Scientific Classification
Domain: Eukaryota
Kingdom: Plantae
Subkingdom: Tracheobionta
Superdivision: Spermatophyta
Division: Magnoliophyta
Class: Liliopsida
Subclass: Commelinidae
Order: Cyperales
Family: Poaceae
Genus: Arundo L.
Species: donax donax L. (commonly notated as A. donax L.)
Synonyms past and present include (from GBIF [Reference Stover, Keller, Dudley and Langendoen2021] unless otherwise cited): Arundo donax f. donax L., Arundo donax var. donax L., Arundo donax collina Ten. (Danin et al. Reference Danin, Raus and Scholz2002, Reference Danin, Domina and Raimondo2006), Arundo collina Ten. (Danin Reference Danin2004), Arundo donax glauca Bubani, Arundo glauca Bubani, Arundo donax latifolia Salisb., Arundo hellenica Danin, Raus & H. Scholz (Danin et al. Reference Danin, Raus and Scholz2002), Arundo mediterranea Danin (Danin Reference Danin2004), Arundo donax sativa Lam., Arundo bifaria Retz., Arundo bengalensis Retz., Arundo aegyptia Delile, Arundo aegyptiaca E. Vilm., Arundo bambusifolia Hook.f, Arundo coleotricha (Hack.) Honda, Arundo collina Ten. (Danin Reference Danin2004), Arundo latifolia Salisb., Arundo sativa Lam., Arundo scriptoria L., Arundo triflora Roxb. Aira bengalensis (Retz.) J. F. Gmel., Amphidonax bengalensis Roxb., Amphidonax bifaria (Retz.) Steud., Scolochloa arundinacea (P. Beauv.) Mert. & Koch, Scolochloa arundinacea (P.Beauv.) Mert. & W.D. J. Koch, S. donax (L.) Gaudin, Cynodon donax (L.) Raspail, Donax arundinaceus P. Beauv., Donax bengalensis (Retz.) P.Beauv., Donax donax (L.) Asch. & Graebn., and Donax sativus C. Presl. A cultivated variety with variegated leaves is often called Arundo donax L. var. versicolor (P. Mill.) Stokes. Synonyms for this variety include: Arundo donax versicolor P. Mill. (DiTomaso and Healy Reference DiTomaso and Healy2003; Hsiao et al. Reference Hsiao, Jacobs, Barker and Chatterton1998; Missouri Botanic Garden 2008), Arundo donax var. versicolor (Mill.) Stokes, Arundo donax ssp. versicolor (P. Mill) Stokes, and Donax versicolor (Mill.) P. Beauv. Other varieties not widely recognized but with occurrences in GBIF (Reference Stover, Keller, Dudley and Langendoen2021) include Arundo donax var. angustifolia Döll, Arundo donax var. coleotricha Hack, and Arundo donax var. variegata E. Vilm.
EPPO Code: ABKDO
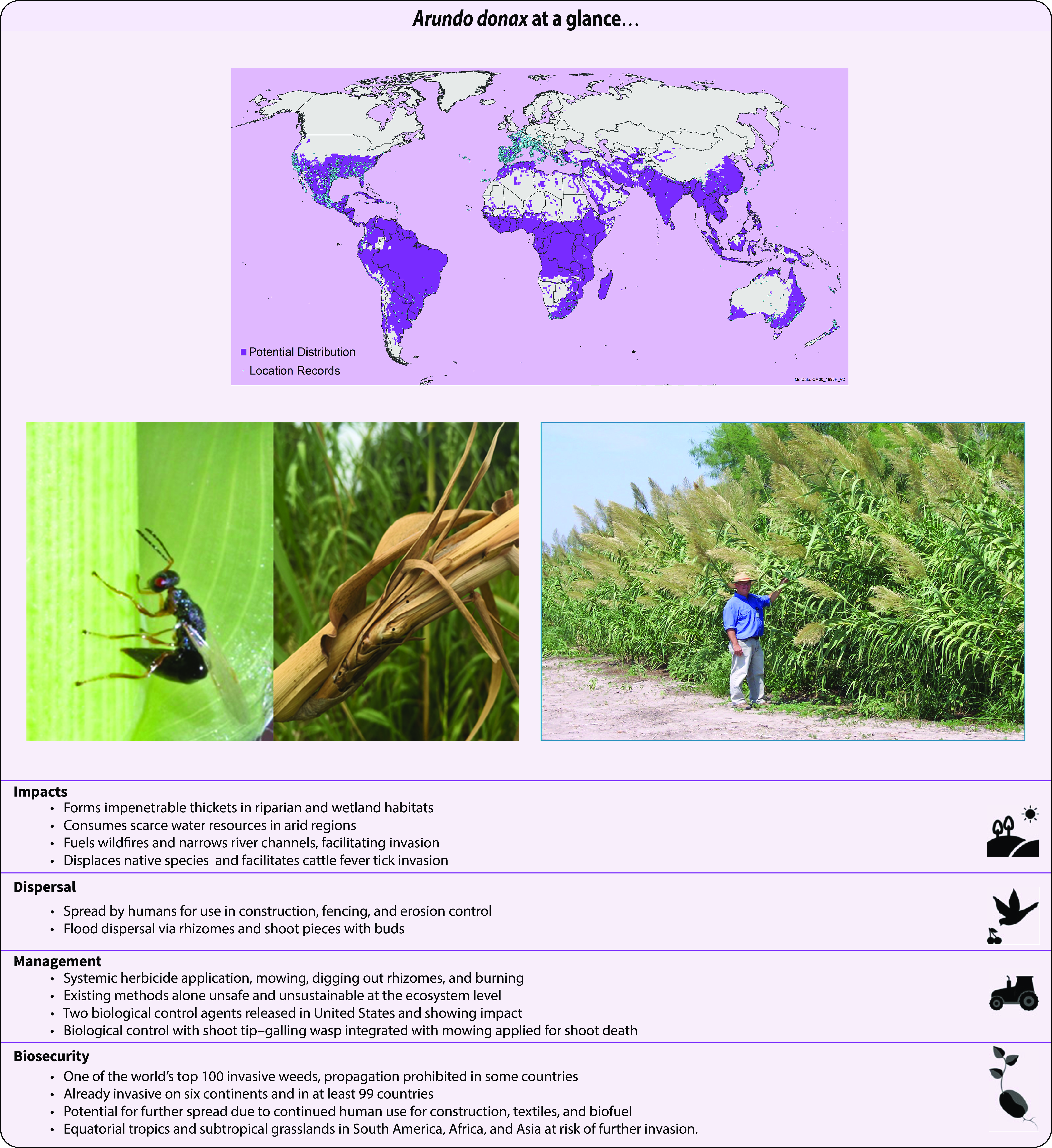
Names and Taxonomy
This species has many common names. Common names in North America include: giant reed, arundo, carrizo cane, bamboo reed (United States), carrizo, carrizo gigante (Mexico), caña India (Cuba); in South America: caña de Castilla (Argentina), cañaveral (Chile), carizo (Bolivia); in South Africa: Spanish reed, Spaanseriet (Sutton et al. Reference Sutton, Bownes, Visser, Mapaura and Canavan2021); in Australia and New Zealand: donax reed, elephant grass, reed cane, reed grass, Spanish reed, giant cane, wild cane; in Europe: Spanisches Rohr, Pfahlrohr (Denmark), giant reed, Spanish cane, bamboo reed (United Kingdom), Spaanseriet, pijlriet, zaairiet, (Netherlands), gewöhnliches Pfahlrohr, italienisches pfahlrohr, pfahlrohr, pfeilrohr, riesenpfahlrohr, riesenschilf, spanisches rohr (Germany), lasecznica trzcinowata (Poland), canne de Provence, arondo donax, canneville, grand roseau, quenouille, roseau canne, roseau de Fréjus, roseau des jardins, roseau donax, roseau à quenouilles (France), caña, caña gigante, caña de techar, caña de la reina, caña de Castilla, carrizo grande, caña común, caña india, cañizo (Spain), kanabera (Basque region of France/Spain), canya, canya communa, canavèra, cannabère (Catalonia region of Spain), cana, canas, cana-comun, cana-do-brejo, cana de roca, cana-do-reino, cana-vieira, cannodo-reino, capim-plumoso, canamilha, caninha (Portugal), canna, canna commune, canna domestica, canna gargana, canna gentile (Italy), kallami (Albania), trestie italiană (Romania), trsť obrovská (Slovakia), navadna kanela (Slovenia), obični trst (Croatia), καλάμι (Greece); in the Middle East: avkaneh shachiach (Israel); in Asia: lú zhú (China), danchiku, yoshitake (Japan), mul dae (Korea).
Importance
Arundo donax is native to the Old World, possibly from the Iberian Peninsula of Europe to south Asia, including North Africa, the Middle East, the Arabian Peninsula, and the Persian Gulf regions (Hardion et al. Reference Hardion, Verlaque, Saltonstall, Leriche and Vila2014, Reference Hardion, Verlaque, Rosato, Rossello and Vila2015, Reference Hardion, Verlaque, Haan-Archipoff, Cahen, Hoff and Vila2017; Tarin et al. Reference Tarin, Manhart, Pepper, Goolsby, Moran, Contreras Arquieta and Kirk2013). The true native range is, however, most likely limited to the Indus River basin and surrounding areas of India, Bhutan, Nepal, Pakistan, Afghanistan, Iran, Turkmenistan, and Uzbekistan, extending possibly to the Middle East (Jiménez-Ruiz et al. Reference Jiménez-Ruiz, Hardion, Del Monte, Vila and Santín-Montanyá2021; Sutton et al. Reference Sutton, Bownes, Visser, Mapaura and Canavan2021), with areas to the west and east of that area being ancient or modern introductions. Arundo donax was cultivated in Asia and Europe for thousands of years for use as a construction material (Dunmire Reference Dunmire2004) and has been widely introduced around the world for use in fencing and construction (Bell Reference Bell, Brock, Wade, Pysek and Green1997; Guthrie Reference Guthrie2007; Haddadchi et al. Reference Haddadchi, Gross and Fatemi2013) (see images in the Supplementary Appendix); for use as a fiber for example, to make baskets (Jiménez-Ruiz et al. Reference Jiménez-Ruiz, Hardion, Del Monte, Vila and Santín-Montanyá2021); as an ornamental; and for use as reeds in woodwind instruments (Perdue Reference Perdue1958; Tracy and DeLoach Reference Tracy, DeLoach and Bell1999). Subsequently, it has become naturalized and invasive in many tropical, subtropical, and warm temperate regions of the world (Figures 1–3). It was introduced to South Africa in the late 1700s for erosion control (Guthrie Reference Guthrie2007) and to Australia in the 1800s (Virtue et al. Reference Virtue, Reynolds, Malone, Preston and Williams2010). Arundo donax is known for its rapid growth, attaining growth rates of 0.7 m wk−1 or 10 cm d−1, putting it among the fastest-growing plants (Bell Reference Bell, Brock, Wade, Pysek and Green1997; Perdue Reference Perdue1958), producing up to 1.4 × 105 kg dry biomass ha−1 in California, USA (Cal-IPC 2020) and 5.4 × 104 kg ha−1 in Italy (Angelini et al. Reference Angelini, Ceccarini and Bonari2005). Because of A. donax’s growth potential and broad environmental tolerance (Jiménez-Ruiz et al. Reference Jiménez-Ruiz, Hardion, Del Monte, Vila and Santín-Montanyá2021), its use for biofuel production (Duke Reference Duke1983; Mack Reference Mack2008; Mariani et al. Reference Mariani, Cabrini, Danin, Piffanelli, Fricano, Gomarasca, Dicandilo, Grassi and Soave2010) and sewage treatment, specifically heavy metal removal (Cristaldi et al. Reference Cristaldi, Conti, Cosentino, Mauromicale, Copat, Grasso, Zuccarello, Fiore, Restuccia and Ferrante2020), have been explored but are largely unrealized. In South Africa, the cost and energy to harvest and transport biomass from invasive populations for use as biofuel negated the benefits (Melane et al. Reference Melane, Ham and Meincken2017).
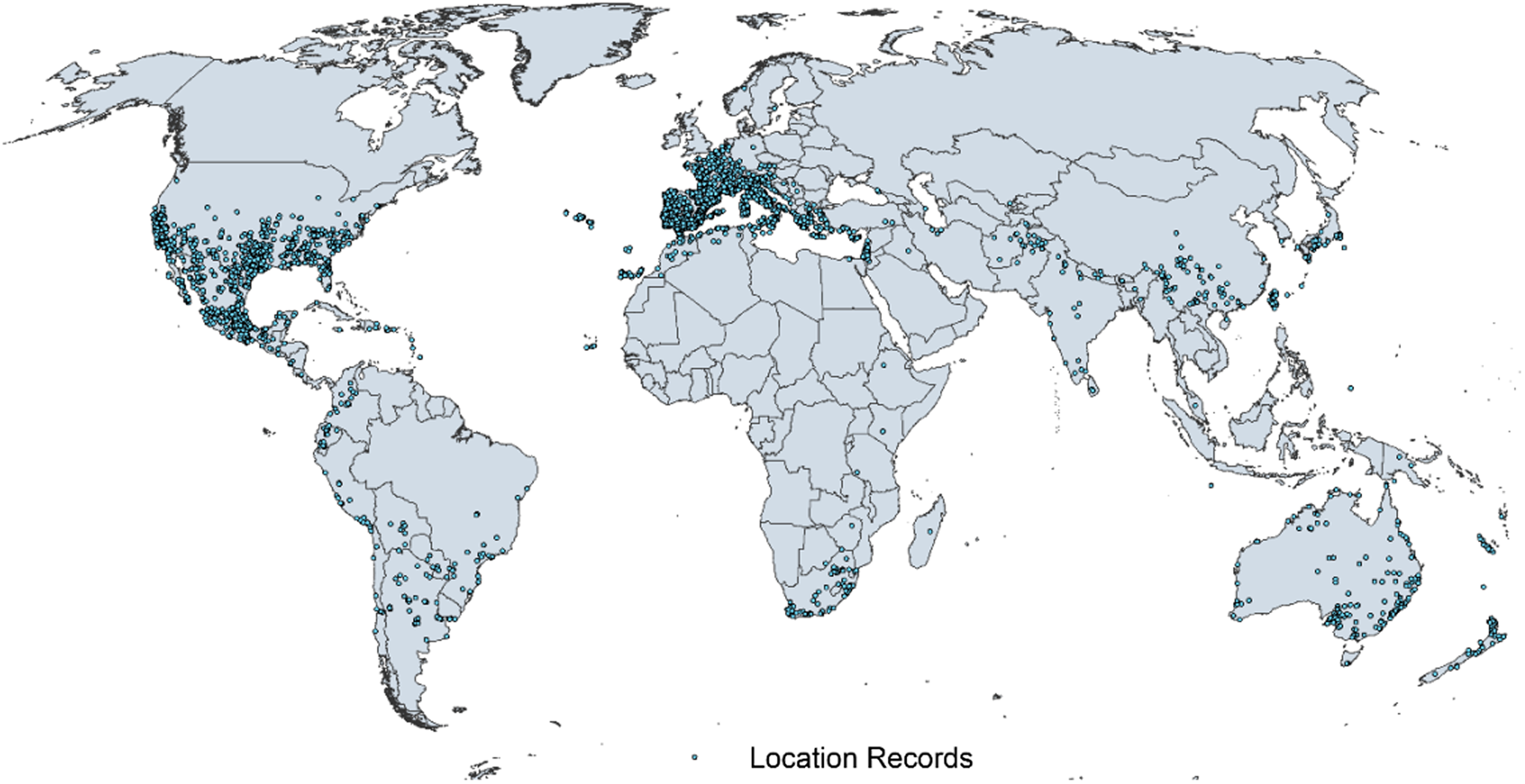
Figure 1. Native and introduced occurrences of Arundo donax across the world. Source: GBIF (Reference Stover, Keller, Dudley and Langendoen2021).
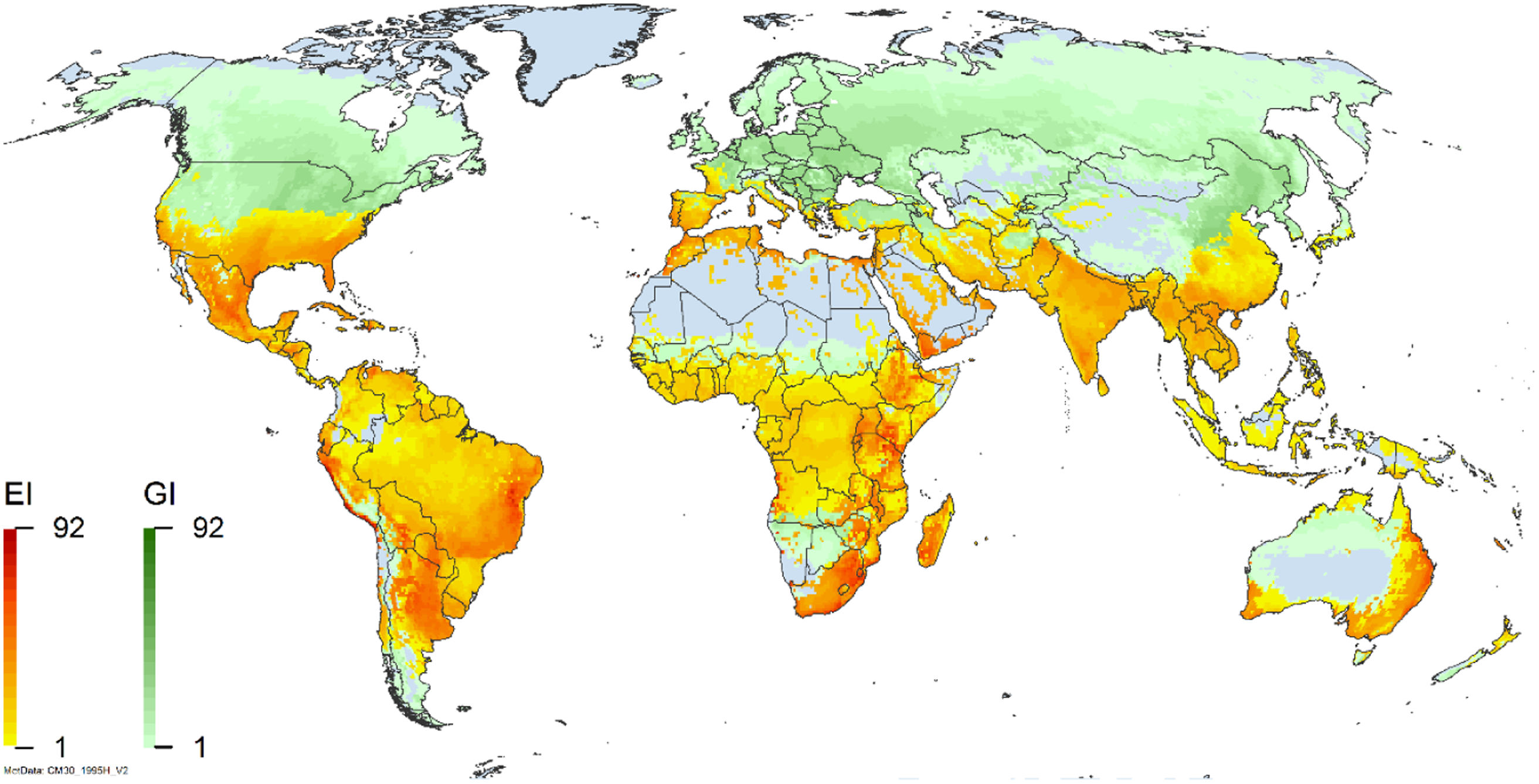
Figure 2. Global climate suitability for A. donax modeled using CLIMEX. The model incorporates both natural rainfall and a top-up irrigation scenario, applied where irrigation is reported in a global map of irrigated areas (Siebert et al. Reference Siebert, Henrich, Frenken and Burke2013). The yellow to red areas (environmental index [EI] ≥1) indicate increasing suitability for year-round population persistence. Outside of those areas, the growth index ([G1] ≥ 1) indicates areas in green that are suitable for growth of A. donax only during a favorable growing season, and are thus expected to be unsuitable for population persistence except in cases of direct human intervention. See Structured Appendix for model details.

Figure 3. Global climate suitability as modeled in CLIMEX (Kriticos et al. Reference Kriticos, Maywald, Yonow, Zurcher, Herrmann and Sutherst2015) for A. donax overlaid on the global distribution. Location records from GBIF (Reference Stover, Keller, Dudley and Langendoen2021).
The detrimental impacts of Arundo donax invasion outweigh the plant’s beneficial uses. This giant grass forms dense, monospecific stands along waterways (Figure 4). The high water usage of A. donax, which causes water waste/loss, is of greatest concern in countries where major invasions have occurred, including Australia (Haddadchi et al. Reference Haddadchi, Gross and Fatemi2013), New Zealand (Virtue et al. Reference Virtue, Reynolds, Malone, Preston and Williams2010), South Africa (Nkuna et al. Reference Nkuna, Visser, Wilson and Kumschick2018; Versfeld et al. Reference Versfeld, Le Maitre and Chapman2000), the United States (Cal-IPC 2020; Lambert et al. Reference Lambert, D’Antonio and Dudley2010a, 2010b; Seawright et al. Reference Seawright, Rister, Lacewell, McCorkle, Sturdivant, Yang and Goolsby2009), and Mexico (IMTA Reference Pillay2008; Martínez Jiménez et al. Reference Martínez Jiménez, Goolsby, Racelis, Perez de Leon and Negrete Arroyos2017). For example, A. donax is considered a national problem in South Africa, primarily due to the threat it poses to water security (Milton Reference Milton2004), as it is the most abundant and widespread invasive alien grass in the country (Tshapa et al. Reference Tshapa, Naidoo and Naidoo2021; Visser et al. Reference Visser, Wilson, Canavan, Canavan, Fish, Maitre, Nänni, Mashau, O’Connor, Ivey, Kumschick and Richardson2017) and is legally categorized as requiring compulsory inclusion in invasive species control programs. A study by Nkuna et al. (Reference Nkuna, Visser, Wilson and Kumschick2018) ranked A. donax as having the second-highest impact score (environmental and socioecological) of the 58 alien grasses assessed in South Africa. Arundo donax is not yet considered to be a major invader in Australia or New Zealand, although it has become widespread especially in the southeastern mainland states of Australia and the North and South Islands of New Zealand (Haddadchi et al. Reference Haddadchi, Gross and Fatemi2013). The New Zealand Department of Conservation has assigned it a high priority for management, because A. donax can form dense tall patches, is hard to control, and represents a serious future threat to biodiversity values.

Figure 4. Dense stand of A. donax on the banks of the Rio Grande on the border of Texas (USA) and Mexico.
A study by Versfeld et al. (Reference Versfeld, Le Maitre and Chapman2000) estimated the water use from infestations is about 4.35 million m3 yr−1 across all provinces in South Africa, exacerbating its invasion of ecologically sensitive biomes such as the fynbos, South Africa’s Cape Floristic Region and plant biodiversity hot spot, with high levels of endemism. The annual water usage of this plant in another biodiverse area, the lower Rio Grande basin of Texas, USA, and northern Mexico, was estimated at 56.2 million m3 yr−1 (Seawright et al. Reference Seawright, Rister, Lacewell, McCorkle, Sturdivant, Yang and Goolsby2009). The plant’s estimated water use in the Central Valley of California, USA, was estimated at 37.8 million m3 yr−1 (Cal-IPC 2020). Arundo donax competes for water resources in arid regions where these resources are critical to the environment and agricultural and municipal users (Cal-IPC 2020; Cloern et al. Reference Cloern, Knowles, Brown, Cayan, Dettinger, Morgan, Schoellhamer, Stacey, van der Wegen, Wagner and Jassby2011; Goolsby et al. Reference Goolsby, Moran, Adamczyk, Kirk, Jones, Marcos and Cortés2009a; Jiménez-Ruiz et al. Reference Jiménez-Ruiz, Hardion, Del Monte, Vila and Santín-Montanyá2021; McGaugh et al. Reference McGaugh, Hendrickson, Bell, Cabral, Lyons, McEachron, Munoz, Lozano-Vilano and Contreras-Balderas2006; Seawright et al. Reference Seawright, Rister, Lacewell, McCorkle, Sturdivant, Yang and Goolsby2009; Watts and Moore Reference Watts and Moore2011). For example, a study using ground-based scintillometers in Laredo, TX, USA, found that A. donax growing in February (winter) used similar amounts (5.2 mm d−1) of water as corn growing in this region during the summer (Gowda et al. Reference Gowda, Goolsby, Yang, Basu, Racelis and Howell2011); similar levels of water use were found in this region using tower-mounted eddy-covariance systems (Racelis et al. Reference Racelis, Wagle, Escamilla, Goolsby and Gowda2022). Several studies (Kui et al. Reference Kui, Li, Moore and West2013; Moore et al. Reference Moore, Li, Kui and West2016) found that A. donax rhizome mats along the Rio Grande can transfer water through rhizomes to increase their growth rate in parts of the stand where soil moisture is limiting. In Texas, peak water evapotranspiration in a greenhouse study was 8.8 mm d−1 m−2 shoots (Watts and Moore Reference Watts and Moore2011). In California, field stands of A. donax used as much as 40 mm of water d−1 m−2 during the peak of the growing season; this rate of water use is at the high end of the spectrum for plants (Cal-IPC 2020). Studies in Europe on A. donax in constructed wetlands set up for biofuel production indicated evaporative water use/loss at 11 to 47 mm m−2 d−1 in summer (Milani et al. Reference Milani, Marzo, Toscano, Consoli, Cirelli, Ventura and Barbagallo2019; Tuttolomondo et al. Reference Tuttolomondo, Licata, Leto, Leone and La Bella2015).
Arundo donax has numerous adverse effects on the physical characteristics of riparian ecosystems. In the United States (Cushman and Gaffney Reference Cushman and Gaffney2010; Decruyenaere and Holt Reference Decruyenaere and Holt2005) and South Africa (Henderson Reference Henderson2001), A. donax has been designated as a “transformer” species in the region due to its ability to alter the structure, integrity, and functioning of the ecosystems that it invades. Dense stands reduce the carrying capacity of waterways by narrowing channels in the southwestern United States (Cal-IPC 2020) (Figure 4; see images in the Supplementary Appendix) and in South Africa (Guthrie Reference Guthrie2007; Holmes et al. Reference Holmes, Richardson, Esler, Witkowski and Fourie2005). During flood events, the shallow rhizomes destabilize riverbanks, increasing erosion (Frandsen and Jackson Reference Frandsen, Jackson, Jackson, Frandsen and Duthoit1994; Stover et al. Reference Stover, Keller, Dudley and Langendoen2018), and the narrowed channels can exacerbate flood damage (Spencer et al. Reference Spencer, Colby and Norris2013) by dislodging mats of shoots that then threaten infrastructure (Cal-IPC 2020). Arundo donax fuels wildfires in riparian habitats, increasing fire risk due to its dense dead/dormant biomass (Guthrie Reference Guthrie2007) acting as a vertical fuel source and increasing fire intensity (Coffman et al. Reference Coffman, Ambrose and Rundel2010; Holmes et al. Reference Holmes, Richardson, Esler, Witkowski and Fourie2005). In the Central Valley of California, fire frequency in watersheds is positively correlated with the area of A. donax invasion (Cal-IPC 2020), and in coastal watersheds, fires promote spread of this weed into native riparian vegetation and impair native plant recovery (Lambert et al. Reference Lambert, D’Antonio and Dudley2010a). Increased eutrophication in river systems controlled by reservoirs and dams, and the control of water flow itself, contribute to the spread and persistence of A. donax globally (Frandsen and Jackson Reference Frandsen, Jackson, Jackson, Frandsen and Duthoit1994; Guthrie Reference Guthrie2007; Haddadchi et al. Reference Haddadchi, Gross and Fatemi2013), as this weed benefits from excess nitrogen (Lambert et al. Reference Lambert, Dudley and Robbins2014; Moran Reference Moran2015; Quinn et al. Reference Quinn, Rauterkus and Holt2007). Dense A. donax stands block access to water and reduce its recreational benefits (Cal-IPC 2020).
Invasion by A. donax leads to loss of plant biodiversity, as demonstrated by studies along the Rio Grande in Texas (Racelis et al. Reference Racelis, Davey, Goolsby, Pérez de León, Varner and Duhaime2012c; Rubio et al. Reference Rubio, Racelis, Vaughan and Goolsby2014) and in California (Bell Reference Bell, Brock, Wade, Pysek and Green1997; Cushman and Gaffney Reference Cushman and Gaffney2010; Quinn and Holt Reference Quinn and Holt2008). In the United States (most studies conducted in California), A. donax invasion reduces diversity of insects (Herrera and Dudley Reference Herrera and Dudley2003; Lovich et al. Reference Lovich, Ervin and Fisher2009), birds and reptiles (Cal-IPC 2020; Dudley Reference Dudley, Bossard, Randal and Hosovsky2000; Tracy and Deloach Reference Tracy, DeLoach and Bell1999), and rare fish populations (Tracy and DeLoach Reference Tracy, DeLoach and Bell1999), likely causing local (Cuatrocienegas, Coahuila) extinction of an endemic Mexican fish species, the Rio Salado darter [Etheostoma segrex (Norris and Mickley); Percidae] (McGaugh et al. Reference McGaugh, Hendrickson, Bell, Cabral, Lyons, McEachron, Munoz, Lozano-Vilano and Contreras-Balderas2006). Top carnivores such as coyotes (Canis latrans Say) and bobcats (Lynx rufus Schreber) tend to avoid A. donax, creating a partial refuge for small mammal prey (Hardesty-Moore et al. Reference Hardesty-Moore, Orr and McCauley2020). The threats of A. donax to biodiversity are recognized in South Africa (Guthrie Reference Guthrie2007), Australia (Haddadchi et al. Reference Haddadchi, Gross and Fatemi2013), and in parts of the ancient European introduced range where human disturbance has aided invasion (Jiménez-Ruiz et al. Reference Jiménez-Ruiz, Hardion, Del Monte, Vila and Santín-Montanyá2021). In the lower Rio Grande basin of Texas, the A. donax invasion represents the first time an exotic weed has been shown to facilitate invasion of an exotic livestock pest, the cattle fever tick [Rhipicephalus microplus (Canestrini)] (Racelis et al. Reference Racelis, Rubio, Vaughan and Goolsby2012a).
Arundo donax is an alternate host for several viruses of crop plants (Tracy and DeLoach Reference Tracy, DeLoach and Bell1999) and also of several fungi that are plant pathogens (Duke Reference Duke1983). Surveys in California indicated that it is a host plant for the blue-green sharpshooter [Graphocephala atropunctata (Signoret); Hemiptera: Cicadellidae], which can vector the bacterium that causes Pierce’s disease of grapes (Vitis vinifera L. or other species/hybrids) (Herrera and Dudley Reference Herrera and Dudley2003). An adventive leaf-feeding aphid [Melanaphis donacis (Passerini); Hemiptera: Aphididae] is known from California (Dudley et al. Reference Dudley, Lambert, Kirk, Kawagama, Julien, Sforza, Bon, Evans, Hatcher, Hinz and Rector2008), South America (Underraga et al. Reference Underraga, Araya, Zuazúa and Alonso2020), and South Africa (Canavan et al. Reference Canavan, Paterson, Hill and Dudley2019) and is spreading invasively in the native range of A. donax in Pakistan (Amin et al. Reference Amin, Bodlah, Qasin and Jajja2019), where it is considered a pest on that plant as well as on common reed [Phragmites australis (Cav.) Trin. ex Steud.; syn.: Phragmites communis Trin.] and on some members of the Rosaceae. A total of 14 other aphid species occur on A. donax across the world, including several pests of sugarcane (Saccharun officinarum L.), wheat (Triticum aestivum L.), and other crops (Blackman and Eastop Reference Blackman and Eastop2022). Dudley et al. (Reference Dudley, Lambert, Kirk, Kawagama, Julien, Sforza, Bon, Evans, Hatcher, Hinz and Rector2008) reported stem tip-mining and rot damage indicative of the adventive presence of a Cryptonevra sp. fly species (Diptera: Chloropidae). Flies of this genus from Mediterranean Europe have been considered for biological control (Thomas and Goolsby Reference Thomas and Goolsby2020); their adventive presence in the United States is unconfirmed. The fly Chaetopsis massyla Walker (Diptera: Ulidiidae), native to North America, was found in shoot tips of A. donax grown in south Texas for mass-rearing of biological control agents (Goolsby and Mangan Reference Goolsby and Mangan2010).
Description
Lewandowski et al. (Reference Lewandowski, Scurlock, Lindvall and Christou2003) and Jiménez-Ruiz et al. (Reference Jiménez-Ruiz, Hardion, Del Monte, Vila and Santín-Montanyá2021) provide botanical descriptions of A. donax in the United States and Mediterranean Europe, respectively. Immature and mature plant: Stem and leaves are glabrous (waxy). Stem canes are erect, semi-woody, inflexible, about 1- to 4-cm thick, up to 6-m tall in wild European populations (Jiménez-Ruiz et al. Reference Jiménez-Ruiz, Hardion, Del Monte, Vila and Santín-Montanyá2021) and 6 to 8 m in biofuel plantings there (Angelini et al. Reference Angelini, Ceccarini and Bonari2005) and in the wild in South Africa (Sutton et al. Reference Sutton, Bownes, Visser, Mapaura and Canavan2021); up to 9 m in California (Perdue Reference Perdue1958); and generally 3 to 6 m in south Texas (Goolsby et al. Reference Goolsby, Moran, Racelis, Summy, Martínez-Jiménez, Lacewell, Perez de Leon and Kirk2016). Stems have hollow internodes. First-year green canes have unbranched stems (Figures 5 and 6) of the same diameter as older canes, but are more pliable. Older canes are often branched, sometimes with leaves only on the branches. Leaves alternate in the same plane (two-ranked), fairly evenly spaced along the stem length. Leaf blades are flat, less than 0.75-m long, mostly 2- to 6-cm wide at the base; the midsection and base are nearly equal in width but taper to a fine point; margins are sharp and rough (scabrous) to touch. Blade bases are broadly round-lobed (Figure 6) to slightly truncate, clasping the stem; margin often has long, wavy hairs. Leaf bases include a membranous, upright collar (ligule) about 1- to 2-mm long without hairs (Figure 6). Leaf sheaths tightly envelop the stem from the blade base to the next node down the stem, splitting open at the margin only when leaf sheaths senesce (Figure 6). Roots and underground structures: Rhizomes are creeping, thick, scaly, solid in cross section, often forming a dense network, and firm and knotty at the stem bases (Figure 6), forming a shallow network of rhizomes and roots (Kui et al. Reference Kui, Li, Moore and West2013; Stover et al. Reference Stover, Keller, Dudley and Langendoen2018), but with individual roots extending as deep as 5 m below the surface (Moore et al. Reference Moore, Li, Kui and West2016). New buds are pink as they emerge from dead root scales, then turn green as they form shoots. Rhizomes and stem fragments with a node readily develop roots and new shoots under suitable conditions (damp soil, silt, sand, or gravel; Cal-IPC 2020; Decruyenaere and Holt Reference Decruyenaere and Holt2001; Goolsby et al. Reference Goolsby, Racelis, Goolsby, Kirk, Cristofaro, Grusak and Perez de Leon2013; Wijte et al. Reference Wijte, Mizutani, Motamed, Merryfield, Miller and Alexander2005). Inflorescences: Panicles (flower/seed stalks) are terminal (Figures 5 and 6), dense, erect, and plume-like, mostly 30- to 60-cm long and 5- to 10-cm wide, silvery cream-colored to purplish or brown, open with ascending branches or contracted (especially after pollination) with nearly erect branches. Spikelets (clusters of florets on a branch of the panicle) are mostly 10- to 15-mm long, with two to five flowers, and detach from above the glumes and between florets. Floret stalk (rachilla) is glabrous (smooth). Glumes (two leaf-like bracts at the base of each spikelet) are three to five veined and membranous. The lemma (a leaf-like bract at the base of each floret) is ca. 11-mm long, with a short-awned (spined) tip between two teeth, appearing as “tears” between three veins. The basal one-fourth of the lemma length is covered with long (5-mm) silky hairs. The palea (a shorter bract on the other side of each floret) is 7 mm, without a spine, and truncated at the apex. Flowering occurs from March to November in temperate regions, but year-round in subtropical and tropical areas. Florets from the United States and Mediterranean do not produce seeds (Hardion et al. Reference Hardion, Verlaque, Rosato, Rossello and Vila2015), likely due to a failure of the mother megaspore to divide (Bhanwra et al. Reference Bhanwra, Choda and Kumar1982). Arundo donax is sterile throughout its invasive New World, African, and Australasian distribution and its ancient introduced Mediterranean range. In its native range in the Indo-Pakistan and Persian Gulf regions, A. donax produces seed (Hardion et al. Reference Hardion, Verlaque, Saltonstall, Leriche and Vila2014). Postsenescence characters: Dead canes lose their leaves, turn brown and then gray, and become brittle and splintery after completion of the 3- to 4-yr life cycle (Spencer et al. Reference Spencer, Ksander and Whitehand2005; Thornby et al. Reference Thornby, Spencer, Hanan and Sher2007), but may remain dead and erect for several years. They eventually fall over and then decompose on-site or are dispersed by flooding or fire events. Effects of control on appearance: Control with herbicides causes leaf blades to first droop and then senesce, but they may remain on canes, falling off during wind events or flooding, leaving bare dead canes that eventually fall over. Control with fire or mechanical removal causes immediate collapse of canes, leaving rough bare ground with blackened, exposed rhizomes. Regrowth after chemical control, if it occurs at all, is distorted and chlorotic (Bell Reference Bell2011), with many small side shoots emerging from nodes of bare canes, forming a club-like witches’ broom. Regrowth after fire consists of thick, dense canes emerging from rhizomes, as A. donax is well adapted to fire (Coffman et al. Reference Coffman, Ambrose and Rundel2010).

Figure 5. Arundo donax with flower panicles in south Texas, USA. In subtropical regions such as this, flowering can occur any time of the year.

Figure 6. Key external features of A. donax and its close relative, Phragmites australis. (A) Arundo donax stand showing 3- to 6-m-tall stem stature and (B) upright inflorescences (panicles). (C) Phragmites australis stand showing 1- to 2-m stature and (D) drooping panicles. (E) Young A. donax shoot showing leaf sheaths tightly wrapped around stem from top to bottom of the internode. (F) On 1-yr-old A. donax shoots, only old, dead leaf sheaths show pronounced splitting from stem. P. australis leaf sheaths are split from the stem on both young (G) and old (H) stems. (I) Base of A. donax leaf blade showing ear-like auricles and collar without hairs, in contrast to (J) base of P. australis leaf blade lacking prominent auricles and with hairy fringe at base of the collar. (K) Tuber-like A. donax rhizome with dense, stubby, sharp-tipped leafy scales and thick pink or red buds. (L) Thinner P. australis rhizome with few leafy, not sharp scales and thin buds. All photos by K. Santa Cruz, USDA-ARS.
Morphologically Similar Species
Two congeners widespread in the Mediterranean Basin, Arundo plinii Turra and Arundo micrantha Lam., can be distinguished by their thinner rhizomes with parenchymous cross section with a small lumen (hole) in the center (as opposed to the solid A. donax rhizome cross section with no central lumen (Jiménez-Ruiz et al. Reference Jiménez-Ruiz, Hardion, Del Monte, Vila and Santín-Montanyá2021).
Phragmites australis is a widespread perennial up to 4 m tall (but up to 6 m in Europe; McKee and Richards Reference McKee and Richards1996) that is cosmopolitan in distribution (Hardion et al. Reference Hardion, Verlaque, Haan-Archipoff, Cahen, Hoff and Vila2017). In the United States, it occurs in native, nonnative and invasive, and hybrid forms that can be distinguished with molecular techniques (Lambert et al. Reference Lambert, Saltonstall, Long and Dudley2016; Meyerson et al. Reference Meyerson, Lambert and Saltonstall2010; Saltonstall Reference Saltonstall2016). Key features distinguishing A. donax from P. australis are summarized and illustrated by Jiménez-Ruiz et al. (Reference Jiménez-Ruiz, Hardion, Del Monte, Vila and Santín-Montanyá2021), and some of these features are shown in Figure 6. Phragmites australis stems/canes may be shorter than those of A. donax and are often less than 1 cm in diameter, but those features alone are not conclusive. Leaf ligules (collars clasping the stem) consist of an upright membrane, usually less than 1-mm long, that is ciliate with hairs ±1-mm long. Arundo donax canes are often much thicker in diameter, and the leaf collar ligule has no hairs. The bases of P. australis leaves are gradually narrowed or truncate, while those of A. donax have more clearly defined auricles or lobes around the stem. Phragmites australis canes bear drooping inflorescences (panicles), while A. donax panicles are upright. The floret stalks (rachillae) of P. australis are covered with long, silky hairs, while A. donax rachillae are glabrous. Floret lemmas on P. australis are glabrous and tapered to a point, as compared with A. donax having hairs on the basal quarter of the lemma with a spine and two “teeth” at the apex. Arundo donax rhizomes are solid in cross section, while P. australis rhizomes are hollow with a ring of cortical aerenchyma and a central lumen. Phragmites australis, unlike A. donax, can produce viable seed. Seed production varies with climate and genotype (McKee and Richards Reference McKee and Richards1996), including degree of hybridization of native and invasive genotypes (Ishii and Kadono Reference Ishii and Kadono2002) and degree of local clonal reproduction, as plants are largely or completely self-incompatible. Hybrid plants produce more seed than parental lineages but with lower viability (Williams et al. Reference Williams, Lamber, Long and Saltonstall2019). Most seeds germinate mid- to late spring. Submersed seeds do not germinate until water recedes. Seeds are short-lived under field conditions, and a persistent seedbank does not accumulate.
Napier grass or Uganda grass [Pennisetum purpureum Schumach.; syn.: Cenchrus purpureus (Schumach.) Morrone], native to tropical African grasslands and introduced in subtropical Eurasia and North and South America, appears superficially similar to A. donax in growth habit and can occur in similar habitats, but the leaf bases are hairy well beyond the ligule/sheath junction, the inflorescences are much more narrow and are shorter (to 20-cm long) than those of A. donax, and asexual reproduction occurs by stolons rather than rhizomes (Farrell et al. Reference Farrell, Simons and Hillocks2002). Several large-statured bunchgrasses, including pampasgrass [Cortaderia selloana (Schult. & Schult. f.) Asch. & Graebn.] and Ravenna grass [Saccharum ravennae (L.) L.] have superficially similar inflorescences but entirely different growth forms with profuse large, narrow, pointy leaves clustered near the base of the plant.
Distribution
Arundo donax is distributed globally across the subtropical and warm temperate regions of the world, with more limited occurrences in equatorial regions and isolated occurrences in colder temperate areas. The Global Biodiversity Information Facility (GBIF Reference Stover, Keller, Dudley and Langendoen2021) lists 50,345 occurrences. National databases and past reviews (Jiménez-Ruiz et al. Reference Jiménez-Ruiz, Hardion, Del Monte, Vila and Santín-Montanyá2021; Martínez Jiménez et al. Reference Martínez Jiménez, Goolsby, Racelis, Perez de Leon and Negrete Arroyos2017) provide additional information. Arundo donax is found in at least 99 countries and is invasive across at least 89 countries and islands that are part of countries, befitting its status as one of the top 100 invasive species (of any type) in the world (Lowe et al. Reference Lowe, Browne, Boudjelas and De Poorter2000). Figure 1 shows worldwide occurrence of A. donax (GBIF Reference Stover, Keller, Dudley and Langendoen2021), Figure 2 shows a CLIMEX model (Kriticos et al. Reference Kriticos, Maywald, Yonow, Zurcher, Herrmann and Sutherst2015) informed by CliMond globally interpolated climate data (Kriticos et al. Reference Kriticos, Webber, Leriche, Ota, Macadam, Bathols and Scott2012), and Figure 3 overlays the distribution on the model. Figure 7 shows the distribution overlaid on the CLIMEX model for North and Central America and the Caribbean. Figure 8 shows similar information for Europe, the Mediterranean Basin, and far western Asia, including the native range of A. donax. Country lists are inferred from records in GBIF (Reference Stover, Keller, Dudley and Langendoen2021) with additional information from Soreng et al. (Reference Soreng, Davidse, Peterson, Zuloaga, Judziewicz, Filgueiras and Morrone2014) and other sources as referenced.
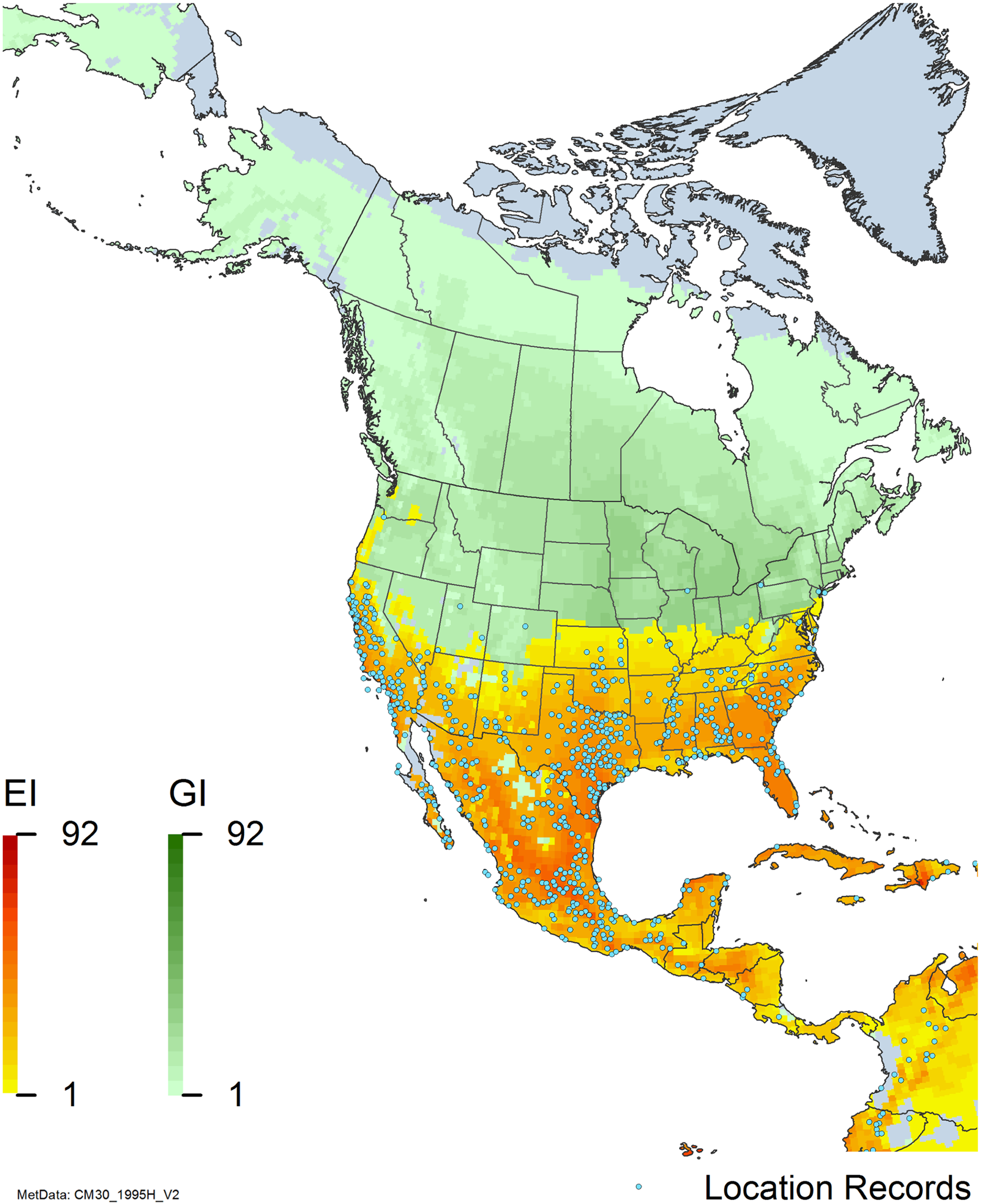
Figure 7. Nonnative, naturalized distribution of Arundo donax in North America, including Central America and the western Caribbean. Location records from GBIF (Reference Stover, Keller, Dudley and Langendoen2021). The map also shows climate suitability as modeled in CLIMEX. The model incorporates both natural rainfall and a top-up irrigation scenario. Yellow and red colors correspond to an increasing environmental index [EI] ≥1, indicating areas of expected population persistence. Outside of those areas, the green color indicates a growth index (G1) ≥ 1, indicating areas where A. donax can grow only in one favorable season, and thus populations are not expected to persist. See Structured Appendix for model details.
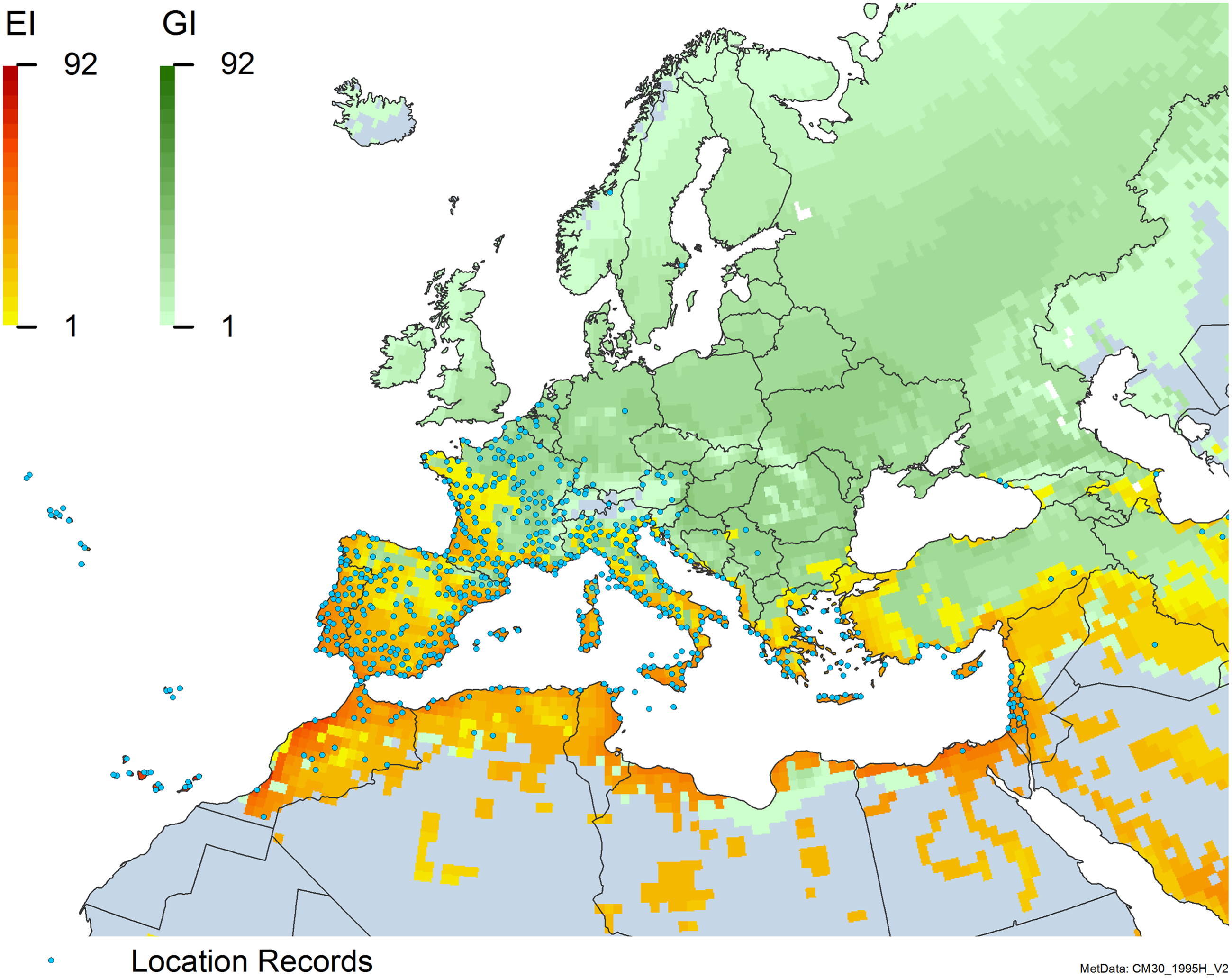
Figure 8. Distribution of Arundo donax in its anciently introduced range in Europe and the Mediterranean Basin and areas of its native distribution in far western Asia. Location records from GBIF (Reference Stover, Keller, Dudley and Langendoen2021). The map also shows climate suitability as modeled in CLIMEX. The model incorporates both natural rainfall and a top-up irrigation scenario. Yellow and red colors correspond to an increasing environmental index [EI] ≥1, indicating areas of expected population persistence. Outside of those areas, the green color indicates a growth index (G1) ≥ 1, indicating areas where A. donax can grow only in one favorable season, and thus populations are not expected to persist.
United States
Arundo donax is found in Hawaii and 29 states in the continental United States (EDDMapS 2022; USDA Reference Racelis, Goolsby, Penk, Jones and Roland2021) (Figures 3, 7, and 9), mainly in a line southward from about 41.5°N latitude from northern California eastward to the New York City area, but with invasive populations found mainly in the arid southwestern United States, from the southern tip of Texas to northern California. Populations in the southeastern United States have separate genetic origins in the Canary Islands and Seville, Spain, compared with the eastern and southern coastal Spanish origins of most of the A. donax populations in the southwestern United States (Tarin et al. Reference Tarin, Manhart, Pepper, Goolsby, Moran, Contreras Arquieta and Kirk2013). The infestation was estimated to be 6,360 ha in the lower Rio Grande basin of Texas (Yang et al. Reference Yang, Goolsby and Everitt2009, Reference Yang, Everitt and Goolsby2011) and 4,095 ha in California (Cal-IPC 2020).
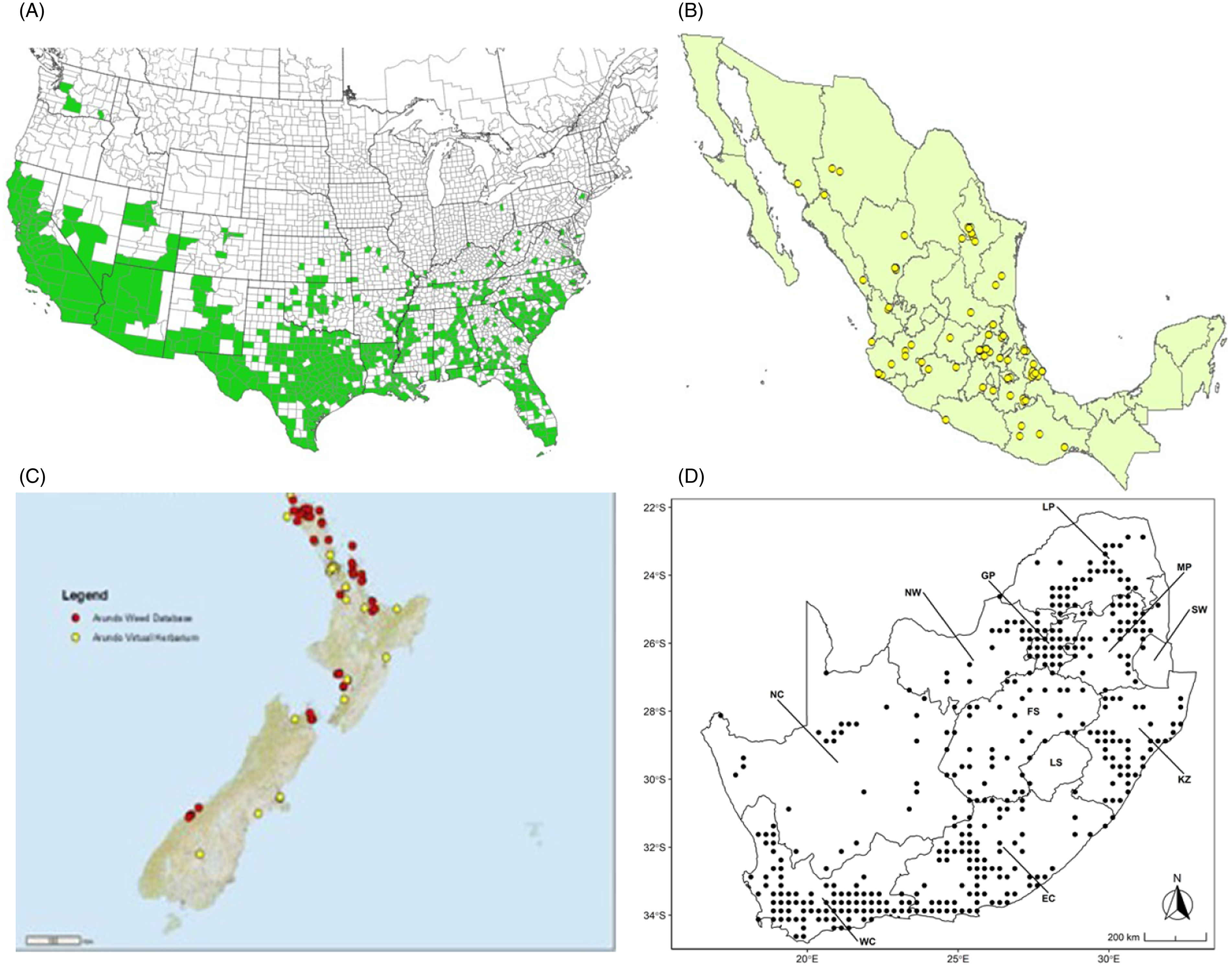
Figure 9. Distribution of Arundo donax based on national databases. (A) Occurrence in U.S. counties and states (from EDDMapS 2022). (B) Point distribution in Mexico (data from Contreras Reference Contreras2007). (C) Distribution in New Zealand, obtained from the New Zealand Flora (yellow) (E-Flora 2022) and the New Zealand Virtual Herbarium (red) (NZVH 2022). (D) Distribution in South Africa (taken from Sutton et al. Reference Sutton, Bownes, Visser, Mapaura and Canavan2021).
Mexico
Arundo donax is found in 27 states (IMTA Reference Pillay2008; Martínez Jiménez et al. Reference Martínez Jiménez, Goolsby, Racelis, Perez de Leon and Negrete Arroyos2017) (Figures 3, 7, and 9) from the Baja Peninsula and Chihuahuan and Sonoran deserts in the northwest to the tropical mountain drainages, forests, and grasslands of the Yucatan Peninsula in the southeast.
Bermuda to the Caribbean
Populations of Arundo donax occur in the Bahamas, Bermuda, Cuba (Figure 7), Curacao, Dominica, Dominican Republic, Granada, Haiti, Jamaica, Trinidad and Tobago, St. Kitts and Nevis, and St. Vincent and the Grenadines.
Central America
Arundo donax occurs in Costa Rica, El Salvador, Guatemala, Honduras, and Nicaragua (Figure 7).
South America
Arundo donax is found in Argentina, Brazil, Bolivia, Chile, Colombia, Ecuador, Peru, Suriname, and Uruguay.
Europe
Populations of A. donax occur in Albania, Andorra, Austria, Belgium, Bosnia and Herzegovina, Bulgaria, Croatia, Cyprus, France, Germany, Greece, Italy, Luxembourg, Monaco, Montenegro, Netherlands, Norway, Portugal, Romania, Serbia, Slovenia, Spain, Sweden, Switzerland, and Turkey (Jiménez-Ruiz et al. Reference Jiménez-Ruiz, Hardion, Del Monte, Vila and Santín-Montanyá2021) (Figures 3 and 8).
Africa
Arundo donax occurs in Algeria, Botswana, Egypt, Ethiopia, Kenya, Lesotho, Madagascar, Morocco, South Africa, Swaziland, Tanzania, Tunisia, and Zimbabwe (Figures 3 and A5). In South Africa, this reed thrives in Mediterranean-type climatic regions, where it has rapidly expanded into riparian habitats, with the most severe infestations occurring in the Western Cape, Gauteng, and KwaZulu-Natal provinces (Sutton et al. Reference Sutton, Bownes, Visser, Mapaura and Canavan2021) (Figure 9). It is estimated that A. donax occupies about 3,200 ha in South Africa (Henderson Reference Henderson2001; Kotzé et al. Reference Kotzé, Beukes, Van den Berg and Newby2010; Le Maitre et al. Reference Le Maitre, Forsyth, Dzikiti and Gush2016). The genetic origin of the South African populations appears to be the “M1” haplotype that is found in the native region and in the Mediterranean Basin, but also in Peru, New Caledonia, and the United States (Canavan et al. Reference Canavan, Paterson and Hill2017). However, more recent genetic analyses using chloroplast DNA spacers and microsatellites indicate a Middle Eastern origin, with plants brought first to Western Cape Province followed by introduction throughout the country (Bhikraj Reference Bhikraj2021).
Eastern Mediterranean, Asia Minor, and Persian Gulf
Populations of A. donax occur in Afghanistan, Israel, Jordan, Kazakhstan, Lebanon, Syria, Iran, Iraq, Pakistan, Tajikistan, Turkmenistan, and Uzbekistan (Figure 3). Several haplotypes are found in this region and are dominant in specific areas within it, suggesting the region comprises the plant’s native range (Canavan et al. Reference Canavan, Paterson and Hill2017; Hardion et al. Reference Hardion, Verlaque, Haan-Archipoff, Cahen, Hoff and Vila2017).
Asia and Melanesia
Arundo donax is found in Bangladesh, Bhutan, China, India, Indonesia, Japan, Myanmar, Nepal, Sri Lanka, South Korea, Taiwan, and Vietnam (Figures 3 and A6).
Oceania
Arundo donax was introduced into Australia in the mid-1800s. It has become widespread in Australia, with scattered records from all mainland states and the Northern Territory (Virtue et al. (Reference Virtue, Reynolds, Malone, Preston and Williams2010) (Figure 3). Genetic analyses indicate multiple genetically distinct origins for Australian populations, in contrast to the United States and South Africa, including possibly one haplotype imported to Australia from South Africa (Bhikraj Reference Bhikraj2021; Haddadchi et al. Reference Haddadchi, Gross and Fatemi2013). Newspaper articles indicate that A. donax was present in New Zealand by the late 1800s (e.g., Anonymous 1894) and naturalized by 1936 (Edgar et al. Reference Edgar, Connor and Shand1991). Arundo donax occurs in both the North and South Islands (NZVH 2022) (Figure 9) with low diversity reflective of a likely Australian origin (Bhikraj Reference Bhikraj2021). Populations are also known from Fiji, Marshall Islands, Papua New Guinea, Samoa, Seychelles Islands, Tonga, and Vanuatu.
Habitat
Arundo donax grows on a variety of soil types from loose sands and gravelly soils to heavy clays and river sediments (Goolsby et al. Reference Goolsby, Racelis, Goolsby, Kirk, Cristofaro, Grusak and Perez de Leon2013; Jiménez-Ruiz et al. Reference Jiménez-Ruiz, Hardion, Del Monte, Vila and Santín-Montanyá2021). Optimum growth occurs in well-drained soil with ample moisture supplied as fresh water or semi-saline waters at margins of brackish estuaries (Perdue Reference Perdue1958). Arundo donax invades riparian and wetland habitats, due to its water consumption requirements (Cal-IPC 2020; Jiménez-Ruiz et al. Reference Jiménez-Ruiz, Hardion, Del Monte, Vila and Santín-Montanyá2021; Quinn and Holt Reference Quinn and Holt2008; Watts and Moore Reference Watts and Moore2011). Occurrences in other areas, such as in planted fencerows, roadsides, canal banks, and escapes from these, are facilitated by anthropogenic factors, including planting and the presence of irrigation systems. As in the United States (Bell Reference Bell, Brock, Wade, Pysek and Green1997), the spread of A. donax in South Africa in riparian areas is largely dependent on past anthropogenic activities that transported the reed to new areas, including plantings for soil stabilization, and the building of dams, which altered river flows and created favorable habitat downstream. Using climate envelope models, a study by Rouget et al. (Reference Rouget, Richardson, Nel, Le Maitre, Egoh and Mgidi2004) estimated that 79% of the area of South Africa and neighboring countries Lesotho and Eswatini (formerly Swaziland) is potentially suitable for A. donax invasion. Disturbed areas adjacent to sensitive, biodiverse natural habitats such as the fynbos biome are the most threatened, but A. donax also thrives in disturbed ecosystems, such as in mining areas (van der Merwe et al. Reference van der Merwe, Schoonbee and Pretorius1990). Arundo donax occurs across at least 3,200 ha and 1,428 localities in South Africa (Guthrie Reference Guthrie2007; Sutton et al. Reference Sutton, Bownes, Visser, Mapaura and Canavan2021).
Comparison of the global distribution of A. donax (Figure 3) to a 0.1667° global Köppen-Geiger climate zone map (Beck et al. Reference Beck, Zimmermann, McVicar, Vergopolan, Berg and Wood2018) shows it commonly occurring in 16 global climate subtypes (Table 1). In the eastern portion of the native distribution of A. donax, from the eastern Mediterranean to India, it is found mainly in arid climate subtypes BSk, BSh, BWh, and BWk (steppe/desert; dry summer or winter, hot or cold overall), with the exception of India, where populations are found in areas with tropical climate subtype Aw (savanna) and, less commonly, in equatorial tropical forests and croplands (Af, Am). In the western and central Mediterranean (native or anciently introduced range), A. donax occurs in the areas characterized by the BSk climate, Csa and Csb climates (Mediterranean), Cfa climate (humid subtropical), and Cfb climate (oceanic) characteristic of immediate coastal areas and islands in subtropical and subtemperate regions. Isolated populations in the Italian Alps, Switzerland, northern France, and Germany occur in Dsb and Dfc climates, and there are highly isolated occurrences in Sweden and Norway under the Dwb climate. Additional European populations may be found in Dfa or Dfb climates. In the United States, arid climate subtypes BWh and BSh predominate in the heavily invaded southwestern part of the country, but there are also many A. donax occurrences in areas of California where the Csa climate is dominant (Csb in coastal areas), and in the Pacific Northwest in temperate climate subtype Csc. In the southeastern United States, A. donax occurs in areas dominated by the Cfa climate subtype, with a few occurrences farther north in the Dfa subtype. In Mexico and Central America, A. donax is found in desert (BWh) and steppe (BSh) areas, with additional occurrences in tropical savanna in the southeast of Mexico (Aw) and both savanna (Aw) and tropical rainforest (Af) regions in Central America. In South America, A. donax is found in regions with climate subtype Cfa in Argentina and southern Brazil, BWh (desert) in Chile and Peru, Aw in Bolivia, and Cfb in the Andes Mountains in Colombia. In Africa south of the Mediterranean Basin, known occurrences of A. donax are confined almost entirely to South Africa; however, it is found in diverse climates there, including desert (BWh) and steppe (BSh) in the northwest and west and also temperate areas in the southern and eastern part of the country (climate subtypes Csa, Cwa, Cwb, and Cfa). In Australia, A. donax is similarly found across highly diverse climates, ranging from BWh in the desert interior, Csa in the west, Cfa and Cfb in the southeast, and Aw in the tropical north. In New Zealand A. donax is found in climate subtypes Cfa and Cfb. In eastern Asia (mainly China, Taiwan, and Japan), A. donax is found in climate types Cfb and Cfa.
Table 1. Global Köppen-Geiger climate zone classifications that are suitable for survival and growth of Arundo donax.

Globally, it can be seen that A. donax is most common in areas with one of three climates: subtemperate coastal continental areas without precipitation seasonality and hot summers (Cfa; including parts of Europe and all invaded continents); on islands and some immediate mainland coastal areas with an oceanic climate (Cfb); and in Mediterranean climates with strongly seasonal rainfall patterns (dry in summer) that are hot (Csa) or warm (Csb) in summer (see also the Structured Appendix at the end of this article: Figure A1, global distribution overlaid on Köppen-Geiger zones, and Figure A4, occurrence distribution). Arundo donax is found in all areas of the world with the uncommon Mediterranean climate Csa and to a lesser degree in areas with its cooler variant, Csb, including: the western Mediterranean Basin; the southwestern coast of the United States; the northern and central coast of Chile in South America; parts of southern coastal South Africa; and the southwestern coast of Australia. The arid, cool/cold steppe climate BSk is the next most common climate type where A. donax is found. Less prevalent but still often found are occurrences in the hot steppe climate type BSh (eastern native range and all invaded areas except Asia), the hot desert climate type BWh (natural and artificial riparian areas worldwide), and the tropical savanna climate type Aw. In subtropical climates Cwa (humid, lowland) and Cwb (highland), dry winters may limit occurrences. Arundo donax appears able to survive only in favorable microclimates and/or with direct human intervention in temperate climates such as Dfa, Dfb, and Dsb; it is known to occur in two isolated locations in boreal/subarctic subtype Dfc in Norway and Sweden (Fig. 1). It has been reported from as high as 4,000-m altitude in Central America (Soreng et al. Reference Soreng, Davidse, Peterson, Zuloaga, Judziewicz, Filgueiras and Morrone2014).
A global CLIMEX model (Kriticos et al. Reference Kriticos, Webber, Leriche, Ota, Macadam, Bathols and Scott2012, Reference Kriticos, Maywald, Yonow, Zurcher, Herrmann and Sutherst2015; Sutherst and Maywald Reference Sutherst and Maywald1985) with additional input from global irrigation data (Siebert et al. Reference Siebert, Henrich, Frenken and Burke2013) (Figure 3), shown also for North America, including Central America and the Caribbean (Figure 7), and for Europe and the Mediterranean Basin (Figure 8) (see also the Structured Appendix), suggests accurate model prediction of the actual distribution of A. donax in subtemperate, subtropical, and tropical regions of the world. Recorded observations are remarkably sparse in areas modeled as being climatically suitable in the equatorial tropics of South America, Africa, and Indonesia and other island nations in the Melanesian region, as well as in subtropical areas south of the equator in South America and Africa, and north of the equator in Asia. It is possible that A. donax is underreported in these areas, or that these areas are less disturbed but still prone to invasion if disturbance occurs.
A visual, qualitative examination of a UN land-use assessment map (FAO Reference Paynter, Poeschko, Mitchell, Probst, Barreto, Colmán, Macedo, Dodd, Johnson, McCormack, Paterson, Sutton, Winks and Hinz2013) in relation to the global distribution (Figure 1) suggests that A. donax is commonly found in both its native and invasive ranges in areas with large-scale irrigated agricultural crop production and livestock use, as well as in rainfed agricultural areas. Riparian environments within sparse grasslands with low-density livestock production in areas such as the India-Pakistan region, China, Australia, Mediterranean Europe, the southwestern United States, Mexico, and South America also overlap with known occurrences. Arundo donax is widely found outside areas where natural soil conditions are suitable for its growth, illustrating the importance of anthropocentric manipulation of water resources in aiding its spread (Nilsson and Berggren Reference Nilsson and Berggren2000).
Invasion History
Arundo donax appears to have spread from its putative ancestral native range in the Indus River basin and central Asia westward to Mediterranean Europe and eastward into other parts of Asia thousands of years ago (Jiménez-Ruiz et al. Reference Jiménez-Ruiz, Hardion, Del Monte, Vila and Santín-Montanyá2021), but a global genetic-based historical analysis is not available. In surveys for biological control agents, diversity of insects on A. donax was highest in the western Mediterranean (Kirk et al. Reference Kirk, Widmer, Campobasso, Carruthers, Dudley and Pirosko2003; Tracy and DeLoach Reference Tracy, DeLoach and Bell1999), even though plant genotypic diversity there is lower there than in the areas between the Middle East and the Indus River basin (Canavan et al. Reference Canavan, Paterson and Hill2017; Sutton et al. Reference Sutton, Bownes, Visser, Mapaura and Canavan2021; Tarin et al. Reference Tarin, Manhart, Pepper, Goolsby, Moran, Contreras Arquieta and Kirk2013), suggesting that the plant acquired novel natural enemies during its spread. It was introduced by European colonizers to North America in the 1500s (Dunmire Reference Dunmire2004), to South Africa in the late 1700s (Guthrie Reference Guthrie2007), to Australia in the mid-1800s (Virtue et al. Reference Virtue, Reynolds, Malone, Preston and Williams2010), and to New Zealand by the late 1800s (Anonymous 1894). Additional introductions into North America occurred in the early 20th century (Perdue Reference Perdue1958). A genetic study of its invasive distribution on islands in the Pacific Basin would possibly reveal an interesting mosaic of genotypes linked to those present in colonizing nations. Efforts to eradicate A. donax from entire rivers or watersheds have generally not been successful because of insufficient support for follow-up control of regrowth (Jiménez-Ruiz et al. Reference Jiménez-Ruiz, Hardion, Del Monte, Vila and Santín-Montanyá2021; van Wilgen et al. Reference van Wilgen, Forsyth, Le Maitre, Wannenburgh, Kotzé, van den Berg and Henderson2012; Visser et al. Reference Visser, Wilson, Canavan, Canavan, Fish, Maitre, Nänni, Mashau, O’Connor, Ivey, Kumschick and Richardson2017), restrictions on access to land or ornamental populations (Cal-IPC 2020), and poor detection of small populations under dense riparian canopies. Projects in two coastal river valleys in southern and central California involving 20 yr of control did, however, reduce populations to near-zero coverage (Cal-IPC 2020; A Lambert, University of California–Santa Barbara, personal communication).
Life-Form and Life History
Arundo donax grows as a bamboo-like perennial to 9-m tall, with thick, well-developed rhizomes. Shoots can emerge from rhizome buds year-round in most locations. This giant grass is well-adapted to periodic flooding (Spencer et al. Reference Spencer, Colby and Norris2013) and is not only adapted to but benefits from fire (Coffman et al. Reference Coffman, Ambrose and Rundel2010). In tropical and subtropical climates, A. donax grows year-round, and the stems produce side shoots in year 2. In temperate climates such as California, first-year main stems senesce in the late fall, transferring nutrients to rhizomes. Second-year main shoots produce lateral shoots in the spring, which often produce third- and fourth-order side shoots during the typical 3- to 4-yr total main shoot life span (Decruyenaere and Holt Reference Decruyenaere and Holt2005; Thornby et al. Reference Thornby, Spencer, Hanan and Sher2007). Dead shoots remain standing, sometimes inhibiting new shoot formation until dead shoots fall over and younger rhizomes reoccupy the soil. Under the system described by Grimes (Reference Grimes, Gottlieb and Jain1988), A. donax uses vegetative expansion to reproduce and the competitor strategy to persist in and dominate riparian ecosystems, as a result of dynamic “foraging” of roots for resources, reducing the predictability of resources for other plants. However, unlike many plants exhibiting the competitor strategy, A. donax can “switch down” its resource use (Grubb Reference Grubb1998) to tolerate severe water-deficit stress under drought conditions (Moore et al. Reference Moore, Li, Kui and West2016) and can thrive in both low-disturbance environments and those subjected to major disturbance from fire (Coffman et al. Reference Coffman, Ambrose and Rundel2010) or flooding (Frandsen and Jackson Reference Frandsen, Jackson, Jackson, Frandsen and Duthoit1994).
Dispersal and Establishment
Rhizomes (Bell Reference Bell, Brock, Wade, Pysek and Green1997) and shoots (Boland Reference Boland2006; Wijte et al. Reference Wijte, Mizutani, Motamed, Merryfield, Miller and Alexander2005) are vegetative propagules of A. donax that disperse via flooding (Stover et al. Reference Stover, Keller, Dudley and Langendoen2018) or human movement. Rhizomes can resprout even under drought conditions (Mann et al. Reference Mann, Kyser, Barney and DiTomaso2013), while detached shoots must be in contact with moist soil to produce new axillary buds and shoots (Boland Reference Boland2006; Wijte et al. Reference Wijte, Mizutani, Motamed, Merryfield, Miller and Alexander2005); they otherwise die, forming large dead piles of debris or “wrack” that alters river flow and threatens flood control (Cal-IPC 2020; Lambert et al. Reference Lambert, D’Antonio and Dudley2010a, 2010b). Establishment is favored by moist but not waterlogged soil (Goolsby et al. Reference Goolsby, Racelis, Goolsby, Kirk, Cristofaro, Grusak and Perez de Leon2013) in open habitat with little vegetation (Quinn and Holt Reference Quinn and Holt2008), as might be found after flooding, fire, or human disturbance. In the Mediterranean, the most robust populations are found on well-drained gravelly or sandy soils (Goolsby et al. Reference Goolsby, Racelis, Goolsby, Kirk, Cristofaro, Grusak and Perez de Leon2013; Jiménez-Ruiz et al. Reference Jiménez-Ruiz, Hardion, Del Monte, Vila and Santín-Montanyá2021).
Invasion Risk
As shown in Figures 3, 7, and 8, A. donax is widely established in watersheds throughout the world in subtemperate and subtropical continental regions and islands, particularly in warm, seasonally or perennially arid regions that are favorable for its high evapotranspiration capacity (Cal-IPC 2020). New invasions in these areas are the result of human transport between watersheds and water-borne transport of shoots and rhizomes within watersheds (Wijte et al. Reference Wijte, Mizutani, Motamed, Merryfield, Miller and Alexander2005). Gaps in the known occurrences of this grass are still apparent in climatically suitable areas (Figures 3, 7, and 8), including subtropical Brazilian grasslands, sub-Saharan African grasslands, and global equatorial tropics. In tropical forested areas, dense native or other vegetation may prevent invasion, but human clearing of forests and manipulation of water flow are likely to increase invasion risk (Bell Reference Bell, Brock, Wade, Pysek and Green1997; Guthrie Reference Guthrie2007; Jiménez-Ruiz et al. Reference Jiménez-Ruiz, Hardion, Del Monte, Vila and Santín-Montanyá2021). This is especially critical from a water conservation aspect, as increased density and distribution of A. donax in a watershed negatively influences availability of water resources.
Invasion Pathways
Arundo donax invasion is linked to human movement of plant propagules. During the first half of the 20th century, intentional introductions led to new invasions, including reintroductions in previously invaded areas (Bell Reference Bell, Brock, Wade, Pysek and Green1997; Guthrie Reference Guthrie2007; Haddadchi et al. Reference Haddadchi, Gross and Fatemi2013; Perdue Reference Perdue1958). Accidental invasions can occur through movement on earthmoving equipment or mowers (Jiménez-Ruiz et al. Reference Jiménez-Ruiz, Hardion, Del Monte, Vila and Santín-Montanyá2021) and even sale of variegated varieties in some areas (Haddadchi et al. Reference Haddadchi, Gross and Fatemi2013). Dredging of ditches and canals often disturbs stands of A. donax and allows movement of propagules to new uninfested areas. Similarly, clearing of A. donax for weed control can dislodge rhizome and shoot fragments that move downstream. The main nonhuman source of invasion is flooding, which can undercut riverbanks, causing downstream movement of rhizome mats and shoot pieces (Boland Reference Boland2006; Cal-IPC 2020; Jiménez Ruiz et al. Reference Jiménez-Ruiz, Hardion, Del Monte, Vila and Santín-Montanyá2021; Stover et al. Reference Stover, Keller, Dudley and Langendoen2018). Haddadchi et al. (Reference Haddadchi, Gross and Fatemi2013) used molecular techniques to investigate the spread of A. donax in southeastern Australia and found evidence of widespread transport (up to 200 km away from the source plant) indicating that rivers serve as an effective dispersal corridor.
Purposeful introductions of A. donax for biofuel cropping or fiber use should be carefully designed to prevent escape of propagules outside cultivation (Raghu et al. Reference Raghu, Anderson, Daehler, Davis, Wiedenmann and Simberloff2006). Alternative biofuel plants, such as native switchgrass (Panicum virgatum L.) in North America, should be considered (Lewandowski et al. Reference Lewandowski, Scurlock, Lindvall and Christou2003). Weed risk assessments (Virtue et al. Reference Virtue, Reynolds, Malone, Preston and Williams2010) and economic suitability studies in South Africa were conducted to determine whether A. donax was suitable to serve as biomass for electricity generation (Melane et al. Reference Melane, Ham and Meincken2017). It was concluded that the use of A. donax was not cost-effective using current thermo-chemical conversion processes.
Growth and Development
Morphology
Under suitable growth conditions, A. donax produces robust, thick (to 6-cm diameter; PJM, personal observation) main shoots or canes, and then one and eventually multiple side shoots at leaf nodes when the canes are 1-yr-old or less (Decruyenaere and Holt Reference Decruyenaere and Holt2005; Thornby et al. Reference Thornby, Spencer, Hanan and Sher2007). Side shoots produce additional biomass and photosynthate, increasing the vigor of the stand. The “Description” section provides more information on structure of individual shoots.
Stress Tolerance
Arundo donax perennates through rhizome branching and new primary shoot growth and by production of lateral shoots on 1-yr-old and older main shoots. In California, USA, a typical shoot senesces each winter but lives 3 to 4 yr (Thornby et al. Reference Thornby, Spencer, Hanan and Sher2007), with photosynthetic capacity lost in dead main shoot leaves after the first year rapidly replaced by leaves on abundant lateral shoots. When primary shoots die, perennation through rhizome growth occurs via production of new shoot buds and shoots, causing expansion of A. donax patches.
Arundo donax is highly tolerant of various forms of abiotic stress (Perdue Reference Perdue1958), including water deficit (Moore et al. Reference Moore, Li, Kui and West2016; Nackley et al. Reference Nackley, Vogt and Kim2014; Zegada-Lizarazu and Monti Reference Zegada-Lizarazu and Monti2019), due to its use of water-efficient C3 photosynthesis, comparable to C4 bioenergy crops (Webster et al. Reference Webster, Driever, Kromdijk, McGrath, Leakey, Siebke, Demetriades-Shah, Bonnage, Peloe, Lawson and Long2016); elevated soil or water salinity (Quinn and Holt Reference Quinn and Holt2008; Sánchez et al. Reference Sánchez, Scordia, Lino, Arias, Cosentino and Nogués2015); and high heavy metal content in soils (Cristaldi et al. Reference Cristaldi, Conti, Cosentino, Mauromicale, Copat, Grasso, Zuccarello, Fiore, Restuccia and Ferrante2020). Arundo donax can survive prolonged water-deficit stress (Zegada-Lizarazu and Monti Reference Zegada-Lizarazu and Monti2019), although shoots are shorter and produce less biomass in greenhouse tests in which water-deficit stress is imposed (Lambert et al. Reference Lambert, Dudley and Robbins2014; Moran Reference Moran2015). While roots have been observed as deep as 5 m in soil cores, reaching groundwater levels (Moore et al. Reference Moore, Li, Kui and West2016), A. donax mainly takes up surface and soil water and does not rely on groundwater (Moore et al. Reference Moore, Li, Kui and West2016; Watts and Moore Reference Watts and Moore2011; L. Kui, personal communication). Water can be carried at least 3 m through interconnected rhizome networks located just below the soil surface (Kui et al. Reference Kui, Li, Moore and West2013). The broad geographic distribution of A. donax, from cold temperate areas of the United States, Europe, and Asia to the equatorial tropics (Jiménez-Ruiz et al. Reference Jiménez-Ruiz, Hardion, Del Monte, Vila and Santín-Montanyá2021) (Figures 3, 7, and 8), demonstrates the ability of this grass to grow and disperse under a wide range of temperature and precipitation regimes. It is most widely distributed in arid temperate to subtropical regions. It is unable to persist outside human influence in the presence of prolonged winter and repeated episodic spring freezes (Perdue Reference Perdue1958). Prolonged flooding and drought limit the ability of first-year A. donax shoots to survive and grow (Mann et al. Reference Mann, Kyser, Barney and DiTomaso2013), but growth from surviving rhizomes will occur if more favorable conditions follow. Arundo donax has a remarkable tolerance to fire, in part because of its ability to utilize abundant soil nutrients and grow rapidly (Decruyenaere and Holt Reference Decruyenaere and Holt2005; Quinn et al. Reference Quinn, Rauterkus and Holt2007). The process by which A. donax comes to dominate riparian ecosystems is linked to fire, as it regrows more rapidly than do surviving native plants (Coffman et al. Reference Coffman, Ambrose and Rundel2010).
Ecophysiology
The substantial water use of A. donax, covered in the “Importance” section, is a key attribute that makes this weed so damaging. Water use is linked to photosynthetic evapotranspiration. Arundo donax uses C3 photosynthesis, but exhibits attributes of C4 photosynthesis, allowing it to take advantage of subsurface water availability under otherwise dry conditions and fix carbon at a high rate (Rossa et al. Reference Rossa, Tuffera, Naidoo and von Willert1998), while closing stomata under drought conditions (Webster et al. Reference Webster, Driever, Kromdijk, McGrath, Leakey, Siebke, Demetriades-Shah, Bonnage, Peloe, Lawson and Long2016).
Phenology
Main shoots (culms) can sprout from rhizomes clonally at any time of year when average daily temperatures are above 7 C and below 30 C (Spencer and Ksander Reference Spencer and Ksander2006). In California, emergence of main shoots from rhizome buds is especially prevalent in early spring (Decruyenaere and Holt Reference Decruyenaere and Holt2001) after the winter rainy season in this Mediterranean climate (Beck et al. Reference Beck, Zimmermann, McVicar, Vergopolan, Berg and Wood2018), but three cohorts of emergence, the first two requiring accumulation of about 500 degree days, and the third requiring about 1,000 degree days (Spencer and Ksander Reference Spencer and Ksander2006), occurred in 1 yr under controlled conditions. Main shoots flower in the first year and produce profuse lateral shoots beginning late in the first year, continuing for the typical 3- to 4-yr life span of each main shoot (Decruyenaere and Holt Reference Decruyenaere and Holt2005; Thornby et al. Reference Thornby, Spencer, Hanan and Sher2007). Mowing or “topping” of main shoots, or natural shoot breakage, leads to production of profuse, bushy nodes of side shoots (Racelis et al. Reference Racelis, Moran and Goolsby2012b; PJM, personal observation).
Mycorrhizal and Bacterial Symbionts
No mycorrhizal or bacterial symbionts are known.
Reproduction
Floral Biology
Sexual reproduction occurs only in the native range. Fertile seeds have been reported in Iran, Afghanistan, Pakistan, India, Nepal, and Bhutan (Hardion et al. Reference Hardion, Verlaque, Saltonstall, Leriche and Vila2014). The terminal inflorescences, which appear in temperate climates most commonly in late summer and fall (Jiménez- Ruiz et al. Reference Jiménez-Ruiz, Hardion, Del Monte, Vila and Santín-Montanyá2021; Spencer and Ksander Reference Spencer and Ksander2006) are single, up to 60-cm long, and feathery with silky hairs. The spikelets are 8- to 16-cm long and lanceolate, and each spikelet contains two to seven florets. The florets are all bisexual, except the reduced uppermost one (Lewandowski et al. Reference Lewandowski, Scurlock, Lindvall and Christou2003).
Seed Production and Dispersal
Seeds of A. donax in North and South America, Europe, Africa, Australia and New Zealand, and Pacific Rim islands are sterile (Jiménez-Ruiz et al. Reference Jiménez-Ruiz, Hardion, Del Monte, Vila and Santín-Montanyá2021), most likely due to failure of the megaspore mother cell to divide (Bhanwra et al. Reference Bhanwra, Choda and Kumar1982), leading to defective pollen and ovary development (Hardion et al. Reference Hardion, Verlaque, Rosato, Rossello and Vila2015; Mariani et al. Reference Mariani, Cabrini, Danin, Piffanelli, Fricano, Gomarasca, Dicandilo, Grassi and Soave2010). In invasive clones, seed-like unfilled caryopses (Balogh et al. Reference Balogh, Herr, Czakó and Márton2012) will fall from spikes when disturbed. These sterile seeds are dispersed by wind and water and on animals.
Seedbanks, Seed Viability, and Germination
None of these factors are relevant in the invasive range, as seeds are sterile.
Vegetative Reproduction
Reproduction is entirely vegetative/clonal in all of the areas A. donax has invaded, including North America (Boose and Holt Reference Boose and Holt1999; Lewandowski et al. Reference Lewandowski, Scurlock, Lindvall and Christou2003), and also in the native range in Mediterranean Europe, while both asexual and sexual reproduction occur in Asia (Jiménez-Ruiz et al. Reference Jiménez-Ruiz, Hardion, Del Monte, Vila and Santín-Montanyá2021). Invasive clonal A. donax has a chromosome count of 2n = ca. 108–110, while some, presumably native Asian accessions, have a count of 2n = 72 (Christopher and Abraham Reference Christopher and Abraham1971; Kalia Reference Kalia1978). The invasiveness of A. donax may thus be caused in part by the formation of pseudo-triploid genomes (Jiménez-Ruiz et al. Reference Jiménez-Ruiz, Hardion, Del Monte, Vila and Santín-Montanyá2021). Arundo donax reproduces prolifically via rhizome budding and branching (Cal-IPC 2020) in undisturbed stands and through layering or lodging of rhizomes, main shoots, or shoot pieces after floods or other disturbance (Boland Reference Boland2006; Boose and Holt Reference Boose and Holt1999; Wijte et al. Reference Wijte, Mizutani, Motamed, Merryfield, Miller and Alexander2005).
Population Dynamics
Arundo donax main shoots complete a 3- to 4-yr life cycle (DeCruyenaere and Holt Reference Decruyenaere and Holt2005; Spencer and Ksander Reference Spencer and Ksander2006; Thornby et al. Reference Thornby, Spencer, Hanan and Sher2007). Stands or patches expand through rhizome branching and shoot bud formation and recolonize areas of old, dead rhizomes as branching of live rhizomes continues, forming a tangled mass of live and dead rhizome material and live and dead standing shoots. This weed thus forms thick, persistent, perennial stands that dominate invaded riparian habitats (Quinn and Holt Reference Quinn and Holt2008).
Management Options
Phytosanitary Measures
To prevent the spread of A. donax between continents and countries, regulatory officials throughout the world should prohibit international and domestic live shipments of this species. Shipments for biomass/bioenergy development represent one potential source of risk. Even in countries where A. donax is already present, new genotypes could become established that are more invasive or present a genetic mismatch with the biological control agents sourced from Mediterranean Europe, should they be released. Earthmoving and mowing equipment should be carefully inspected to prevent movement of A. donax propagules. Shoot pieces and rhizomes may be accidentally transported with soil used for fill or construction (Cal-IPC 2020). Movement of plant or soil material from areas in or around patches of A. donax to uninvaded areas should be avoided and care taken when disposing of debris from control operations. As both rhizome and shoot pieces can readily produce new buds and plants given adequate moisture, debris should be burned, mulched, or dried at elevated temperature (e.g., under a tarp) for an extended period (Cal-IPC 2020, Reference Nilsson and Berggren2021). Mowing along the bank edges of infested waterways can lead to movement of propagules in flotsam, leading to downstream infestations. Treatment of A. donax with approved herbicides before mowing may reduce the risk of starting new infestations. Treatment of upstream areas of watersheds to avoid downstream invasion through flood-borne transport of rhizomes and shoots can be an effective strategy (Cal-IPC 2020).
A determination of the area of invasion in riparian systems is essential for the effective prevention of spread of A. donax, as this information permits informed prioritization of resources for eradication and management. As an example, remote sensing studies of A. donax in the lower Rio Grande basin of Texas and Mexico have demonstrated that aerial airborne multispectral and hyperspectral imagery and high-resolution satellite imagery can be used to detect and map infestations. Aerial color-infrared (CIR) photographs and video images were taken along the Rio Grande between Brownsville and El Paso, TX, in June and July 2002 (Everitt et al. Reference Everitt, Yang, Alaniz, Davis, Nibling and Deloach2004, Reference Everitt, Yang and Deloach2005) (Figure 10). Approximately 600 river-km of the Rio Grande area surveyed was infested with A. donax. The densest populations were located over a 300 river-km stretch between Laredo and Del Rio. Ground surveys confirmed the presence of A. donax at plotted locations. Digitization and classification of aerial images showed that A. donax was present in riparian patches at 10.6 ha river-km−1. Thus, the total infested area for the 600 km of the Rio Grande was estimated as 6,360 ha (Yang et al. Reference Yang, Goolsby and Everitt2009, Reference Yang, Everitt and Goolsby2011). Release sites for biological control agents (Goolsby et al. Reference Goolsby, Gaskin, Tarin, Pepper, Henne, Auclair, Racelis, Summy, Moran, Thomas, Yang, Martínez-Jiménez, Ciomperlik, Perez de Leon and Kirk2014) and later for implementation of an integrated control regime (see section Biological Control—United States and Mexico) were selected to cover this large area of critical importance for water resources. To quantify the distribution of infested areas in the Mexican portion of the Rio Grande Basin, QuickBird® image scenes with 2.4-m spatial resolution and four spectral bands were selected (Figure 11) and classified (Everitt et al. Reference Everitt, Yang and Deloach2005). The total estimated A. donax infestation area in the Mexican tributaries of the Rio Grande was estimated at 4,775 ha. These results helped inform control decisions using biocontrol agents and other methods in Mexico (Martínez Jiménez et al. Reference Martínez Jiménez, Goolsby, Racelis, Perez de Leon and Negrete Arroyos2017). The use of publicly available satellite images to estimate the area of invasion in the Central Valley of California is informing a prioritization system for ongoing local eradication and long-term management (Cal-IPC 2020).

Figure 10. Color-infrared composite for a portion of a QuickBird® image along the Rio Grande between the United States and Mexico. Color codes in the classification map are: red, Arundo donax; dark green, mixed vegetation; light gray, soil; blue, water.

Figure 11. Polygons showing archived (blue) and selected but not archived (green) QuickBird® image scenes in the Mexican portion of the Rio Grande basin.
Chemical
Glyphosate (2.5 to 5.0 kg ai ha−1), imazapyr (0.54 to 1.1 kg ha−1), or both in combination (with surfactant in all cases) are the chemical tools most commonly used to control A. donax (DiTomaso et al. Reference DiTomaso, Kyser, Oneto, Wilson, Orloff, Anderson, Wright, Roncoroni, Miller and Prather2013; Spencer et al. Reference Spencer, Wailun, Liow, Ksander, Whitehand, Weaver, Olsson and Newhouser2008, Reference Spencer, Tan, Liow, Ksander and Whitehand2009). Applications of glyphosate should be made between late summer and mid-autumn after peak shoot growth has occurred (Ana et al. Reference Ana, Teixeira and Frazão Moreira2015; Anonymous 2016; Bell Reference Bell, Brock, Wade, Pysek and Green1997; DiTomaso et al. Reference DiTomaso, Kyser, Oneto, Wilson, Orloff, Anderson, Wright, Roncoroni, Miller and Prather2013; Spencer et al. Reference Spencer, Ksander, Tan, Liow and Whitehand2011), as rhizomes are low in reserves at this time and are receiving translocates from the shoots (Decruyenaere and Holt Reference Decruyenaere and Holt2001) and shoots are beginning to show signs of senescence. Imazapyr can be effectively applied when shoots are 1.5- to 2.5-m tall and growing rapidly, in late spring or early summer (Anonymous 2004; A Lambert, personal communication). In one study (Bell Reference Bell2011), imazapyr applied alone or with glyphosate was most effective when applied in the fall, and imazapyr alone was more effective than glyphosate alone (Bell Reference Bell2011). Aerial foliar applications, for example, with a helicopter or a boom-mounted sprayer, are necessary to treat large areas and must typically be accompanied by avoidance or pruning of nontarget vegetation. Follow-up spot treatments for 2 to 3 yr or longer are needed. Undiluted herbicide can be used in cut stump operations for small patches. Chemical application has been widely used across several thousand hectares in California (Cal-IPC 2020), in central and southern Texas (TPWD Reference Racelis, Goolsby and Moran2022), and throughout A. donax’s invaded range in the United States. In South Africa, A. donax is the only invasive alien grass to receive its own budget for management (Visser et al. Reference Visser, Wilson, Canavan, Canavan, Fish, Maitre, Nänni, Mashau, O’Connor, Ivey, Kumschick and Richardson2017), but only 0.33% of the total budget for the Working for Water program for alien plant control was spent on A. donax between 1995 and 2008 (van Wilgen et al. Reference van Wilgen, Forsyth, Le Maitre, Wannenburgh, Kotzé, van den Berg and Henderson2012). It was found that herbicide initially reduces density and height and growth rates; however, in the long term, it increases A. donax density (Guthrie Reference Guthrie2007). Chemical control is therefore not generally supported in South Africa due to cost, the potential harm to non-target species, and the temporary nature of the benefits of this approach.
Physical/Mechanical
Successful large-scale (>10 ha) control projects targeting A. donax in California, USA, have, in most cases, involved a combination of mechanical/physical control and herbicides with repeated treatment (Cal-IPC 2020); mowing or tilling are rarely if ever effective on their own. This integrated physical–chemical approach is costly (e.g., estimated US$70 million to effectively treat all A. donax–occupied habitat in the Central Valley of California (Cal-IPC 2020). Mowing appears to be most effective in the late summer or early fall when shoots are flowering, as both rhizome reserves and soil moisture for regrowth are likely to be low (DiTomaso et al. Reference DiTomaso, Kyser, Oneto, Wilson, Orloff, Anderson, Wright, Roncoroni, Miller and Prather2013). In South Africa, present control methods are largely restricted to hand or mechanical cutting (Guthrie Reference Guthrie2007). However, little success has been achieved in most populations due to the scale of infestations. Combined mowing and herbicide application was largely ineffective at suppressing A. donax growth and biomass recovery in South Africa (Guthrie Reference Guthrie2007; Visser et al. Reference Visser, Wilson, Canavan, Canavan, Fish, Maitre, Nänni, Mashau, O’Connor, Ivey, Kumschick and Richardson2017). Topping at approximately 1 m has been implemented as a method to quickly improve visibility for law enforcement Additional control options are being investigated in South Africa that integrate mechanical and biological control, including mowing or topping of stems to encourage growth in Tetramesa romana populations (Sutton et al. Reference Sutton, Bownes, Visser, Mapaura and Canavan2021), as has been implemented in the lower Rio Grande basin of Texas (Figure 12) and on a smaller scale in California (see section Biological Control—United States and Mexico). Mowing in autumn can be integrated with spring application of imazapyr (Bell Reference Bell2011; A Lambert, personal communication) or spring stem cutting can be combined with herbicide application to stumps in the summer (Bell Reference Bell, Brock, Wade, Pysek and Green1997).
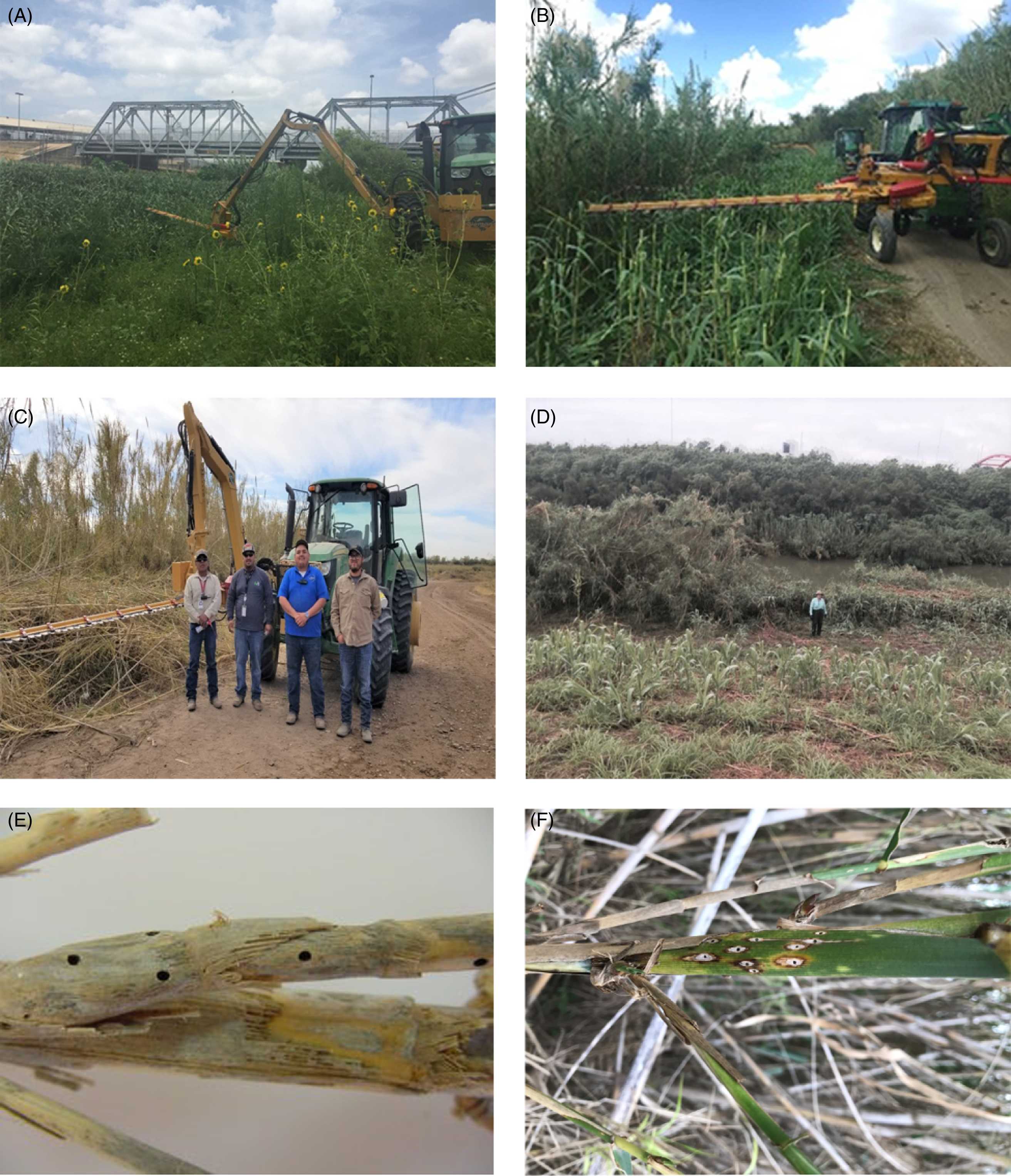
Figure 12. Methods for topping of Arundo donax to integrate with biological control methods. (A–C) Specialized tractor-mounted mow bars used for large-scale topping of A. donax along the Rio Grande. The tractor has a reciprocating blade that cuts canes at 1 m. (D) Topping opens view to Rio Grande across to Mexico from the U.S. side of the river. (E and F) Tetramesa romana, the arundo wasp, responds to an increase in side shoot abundance after cutting by galling young side shoots, as evidenced by abundant exit holes made by progeny wasps. All photos by JAG and staff, USDA-ARS.
Fire has been used widely as a physical control technique, but is ineffective on its own, as A. donax burns intensely and recovers quickly after fire (Coffman et al. Reference Coffman, Ambrose and Rundel2010), exacerbating its displacement of other plants killed by the fire; areas of frequent fire disturbance are particularly vulnerable to invasion (Quinn and Holt Reference Quinn and Holt2008). In areas along the Rio Grande on the U.S.–Mexico border, fire has been used to reduce A. donax stands (JAG and PJM, personal observation) to facilitate cattle grazing on young regrowth shoots (Perdue Reference Perdue1958; Racelis et al. Reference Racelis, Rubio, Vaughan and Goolsby2012a).
Biological
Insect Agents
Two specialist, insect biological control agents from the native range of A. donax in Spain, including the wasp Tetramesa romana Walker (Hymenoptera: Eurytomidae) (Figure 13) and the armored scale Rhizaspidiotus donacis (Leonardi) (Homoptera: Diaspidae) (Figure 14) were mass-reared and first released in the lower Rio Grande basin of Texas, USA, and Mexico between 2009 and 2012 (Moran et al. Reference Moran, DeClerck-Flaoate, Hill, Sathyamurthy, Hill, Paynter, Goolsby, Morales-Ramos, Rojas and Shapiro2022; Racelis et al. Reference Racelis, Goolsby, Penk, Jones and Roland2010; Villarreal et al. Reference Villarreal, Goolsby, Vacek, Perez de Leon and Racelis2016). The wasp T. romana lays eggs in canes and side shoots, causing formation of galls (abnormal plant growth) clustered at main and lateral shoot tips and resembling one large gall (Figure 13). No plants outside the genus Arundo spp. are galled (Goolsby and Moran Reference Goolsby and Moran2009; Sutton et al. Reference Sutton, Bownes, Visser, Mapaura and Canavan2021). One larva feeds and pupates inside each gall. Adults emerge from their galls by chewing exit holes (Figure 12E and 12F) (Goolsby and Moran Reference Goolsby and Moran2009), which can be easily used in the field to diagnose wasp presence and estimate density (Goolsby et al. Reference Goolsby, Gaskin, Tarin, Pepper, Henne, Auclair, Racelis, Summy, Moran, Thomas, Yang, Martínez-Jiménez, Ciomperlik, Perez de Leon and Kirk2014). The wasp can complete its life cycle in 35 to 60 d (Moran and Goolsby Reference Moran and Goolsby2009). Pre-release efficacy testing in a greenhouse showed that galling reduced shoot growth by 90% (Goolsby et al. Reference Goolsby, Spencer and Whitehand2009b) and reduced photosynthetic processes by up to 32% (Moore et al. Reference Moore, Watts and Goolsby2010).

Figure 13. (Left) The wasp Tetramesa romana ovipositing into stems of Arundo donax. Adult wasps are 0.5 to 1.2 cm in length, including antennae. (Right) Shoot tip distortion caused by galls made on A. donax by T. romana. Photos by JAG.

Figure 14. (Left) The armored scale Rhizaspidiotus donacis isolated from a rhizome, with waxy scale covering removed, showing fully expanded adult female (about 1.2-mm wide) with crawlers on left and newly molted adult female (about 0.8-mm wide) before crawler development on the right. (Right) Lateral shoot node on Arundo donax infested with R. donacis, showing females at the base of the node and witches’ broom distortion of shoots. Photos by PJM (left) and JAG (right).
The armored scale R. donacis feeds belowground on rhizomes and the bases of side shoots of A. donax (Goolsby et al. Reference Goolsby, Moran, Adamczyk, Kirk, Jones, Marcos and Cortés2009a; Moran and Goolsby Reference Moran and Goolsby2010). Females (Figure 14) release tiny (ca. 0.4 mm; Moran and Goolsby Reference Moran and Goolsby2010) “crawlers” that settle on suitable tissues, become immobile, and complete their life cycle in 5 to 6 mo, while males have a life span of only 2 mo, emerging as short-lived winged adults that mate with immobile females. Newly molted adult females increase about 50% in size to 1.2 mm as they develop live crawlers (Figure 14). Limited (ca. 50- to 100-fold less than on A. donax) development and reproduction occur on P. australis (Braman et al. Reference Braman, Lambert, Özsoy, Hollstein, Sheehy, McKinnon, Gaskin, Goolsby and Dudley2021; Goolsby et al. Reference Goolsby, Moran, Adamczyk, Kirk, Jones, Marcos and Cortés2009a), which has both native and invasive genotypes in North America (Lambert et al. Reference Lambert, Saltonstall, Long and Dudley2016; Saltonstall Reference Saltonstall2016). In pre-release evaluations in its native range in France and Spain, infestation by this scale reduced A. donax shoot growth and rhizome size by 50% (Cortes et al. Reference Cortes, Goolsby, Moran and Marcos-Garcia2011a, Reference Cortes, Kirk, Goolsby, Moran, Racelis and Marcos-Garcia2011b), and infestation reduced photosynthesis in a greenhouse test (Moore et al. Reference Moore, Watts and Goolsby2010). Abundant populations were associated with well-drained, gravel-based soils in the native range (Goolsby et al. Reference Goolsby, Racelis, Goolsby, Kirk, Cristofaro, Grusak and Perez de Leon2013), informing decisions on release sites and interpretation of establishment success. A characteristic “witches’ broom” distortion of lateral shoots has been observed at release sites in Texas (Figure 14).
A third biological control agent, Lasioptera donacis Coutin (Diptera: Cecidomyiidae), is a leaf miner, with larvae feeding and developing in the leaf sheath of A. donax. Adult females (Figure 15) deposit eggs in holes or cracks in the leaf sheath (Goolsby et al. Reference Goolsby, Vacek, Salinas, Racelis, Moran and Kirk2017; Thomas and Goolsby Reference Thomas and Goolsby2015), including in exit holes made by T. romana. Damage to leaf sheaths occurs due to larval feeding (Figure 15) and growth of one or several fungal species on which larvae also feed (Bon et al. Reference Bon, Guermache, de Simone, Cristofaro, Vacek and Goolsby2018, Reference Bon, Goolsby, Mercadier, Guermache, Kashefi, Cristofaro, Vacek and Kirk2023), as adult females can carry the fungus Arthrinium arundinis (Corda) Dyko & B. Sutton. The leaf miner L. donacis was permitted and released at one site along the Rio Grande in 2017, but it did not establish. Studies are underway to refine rearing and release methods. In the native range, infestation by L. donacis and fungal growth causes necrosis and accelerates leaf death (Marshall et al. Reference Marshall, Goolsby, Vacek, Mastoras, Kashefi, Chaskopoulou, Smith, Badillo, Reilly, Perez de Leon and Racelis2018a), leading to shoot defoliation (Figure 15). Established populations are thus expected to increase light penetration through the canopy, which may accelerate the recovery of native riparian plant communities along the Rio Grande (Racelis et al. Reference Racelis, Davey, Goolsby, Pérez de León, Varner and Duhaime2012c; Rubio et al. Reference Rubio, Racelis, Vaughan and Goolsby2014) and other invaded watersheds, including in California, where climatic conditions are less favorable for the wasp T. romana (Marshall et al. Reference Marshall, Goolsby, Vacek, Moran, Kirk, Cortes Mendoza, Cristofaro, Bownes, Mastoras, Kashefi, Chaskopoulou, Smith, Goldsmith and Racelis2018b). Along the Rio Grande, defoliation could also make riparian habitats less hospitable to survival of cattle fever ticks [R. microplus and Rhipicephalus annalatus (Say)], as tick survival is enhanced by dense, healthy A. donax (Racelis et al. Reference Racelis, Rubio, Vaughan and Goolsby2012a), and could also increase within-stand visibility, improving the safety and effectiveness of law enforcement and cattle fever tick exclusion personnel working along the U.S.–Mexico border.

Figure 15. (Left) Adult of Lasioptera donacis leaf miner that feeds on Arundo donax. (Center) Larva of L. donacis inside leaf sheath, surrounded by fungal mycelia. (Right) Defoliation of shoots in Spain resulting from premature leaf death caused by L. donacis infestation. Photos by JAG and staff.
United States and Mexico
A binational biological control program between the U.S. Department of Agriculture–Agricultural Research Service (Edinburg, Texas) and Instituto Mexicano de Tecnología del Aguas (Jiutepec, Morelos, Mexico) was initiated in 2007 (Contreras Reference Contreras2007; Martínez Jiménez et al. Reference Martínez Jiménez, Goolsby, Racelis, Perez de Leon and Negrete Arroyos2017) with the objective of developing and implementing biological control of A. donax for protection of water resources. Additional funding was provided by the U.S. Department of Homeland Security, Customs and Border Protection to meet the operational visibility needs of the U.S. Border Patrol patrolling the international border with Mexico. Biological control of the invasive cane with specialized insects was considered to be the best long-term option for managing A. donax, because it is low in cost, sustainable, and suitable for use in large areas (Seawright et al. Reference Seawright, Rister, Lacewell, McCorkle, Sturdivant, Yang and Goolsby2009). More than 1.2 million T. romana wasps were released through a mass-rearing (Moran et al. Reference Moran, DeClerck-Flaoate, Hill, Sathyamurthy, Hill, Paynter, Goolsby, Morales-Ramos, Rojas and Shapiro2022) and release program, including releases in specialized boxes dropped from airplanes in remote areas (Racelis et al. Reference Racelis, Goolsby, Penk, Jones and Roland2010). The wasp T. romana can disperse 10 to 15 km yr−1 (Racelis et al. Reference Racelis, Goolsby and Moran2009). Populations have established in A. donax stands along 600 river-km of the Rio Grande between Brownsville and Del Rio, TX (Goolsby et al. Reference Goolsby, Gaskin, Tarin, Pepper, Henne, Auclair, Racelis, Summy, Moran, Thomas, Yang, Martínez-Jiménez, Ciomperlik, Perez de Leon and Kirk2014). In 2014, five years after the release of the wasp, live aboveground biomass (inferred from main shoot length measurement, as in Spencer et al. [Reference Spencer, Liow, Chan, Ksander and Getsinger2006]) of A. donax had decreased on average by 22% compared with assessments in 2007 across 10 sites along the Rio Grande (Goolsby et al. Reference Goolsby, Moran, Racelis, Summy, Martínez-Jiménez, Lacewell, Perez de Leon and Kirk2016), with a further decrease of 32% (total 54%) across 5 sites by 2016 (Moran et al. Reference Moran, Vacek, Racelis, Pratt and Goolsby2017), associated with galling caused by T. romana on main and lateral shoots. A total of 44 native plant species were recorded in 2016 where there were once solid monotypic stands of A. donax (Moran et al. Reference Moran, Vacek, Racelis, Pratt and Goolsby2017). The wasp T. romana has established populations in Mexico, along the Rio Grande at its northern border and in Morelos in southern Mexico (Martínez Jiménez et al. Reference Martínez Jiménez, Goolsby, Racelis, Perez de Leon and Negrete Arroyos2017). The armored scale R. donacis has established populations at more than 50 sites along the Rio Grande in Texas and Mexico, with none found on P. australis or other non-target plants (Goolsby et al. Reference Goolsby, Hathcock, Vacek, Kariyat, Moran and Martínez Jiménez2020), and its additive impact in release plots, in combination with T. romana, reduced biomass by 55% compared with plots with only T. romana at two sites on the Rio Grande (Goolsby and Moran Reference Goolsby and Moran2019). The limited dispersal ability of R. donacis has reduced its wider impacts.
Biological control has been integrated with mechanical topping at 1- or 2-m height to increase impact in the lower Rio Grande basin. Studies early in the program found that topping increased biomass allocation to lateral shoots by more than 60% compared with the more traditional practice of cutting shoots to the ground, while increasing density and length of lateral shoots by 50% to 90%, leading to increased T. romana gall density per shoot compared with regrowth from ground-cut plots (Racelis et al. Reference Racelis, Moran and Goolsby2012b) (Figure 16). Current large-scale topping projects along the Rio Grande provide immediate benefits for visibility and international border enforcement, followed by development of abundant T. romana wasp populations on lateral shoot regrowth (Figure 12) to maintain control and disperse to uncut A. donax stands (Goolsby et al. Reference Goolsby, Racelis, Moran, Kirk, Kashefi, Smith, Bon, Lacewell, Vacek, Martínez-Jiménez, Perez De Leon, Hinz, Bon, Bourdôt, Cristofaro, Desurmont, Kurose, Müller-Schärer, Rafter, Schaffner, Seier, Sforza, Smith, Stutz, Thomas, Weyl and Winston2019). The result has been widespread death of lateral shoots in both uncut and cut stands, and also death of young main shoots in the understory (Figure 17).

Figure 16. Graph (top) showing percent allocation of Arundo donax to stem and leaf tissues (left axis, main, and lateral shoots combined) and percent allocation to main and lateral shoots (right axis, stem, and leaf tissues combined) in plants allowed to regrow for 5 mo after being cut under summer conditions in Texas, USA, to ground level, 1 m, or 2 m, or left uncut. Bottom images show plots in northern California in late summer that were cut to ground in the spring and allowed to regrow without (left) and with (right) topping of shoots at 1.5-m height. Graph taken from Racelis et al. (Reference Racelis, Moran and Goolsby2012b). Photos by PJM.

Figure 17. Death of Arundo donax shoots resulting from galling infestation by the wasp Tetramesa romana. (Left) Death of lateral shoot node in uncut stand. (Center) Death of shoot that regrew after the topping protocol described in Figure 12. (Right) Death of young main shoot in the understory. Photos by JAG.
The impact of T. romana along the Rio Grande has most recently been documented in terms of water use of A. donax stands, with a 25% decrease in cumulative evapotranspiration between May and October 2015 compared with 2014, despite 2015 being wetter, coinciding with 28% to 53% higher wasp populations in 2015 (Racelis et al. Reference Racelis, Wagle, Escamilla, Goolsby and Gowda2022). Studies are needed at additional sites, but these results align with the predictions made in Moran et al. (Reference Moran, Vacek, Racelis, Pratt and Goolsby2017) that reduction of live biomass accumulation caused by T. romana would reduce the ability of A. donax to consume/waste water, leading to increased availability of water worth several millions of dollars (US$) per year (Seawright et al. Reference Seawright, Rister, Lacewell, McCorkle, Sturdivant, Yang and Goolsby2009).
In California, the wasp T. romana was first released in the Central Valley in 2010, and, with pre-topping or ground-cutting of release plots, at a total of 12 sites in northern California by 2020, with evidence of establishment (overwintering, reproduction, and dispersal) at 7 sites by 2022 (Cal-IPC 2020; Moran Reference Moran2021; PJM, unpublished data). Results from plot studies support findings from Texas that integration with cutting improves local establishment of T. romana (Cal-IPC Reference Nilsson and Berggren2021). An adventive population of this wasp is present in some coastal drainages in Southern California (Dudley et al. Reference Dudley, Lambert, Kirk, Kawagama, Julien, Sforza, Bon, Evans, Hatcher, Hinz and Rector2008). Efforts to establish this wasp population on A. donax on the Colorado River and in the Mojave Desert of southeastern California have not been successful (A Lambert, personal communication). The armored scale R. donacis was released in northern California beginning in 2014, with early evidence of establishment in release plots at sites in the Central Valley; a localized, genetically distinct adventive population was recently found near Ventura in Southern California (Braman et al. Reference Braman, Lambert, Özsoy, Hollstein, Sheehy, McKinnon, Gaskin, Goolsby and Dudley2021). The impact of the wasp and armored scale will be assessed initially in terms of changes in live biomass and shoot density. The leaf miner L. donacis is planned for release in California, targeting cooler areas at which temperatures are likely insufficient for development of large populations of T. romana (Marshall et al. Reference Marshall, Goolsby, Vacek, Moran, Kirk, Cortes Mendoza, Cristofaro, Bownes, Mastoras, Kashefi, Chaskopoulou, Smith, Goldsmith and Racelis2018b).
Programs Outside North America
South Africa
Arundo donax was ranked as the top priority for all types of control among invasive grasses in South Africa (Sutton et al. Reference Sutton, Bownes, Visser, Mapaura and Canavan2021) and at the high priority level for biocontrol among all nonnative, invasive plants in the country (Canavan et al. Reference Canavan, Paterson, Ivey, Sutton and Hill2021). A biocontrol program for A. donax was initiated in 2013 with funding from the Department of Environmental Affairs–Natural Resource Management Programmes. The program has largely been guided by developments in the biocontrol program for A. donax in the United States and has focused on T. romana and R. donacis. Herbivore surveys recorded 13 herbivorous insects, including the biological control agent T. romana (Canavan et al. Reference Canavan, Paterson, Hill and Dudley2019), accidentally introduced, widespread, and abundant on A. donax across the country (Canavan et al. Reference Canavan, Paterson, Hill and Dudley2019). Two T. romana genotypes were imported in 2018 from Granada, Spain, and Perpignan, France. Molecular analysis revealed that there are two adventive genotypes in South Africa, one found in two provinces in the tropical northeast and the other throughout the rest of the country, and they are genetically distinct from all five T. romana genotypes released in the United States (Bownes Reference Bownes2010; Sutton et al. Reference Sutton, Bownes, Visser, Mapaura and Canavan2021). Tetramesa romana is suitably host specific, with no galling of South African P. australis or Phragmites mauritianus Kunth (Sutton et al. Reference Sutton, Bownes, Visser, Mapaura and Canavan2021). In laboratory tests, wasps imported from Spain produced higher numbers of progeny compared with the adventive genotypes, but because the wasp is already widespread, release of the imported wasp accessions in South Africa are not likely (A Bownes, Landcare Research, Lincoln, New Zealand, personal communication). Integration of ongoing manual or mechanical cutting under the Working for Water Programme (Sutton et al. Reference Sutton, Bownes, Visser, Mapaura and Canavan2021) with biological control by T. romana is being investigated.
Research on R. donacis in South Africa has involved host-range studies, climate modeling, and impact and performance studies. Reproductive females were imported into a quarantine laboratory from Alicante, Spain, in 2016 and 2017. Pillay (Reference Pillay2016) conducted climate modeling to assess the suitability of South Africa’s climates for establishment of R. donacis. Using model parameters based on the native (Mediterranean Europe) and prior-introduced ranges (Texas, USA) of R. donacis, modeling using CLIMEX 4.0 software (Kriticos et al. Reference Kriticos, Maywald, Yonow, Zurcher, Herrmann and Sutherst2015) predicted that most regions of South Africa are suitable for R. donacis (Pillay Reference Pillay2016). After adjustment of environmental parameters, crawler survival rates on A. donax from South Africa averaged 25%, comparable to rates observed on the A. donax genotype common in the Rio Grande (Goolsby et al. Reference Goolsby, Racelis, Goolsby, Kirk, Cristofaro, Grusak and Perez de Leon2013). Future work is planned to finalize the host-range trials.
New Zealand
A biocontrol program for A. donax was conducted with T. romana and R. donacis imported from colonies in Texas, USA (originally from Mediterranean Spain) into a quarantine lab for a planned release on the tropical island of Rarotonga (part of the Cook Islands). This introduction did not proceed when it was found that abundance of A. donax on Rarotonga had been greatly overestimated due to confusion with the invasive grass P. purpureum (Paynter et al. Reference Paynter, Poeschko, Mitchell, Probst, Barreto, Colmán, Macedo, Dodd, Johnson, McCormack, Paterson, Sutton, Winks and Hinz2019). Funding was obtained from the National Biocontrol Collective to release both T. romana and R. donacis in New Zealand as a preemptive program to tackle a potentially major weed in the early stages of invasion. Host-specificity testing already conducted in the United States was sufficient to gain approval to release T. romana in New Zealand. To confirm that R. donacis posed no risk to New Zealand native plant species, additional testing was performed on the native grasses Isachne globosa (Thunb.) Kuntze, Spinifex sericeus R.Br., and Zoysia minima (Colenso) Zotov, and indicated minimal risk to these species. Approval to release both T. romana and R. donacis in New Zealand was granted by the Environmental Protection Authority in 2017. Tetramesa romana was released at five localities, all on the North Island of New Zealand, during the summer of 2017 to 2018 and at two additional localities in 2021. Only two sites have been checked subsequently, with no evidence of establishment. Rhizaspidiotus donacis was released for the first time at three sites (all on the North Island of New Zealand) in 2021.
General Outlook
Arundo donax has become a damaging and widespread invader on at least four continents—the Rio Grande basin of Texas and Mexico and California in North America; subtropical and low mountainous regions of South America; diverse climates in coastal desert and humid equatorial regions of Africa and in South Africa; and in Oceania (Australia, New Zealand, and at least eight island nations). It can also be problematic in disturbed riparian habitats in its ancient areas of introduction in Mediterranean Europe; and in areas of Asia close to its native range (Jiménez-Ruiz et al. Reference Jiménez-Ruiz, Hardion, Del Monte, Vila and Santín-Montanyá2021). Arundo donax forms dense thickets along rivers, canals, and reservoirs, removing water that is needed for agriculture and human consumption in regions where rainfall is scarce, and A. donax also transforms riparian ecosystems, promotes wildfires, displaces native plants and animals, hinders access and visibility for law enforcement, and can facilitate the invasion of other species. Climate modeling successfully predicted the native and global introduced range of A. donax. Based on the CLIMEX model, and with due regard for possible underreporting of localities, A. donax is likely to expand its range in riparian and wetland habitats in sub-Saharan Africa, especially eastern Africa, including Madagascar, in tropical and subtropical lowlands in South America, in the Indian subcontinent, and in southeast Asia. Genetic evidence we obtained indicates Spain is the proximal source of invasive populations in North America (Tarin et al. Reference Tarin, Manhart, Pepper, Goolsby, Moran, Contreras Arquieta and Kirk2013), while an accession from the Indus Valley (Canavan et al. Reference Canavan, Paterson and Hill2017) or Middle East (Bhikraj Reference Bhikraj2021) is the source of the invasion in South Africa. Analysis of satellite images made in 2002 and 2007 along the Rio Grande in Texas indicated an increase in the invaded area before implementation of biological control (Yang et al. Reference Yang, Goolsby and Everitt2009), illustrating that A. donax was able to increase its distribution in favorable climates even in areas where it is already widespread. Invasions have been facilitated by human-aided dispersal of plants and favored by human alteration of riparian ecosystems, especially the damming of rivers and resulting conveyance flows. Invasion risk is high in areas disturbed by wildfires and floods, as A. donax recovers quickly from these disturbance events (Coffman et al. Reference Coffman, Ambrose and Rundel2010; Frandsen and Jackson Reference Frandsen, Jackson, Jackson, Frandsen and Duthoit1994).
Arundo donax is a stress-tolerant plant, particularly water-deficit stress, due to its ability to photosynthesize efficiently. Nonetheless, the distribution of A. donax could be influenced by climate change, as stress caused by water deficit and/or climatic extremes could reduce its distribution in some equatorial and subtropical regions, with concomitant spread into temperate areas as they become more favorable (Bradley et al. Reference Bradley, Blumenthal, Wilcove and Ziska2010). Early detection and rapid response approaches are essential to slow invasion of new regions. Chemical and mechanical control programs targeting well-established A. donax populations are costly, requiring long-term follow-up; for example, 20 yr in one California watershed (Cal-IPC 2020), and in the Big Bend region of the Rio Grande in western Texas (JAG, personal observation). Short-term initiatives targeting established invasions result in dramatic visual control but are ineffective due to regrowth and/or reinvasion. Chemical and mechanical may control reduce plant biodiversity and pose other environmental risks in sensitive riparian and aquatic habitats, as well as occupational risks to humans.
Starting in the 1990s (Tracy and DeLoach Reference Tracy, DeLoach and Bell1999), the USDA-ARS developed the world’s first biological control program targeting an invasive grass, focusing on A. donax. By the seventh year after release in 2009, the shoot tip-galling wasp T. romana had reduced live biomass by 54% along the Rio Grande between Del Rio and Brownsville, TX, translating to water savings valued at US$4.4 million yr−1 (Moran et al. Reference Moran, Vacek, Racelis, Pratt and Goolsby2017). The armored scale R. donacis, now established at many sites in the lower Rio Grande basin, does not disperse rapidly, but in release plots, 8 yr after release, it reduced live biomass is reduced by up to 55% in combination with T. romana compared with plots with only the wasp. The wasp and armored scale have also been released in California, USA, and in New Zealand. Mechanical topping and biological control strategies have been integrated in the lower Rio Grande basin of Texas and Mexico for increased impact, and similar efforts are being initiated elsewhere. Biological control agents are critical tools contributing to an adaptive integrated weed management approach to reduce the economic and environmental damage caused by A. donax. All control programs should be accompanied by evaluations to verify impacts on A. donax size, growth, density, and spread, but also benefits for water resource conservation, biodiversity, and human economic activity and well-being.
Supplementary material
The supplementary material for this article can be found at https://doi.org/10.1017/inp.2023.17
Acknowledgments
The USDA-ARS authors thank Alan Kirk (USDA-ARS, European Biological Control Laboratory (EBCL), Montpellier, France (retired)) and Gerhild Kirk for their tireless efforts to find and collect the biological control agents. We also thank Ann Vacek and Crystal Salinas for their keen observations and contributions to knowledge about the biological control agents; Elena Cortés and Ángeles Marcos-García at the University of Alicante, Spain, for additional collection efforts and biological studies; the USDA-APHIS Insect Management and Molecular Diagnostics Laboratory in Edinburg, TX, for logistical support; the many students, interns, and technicians who worked at the Arthropod Quarantine Laboratory there and in the T. romana mass-rearing program; and Alexis Racelis of the University of Texas–Rio Grande Valley for many years of fruitful collaboration. We thank Adam Lambert (University of California-Santa Barbara) for additional information and many suggestions that improved the article, and an anonymous reviewer for additional suggestions. Funding was provided in part by the Department of Homeland Security, Customs and Border Protection. Mention of trade names or commercial products in this publication is solely for the purpose of providing specific information and does not imply recommendation or endorsement by the U.S. Department of Agriculture. USDA is an equal opportunity provider and employer. No conflicts of interest have been declared by the authors.
Structured Appendix
Köppen-Geiger Climate Distribution
Arundo donax is found most frequently in the warm temperate and Mediterranean climate types Cfa, Cfb, Csa, and Csb globally (Beck et al. Reference Beck, Zimmermann, McVicar, Vergopolan, Berg and Wood2018) (Figure A1), in North and Central America (Figure A2), and in Europe, the Mediterranean Basin (including far northern Africa, the Middle East, the Arabian Peninsula, and far western Asia (Figure A3); over 65% of all occurrences globally are found in these four climate types (Figure A4). Records found in continental climates (mainly Dfa, Dfb) are likely artificially protected sites, with very rare, cultivated occurrences, even in polar climates (E) (Figures A1 and A4). Frequent occurrences in arid climates (warm, cool, and cold) (BWh, BWk, BSh, BSk) are in artificially irrigated locations. There are relatively few recorded occurrences in tropical climates (Af, Am, and Aw) (Figure A4), which may represent underreporting, rather than unsuitable climates. See the main article text for more information on climate types.

Figure A1. Overlay of global distribution points for Arundo donax on Köppen-Geiger polygons. Köppen-Geiger classification data set derived using classification methods in Beck et al. (Reference Beck, Zimmermann, McVicar, Vergopolan, Berg and Wood2018) applied to data from CliMond (Kriticos et al. Reference Kriticos, Webber, Leriche, Ota, Macadam, Bathols and Scott2012), 1980 to 2010.
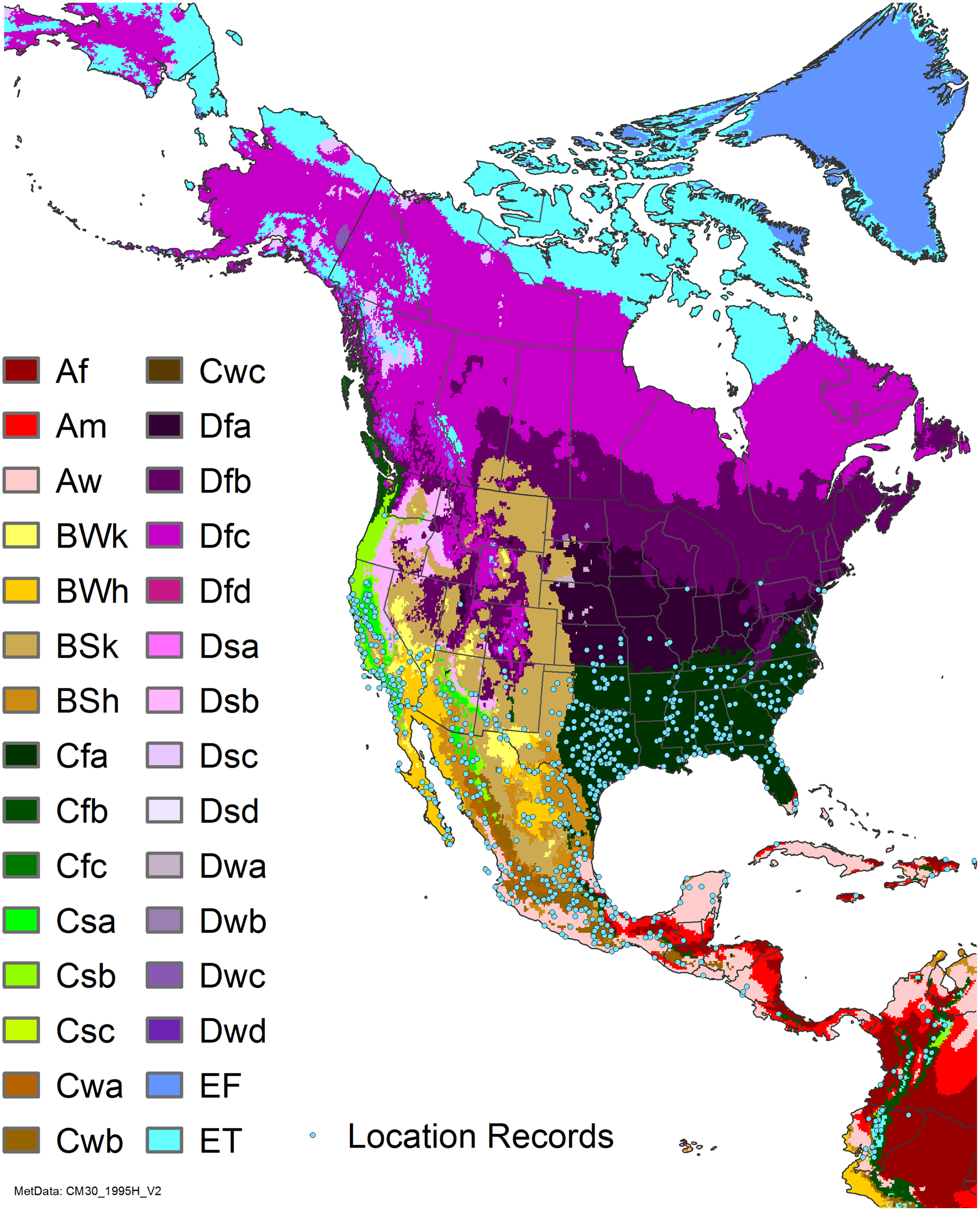
Figure A2. Overlay of distribution points for Arundo donax on Köppen-Geiger polygons covering North America. Classification data set as in Figure A1.

Figure A3. Overlay of distribution points for Arundo donax on Köppen-Geiger polygons covering Europe, the Mediterranean Basin, and western Asia. Classification data set as in Figure A1.

Figure A4. Number of occurrences of global location records for Arundo donax in relation to Köppen-Geiger climate classes. See Table 1 in the main article for climate characteristics of the climate class acronyms.
CLIMEX Model
We used CLIMEX v. 4.1 (Kriticos et al. Reference Kriticos, Maywald, Yonow, Zurcher, Herrmann and Sutherst2015; Sutherst and Maywald Reference Sutherst and Maywald1985) to model the potential distribution of A. donax. The CLIMEX model was originally fit to European distribution data in 2007, based on the Mediterranean template and using a historical climatology centered on 1975. Subsequently, Barney and DiTomaso (Reference Tracy, DeLoach and Bell2011) published CLIMEX model parameters for A. donax. Here we have refit the model using a more contemporary climatology.
Model Fitting
Climate Database. We used the CliMond CM30 1995H V2 data set for all model simulations (Kriticos et al. Reference Kriticos, Webber, Leriche, Ota, Macadam, Bathols and Scott2012). This data set contains 30-yr monthly averages of daily minimum and maximum temperature, relative humidity at 0900 and 1500 hours, and monthly precipitation totals centered on 1995. The spatial resolution is 30’ (= minutes) (approximately 50 km).
Distribution Records. We downloaded data from the Global Biodiversity Information Facility (GBIF Reference Stover, Keller, Dudley and Langendoen2021).
Growth Indices. All model parameters are summarized in Table A1. The lower temperature limit for growth was set to 10 C and the upper limit was set to 28 C. The lower and upper limits for optimal growth were set to 20 and 24 C, respectively. The DV3 value was too low. Arundo donax is observed in the Yucatan Peninsula of Mexico, where maximum temperatures often exceed 36 C. Spencer and Ksander (Reference Spencer and Ksander2006) note that A. donax grows in locations where daily average temperatures are 30 C. Accordingly, DV3 was set to 40 C to accord with heat stress temperature threshold (TTHS), which was fit to southern Algeria.
Table A1. CLIMEX parameter values for Arundo donax.

Soil Moisture. The soil moisture growth index parameters were set based on consideration of ecophysiological factors. The lower limit for growth, SM0, was set to approximate the permanent wilting point (0.1). The upper limit for growth was set to 1.5 in acknowledgment of the fact that the leaves on A. donax are carried moderately high up the stem and that it occurs in areas subject to moderate levels of inundation. The lower and upper soil moisture levels for optimal growth were set to 0.4 and 0.7, respectively, because A. donax does not grow best under inundated conditions.
Stresses
Cold Stress. Two cold stress mechanisms were explored. The lethal temperature threshold mechanism did not fit the known distribution well, requiring a biologically implausibly low accumulation rate. Conversely, the degree-day cold stress mechanism was fit to the known suitable locations in Europe provided by Alan Kirk (European Biological Control Laboratory [retired], Montpellier, France, personal communication). A location in the Massif centrale (Mirambeau, France) appears to be an outlier. It was not possible to reduce cold stress sufficiently to enable persistence at that location while still restricting Arundo donax’s projected northern range in France within reasonable limits. When considering the European range, including single occurrences in Norway and Sweden, it is important to bear in mind that it is extensively planted as a garden ornamental.
Hot Wet Stress. Hot wet stress was removed from the model. It was only impacting in limited areas around Slovenia and northern Italy. We now have known suitable locations in southern Slovenia.
Heat Stress. The heat stress factor was relaxed from the Mediterranean template in order to allow A. donax to persist in southern Algeria under irrigated conditions representative of oases.
Dry Stress. The dry stress parameters were adjusted by first setting the threshold at around permanent wilting point (0.1), then adjusting the stress accumulation rate (HDS) so as to limit the potential range to where A. donax has been observed in nonirrigated regions.
PDD. The A. donax location at Mirambeau, France, experienced a heat sum of about 815 C-days above 10 C yr−1, whereas all other known suitable locations had degree-day sums of around 1,600 C-days or more above 10 C yr−1.
Simulations
Arundo donax is frequently observed in habitats that are irrigated. The model fit to natural rainfall situations was run with a top-up irrigation scenario of 2.5 mm d−1. A composite risk map was developed using the Global Map of Irrigated Areas (GMIA) (Siebert et al. Reference Siebert, Henrich, Frenken and Burke2013) to define those areas where the results of the irrigation or natural rainfall scenarios were included in the composite risk map (Yonow et al. Reference Yonow, Ramirez-Villegas, Abadie, Darnell, Ota and Kriticos2019). The original 5’ (minute) GMIA data set was resampled up to 30’ spatial resolution, and a threshold area of 10 ha was selected to indicate that irrigation was practiced in a cell of that size.
Validation
The vast majority of records of A. donax outside its native range are modeled as suitable. In the invaded range in North America, almost all known points fall in areas modeled as suitable for persistence, and the cold range limits coincide with the northernmost bounds of the known distribution (apart from two outliers that fall in locations modeled as being unsuitably cold, South Bend, IN, and Coeur d’Alene, ID) and similar locations around New York City, NY; Erie, PA; Richmond, IL, west of Chicago on the state’s northern border; Fort Collins, CO; and Park City, UT (Figure 7 in main article). These populations are presumed to be cultivated or recent escapes from such operations. There are a few records in the Himalayas that experience a temperature regime that is infeasibly cold for A. donax persistence. This is an area of extreme topographic relief that is not well sampled at a resolution of 30’ (Kriticos and Leriche Reference Kriticos and Leriche2010), and it is likely that A. donax grows in the relatively warm valleys. There is also a location record in western China that experiences a temperature regime that is infeasibly cold for A. donax persistence. This is likely a misleading record. In Australia, there are many records that fall outside the area predicted to be suitable for persistence (environmental index [EI] > 0). These inland records fall around water bores and pastoral homesteads within the arid zone and are not invasive. In New Zealand, there is one location on the South Island (in the town of Cromwell) that falls in the growth suitability zone (GI > 0, EI = 0). This was a cultivated specimen, protected from the extremes of cold.
Results
The fitted parameters are summarized in Table A1. In the introduced range in western Europe, the distribution records in GBIF far exceed the modeled distribution in the far northern regions (EI > 0) (Figure 8 in main article), highlighting the role of this plant as a garden ornamental in this region. In these locations, we and others have observed that A. donax is protected from the effects of a harsh winter (A Kirk, personal communication). The model correctly predicts the presence of Arundo donax on every continent apart from Antarctica (Figure 1 in main article). It is most extensively observed in Europe (Figure 8 in main article) and North America (Figure 7 in main article). The records in South America, Asia, and Australia are widespread, but far less dense. In Africa (Figure A5), there is a concentration of records in southern Africa. We are mindful of the possible effects of possible underreporting biases in tropical and subtropical South America, Africa, southeast Asia including Indonesia, and island nations in Melanesia.
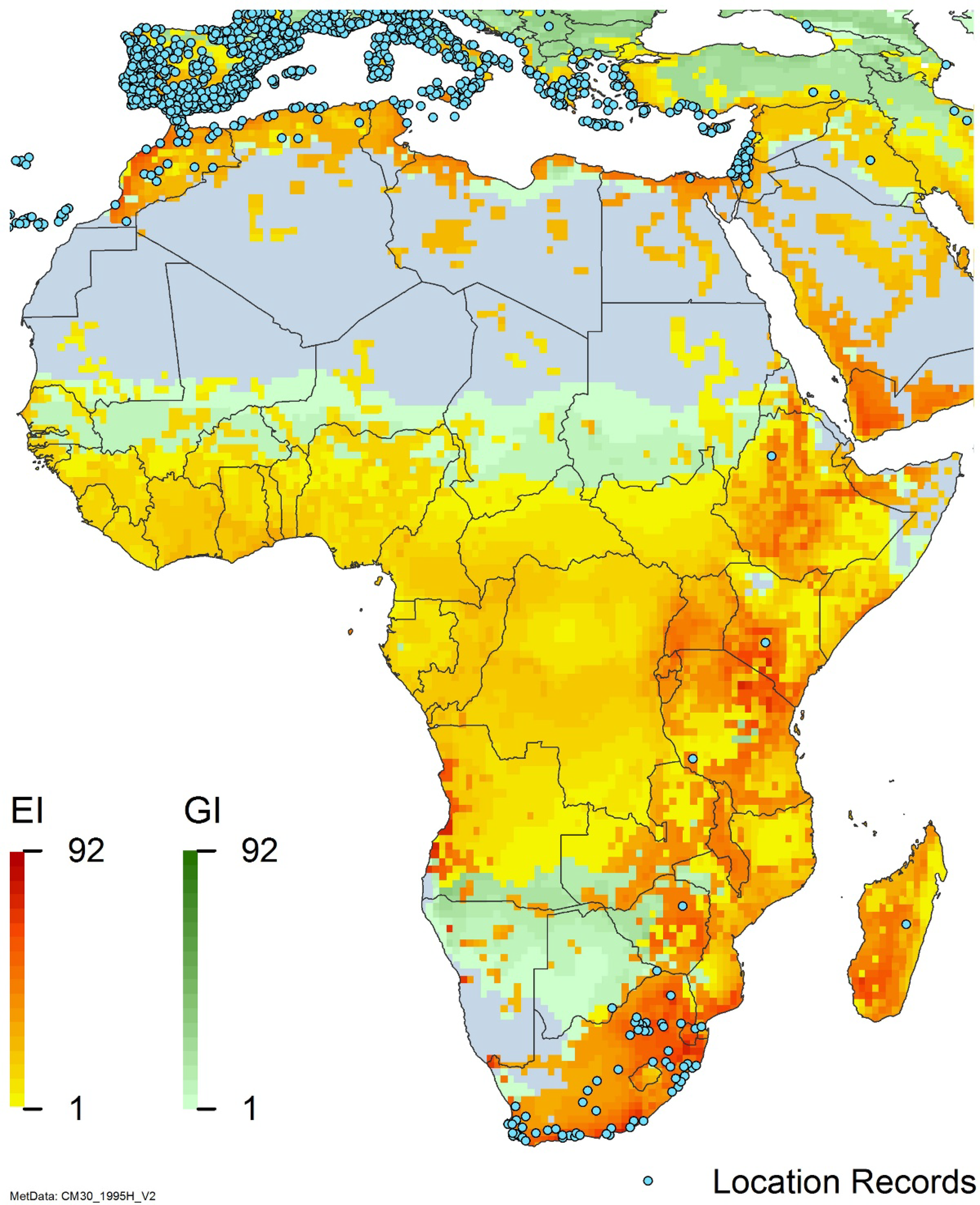
Figure A5. Climate suitability for Arundo donax in Africa, the Mediterranean Basin, and far western Asia modeled using CLIMEX, incorporating a composite of natural rainfall and a top-up irrigation scenario. Climate suitability in yellow to red colors (environmental index [EI] ≥1) indicates areas of expected year-round population persistence, while green colored areas (growth index [G1] ≥ 1) indicate regions where A. donax can grow only in one favorable season, and thus populations are not expected to persist.
In the Middle East (Figure 8 in main article), the effects of irrigation on the potential distribution of A. donax are marked, with suitable habitat apparent in the patchwork pattern of climate suitability. A similar association exists along the Nile valley through the Sahara Desert (Figure A5). The latent invasion threat is most marked in eastern Africa, though there may be substantial underreporting of its presence there. In Central Africa, as in other equatorial tropics, A. donax probably faces strong competition.
In Asia (Figure A6), A. donax is widely distributed from its native Indo-Pakistan range through areas modeled as suitable in India through to central China, and the model predicts actual localities as occurring in suitable areas. It is notable, however, that there are localities in Afghanistan, in the presumed ancient native range, where EI was 0 (Figure A6).

Figure A6. Climate suitability for Arundo donax in Asia, including its native range in the Indo-Pakistan region, modeled using CLIMEX, incorporating a composite of natural rainfall and a top-up irrigation scenario. Climate suitability in yellow to red colors (environmental index [EI] ≥1) indicates areas of expected year-round population persistence, while green colored areas (growth index [G1] ≥ 1) indicate regions where A. donax can grow only in one favorable season, and thus populations are not expected to persist.
Globally, most observations of A. donax fall within EI > 0 in the CLIMEX model (Figure 3 in the main article), indicative of successful model validation (Kriticos et al. Reference Kriticos, Maywald, Yonow, Zurcher, Herrmann and Sutherst2015).
Discussion
Arundo donax has expanded far beyond its native range globally into climates that are as suitable or more suitable than those in its native range, mainly through anthropogenic spread followed by regional invasion. Despite exhibiting clonal reproduction throughout its invasive range, the wide range of climates that are suitable for A. donax invasion reflect its ability to adapt physiologically and phenologically. It is not clear whether significant gaps in the known distribution in the global equatorial tropics, modeled as climatically suitable, reflect underreporting, lack of introduction, or biotic competition factors not considered in the model. There appears to be room for much infill of the suitable range, and the potential for a great deal of range expansion into tropical regions, as well as in northeastern China and throughout Southeast Asia, Melanesia, and Australasia.