In the Netherlands and Denmark, ampicillin-resistant, vancomycin-susceptible E. faecium (ARE) has been the dominant hospital phenotype of Enterococcus faecium for years, stably contributing to >80% of all invasive E. faecium isolates. Reference Top, Willems and Bonten1,2 As elsewhere, these countries are faced with increased polyclonal hospital outbreaks of E. faecium with combined resistance to ampicillin and vancomycin (VRE). Reference de Greeff and Mouton3,Reference Borck Høg, Korsgaard and Sönksen4 VRE presumably originated directly from the omnipresent ARE through acquisition of vanA or vanB genes. Reference Guzman Prieto, van Schaik and Rogers5–Reference Raven, Reuter and Reynolds8 In the Netherlands, 1%–2% of invasive E. faecium isolates are VRE, and in Denmark, prevalence had risen to 11% by 2019, with particular predominance in the capital region. 2,Reference Hammerum, Baig and Kamel9 In both countries, infection control policies are in place to prevent nosocomial transmission of VRE (contact precautions for VRE carriers supplemented by contact tracing and augmented general hygiene measures during outbreaks), 10,11 but not for ARE. Failure to control VRE transmission will most likely result in VRE endemicity due to replacement of nosocomial ARE populations by VRE. Reference Top, Willems and Bonten1,Reference Johnstone, Policarpio and Lam12
Controlling VRE outbreaks imposes a great burden on finances and hospital personnel. Reference Dik, Dinkelacker and Vemer13 To make an appropriate cost–benefit analysis of containing VRE spread in a healthcare system, it is essential to quantify the benefits of such a strategy. The most important threat for individual patients is the adversity patients experience due to VRE infection compared to ARE infection. A meta-analysis reported increased mortality after VRE bacteremia compared to ARE bacteremia, Reference DiazGranados, Zimmer, Klein and Jernigan14 but most studies included had been performed before effective antibiotics for VRE were available. Since then, few have attempted to quantify the effects of VRE infection compared to ARE infection, and those available often suffered from methodological drawbacks, such as combining E. faecium and E. faecalis infection and incomplete control for confounding. Reference Prematunge, MacDougall and Johnstone15
We investigated how much mortality is increased when vancomycin resistance is superimposed on ampicillin resistance in E. faecium bacteremia in both the Netherlands and the capital region of Denmark. We also analyzed whether any such attributable mortality is the result of a delay in appropriate antibiotic therapy because this is the most likely mediating mechanism.
Methods
Study design, setting, and participants
We addressed a causal research question with an observational study in which confounding bias was dealt with using a 2-step approach. First, by means of matching, patients with ARE bacteremia and with underlying disease severity similar to the VRE bacteremia patients were chosen as comparison group. Second, we controlled for remaining imbalances in confounding factors after matching by means of adjustments in multivariable models.
This method resulted in a retrospective, matched-cohort study in which episodes of bacteremia caused by E. faecium with co-resistance to ampicillin and vancomycin (designated VRE) were compared to control episodes of bacteremia caused by E. faecium with resistance to ampicillin and susceptibility to vancomycin (designated ARE). Episodes with ampicillin-susceptible VRE bacteremias were excluded. Depending on the availability, a maximum of 4 ARE bacteremias were matched to each VRE bacteremia on 4 variables. Matched ARE bacteremias always originated from the same hospital and ward (categorized into 8 specialties). Subsequently, for matching length of hospital stay prior to bacteremia, the matching interval was determined such that from at least 5 ARE bacteremias (if available), patients could be selected with age most similar to the patient with VRE bacteremia (Supplementary Material online).
No formal sample size calculation was performed because, VRE bacteremias are rare in both countries, and we had to rely on the willingness of hospitals to participate. In the Netherlands, VRE bacteremia episodes were identified in 13 hospitals through the national surveillance system ISIS-AR Reference Altorf-van der Kuil, Schoffelen and de Greeff16 ; 11 participated in this study, as did 5 hospitals not linked to ISIS-AR (see Supplementary Table S1 online for details of participating hospitals). In Denmark, the DACOBAN database was used to identify patients with VRE bacteremia. DACOBAN is a registry of all positive blood cultures from 10 of 11 hospitals in the capital region. Reference Gradel, Schønheyder, Arpi, Knudsen, Østergaard and Søgaard17 Patients with VRE bacteremia were identified in 4 of the 10 hospitals.
In the Netherlands, we included patients with VRE bacteremia that occurred between January 1, 2009, and December 31, 2012, with deviations in some hospitals (Supplementary Table S1 online). In Denmark, we included patients with VRE bacteremia that occurred between January 1, 2012, and December 31, 2014.
For resistance classification, ampicillin and vancomycin minimum inhibitory concentrations (MICs) or zone diameters were used as reported by local laboratories. All Danish laboratories interpreted antimicrobial susceptibility according to EUCAST standards, but most Dutch laboratories switched from CLSI to EUCAST standards around the study period. Reference Altorf-van der Kuil, Schoffelen and de Greeff16 Vancomycin resistance had to be confirmed by E-test or demonstration of the presence of vanA or vanB. The specific VRE genotype was based on PCR-testing or on teicoplanin susceptibility (resistant categorized as vanA, susceptible as vanB) if PCR testing had not been performed.
The institutional review board of the coordinating center judged the study to be exempt from the Dutch Medical Research Involving Human Subjects Law due to its retrospective nature. Informed consent was not necessary because data were anonymized by treating physicians. In all participating study sites, local regulations for such studies were followed. In Denmark, the study was approved by the Danish Data Protection Agency (no. 2012-58-0004) and the Danish Health and Medicines Authority (no. 3-3013-1118/1).
Data collection
After selection of cases and controls, charts were reviewed with the date of the index blood culture (bacteremia onset) as the reference date. Follow-up data (censoring date or date of death) for at least 30 days after bacteremia onset, but preferably up to 1 year after bacteremia onset, were collected (Supplementary Material online). However, the primary outcome of the study was defined as mortality within 30 days of bacteremia onset. A description of the potential confounding variables, infection-related variables, and secondary outcomes for which data were collected is provided in the Supplementary Material (online). For Dutch patients, additional data were collected to calculate an Acute Physiology Score before bacteremia onset to better capture the prior state of health of patients aside from registered comorbidities. Reference Knaus, Wagner and Draper18
Antibiotic use was registered from 30 days prior to bacteremia onset until 14 days after onset, including type of antibiotic, route of administration, and starting and stopping dates. Antibiotic use prior to bacteremia was considered a potential confounder, whereas treatment provided for the E. faecium bacteremia episode was considered the main intermediate variable on the causal pathway leading from vancomycin resistance to increased mortality. To analyze this variable, on each calendar day from bacteremia onset onward (considered day 0), antibiotic therapy was categorized as (1) either E. faecium-covering (ie, including vancomycin, linezolid, daptomycin, teicoplanin, quinupristin/dalfopristin and/or tigecycline, regardless of vancomycin resistance phenotype) or not, and (2) appropriate (ie, all of the aforementioned antibiotics for ARE infection, all except vancomycin for vanB VRE infection, and all except vancomycin and teicoplanin for vanA VRE infection) or inappropriate.
Statistical analysis
The relation between ARE or VRE and 30-day mortality was estimated using Cox regression models, unadjusted as well as adjusted for potential confounding variables. All models were Cox proportional hazards models, with stratification on matched sets, robust standard errors, and correlation between individuals that were included multiple times. For models without censoring (ie, all except the model with 1 year follow-up), all episodes were given the same arbitrary follow-up time. The Efron approximation for tied survival times was used so that hazard ratios (HRs) could be interpreted as risk ratios (RRs). Reference Cummings19 The creation of adjusted models involved inclusion of all potential confounders deemed relevant by us a priori to achieve optimal correction, followed by the removal of redundant variables to increase precision. Reference Greenland, Daniel and Pearce20 For the Dutch subgroup, an additional analysis was performed in which the variable Acute Physiology Score before bacteremia onset was added to a model already adjusted with regular confounders. All procedures are described in detail in the Supplementary Material (online). In case of missing values for the variables included in a model, individuals were excluded from the analysis.
Several additional models were created to evaluate mediation of the effect of VRE on mortality through appropriateness of antibiotic therapy. An interaction between vancomycin resistance and appropriateness of therapy was included. Because appropriateness of therapy is a time-varying variable, 3 models were created in which the baseline was moved to the end of day 0 (day of the index blood culture), +1, and +2, respectively. Patients having died or censored before or on the day of the baseline were removed from the analysis. Appropriateness of therapy in each model reflected the state at baseline.
All statistical analyses were performed in R version 4.0.3 software (R Foundation for Statistical Computing, Vienna, Austria) 21 using several packages. Reference Therneau22–Reference Kassambara, Kosinski and Biecek29
Results
Patient characteristics
Overall, 63 VRE episodes were matched with 234 ARE episodes: 36 and 130 for the Netherlands and 27 and 104 for Denmark. VRE and matched ARE bacteremia episodes had largely similar characteristics (Table 1). Differences between the 2 countries involved most prominently treatment restriction prior to bacteremia. The latter variable is generally registered on a dedicated location in Dutch health records but had to be extracted from written notes in Denmark. Also, comorbidities were retrieved from the DACOBAN registry in Denmark, whereas they were extracted from medical notes in the Netherlands.
Table 1. Characteristics and Outcomes of Vancomycin-Resistant Enterococcus faecium (VRE) and Matched Ampicillin-Resistant E. faecium (ARE) Bacteremias a

Note. ICU, intensive care unit; IQR, interquartile range.
a This table presents a selection of recorded variables. A full overview is available in Supplementary Table S2 (online).
Most VRE were vanA (n = 41, 65%); 19 were vanB (30%); 1 isolate carried both vanA and vanB; and 2 isolates could not be categorized. All VRE isolates from Denmark (n = 27) were vanA. Also, 17 isolates were categorized based on teicoplanin susceptibility (3 vanA and 14 vanB, all from the Netherlands).
Mortality
Crude 30-day mortality rates were 40% for VRE and 32% for ARE: 33% and 27% for VRE and ARE, respectively, in the Netherlands and 48% and 38% in Denmark. In the Netherlands, the 30-day mortality rates per VRE phenotype were 29% for vanA and 37% for vanB. The unadjusted RR for 30-day mortality of VRE (compared to ARE) was 1.27 (95% confidence interval [CI], 0.87–1.84; Table 2). Adjustment for confounding increased the RR to 1.54 (95% CI, 1.06–2.25). Within the Dutch subgroup, addition of the confounder Acute Physiology Score before bacteremia onset to an otherwise optimally adjusted model reduced the RR of vancomycin resistance from 1.62 (95% CI, 0.85–3.12) to 1.17 (95% CI, 0.55–2.52) (Supplementary Table S3 online).
Table 2. Regression Models for 30-Day Mortality a

a This table presents risk ratios (RR, with 95% confidence intervals) for 30-day mortality for the exposure of interest (vancomycin resistance), with and without adjustment for confounding variables, and with and without the intermediate variable inappropriate antibiotic therapy on the day of the index blood culture. In the Supplementary Material (online), the adjustment procedure is described. Combined models include data from both the Netherlands and Denmark.
Moreover, 1-year mortality appeared to be higher among Danish patients with VRE compared to patients with ARE bacteremia or Dutch patients with VRE or ARE bacteremia, although confidence intervals (CIs) of survival curves were largely overlapping (Fig. 1). In the Cox model for mortality up to 1 year, the adjusted HR for VRE amounted to 1.25 (95% CI, 0.80–1.98).

Fig. 1. Kaplan-Meier curves showing 1-year survival for vancomycin-resistant Enterococcus faecium (VRE) and matched ampicillin-resistant E. faecium (ARE) bacteremias, stratified by country (the Netherlands vs Denmark). Dashed lines indicate boundaries of the 95% confidence interval of each survival curve. Plus signs on survival curves indicate censoring events. The table below shows the number of patients in the risk set (ie, neither deceased nor censored) at 60-day intervals.
Antibiotic therapy
Visual inspection of cumulative incidence plots revealed that initiation of E. faecium-covering antibiotic therapy occurred faster in VRE than in ARE episodes (Fig. 2) but that initiation of appropriate antibiotic therapy occurred faster for ARE compared to VRE bacteremia (Fig. 3). In Denmark, appropriate antibiotic therapy for both ARE and VRE bacteremia was started earlier than in the Netherlands, and VRE treatment often consisted of combination treatment with linezolid and daptomycin (Table 3).

Fig. 2. Cumulative incidence plots showing time to Enterococcus faecium–covering antibiotic therapy (Efm Tx), and its competing risk 'mortality before onset of Efm Tx', separately for vancomycin-resistant E. faecium (VRE) and matched ampicillin-resistant E. faecium (ARE) bacteremias.

Fig. 3. Cumulative incidence plots showing time to appropriate antibiotic therapy (appr Tx), and its competing risk 'mortality before onset of appr Tx', separately for vancomycin-resistant Enterococcus faecium (VRE) and matched ampicillin-resistant E. faecium (ARE) bacteremias.
Table 3. Overview of Antibiotic Therapy for Vancomycin-Resistant Enterococcus faecium (VRE) and Matched Ampicillin-Resistant E. faecium (ARE) Bacteremias
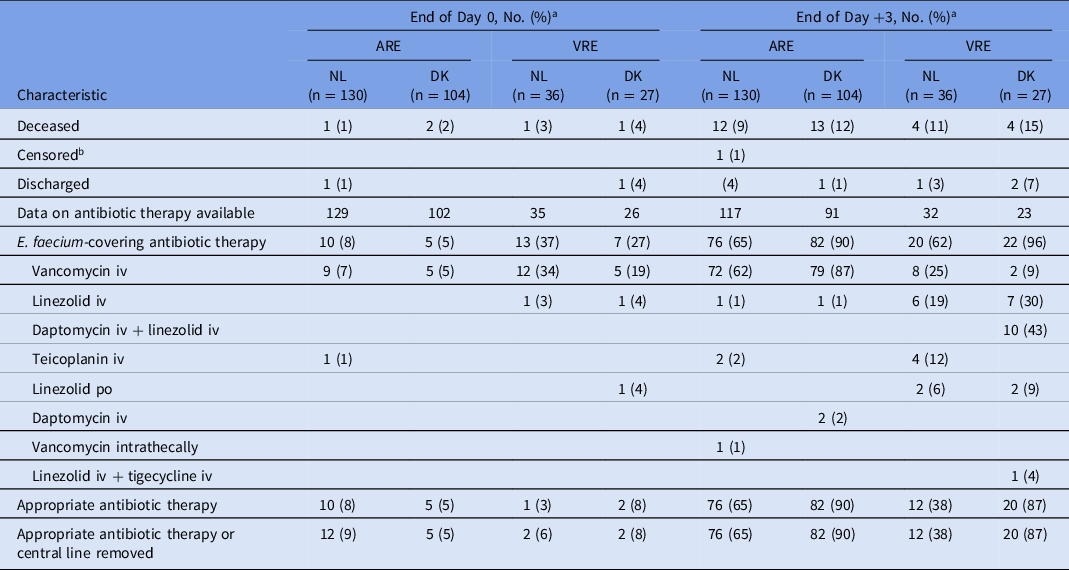
Note. ARE, ampicillin-resistant Enterococcus faecium; VRE, vancomycin-resistant Enterococcus faecium; DK, Denmark; iv, intravenously; NL, Netherlands; po, orally.
a Day 0 is the day of the index blood culture of the ARE/VRE bacteremia episode.
b 1 Dutch patient with ARE bacteremia was censored for the assessment of antibiotic therapy (not for mortality) due to transfer to another hospital.
Inappropriate antibiotic therapy on the day of the index blood culture (day 0) is a potential mediator of an increase in mortality in VRE cases, but inclusion of this variable in the model relating VRE to 30-day mortality did not reduce the point estimate of VRE (Table 2). In models with an interaction between vancomycin resistance and appropriateness of therapy, VRE patients on inappropriate therapy consistently fared worse than ARE patients on inappropriate therapy (Table 4). The effect estimates for both ARE and VRE patients on appropriate therapy were uncertain.
Table 4. Regression Models for 30-Day Mortality Evaluating Appropriateness of Antibiotic Therapy a

Note. ARE, ampicillin-resistant Enterococcus faecium bacteremia; VRE, vancomycin-resistant Enterococcus faecium bacteremia; NA, not available.
a This table presents risk ratios (RR, with 95% confidence intervals) for 30-day mortality for the interaction between vancomycin resistance and appropriateness of therapy. The baseline for these models (at which appropriateness of antibiotic therapy is assessed each time) is positioned on 3 different moments, namely the end of the day of the index blood culture (day 0), the end of the day after (day +1), and the end of the day thereafter (day +2). RRs are presented with and without adjustment for confounding variables. In the Supplementary Material (online), the adjustment procedure is described. All models include data from both the Netherlands and Denmark.
Discussion
The results of this study reveal that, after matching and further analytic control for confounders, VRE bacteremia was, compared to ARE bacteremia, associated with 54% higher risk for mortality after 30 days (RR, 1.54; 95% CI, 1.06–2.25). However, this increased risk of death must be explained by other factors than a delay in appropriate antibiotic therapy.
Our estimate for the effect of VRE on mortality is similar to the reported pooled OR of 2.52 (95% CI, 1.9–3.4) in a meta-analysis from 2005, Reference DiazGranados, Zimmer, Klein and Jernigan14 which translates to an RR of 1.70 with a 32% mortality rate in the nonexposed group. Reference Zhang and Yu30 This seems remarkably identical; the studies included in that meta-analysis had been performed before the availability of effective antibiotics for VRE, such as linezolid and daptomycin. However, a small study comparing older and newer antibiotics in 113 VRE bacteremia cases concluded that newer antibiotics had not brought discernible benefits to patient outcome. Reference Erlandson, Sun, Iwen and Rupp31 A later meta-analysis on the effect of VRE on mortality in the era of effective antibiotic therapy could only present an unadjusted estimate and thus could not be compared to our study. Reference Prematunge, MacDougall and Johnstone15 Recent Australian and German studies all found point estimates reflecting increased mortality in case of vancomycin resistance, with confidence intervals indicating nonsignificance. Reference Cheah, Spelman and Liew32–Reference Dubler, Lenz and Zimmermann34 By analyzing the E. faecium bacteremia subset and not including intermediate variables in the adjusted model, the OR of 1.283 (95% CI, 0.801–2.057) from Kramer et al Reference Kramer, Remschmidt and Werner33 most closely reflects our estimation procedure.
There are 3 causal pathways along which vancomycin resistance could lead to increased mortality: (1) increased virulence of VRE compared to ARE, (2) less effective or more toxic antibiotics for VRE than for ARE, and (3) a delay in initiation of appropriate antibiotic therapy for VRE bacteremia. We cannot fully exclude a systematic difference in pathogenicity between ARE and VRE. For example, Bender et al Reference Bender, Kalmbach, Fleige, Klare, Fuchs and Werner35 showed that acquisition of vanB by E. faecium is accompanied by the transfer of larger genetic fragments. However, most studies conclude that both phenotypes belong to the same, well-characterized, core genomic lineage of E. faecium. Reference Guzman Prieto, van Schaik and Rogers5,Reference Raven, Reuter and Reynolds8
Regarding the comparative intrinsic effectiveness of the antibiotic options for VRE, the data are limited. In studies on gram-positive infections including bacteremia in general and on methicillin-resistant Staphylococcus aureus (MRSA) infection specifically, linezolid and daptomycin have performed on par with vancomycin. Reference Sparham, Linezolid, Grayson, Cosgrove and Crowe36,Reference Muller, Gyssens, Grayson, Cosgrove and Crowe37 However, whether even high-dose daptomycin (10–12 mg/kg/day) achieves drug exposure levels to reliably treat enterococcal infections remains uncertain. Reference Turnidge, Kahlmeter, Cantón, MacGowan and Giske38 In this study, daptomycin for VRE bacteremia (dose, 6 mg/kg/day) was consistently prescribed in combination with linezolid.
The observed increased mortality in cases of VRE bacteremia, therefore, could be expected to result from a delay in appropriate antibiotic therapy, which has been implicated previously in worse outcomes in cases of enterococcal bacteremia. Reference Zasowski, Claeys, Lagnf, Davis and Rybak39 Our models that included appropriateness of therapy would have lent support to this hypothesis if mortality risk had no longer depended on the presence of vancomycin resistance after stratification on appropriateness of therapy. Instead, VRE patients on inappropriate therapy continuously fared worse than ARE patients on inappropriate therapy. Importantly, therapy over time may be reflective of the evolving disease severity of the patient, and collider bias may be induced by conditioning on appropriateness of therapy. Reference Hernán, Hernández-Díaz and Robins40 Thus, the effect estimates of appropriateness of therapy after baseline may not reflect true causal associations. However, this trend is discernible from the day of the index blood culture onward, which increases our confidence that the difference in duration until appropriate therapy is unable to explain the increased mortality in case of VRE bacteremia. A final indication for this hypothesis stems from the comparison between countries in this study. Overall mortality in Denmark for both ARE and VRE bacteremia was higher than in Netherlands, although appropriate therapy for both types of bacteremia was initiated considerably faster in Denmark than in the Netherlands.
These biologically plausible mediators cannot explain increased mortality due to vancomycin resistance; thus, the possibility remains that these observed effects are due to unmeasured confounding. This possibility is supported by 2 additional observations. First, a measure for clinical disease severity immediately before bacteremia onset was not available for the Danish patients. For the Dutch patients, we could calculate the Acute Physiology Score, and when included in our country-specific analyses, it substantially reduced the effect estimate for mortality. Second, the association between vancomycin resistance and mortality persisted over the course of a full year. Infections may have long-term sequelae, Reference Leibovici41 but it seems unlikely that sustained mortality differences will emerge that can be causally related to vancomycin resistance. A Dutch population-based study reported that the incidence of recurrent bacteremia, an example of a long-term consequence, only marginally differed between ARE and VRE bacteremia. Reference Woudt, de Greeff, Schoffelen, Vlek and Bonten42 An alternative explanation is that underlying prognostic factors at the time of onset of enterococcal bacteremia were dissimilar.
This study has several limitations. First, results of this study may not apply to E. faecium bacteremia in general because a nonrandom subset of ARE bacteremias was included. The matched design does not allow direct comparisons of raw proportions other than for VRE versus ARE. Second, some loss in precision may be expected in stratified analyses because not all matched sets can be used for parameter estimation. Third, measurements of comorbidities and treatment restrictions differed between both countries, whereas these differences were not included in models. Fourth, duration until initiation of appropriate antibiotic therapy could not be reliably measured in hours and was reflected instead by calendar days.
Finally, some studies suggest that the incidence of infections with VRE occur on top of the existing incidence of infections caused by vancomycin-susceptible enterococci. Reference Ammerlaan, Harbarth and Buiting43,Reference Popiel and Miller44 In that case, a comparison between VRE bacteremia and an uninfected control group would be more appropriate, as described by Chiang et al. Reference Chiang, Perencevich and Nair45 Notably, however, these incidence rates may have been confounded by the fact that E. faecalis was not separated from E. faecium, and results from molecular epidemiological studies provided strong evidence that ARE and VRE occupy the same niche within the bacterial hospital ecology. Reference Guzman Prieto, van Schaik and Rogers5
In conclusion, VRE bacteremia was, when compared to ARE bacteremia, associated with higher mortality. This finding could not be explained by delays in initiation of appropriate antibiotic therapy, although the relevant models may be affected by collider bias, post hoc detection of spurious associations, and simultaneous lack of statistical power for some subgroups. One possible explanation for the higher mortality is unmeasured confounding. An alternative explanation is that VRE is more virulent than ARE, for which the genetic basis would then most likely be encoded in the accessory genome. Further studies are warranted to explore this possibility.
Acknowledgments
We thank all participating hospitals for making data collection possible. We specifically thank Marja Smid for her assistance with the data collection in the Netherlands, and Kim Gradel and Steen Rasmussen for extraction of comorbidity data in Denmark. We also thank Rob Willems for critically reviewing an earlier version of this manuscript.
Financial support
This work was supported by a research grant from the Netherlands Organisation for Health Research and Development (project no. 205200007).
Conflicts of interest
All authors report no conflicts of interest relevant to this article.
Supplementary material
To view supplementary material for this article, please visit https://doi.org/10.1017/ice.2021.216