Introduction
Invasive plant control is often undertaken with the explicit goal of conserving native biodiversity. Unfortunately, the two goals—eradication of exotic species and promotion of native species—are not necessarily coupled (Ogden and Rejmánek Reference Ogden and Rejmánek2005). For example, control methods including herbicide application and biological control can cause damage to native species that the project is supposed to conserve (e.g., Denslow and D’Antonio Reference Denslow and D’Antonio2005; Rinella et al. Reference Rinella, Maxwell, Fay, Weaver and Sheley2009; Simberloff Reference Simberloff2012; Thomas and Reid Reference Thomas and Reid2007). Furthermore, open niches created by the removal of the target species may be colonized by other exotic species rather than the intended native species (Abella Reference Abella2014; Case et al. Reference Case, Harrison and Cornell2016; DeMeester and Richter Reference DeMeester and Richter2010; Luken et al. Reference Luken, Kuddes and Tholemeier1997; Turner et al. Reference Turner, Scott and Spafford2008; Zavaleta et al. Reference Zavaleta, Hobbs and Mooney2001).
Management Implications
The ability of habitat managers to design invasive plant control strategies that will promote the recovery of native biodiversity is hampered by a paucity of data monitoring plant community responses. Our study demonstrated that relatively short-term herbicide treatments reduced abundance of, but did not eradicate, Phragmites and also increased native plant cover. Future studies should expand on our findings to test the whole-community response to a wider range of treatments, in various environmental conditions, and for longer timescales. Such information will aid in the design of exotic plant strategies that facilitate ecosystem recovery including native species composition and abundance. In the meantime, our study demonstrates multiple positive outcomes of Phragmites control, including recovery of native plant community composition.
Unfortunately, data regarding community responses to invasive species control are scarce (Abella Reference Abella2014; Denslow and D’Antonio Reference Denslow and D’Antonio2005; Hazelton et al. Reference Hazelton, Mozdzer, Burdick, Kettenring and Whigham2014; Kettenring and Adams Reference Kettenring and Adams2011; Reid et al. Reference Reid, Morin, Downey, French and Virtue2009). For example, of the 95 papers describing control of “weeds of national concern” in Australia examined by Reid et al. (Reference Reid, Morin, Downey, French and Virtue2009), only 18 assessed the response of the plants besides the target species. Kettenring and Adams (Reference Kettenring and Adams2011) found that only 84 of the 355 studies of exotic species control included any monitoring of postcontrol vegetation; of those, 49 reported exotic species response only, 10 reported native species response only, and 25 reported both. Treatment area was also typically small, as 46% of the reported studies applied exotic species control to areas smaller than 30 m2. Hazelton et al. (Reference Hazelton, Mozdzer, Burdick, Kettenring and Whigham2014) highlighted the paucity of studies assessing community response to common reed [Phragmites australis (Cav.) Trin. ex Steud.] control as a critical knowledge gap. Where native response to invasive species control has been examined, it often falls short of stated goals (Abella Reference Abella2014; González et al. Reference González, Sher, Anderson, Bay, Bean, Bissonnete, Bourgeois, Cooper, Dohrenwend, Eichhorst, El Waer, Kennard, Harms-Weissinger, Henry, Makarick, Ostoja, Reynolds, Robinson and Shafroth2017; Martin and Blossey Reference Martin and Blossey2013; Reid et al. Reference Reid, Morin, Downey, French and Virtue2009).
Habitat managers’ ability to design successful strategies to promote native biodiversity is hampered by this lack of quantitative information. A variety of authors have called for greater emphasis on monitoring in conservation and management projects, both for the benefit of the project itself (e.g., “adaptive management”) and as a guide for future projects (e.g., Lyons et al. Reference Lyons, Runge, Laskowski and Kendall2008; Nichols and Williams Reference Nichols and Williams2006). Such data, including the responses of the plant community and the target species to treatments, can provide objective evidence of what techniques, across a range of conditions, are best employed to achieve specific management goals.
We monitored the response of the plant community to repeated herbicide treatments designed to eradicate P. australis (hereafter Phragmites) from a tidal marsh complex. We assessed Phragmites abundance, vegetation cover, and community composition starting before treatments began and for 3 yr following treatments in five Phragmites stands that ranged from 0.04 to 0.8 ha in size. We tested whether 3 yr of treatment were able to eradicate Phragmites and increase the cover of native species. We also compared species composition in treated and uninvaded reference areas to assess plant community recovery following treatment. By comparing the responses across a range of infestation sizes, we can suggest conditions in which management goals are most likely to succeed and whether those conditions align with already established expectations regarding exotic species control (e.g., Quirion et al. Reference Quirion, Simek, Dávalos and Blossey2018; Rejmánek and Pitcairn Reference Rejmánek and Pitcairn2002).
Materials and Methods
Phragmites is capable of forming dense stands that decrease plant species richness and cover (Ailstock et al. Reference Ailstock, Norman and Bushmann2001; Warren et al. Reference Warren, Fell, Grimsby, Buck, Rilling and Fertik2001). Retrospective studies using historical aerial photographs have documented the replacement of plant communities dominated by cattail (Typha spp.) and saltmeadow cordgrass [Spartina patens (Aiton) Muhl.] by Phragmites (Wilcox et al. Reference Wilcox, Petrie, Maynard and Meyer2003; Winogrond and Kiviat Reference Winogrond and Kiviat1997). Attempts to remove established stands of Phragmites or control its spread employ a wide array of cultural, mechanical, and chemical methods (Hazelton et al. Reference Hazelton, Mozdzer, Burdick, Kettenring and Whigham2014; Marks et al. Reference Marks, Lapin and Randall1994). Herbicide application is the predominant technique used by managers (Hazelton et al. Reference Hazelton, Mozdzer, Burdick, Kettenring and Whigham2014; Martin and Blossey Reference Martin and Blossey2013) and is considered to be the most effective (Marks et al. Reference Marks, Lapin and Randall1994; Warren et al. Reference Warren, Fell, Grimsby, Buck, Rilling and Fertik2001).
Study Site
We conducted our experiment in Ramshorn Marsh, an approximately 308-ha freshwater tidal wetland located on the west shore of the Hudson River, New York, USA (42° 12′ N; 73° 51′ W; Figure 1) (Alldred et al. Reference Alldred, Baines and Findlay2016). This marsh contained five wetland plant communities: mudflat, Typha marsh, mixed marsh, Phragmites, and wooded swamp. The Typha and mixed marsh communities constituted 45 ha of the site and were dominated by narrowleaf cattail (Typha angustifolia L.), green arrow-arum [Peltandra virginica (L.) Raf. ex Schott], spotted touch-me-not (Impatiens capensis Meerb.), and single-veined sweetflag (Acorus calamus L.). They also contained several statewide and regionally rare plant species, including northern beggarticks (Bidens hyperborea Greene) and swamp lousewort (Pedicularis lanceolata Michx.). Five Phragmites stands were present in the wetland before treatment and were surrounded by Typha and mixed marsh. They ranged in size from 0.04 to 0.8 ha, totaled 1.5 ha, and comprised ~3% of the emergent marsh area (Figure 1). The age of each infestation was not known, though the larger ones were visible in 1998 aerial photos, meaning they were established at least 12 yr before the start of treatment.
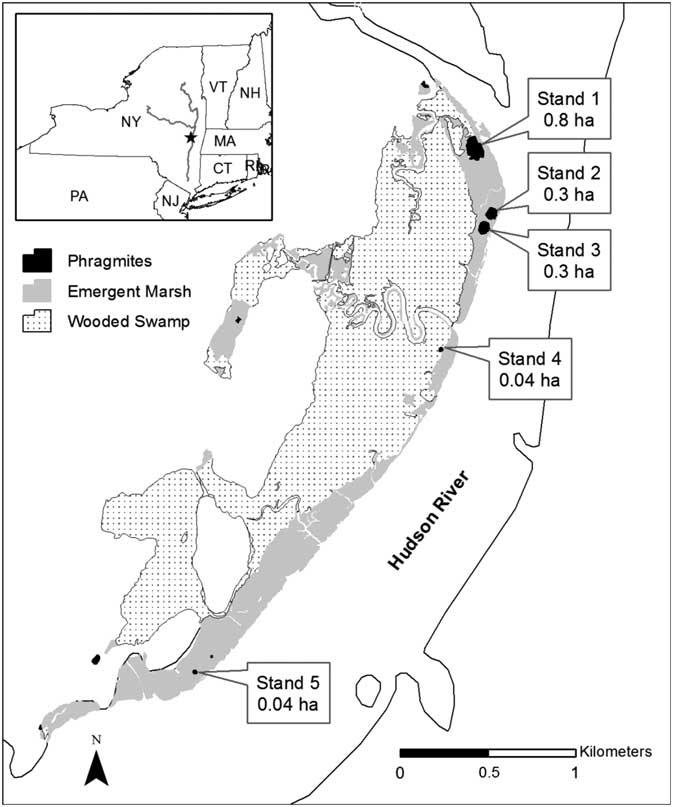
Figure 1 Locations of five Phragmites stands in Ramshorn Marsh along the Hudson River, NY. Community types—Phragmites, emergent marsh (e.g., Typha and mixed marsh), and wooded swamp—found at the site are indicated by shading. All treatments were done in the five Phragmites stands, and sampling was done in the Phragmites and emergent marsh habitats. Inset map depicts the location of the site within New York State.
Phragmites Treatments and Sampling Design
The five Phragmites treatment stands were sprayed with a solution of glyphosate-based herbicide (AquaPro®, SePRO Corporation, Carmel, IN) plus an aquatic-approved surfactant (LI 700®, Loveland Products, Loveland, CO) in September 2010. Four of the five Phragmites stands (1 to 4) were treated from a vehicle-mounted spray system (Marsh Master, Coast Machinery, Baton Rouge, LA); the remaining stand was treated with a low-volume backpack sprayer due to access issues. The dead Phragmites stems were knocked down in the three largest stands (1 to 3) in spring 2011. Follow-up herbicide treatments were applied in all stands in September 2011 and 2012 via backpack sprayer using a 1% solution of AquaPro with surfactant. In 2012, all Phragmites stems were flagged and mapped before treatment in an attempt to treat 100% of the stems.
We assessed treatment efficacy and vegetation recovery by surveying in and adjacent to each Phragmites infestation. Pretreatment surveying was performed in August 2009 and for 3 yr posttreatment in August in 2011 to 2013. Each year, we estimated percent cover of all plant species to the nearest 5% and counted the number of Phragmites stems in 10 to 12 randomly located 1-m2 plots within the interior of each stand. The plot locations within each stand and year were chosen spatially using a generalized random tessellation stratified survey design (Kincaid and Olsen Reference Kincaid and Olsen2013). We did not distinguish between nodding beggarticks (Bidens cernua L.) and burmarigold [Bidens laevis (L.) Britton, Sterns & Poggenb.], as specimens from this marsh often had characteristics of both species. In 2013, we completed a Phragmites stem census covering the entirety of each stand.
Each year, we sampled outside the area of the initial Phragmites infestation to characterize a reference plant community for each stand. We located 10 equidistant points along the perimeter of each stand using Xtools Pro (v. 8.2; Data East, Novosibirsk, Russia) in ArcGIS (v. 10.0; Esri, Redlands, CA) and then established a 1-m2 reference plot a random distance between 6 to 10 m away from that point in the nearest cardinal direction. This distance was chosen so as to reduce the possibility of non-target impacts to the vegetation from the herbicide application; evidence from community composition in plots 1 to 5 m away from treated patches (unpublished data) confirmed this assumption. Plots that fell within mudflat habitat were not sampled, as they were not representative of marsh habitat.
Plot locations and sampling data are available from the Dryad Digital Repository (Reference Zimmerman, Shirer and CorbinZimmerman et al. 2018).
Statistical Analysis
We calculated the mean number of live Phragmites stems, mean percent cover of native species, and mean percent cover of non-Phragmites exotic species for each stand in each year. We tested for changes in each log-transformed dependent variable over time using separate repeated-measures analyses.
We assessed the recovery of native vegetation by comparing community composition in treated Phragmites stands with uninvaded neighboring reference plots before and after herbicide treatment. We calculated Euclidian distances for treated and reference plots in each stand in years 2009 and 2013, using percent of all species in each plot (‘vegan’ package of R). Euclidian distance was found to be the most effective of several distance methods in separating plots along a gradient of stand (RANKINDEX command in ‘vegan’), and results using other methods did not yield qualitatively different results. These distances were plotted using nonmetric multidimensional scaling (NMDS). The final stress values of the 2009 and 2012 analyses were 0.146 and 0.139, respectively.
All analyses were performed using R (v. 3.4.2).
Results and Discussion
Conditions before Treatment Application
Before herbicide treatments began, Phragmites stem density ranged from 9.3 to 40.1 stems m−2, while percent cover of native species ranged from 40.9 to 94.4 (Figure 2A and B). These two variables were strongly negatively correlated (Pearson’s correlation coefficient = −0.91, t 3 = 4.0, P = 0.028). Specifically, the three largest stands, 1, 2, and 3, had the highest Phragmites density and the lowest percent native cover. Pretreatment cover of exotic species besides Phragmites was below 8% in all stands (Figure 2C).

Figure 2 Responses of (A) Phragmites density (stems m−2), (B) total native plant cover, and (C) total non-Phragmites exotic plant cover in each stand in 2009 (pretreatment) and 2011 to 2013. Each symbol is the mean (±1 SE) of 10 to 12 plots (1-m2) per stand.
NMDS analysis revealed sharply distinct communities in Phragmites stands versus reference stands in 2009, as invaded stands clustered to the left of the ordination space, while reference stands clustered to the right (Figure 3A). There was a further breaking out of stands within the ordination space, as invaded areas of Stands 4 and 5 plotted more closely to reference stands than did invaded areas of Stands 1, 2, and 3. There were three distinct community types: one, dominated by T. angustifolia and P. virginica, was found mostly in reference areas of Stand 5; a second, dominated by Phragmites, was found exclusively in invaded areas of Stands 1, 2, and 3; and a third community type was dominated by I. capensis, P. virginica, and the nonnative A. calamus. Within this third community type, there was a further separation between invaded (to the left side of the ordination space in Figure 3A) and uninvaded reference areas (to the right in ordination space in Figure 3A) driven by the presence/absence of Phragmites.

Figure 3 Nonmetric multidimensional scaling (NMDS) of Euclidian dissimilarity indices of (A) 2009 (pretreatment) and (B) 2013 vegetation composition in treatment (red) and reference (blue) areas. Stand number is indicated with the appropriate symbol. Dominant species in the analysis are shown by their six-letter code: ACOCAL, Acorus calamus; BIDCER, Bidens cernua/laevis; IMPCAP, Impatiens capensis; LEEORY, Leersia oryzoides; PELVIR, Peltandra virginica; PERARI, Persicaria arifolia; PHRAUS, Phragmites australis; SAGLAT, Sagittaria latifolia; TYPANG, Typha angustifolia.
Response of Phragmites to Treatments
Herbicide treatment had a dramatic negative effect on the density of live Phragmites stems (Figure 2A; repeated-measures ANOVA F(3, 12) = 94.2; P < 0.0001). Stand-wide stem census counts revealed that overall stem density in 2013 was <1.5 stems m−2 in all stands and was particularly low in Stands 4 and 5, with <50 total stems detected per stand.
Effective Phragmites control to the point of eradication frequently requires at least several more years of treatment beyond the 3 yr we applied treatment (e.g., Lombard et al. Reference Lombard, Tomassi and Ebersole2012). Phragmites abundance was very low following treatment in all stands, but the prospects for successful eradication were lower for larger stands, where some small but dense Phragmites patches persisted even after 3 yr of control. Our results suggest that smaller stands (<0.1 ha) that begin with lower stem density are easier to eliminate. Previous assessments of Phragmites control have reported successful reduction in Phragmites abundance, particularly in smaller stands (Martin and Blossey Reference Martin and Blossey2013; Quirion et al. Reference Quirion, Simek, Dávalos and Blossey2018). However, the likelihood of eradication is strongly dependent on infestation size: Quirion et al. (Reference Quirion, Simek, Dávalos and Blossey2018) reported that the probability of eradication within 7 yr was 0.83 for stands of 0.34 m2, 0.26 for 45 m2, and only 0.02 for stands of more than 3,000 m2. The challenge in eradicating large stands has been reported elsewhere for Phragmites (Myers et al. Reference Myers, Heffernan, Clarke and Field2009) and other exotic plants (Rejmánek and Pitcairn Reference Rejmánek and Pitcairn2002; Taylor and Hastings Reference Taylor and Hastings2004).
Community Response to Treatments
The total percent cover of native species in the treated marsh increased from pretreatment (2009) through the following 3 yr that Phragmites was removed (Figure 2B; repeated-measures ANOVA F(3, 12) = 7.3; P = 0.005). In 2013, total native cover per plot ranged from 65.5 to 109.5. The increase in native cover from 2009 to 2013 was particularly great in Stands 1, 2, and 3, which were larger and had the highest Phragmites density and lowest native cover before treatments began; their native cover doubled during the course of the study (Figure 2B). Cover of exotic species besides Phragmites increased significantly from 2009 to 2013 (Figure 2C, repeated measures ANOVA F(3,12) = 5.0; P = 0.018). This effect was driven by changes in Stands 1 to 3, where non-Phragmites exotic cover more than tripled; their cover remained largely unchanged in Stands 4 and 5. Exotic species that increased in cover included A. calamus, yellow iris (Iris pseudacorus L.), and purple loosestrife (Lythrum salicaria L.).
After 3 yr of herbicide treatment, the sharp differences in community composition that were evident in 2009 had mostly disappeared. Treated and reference stands occupied similar ordination space, except for a cluster of treated plots in the larger Stands 1, 2, and 3 that were dominated by rice cutgrass [Leersia oryzoides (L.) Sw.] and common arrowhead (Sagittaria latifolia Willd.) plus the B. cernua/laevis complex (left side of Figure 3B). These species are primarily early-successional species that respond quickly to disturbed habitat. Both treatment and reference plots in Stand 5 were strongly associated with the Typha–Peltandra community identified in 2009 and plotted to the right of Figure 3B. The center of the NMDS ordination included a mixture of treatment and reference plots of mostly Stands 3 and 4, characterized by such species as I. capensis, A. calamus, and halberd-leaved tearthumb [Persicaria arifolia (L.) Haroldson].
Relatively few studies have previously documented the influence of Phragmites control on native species composition (Hazelton et al. Reference Hazelton, Mozdzer, Burdick, Kettenring and Whigham2014), but those that did reported outcomes resembling the responses we observed in the larger stands. Martin and Blossey (Reference Martin and Blossey2013) surveyed managers with respect to their restoration goals and outcomes and found that although some believed that management had increased the frequency or number of native species, few reported that management successfully restored preinvasion plant communities similar to what we saw in smaller stands. Farnsworth and Meyerson (Reference Farnsworth and Meyerson1999) reported that L. oryzoides and purple-stemmed beggarticks (Bidens connata Muhl. ex Willd.) were dominant 1 and 2 yr after Phragmites control treatments in a freshwater tidal wetland along the Connecticut River. Carlson et al. (Reference Carlson, Kowalski and Wilcox2009) found that B. cernua increased after Phragmites control in a nontidal wetland, as we did.
Synthesis: Effect of Herbicide Treatments on Community Composition
Three years of herbicide treatment led to a substantial decrease in Phragmites abundance and a recovery of the native plant community in all stands. In small stands, the community composition converged to resemble uninvaded reference ecosystems during the 3 yr of treatment. Meanwhile, native species cover in the large stands more than doubled as early-successional species colonized openings created by the loss of Phragmites. Other nonnative species also increased in cover following Phragmites removal in the larger stands, but they likely had less of an impact on community structure—for example, productivity and plant height—than would dense stands of Phragmites (Gagnon et al. Reference Gagnon, Gauthier and Lavoie2015; Lavoie Reference Lavoie2010). Though there are concerns in the literature about the environmental fate of glyphosate and surfactants used in this study (e.g., Battaglin et al. Reference Battaglin, Meyer, Kuivila and Dietze2014), chemical treatment was able to achieve the broad management goals set out at the start of the project.
Our results suggest that successful management and recovery of native biodiversity was more likely in smaller stands than larger stands. A number of Phragmites stems persisted in the larger stands even after 3 yr of treatment, to a much greater degree than in the smaller stands. Meanwhile, when we compared community composition of restored versus reference ecosystems, a common metric for assessing restoration success (e.g., Holl Reference Holl2002; Matthews and Spyreas Reference Matthews and Spyreas2010; McDonald et al. Reference McDonald, Gann, Jonson and Dixon2016; Ruiz-Jaen and Mitchell Aide Reference Ruiz-Jaen and Mitchell Aide2005), we saw more evidence of successful community recovery in smaller stands than larger ones. The larger Stands 1, 2, and 3 were mostly missing species characteristic of uninvaded habitat, while the species composition of the smaller Stands 4 and 5 converged toward that of reference ecosystems. Our findings are largely consistent with other studies that have found that exotic species control is more feasible in smaller stands than larger ones (Quirion et al. Reference Quirion, Simek, Dávalos and Blossey2018; Rejmánek and Pitcairn Reference Rejmánek and Pitcairn2002).
We caution, however, that this study reports responses within a relatively short time period relative to the longevity of the plant species and dynamics of tidal marsh ecosystems. We acknowledge that community composition in treated stands will likely continue to change beyond the initial responses we document here. Over time, the community in the larger stands could more closely resemble uninvaded tidal marsh habitat found in the reference areas (e.g., Matthews and Endress Reference Matthews and Endress2010). We hope that future studies will advance our understanding of marshlands’ responses to exotic species removal even further.
Given that exotic plant control is often undertaken with the specific goal of improving native plant biodiversity (Denslow and D’Antonio Reference Denslow and D’Antonio2005; Martin and Blossey Reference Martin and Blossey2013), more effort should be given to assessing the responses of the plant community and ecosystem processes (Hazelton et al. Reference Hazelton, Mozdzer, Burdick, Kettenring and Whigham2014). Such responses are likely to be highly context dependent, even within a single habitat or target species. Habitat managers’ ability to plan and design successful control strategies would greatly benefit from a wider foundation of studies that quantify the whole-community effects of exotic plant control.
Acknowledgments
This project was funded by the Hess Corporation and is an Environmental Benefits Project. It would not have been possible without the collaboration of Audubon New York and Scenic Hudson and without the assistance of their preserve managers: Larry Federman and Dan Sorenson. Roger Hoerl provided statistical guidance. Stuart Findlay, Troy Weldy, Jason Bried, Claude Lavoie, and two anonymous reviewers offered thoughtful comments on this article. Finally, we would especially like to thank all the interns and staff who assisted with the field data collection. No conflicts of interest have been declared.