malusà and Padoan2012).
Phytoliths
Another analysis conducted involved identifying phytoliths from sediments. Phytoliths, which are silicified plant cells that are deposited into sediment when plants decay, were extracted from samples using a protocol by Rosen (Reference Rosen, Rapp and Mulholland1992, Reference Rosen and Hodder2005; Fig. 4). Each sediment sample analysed, which contained phytoliths, was about 2.5 g. Hydrochloric acid (10%) was added to eliminate the carbonates. Clays were then separated out, using the manual settling method, and the samples were dried and then furnaced at 500 degrees Celsius for two hours to burn off any organic material. The phytoliths and other silica microfossils were separated from the remaining sediment using sodium polytungstate. The extracted microfossils were then placed on slides and mounted with Merck New Entellan. Slides were examined under an Alpha shot transmitted light microscope. Phytoliths were identified and counted, with an ideal minimum of 400 for single cells and 100 for multicells.

Fig. 4 Phytoliths; A) trichome from an oak (Quercus sp.), B) date palm (Phoenix dactylifera), C) multicell grass husk, and D) burnt cereal (probably barley)
Count size is important as a too-low count can introduce biases into the results, mainly via misidentification and under-representation of taxa that do not produce as many phytoliths (Strömberg Reference Strömberg2009). Monocotyledons (i.e., grasses, reeds, sedges, palms, etc.) generally produce the largest numbers of phytoliths, both single- and multicells; many trees and shrubs (dicotyledons) also produce them although generally in smaller numbers (Piperno Reference Piperno2006). Differential preservation may also introduce biases. Sediment geochemistry, sediment deposition, sediment transport, soil formation and phytolith types all can impact preservation, with some more robust morphotypes, such as bulliforms, being over-represented. This is somewhat mitigated with higher minimum counts where possible.
In addition to the boreholes from the Dalmaj and Nahrawan regions, previously excavated sediments from a machine cut near the Uruk region (Jotheri et al. Reference Jotheri, Altaweel, Tuji, Anma, Pennington, Rost and Watanabe2017; M38) are used to provide phytoliths newly studied here. Sediment samples from Nippur's Shatt en-Nil (Trench 2) are also analysed for their phytoliths.
Speleothems
An approximately three meter long stalagmite sample was recovered from Shalaii cave from the Kurdistan region of Iraq (SHC-3) and was analysed for proxy palaeoclimate data. This provides proxy paleoclimate data relevant for informing on rainfall that would have affected southern Mesopotamia's river systems. Analysis of the speleothems included measuring thickness of annual growth bands in the stalagmites, measured in high-resolution (4,800 dpi) with the software ImageJ. Stable carbon (δ13C) and oxygen (δ18O) isotope analyses were also applied; samples were micromilled at 1 cm increments. The powdered samples were then measured using a Finnigan Delta V Advantage mass spectrometer equipped with an automated carbonate preparation system (Gas Bench II) from the Chemical Analytical Facilities (CAF) at the University of Reading. The reported values are provided relative to the international Vienna Peedee Belemnite (VPDB) standard. The methodology is the same as Flohr et al. Reference Flohr, Fleitmann, Zorita, Sadekov, Cheng, Bosomworth, Edwards, Matthews and Matthews2017 and Marsh et al. Reference Marsh, Fleitmann, al-Manmi, Altaweel, Wengrow and Carter2018.
Stable isotopes of oxygen and carbon (δ18O and δ13C) are among the most widely used proxies for palaeoclimate research and provide a ‘common currency’ that can be used to link records from across a region (Roberts et al. Reference Roberts, Woodbridge, Bevan, Palmisano, Shennan and Asouti2018). Oxygen isotope variation in calcite can be caused by changes in a number of variables, including moisture source, temperature, rainfall amount and effective-moisture (P:E; Lachniet Reference Lachniet2009). δ18O calcite values from Near Eastern speleothems are thought to reflect changes in the amount of rainfall and effective moisture (P-E; Bar-Matthews et al. Reference Bar-Matthews, Ayalon and Kaufman1997; Cheng et al. Reference Cheng, Sinha, Verheyden, Nader, Li, Zhang, Yin, Yi, Peng, Rao, Ning and Edwards2015; Flohr et al. Reference Flohr, Fleitmann, Zorita, Sadekov, Cheng, Bosomworth, Edwards, Matthews and Matthews2017), although the source effect is a significant control on long-term time-scales. This is the case with Shalaii cave, where previous monitoring has established calcite growth, moisture level change, temperature and δ18O of cave drip water. These values were used to calculate the theoretical calcite value of δ18O, which indicated the speleothems were deposited very close to isotopic equilibrium (Marsh et al. Reference Marsh, Fleitmann, al-Manmi, Altaweel, Wengrow and Carter2018). Speleothems formed at or close to isotopic equilibrium are primarily influenced by variations in drip-water δ18O, which, in turn, iare primarily influenced by rainfall amount (McDermott et al. Reference McDermott, Schwarcz, Rowe and Leng2006).
Results
Below, we summarize the new results obtained by our work and present a discussion that brings in outside data.
The Dalmaj Samples
The sediments are mainly clay, with varying components of silt and sands. The sediments indicate generally low velocity flow, which is typical for a low-lying region. Figure 2 shows switching between channel and floodplain deposits, with A1 and A3-5 showing evidence of crevasse splays and A2 showing evidence of marsh deposits. Three samples were collected for mineralogical analysis from the Dalmaj area (A1-5, A2-3, A4-4). The samples were found to be quartzofeldspathic lithic sands (i.e., comprised of silica and feldspars), characterised by common plagioclase (feldspar), carbonate rock fragments, and intermediate to mafic volcanic grains. Relative to the Nahrawan samples (see below), they have higher quartz (monocrystalline/polycrystalline quartz ratio), K-feldspar, volcanic, plutonic rock fragments, and medium-rank to high-rank metasedimentary grains. Cellular serpentinite and serpentine-schist grains are particularly distinctive. Micas are common, with biotite dominating over muscovite. Heavy mineral assemblages are moderately rich, with amphibole prevailing over epidote and clinopyroxene, and minor garnet, Cr-spinel, apatite, hypersthene, and enstatite. Dalmaj palaeochannel sands are richer in volcanic detritus than Tigris sand and richer in sedimentary detritus than Euphrates sand. Mica is more common, and quartz and heavy mineral concentrations are lower than in both Euphrates and Tigris bedload, which is accounted for by the fine grain size of the samples analysed (Garzanti et al. Reference Garzanti, Andò, France-Lanord, Vezzoli, Censi, Galy and Najman2010). Forward compositional modelling indicates equal contribution of the Euphrates and Tigris rivers in the formation of the Dalmaj sediments. This suggests that the Dalmaj region was likely located close to or downstream of a confluence between the Tigris and Euphrates in earlier parts of the Holocene. As we could not obtain dates for those samples, we do not know when this would have occurred, but given the depths and dating we have from A3, we suggest a early to mid-Holocene date (i.e., pre-Ubaid up to the Uruk period). Throughout much of the Holocene, the two rivers were generally near each other in this part of the plain.
Figure 5A shows the absolute phytolith and microfossil counts per gramme. For all of the samples, it is quite low, likely due to soil processes. As can be seen from Figure 5B, monocotyledons (mainly grasses) outnumber dicotyledons (mainly trees and shrubs), which is to be expected given that monocotyledons produce more phytoliths overall. The proportions fluctuate in all of the samples, although no pattern is evident. Figure 5C shows the proportions of grasses (wild and cereals), wetland plants (reeds and sedges), date palms and dicotyledons, while excluding other monoctyledons. As can be seen, there are temporal fluctuations in the proportions, with increasing grasses in relatively more recent times (i.e., A1-1, A1-2; A2-1, A2-2). Figure 5D shows the numbers of other types of silica microfossils found in these cores. Other microfossils include non-diatom algae and sponges, all of which indicate aquatic conditions, indicating the Dalmaj has long been an alluvial area.

Fig. 5 Dalmaj: A) phytolith counts, B) proportion of monocotyledons to dicotyledons, C) types of plants, and D) other types of silica microfossils
Dalmaj samples, A3-4 and A3-3, dated to approximately 7600-7550 cal. B.C. and 5750-5650 cal. B.C. respectively. Overall, we see that the plant communities changed, with changing proportions of dicotyledons, wetland plants and grasses, and a hint of fruit cultivation through the presence of date palm in some samples. The samples generally indicate a more temperate climate (as compared to the present) with C3 grasses dominating and few C4 grasses. There are also few reeds (mainly Arundo donax). From A3-4, dicotyledons found include Quercus sp., what appears to be oak (i.e., similar to Figure 4A), while date palm (Phoenix dactylifera; similar to Figure 4B) was also found. Date palm in southern Iraq is expected, although in the eighth millennium B.C. this is relatively early. What is particularly unexpected is the presence of oak this far south. These results also suggest likely more humid conditions in southern Iraq between the eighth and sixth millennia B.C. Oak disappears above A3-3, indicating that sometime after approximately 5750-5650 cal. B.C. oak is no longer present.
The Nahrawan Samples
In general, the Nahrawan samples show a similar pattern of channel and floodplain deposits as the Dalmaj samples and have similar characteristics in grain size, colour and composition. More specifically, samples A9-2 and A9-4 were both clay, indicating a low velocity depositional environment, such as a backswamp floodplain, located away from the main channel. A9-3 consisted of silty fine sands, indicating a slight increase in velocity and more characteristic of overbank channel deposits. This indicates some movement in the river channel. Sample A10-4 consisted of sandy silts and fine sand, which are indicative of channel deposits. Samples from A9-3 and A10-4 were used for heavy mineral analysis. The Nahrawan palaeochannel sands are quartzo-feldspatho-lithic like Dalmaj sands, but much richer in limestone and chert grains. Heavy mineral assemblages are similar but much poorer and contain kaersutite and oxy-hornblende but lack orthopyroxenes. Chert abundance indicates significant supply from the Diyala River, whereas low heavy mineral concentration and relative abundance of Cr-spinel indicates recycling of foreland-basin sediments accreted to the Zagros foothills (Garzanti et al. Reference Garzanti, Al-Juboury, Zoleikhaei, Vermeesch, Jotheri, Akkoca and Vezzoli2016). Quartz, serpentinite, medium/high-rank metamorphic rock fragments, and mica are lower than in Tigris sand; forward compositional modelling indicates contribution from the Tigris and Diyala in about a 2:1 ratio. In other words, during the time these channels were active, between about 10,500-5200 cal. B.C., they were influenced by those two rivers, with a likelihood that the Nahrawan was a part of the ancient Tigris prior to it becoming a canal. This would substantiate claims by Hritz (Reference Hritz2010) about the Nahrawan's origin being linked to an ancient branch of the Tigris and the canal's reuse of an older, relict channel.
Figure 6A shows the phytolith counts per gramme for the Nahrawan. Single cells dominate, with very few multicells represented (Fig. 6B). Figure 6C shows the ratios of monocotyledons (grasses, wetland plants, palms) and dicotyledons (mostly trees and shrubs); monocotyledons dominate, but dicotyledons are significant. There is some fluctuation through time as indicated by borehole A9. Figure 6D compares proportions of dicotyledons, grasses (cereals and wild), wetland plants and date palms. We were also able to obtain two dates from A9: about 10,500-10,175 cal. B.C. (A9-4) and 5300-5200 cal. B.C. (A9-2), which also indicates that about two meters of sediment was deposited in about 5000 years and two meters was deposited in the subsequent 7000 years, not accounting for erosion and compaction. The sediments reflect a changing river course, shifting from clay (distal backswamp) to fine sands (overbank deposits) and back to clay. In the first clay sample (A9-4), there are far more wetland plants, but in A9-2, the other clay sample, dicotyledons are much more dominant. The first sample dates to around 10,000 B.C., near the beginning of the Holocene, which was particularly wet and warm, so the results are not surprising.

Fig. 6 Nahrawan: A) phytolith counts, B) proportion of single cell vs. multicell phytoliths, C) monocotyledons versus dicotyledons, and D) plant types
In borehole A9, there is a trend of increasing numbers of dicotyledons and date palms; increases in date palms could reflect increased cultivation in the late sixth millennium B.C. or early Ubaid. In all of the samples, there is also a correspondence between dicotyledons and date palm (which is a monocotyledon). The presence of date palm (Phoenix dactylifera) in sample A9-4 is significant because it shows that the tree was present in Iraq at a very early period. In fact, as far as we are aware, this is the earliest Holocene date for the presence of date palm in Iraq, indicating that it was likely a native species.
Once again, C3 grasses dominate, with very few C4 grasses, indicating, as with the other samples, that a more temperate climate regime operated, as compared to the present. There were also other types of silica microfossils, including diatoms and sponge spicules, which indicate a wet environment, typical for an alluvial environment.
Uruk Region Borehole
Borehole M38 comes from an area near Uruk and Larsa. The samples analysed date from just after about 7750-7600 cal. B.C. (M38-12.5) and to before about 4900-4860 cal. B.C. (M38-7). Additional samples from this borehole (not analysed here) were previously assessed for microfossils including diatoms (Jotheri et al. Reference Jotheri, Altaweel, Tuji, Anma, Pennington, Rost and Watanabe2017). The total number of phytoliths and other silica microfossils in this borehole is the highest of all samples analysed for off-site materials. They also fluctuate in a corresponding manner (Fig. 7A), which could be a result of preservation conditions, due to, for instance, sedimentation rates and soil processes; the numbers for both are highest from 10 to 11.5 m, which correspond to after about 7750 cal. B.C. and before 4900 cal. B.C.

Fig. 7 M38 : A) phytolith counts, B) proportion of monocotyledons to dicotyledons, C) types of plants, and D) other evidence for freshwater microfossils
Figure 7B shows the proportions between monocotyledons and dicotyledons. The proportions fluctuate through time, starting near 60/40 at 12 m, shifting to 90/10 at 11.5 m and 11 m, 80/20 (10.5 m), then back to 90/10 and finally to 50/50 at 9 m. The proportions also vary for single cells versus multicells, with the highest numbers of multicells found at 12 and 9 m depth. Figure 7C shows the varying proportions of grasses (cereals/wild grasses), wetland plants (sedges and reeds), dicotyledons and date palms. The results here are interesting in that they again reflect a changing environment. For example, at 12 m, the proportion of dicotyledons increases. This reflects a typical riparian environment, consisting of trees, shrubs, grasses and wetland plants. At 11 and 11.5 m, the number of dicotyledons decreases, while the numbers of grasses and wetland plants increase, reflecting a more marshy/wetlands type environment. At 10.5 m, dicotyledons increase again, indicating an increase in riparian type environment, one that is positioned more closely to the channel. This could be overbank or a crevasse splay deposit. In the 10 m sample, once again, oak is present, but disappears from the later samples. Interestingly, barley is also evident, showing grain cultivation was happening nearby and was contemporary with the presence of oak. At 9.5 to 9 m, we again have a marshy environment (channel likely moving away), as reflected by an increase in wetland plants and grasses, and finally at 9 m, we have an increase of dicotyledons. The sediment here is a reddish clay, interpreted as floodplain deposits. These would be distal deposits and likely were exposed to oxygen (i.e., drier conditions) for the majority of the time due to their colour. The lack of water could also explain the reduction in wetland plants. Date palm is evident in most samples. They appear early on (just after 7750 cal. B.C.) and indicate firstly a fluctuating climate, followed by increasing seasonality towards the mid-Holocene. The numbers of sponge spicules are very high, especially at 11.5, 11 and 10 m, with high numbers as well at 10.5 m. The sponges are most prevalent in the marshy sediments, indicating standing or flowing freshwater; the presence of diatoms also supports this. In fact, in Jotheri et al. (Reference Jotheri, Altaweel, Tuji, Anma, Pennington, Rost and Watanabe2017), the diatoms from the 12.5 m level indicate the area was a possible swamp. The previous results published from M38-12.5 (Jotheri et al. Reference Jotheri, Altaweel, Tuji, Anma, Pennington, Rost and Watanabe2017) and the phytoliths analysed here indicate that the area of M38 remained a freshwater environment between approximately 7750 cal. B.C. and 4900 cal. B.C. This supports conclusions that the region was conducive for settlement as early as the early eighth millennium B.C., with favourable freshwater conditions persisting afterwards.
Nippur Samples
Trench 2 showed limited stratification, with relatively homogeneous deposits of mostly buff-coloured clay and silt (Fig. 3). In several locations, there were concentrations of small shell fragments that were originally attributed to desiccation of marshes and deposits of highly wind-eroded pottery fragments, probably washed from the mound of Nippur. Identifiable cultural material in the trench included fragments of Kassite goblets in several locations, mostly concentrating at or around the levels of samples 302 and 303. The earliest sample is 300 and is at 5.5 m depth, and sample 311 was the last sample, near the surface; other samples come from 0.5 m intervals above 300. Sample 301, for instance, is at 5.0 m depth. Dates for samples are in Table 1. Interestingly, no abandonment of Nippur is evident in the sediments, but this could mean such evidence may have been eroded or even dug out. The dated levels suggest that the Shatt-en Nil existed for much of Nippur's history.
While the sediments appeared largely undifferentiated, clear differences in plant remains were evident from phytolith analysis. The numbers of phytoliths per gramme far exceeded those from the other samples and are dominated (at around 80%) by monocotyledons (mainly grasses; Fig. 8A–B). Wetland plants remain fairly consistent throughout the samples, but grasses and date palms fluctuate much more and seem to be negatively correlated (when there are more grasses, there are fewer palms and vice versa). There are fewer dicotyledons present in these samples than the other areas investigated, which could indicate a decline in trees/shrubs in the region (Fig. 8C). The overall high presence of date palms in the samples suggests these were grown very near Nippur's main channel throughout much of its history.

Fig. 8 Nippur: A) phytolith counts, B) proportion of monocotyledons to dicotyledons, C) types of plants, and D) single and multicells
The negative correlation between date palm and grasses, which includes cereals, could indicate landscape management, whereby the main focus in this area switched between date palm and cereal cultivation. The presence of date palm is certainly more obvious in samples dating from the 26th century B.C. and onwards, where this represents extensive cultivation likely occurring near or within Nippur. Date cultivation seemed to intensify after the mid-third millennium B.C., as indicated by samples 302, 303, and especially 304 (Parthian). Based on the ceramics from the levels of 302 and 303, we can roughly assume that 302 and 303 date to the late second millennium B.C.
The presence of the date palm and Arundo donax, reeds, as well as other C3 plants indicates the continuing presence of water and likely use of the channel for almost the entire recorded history of Nippur. There were also a few samples with large multicells (consisting of 10+ cells), which is an indicator of increased water availability according to Rosen and Weiner (Reference Rosen and Weiner1994). Additionally, Madella et al. (Reference Madella, Jones, Echlin, Powers-Jones and Moore2009) showed that the ratio of fixed to sensitive forms of phytoliths can also be used to detect irrigation, while more recently Jenkins et al. (Reference Jenkins, Jamjoum, Nuimat, Stafford, Nortcliff and Mithen2016) showed similar results to Madella et al. Given that rainfall levels are generally low in this region at this point, we suggest the presence of multicells likely resulted from irrigation from the channel that leads to increasingly saturated soils. It should be noted that not all samples contained these larger multicells, so there is an indication of either changing agriculture management strategies (i.e., less reliance on irrigation). There is a sudden increase in large multicells from sample 305 to 306, sometime around the Sasanian period (Fig. 8D).
Analysis of the Nippur samples indicates an environment where the climate regime was more set, although variable over time. The continued presence of C3 grasses and Arundo donax indicate a more temperate climate; however, it is likely that these are present due to irrigation/agriculture and the general dampness of the immediate vicinity, rather than reflecting regional climatic trends. The area was also much more intensively managed. Fluctuations in grains and date palm phytoliths seem to reflect agricultural strategies, rather than the result of natural environmental change from major shifts in climate. Date palms and grains appear to show a clear negative relationship, suggesting that land management and choice of cultivation of one affected the other. The increase in multicells during roughly the Sasanian period, or samples 305–306, is likely evidence for the intensification of the use of irrigation, at a rate likely more intense than earlier periods. In other words, this likely reflects irrigation expansion in the vicinity with more intense water flow. Overall, the results from Nippur are important because they span a long period (around three millennia) and illustrate the shifting agricultural strategies used at least in one area in and around the site.
Speleothem Climate Results
Our results for SHC-3, dated from about 8500 B.C. to today, are preliminary and require more work for accuracy, but a general assessment is made here. The SHC-3 record would appear to be the longest and most complete paleoclimate record for all of Mesopotamia during the Holocene. Figure 9 shows results from Shalaii's speleothems as well as additional regional data; it is further discussed below. Like existing records, the δ18O variation values are thought to reflect changes in rainfall amount (Marsh et al. Reference Marsh, Fleitmann, al-Manmi, Altaweel, Wengrow and Carter2018) and, therefore, evidence of a wetter early-mid Holocene climate relative to today is evident, while the more recent sample shows drying conditions similar to today but with volatile climate conditions. δ18O trends show that after the end of the fourth millennium B.C., there could be a shift towards generally drier conditions. These conditions could have prevailed for much of the rest of the Holocene, although periods of enhanced rainfall exist during this time. By the mid-first millennium B.C., and particularly over the last two thousand years, dry conditions appear evident, but conditions were volatile as well, sometimes reflecting short, wetter periods. These results need to be analysed further to develop a more detailed understanding for different climate trends within key archaeological periods and palaeoclimate events (e.g., 4.2 kya event).

Fig. 9 Summary of palaeoclimate data from Shalaii cave reflecting trends from recovered speleothems and showing similarity to regional data (see Sharifi et al. Reference Sharifi, Pourmand, Canuel, Ferer-Tyler, Peterson, Aichner and Lahijani2015).
Discussion and Conclusion
North Iraq Climate Discussion
This section summarises evidence for general changes in regional hydrological conditions using existing palaeoclimate records and other investigated records from northern Iraq. The Near East is still relatively neglected in terms of palaeoclimate investigations. High resolution palaeoclimate records that cover the Holocene are rare, and there exist large spatiotemporal gaps between those that do exist (Finné et al. Reference Finné, Holmgren, Sundqvist, Weiberg and Lindblom2011); this leaves a lot of uncertainty in our knowledge of hydrological conditions during the Holocene.
The δ18O records from the region show significant shifts in climate during the Holocene and are generally coeval, which are also supported by other proxy evidence, although there were likely some fine-scale, regional variations. In fact, these variations necessitate local collection of palaeoclimate data. However, we can summarise a general picture of the region, which can then be compared to the records from southern Iraq. Following a short recovery after the Younger Dryas glacial reversal, the early Holocene ~9,700 – ~4,000 B.C. was characterised by relatively depleted δ18O in speleothem and lake sediment records, an indication of wetter conditions; some of this depletion was caused by a change in source (Eastern Mediterranean) δ18O, that is rain deriving from the Mediterranean region (Cheng et al. Reference Cheng, Sinha, Verheyden, Nader, Li, Zhang, Yin, Yi, Peng, Rao, Ning and Edwards2015). A decrease in dust flux evident from a lake record in northern Iran (Sharifi et al. Reference Sharifi, Pourmand, Canuel, Ferer-Tyler, Peterson, Aichner and Lahijani2015), where abundance of titanium (Ti) generally reflects wind blown dust, supports evidence of increased rainfall for the early Holocene, similar to our results. During the end of the mid-Holocene to late Holocene, by around ~3,000 B.C., there was a shift to more enriched δ18O values, similar to the present day. This is suggestive of drier conditions prevailing. Values remain similar for much of the rest of the Holocene, although there were periods of enhanced rainfall and prolonged drought, with a few records indicating that a wet phase prevailed between ~2,000 and ~1,000 B.C. (Cheng et al. Reference Cheng, Sinha, Verheyden, Nader, Li, Zhang, Yin, Yi, Peng, Rao, Ning and Edwards2015; Roberts et al. Reference Roberts, Eastwood, Kuzucuoğlu, Fiorentino and Caracuta2011). δ18O values from lake sediments also support this trend (e.g., Roberts et al. Reference Roberts, Eastwood, Kuzucuoğlu, Fiorentino and Caracuta2011).
Previous analysis was conducted on speleothem samples from Shalaii cave, Kurdistan region of Iraq (Fig. 9). Two samples (SHC-1 and SHC-2; Marsh et al. Reference Marsh, Fleitmann, al-Manmi, Altaweel, Wengrow and Carter2018) cover different periods of the Holocene and allow for an insight into the change of δ18O and δ13C values between these periods in Northern Iraq. One sample, SHC-2, which was deposited between 8,025 B.P. (6,075 B.C.) ± 38 and 6,977 B.P. (5,027 B.C.) ± 219, has a mean oxygen isotope value of −6.54‰ (VPDB). The younger sample, SHC-1, which grew between 1,012 B.P. (938) ± 42 and 494 B.P. (1456) ± 29 B.P., shows an average enrichment of ~1.10% from SHC-02, correlating well with other speleothem records from the region, which show similar δ18O decreases of between 0.6% (Jeita) – 1.10% (Soreq) during the same period. While these two samples are relatively short in time, the third (SHC-3) more detailed sample shows some similar trends to the regional picture, although evidence for regional variations affecting northern Iraq are also evident (Table 1).
Southern Mesopotamia Environment
Several noteworthy results relevant to the environmental history of southern Iraq are presented in this work. We see similar environmental patterns in the alluvial regions of the Nahrawan and Dalmaj. The general trend from phytolith and sedimentary results shows a more temperate (i.e., as compared to the present) climate regime was established early in the Holocene (c. 10,000 B.C.) in Iraq. The microfossils all indicate changes within alluvial and surrounding environments, changing from marsh/riverine, with mainly wetland plants and grasses, to floodplain and switching to river banks, with trees often composed of date palm. South of Baghdad and the region of Nahrawan are likely to already have been habitable by the eleventh millennium B.C. To the south around Uruk, the region was habitable for settlement at least by the early eighth millennium B.C. These results suggest early habitation prior to the earliest phases of the Ubaid was likely to be present south of Baghdad, even if material culture is lacking. A lack of evidence for the Persian Gulf in areas investigated and favourable settlement conditions from phytolith and diatom results suggest that by the early Holocene, and before the Ubaid, settlement was likely in many regions south of Baghdad and as far as the regions near Uruk. It is possible that earlier, pre-Ubaid cultures used less mud brick and, given the presence of more abundant wood and other building materials such as reeds, simply relied on these perishable resources more, thus making the detection of these cultures difficult using standard archaeological methods. The sediments suggest a very different Tigris-Euphrates system once existed. The Dalmaj region suggests the Tigris and Euphrates could have been joined near this area, sometime in the early to mid-Holocene, while the Nahrawan appears to have been a branch or part of the ancient Tigris prior to the development of the canal.
One key result is the evidence of date palms. From our results, date palms were already present by around cal. 10,500-10,175 B.C. In fact, results produced here show the earliest date for date palm from Holocene Iraq. Date palms require hot dry summers for the fruit to grow and ripen, but a perennial source of groundwater for their roots. It seems that quite early on (c. 10,000 years ago), the alluvial region of Iraq was conducive for date palms, and possibly the one rain system (winter and spring rains) may have been set in this region at the beginning of the Holocene, although other works suggest increased rainfall due to summer monsoon rains (e.g., Kennet and Kennet Reference Kennett and Kennett2006). Regardless, the palaeoclimate does suggest wetter conditions in the periods prior to the fifth millennium B.C., which could suggest stronger rainfall feeding the rivers of southern Mesopotamia. Previously, the earliest archaeological evidence for the domesticated form of dates is from Eridu, from Ubaid levels (c. 7,000-6000 years ago; Zohary et al. Reference Zohary, Hopf and Weiss2013). According to archaeological evidence, fruit cultivation started during the Ubaid period in this region; it is likely, then, that the earliest phytoliths (i.e., pre sixth millennium B.C.) of date palms are from wild forms, whereas later date palms are more likely to be of the cultivated variety. The expansion of date palm in regions such as the Nahrawan and near Uruk, in the later layers, likely reflects at least some anthropogenic signature. However, we cannot exclude the possibility that date palms were cultivated even earlier than the Ubaid, as we suggest settlement in southern Mesopotamia likely occurred prior to the Ubaid. Regardless, date palm is clearly present throughout much of Iraq from the earliest phases of the Holocene, suggesting that dates grown in later periods likely derived from Iraq itself.
Another novel result is that oak was also present in southern Iraq, both in the Dalmaj and Uruk regions, between the eighth and sixth millennia B.C. No previous archaeological or historical data have discussed oak this far south in Iraq. Moreover, oak disappeared from later samples, which could occur due to increased aridity and/or increased human activity in southern Mesopotamia. From our results, we note that oak disappeared in records by the time of the earlier Ubaid and later Uruk expansions (Stein Reference Stein2012; Algaze Reference Algaze2008). In other words, part of the motivation for expansion or even colonization/trade could have been the lack of quality wood materials in southern Iraq after the sixth millennium B.C.
At Nippur, the Shatt en-Nil was a long-lived channel that existed as an active canal/river channel throughout much of the city's history. The remains from Nippur suggest that during the late second millennium B.C. and Parthian period, date palms would have been substantially cultivated in parts of Nippur, with some date palm cultivation happening in the mid-third millennium B.C. Dates, in general, do not appear very frequently in textual sources from the third and second millennia B.C. Administrative texts from the third millennium B.C. indicate the use of date palm, via indirect citation in legal contexts such as transactions or agreements (Cocquerillat Reference Cocquerillat1968; Landsberger Reference Landsberger1967). Date palms were grown in irrigated orchards along with other fruit trees, such as figs, grapes, pomegranates, and apples in the late third millennium B.C. (Focke Reference Focke2015; Greco Reference Greco2015; Postgate Reference Postgate1987; Powell Reference Powell1992). Given that most of the written records from the third and second millennia B.C. derive from public/state institutions, the lack of information on date cultivation is not entirely surprising. State and public institutions in the third and second millennia B.C. were heavily invested in cereal cultivation and to a much lesser degree in horticulture, as for example the cultivation of dates. There are some texts suggesting institutional involvement in date cultivation in the late third millennium B.C. (Focke Reference Focke2015; Greco Reference Greco2015), but it has been assumed that date cultivation was a more private/individual enterprise, as was true for example in pharaonic Egypt (Moreno Garcia, Reference Moreno García and Rostin press). However, until now, there were no empirical data that could provide a clue about the size of the non-institutional sector of early states’ economies that was based on date cultivation. Hence, one result of the presented study is a more nuanced and holistic understanding of these early state economies. Additionally, trees were grown for timber in irrigated lands in the third millennium B.C. (Selz Reference Selz and Vacín2011). Textual sources suggest that dates were part of the regular diet, but the volume of grain-related texts has generally minimized the importance of dates. Our results suggest dates were relatively important for the Bronze Age economy, although in the mid-third millennium B.C. they were not as prevalent as in the second millennium B.C. (Kassite) levels, and their importance continued well into the first millennium A.D. Overall, there appears to be a negative relationship between date palm and grain cultivation in the area sampled.
The most detailed insight into the agricultural regime of the Nippur region is provided by the archive of the Murašu agricultural firm, which managed large amounts of arable land around Nippur in the fifth century B.C. on behalf of their owners. A major shift towards intensive horticulture of date palms at the expense of cereal cultivation took place during that period in other areas of the alluvial plain. From texts in the first millennium B.C., during the Achaemenid and Hellenistic periods, there is evidence of widespread date cultivation, in particular in more northern regions such as around Sippar, with even a cash crop economy revolving around the date palm (Jursa Reference Jursa, Briant and Joannès2006). However, the Murašu archive deals primarily with cereal cultivation, with very little direct information on date cultivation (Jursa Reference Jursa2010: 407). References to the cultivation of dates are only found in debt notes, in which those to be paid by members of the Murašu agricultural firm were reckoned in large quantities of dates. Other references to date cultivation are rents collected in the form of quantities of dates for the leasing of garden plots (Jursa Reference Jursa2010: 410–411). Furthermore, Jursa (Reference Jursa2010: 409, 413) suggests that date cultivation was not as prevalent in the Nippur region as elsewhere, consisting probably of medium to modest sized gardens tended to by individual families. The large quantities of dates listed in debt notes are thus to be understood as the obligation of a collective of tenants or farmers.
Although irrigation was present in Nippur in the earliest levels from the phytoliths analysed, it greatly expanded around the time of the Sasanian period, from the third century A.D. or later. This is supported by historical sources from the region that show expansion of irrigation during the Sasanian period (Campopiano Reference Campopiano2017). Our results likely reflect the major irrigation works that began to be created by this period. Volatile climate could have been one problem faced in irrigation, as evident from climate data, where alternating periods of high rainfall and very dry years could have been frequent at least by the first millennium B.C., and into later periods. From a methodological view, phytolith analysis proved useful in differentiating the homogeneous remains from the Shatt en-Nil.
What the results most clearly demonstrate is the potential use of these multidisciplinary approaches, applied here in southern Mesopotamia. Furthermore, linkages between rainfall patterns in northern Iraq, via speleothem data, can be made to events in southern Iraq, including changes in the hydrologic regime and changes to the environment and plant communities (e.g., the disappearance of oak in southern Iraq). Future work should certainly focus on validating the observations presented here, as we recognize that remains from a limited number of samples do not mean wide-scale trends across southern Mesopotamia. Nevertheless, we are now better able to reconstruct plant communities and the local environments and how they were affected/modified by human activity and natural climate variations.
Acknowledgements
We are deeply grateful to the Neubauer Collegium for Culture and Society at the University of Chicago for funding the fieldwork, analysis and a 2018 conference that allowed collaborators to share results. The Neubauer also generously organized and hosted the conference. In addition, earlier funding from the Japanese Society for the Promotion of Science (JP26283012: C.E. Watanabe) helped fund our work near Uruk. The National Geographic Society also provided funding to conduct fieldwork and analysis. Funding from the British Institute for the Study of Iraq helped cover our work in Shalaii cave.