Introduction
Gestational diabetes mellitus (GDM), defined as glucose intolerance that results in hyperglycaemia with first recognition during pregnancy (Eades et al., Reference Eades, Cameron and Evans2017; Hasain et al., Reference Hasain, Mokhtar, Kamaruddin, Mohamed Ismail, Razalli, Gnanou and Raja Ali2020), is an increasing public health concern with a rising prevalence of 5.4% in European and 7.6% in North American pregnant women (Casagrande et al., Reference Casagrande, Linder and Cowie2018). GDM impacts maternal-foetal health causing both short- and long-term adverse effects, including higher risk of preeclampsia and caesarean delivery for mothers; and macrosomia, preterm birth, respiratory distress, and shoulder dystocia for foetuses (HAPO Study Cooperative Research Group et al., Reference Metzger, Lowe, Dyer, Trimble, Chaovarindr, Coustan, Hadden, McCance, Hod, McIntyre, Oats, Persson, Rogers and Sacks2008; Schneider et al., Reference Schneider, Hoeft, Freerksen, fischer, Roehrig, Yamamoto and Maul2011; Wendland et al., Reference Wendland, Torloni, Falavigna, Trujillo, Dode, Campos, Duncan and Schmidt2012; Kc et al., Reference Kc, Shakya and Zhang2015; Billionnet et al., Reference Billionnet, Mitanchez, Weill, Nizard, Alla, Hartemann and Jacqueminet2017). More than half of pregnant women who develop GDM have at least one risk factor: >25 years old, body mass index (BMI) > 30 kg/m2, history of impaired glucose tolerance, previous pregnancies with GDM or macrosomia, multiple pregnancies, family history of type 2 diabetes, among others (Lefkovits et al., Reference Lefkovits, Stewart and Murphy2019). GDM diagnosis is made in the first trimester if a fasting glucose test returns a plasma glucose value ≥92 and <126 mg/dL or if returning a normal value, through an oral glucose tolerance test (OGTT) made at 24–28 weeks of gestation if a fasting plasma glucose value ≥92 mg/dL or ≥180 mg/dL at one hour or ≥153 mg/dL at 2 hours (Wendland et al., Reference Wendland, Torloni, Falavigna, Trujillo, Dode, Campos, Duncan and Schmidt2012). Appropriate management of hyperglycaemia combined with lifestyle interventions, diet, and exercise prevents maternal obesity and GDM (Koivusalo et al., Reference Koivusalo, Rönö, Klemetti, Roine, Lindström, Erkkola, Kaaja, Pöyhönen-Alho, Tiitinen, Huvinen, Andersson, Laivuori, Valkama, Meinilä, Kautiainen, Eriksson and Stach-Lempinen2016; Wang et al., Reference Wang, Wei, Zhang, Zhang, Xu, Sun, Su, Zhang, Liu, Feng, Shou, Guelfi, Newnham and Yang2017). Other approaches are also available when diet and exercise alone are insufficient to control blood glucose levels, including pharmacological (insulin and/or oral hypoglycaemic agents, such as metformin) and non-pharmacological therapies (probiotics; Simmons, Reference Simmons2015). Pharmacotherapy has benefits for glucose control, especially insulin since it is unable to cross the placental barrier. However, this approach may result in side effects, including hypertensive disorders, when using insulin (Brown et al., Reference Brown, Grzeskowiak, Williamson, Downie and Crowther2017) and diarrhoea, abdominal pain, and headache, when using metformin (Dodd et al., Reference Dodd, Grivell, Deussen and Hague2018). Probiotics are defined as “live microorganisms which when administered in adequate amounts confer a health benefit on the host” (Food and Agricultural Organization [FAO] of the United Nations and World Health Organization [WHO], 2001] and thus may be part of a non-pharmacological therapy. Gut microbiota, the group of living microorganisms colonising the gastrointestinal tract, is linked to human metabolism regulation. Alterations in gut microbiota composition are associated with metabolic diseases such as obesity, type 2 diabetes, and metabolic syndrome (Pascale et al., Reference Pascale, Marchesi, Marelli, Coppola, Luzi, Govoni, Giustina and Gazzaruso2018) and can play an essential role in modulating insulin resistance (IR) and inflammatory response in GDM (Kuang et al., Reference Kuang, Lu, Li, Li, Yuan, He, Chen, Xiao, Shen, Qiu, Wu, Hu, Wu, Li, Chen, Deng, Papasian, Xia and Qiu2017). Diet and probiotics can affect gut microbiota composition (Wen and Duffy, Reference Wen and Duffy2017; Ponzo et al., Reference Ponzo, Fedele, Goitre, Leone, Lezo, Monzeglio, Finocchiaro, Ghigo and Bo2019,Reference Ponzo, Ferrocino, Zarovska, Amenta, Leone, Monzeglio, Rosato, Pellegrini, Gambino, Cassader, Ghigo, Cocolin and Bob) and therefore can be a potential associative therapy for GDM if applied simultaneously.
The aim of this literature review was to compare the results of studies reporting the efficacy of both approaches (probiotics and diet) to prevent and manage GDM during pregnancy and to clarify if they could be potential therapeutic adjuvants for GDM treatment.
Current status of knowledge
The role of human gut microbiota in homeostasis
The human gastrointestinal tract is colonised by a collection of approximately 100 trillion microorganisms (Lozupone et al., Reference Lozupone, Stombaugh, Gordon, Jansson and Knight2012), whose composition is determined by host genetics, diet, immune responses, and the environment (Lynch and Pedersen, Reference Lynch and Pedersen2016). The development of our gut microbiota begins immediately at birth, being initially undifferentiated and progressively shaped by three major factors – type of delivery, breastfeeding, and weaning (Nicholson et al., Reference Nicholson, Holmes, Kinross, Burcelin, Gibson, Jia and Pettersson2012). In adult life, the gut microbiota has similar patterns between individuals at higher phylogenetic levels, being mainly composed of five bacteria phyla – Bacteroidetes, Firmicutes, Proteobacteria, Actinobacteria, and Verrucomicrobia (Tremaroli and Bäckhed, Reference Tremaroli and Bäckhed2012). Firmicutes and Bacteroidetes are the most prevalent, representing more than 90% of the overall intestinal microbiota (Shen et al., Reference Shen, Obin and Zhao2013). At a genus level, some of the most frequently found bacteria are Bifidobacterium, Lactobacillus, Bacteroides, Clostridium, Escherichia, Streptococcus, and Ruminococcus (Conlon and Bird, Reference Conlon and Bird2015). At a species level, the variability is much higher, with healthy individuals having highly diverse gut microbiota (Flint et al., Reference Flint, Duncan, Scott and Louis2015). This complex micro-ecosystem holds a synbiotic relationship between each other (the microbes) and the host, who provides them a balanced environment to live while microbes provide the host with several benefits through a variety of functions such as modulating the intestinal barrier (Natividad and Verdu, Reference Natividad and Verdu2013), producing bioactive compounds like short-chain fatty acids (SCFA; Topping and Clifton, Reference Topping and Clifton2001), maintaining host immune system (Gensollen et al., Reference Gensollen, Iyer, Kasper and Blumberg2016), and protecting the host against ingested pathogens (Bäumler and Sperandio, Reference Bäumler and Sperandio2016). Alterations in the intestinal microbiota and its metabolic pathways, termed dysbiosis (major changes in the resident microbial community with impairments for the host; Clemente et al., Reference Clemente, Ursell, Parfrey and Knight2012), may contribute to the development of several chronic diseases, such as inflammatory bowel disease, multiple sclerosis, arthritis, and allergic inflammation (Kamada et al., Reference Kamada, Seo, Chen and Núñez2013). Hence, it is important to maintain the balance of our gut microbiota composition to ensure homeostasis. Gut microbial fermentation of non-digestible foods (dietary fibres and resistant starches) maintains bowel health (Canfora et al., Reference Canfora, Jocken and Blaak2015) and leads to the production of SCFA, mainly butyrate, acetate, and propionate, via diverse biochemical pathways (Topping and Clifton, Reference Topping and Clifton2001). These metabolites can be used as energy sources by colonic epithelial cells or absorbed into the bloodstream (den Besten et al., Reference den Besten, van Eunen, Groen, Venema, Reijngoud and Bakker2013). In the colon lumen, acetate, and propionate, mainly produced by Bacteroidetes (LeBlanc et al., Reference LeBlanc, Chain, Martín, Bermúdez-Humarán, Courau and Langella2017), release peptide YY (PPY) and glucagon-like peptide (GLP-1), which influence satiety and bowel transit; whereas butyrate, mainly produced by Firmicutes (LeBlanc et al., Reference LeBlanc, Chain, Martín, Bermúdez-Humarán, Courau and Langella2017), inhibits histone deacetylases (HDACs) and a specific G protein-coupled receptor (GPR109A), conferring anti-inflammatory effects (Koh et al., Reference Koh, de Vadder, Kovatcheva-Datchary and Bäckhed2016), apart from modulating the expression of tight junction protein and mucins (Canfora et al., Reference Canfora, Jocken and Blaak2015). Additionally, SCFA also plays an important role in blood glucose level regulation and glucose homeostasis by inhibiting glycolysis and stimulating lipogenesis or gluconeogenesis, along with managing excessive production of cholesterol and conferring anti-carcinogenic action (Pascale et al., Reference Pascale, Marchesi, Marelli, Coppola, Luzi, Govoni, Giustina and Gazzaruso2018). Further studies are needed to understand the impact of the different compounds produced by gut microbiome in the metabolism of both mother and offspring, and elucidate which microorganisms are responsible for these changes.
Modification of gut microbiota during pregnancy
Pregnancy imposes a great number of physiological adaptations. Currently, there is no specific definition of healthy gut microbiota; however, it is known that its composition is highly diverse in healthy individuals (Meijnikman et al., Reference Meijnikman, Gerdes, Nieuwdorp and Herrema2018). Studies that explored the gut microbiota composition of healthy pregnant women found some important changes associated with an increase in maternal body weight by fat deposition and new dietary habits that culminate in a progressive increase in the food intake, essential for the foetus growth (Reference di Simone, Santamaria Ortiz, Specchia, Tersigni, Villa, Gasbarrini, Scambia and D’Ippolitodi Simone et al., 2020). Physiological changes are also part of a normal pregnancy, with maternal tissues becoming increasingly resistant to insulin in approximately 50–60% of women with normal glucose tolerance or with GDM (Kampmann et al., Reference Kampmann, Knorr, Fuglsang and Ovesen2019). In addition to weight gain and adiposity, pregnant women have significantly higher leptin, insulin and IR, cholesterol, and glycated haemoglobin levels as compared with nonpregnant women (Collado et al., Reference Collado, Isolauri, Laitinen and Salminen2008). In the pregnancy first trimester, the gut microbiota is expected to be similar to that of a healthy nonpregnant woman, classified into different enterotypes, considering three different groups of bacteria: one enterotype characterised by the presence of Bacteroides; another enterotype by higher proportion of Prevotella; and a third enterotype dominated by Ruminococcus (di Simone et al., Reference di Simone, Santamaria Ortiz, Specchia, Tersigni, Villa, Gasbarrini, Scambia and D’Ippolito2020). Between the first and the third trimester, gut microbiota changes substantially decreasing its diversity, with increased proportions of Proteobacteria, commonly associated with inflammation, while the number of butyrate-producing bacteria with anti-inflammatory effects decreases (Koren et al., Reference Koren, Goodrich, Cullender, Spor, Laitinen, Kling Bäckhed, Gonzalez, Werner, Angenent, Knight, Bäckhed, Isolauri, Salminen and Ley2012).
Studies comparing the intestinal microbiota between healthy-weight pregnant women and overweight and/or obese pregnant women were also considered. Gomez-Arango et al. (Reference Gomez-Arango, Barrett, McIntyre, Callaway, Morrison and Dekker Nitert2016) indicate that the ratio between phyla Firmicutes and Bacteroidetes was approximately 3:1 when analysing stool samples of both overweight and obese pregnant women, showing a slightly higher abundance of Firmicutes in obese women. These results were linked with the higher expression of enzymes engaged in the digestion of polysaccharide, highlighting that more energy can be obtained from the same diet (Cani, Reference Cani2013). Some correlations between specific taxa and pregnancy variables were observed. Lachnospiraceae and Ruminococcaceae families (both from Firmicutes phyla) were strongly correlated with leptin and positively associated with BMI. Bacteroidaceae relates with ghrelin that, in turn, was negatively associated with BMI, and positively correlated with Rikenellaceae (both from Bacteroidetes phyla). Collinsella positively correlated with insulin levels and triglycerides while Coprococcus (butyrate producer) correlated with gastric-inhibitory polypeptide (GIP), an incretin hormone (Gomez-Arango et al., Reference Gomez-Arango, Barrett, McIntyre, Callaway, Morrison and Dekker Nitert2016). When gestational weight gain (GWG) is excessive, pregnant women have an increased risk of developing GDM, obesity, metabolic syndrome (Carreno et al., Reference Carreno, Clifton, Hauth, Myatt, Roberts, Spong, Varner, Thorp, Mercer, Peaceman, Ramin, Carpenter, Sciscione, Tolosa, Saade and Sorokin2012; Gilmore et al., Reference Gilmore, Klempel-Donchenko and Redman2015) and delivering a baby larger-for-gestational age (Carreno et al., Reference Carreno, Clifton, Hauth, Myatt, Roberts, Spong, Varner, Thorp, Mercer, Peaceman, Ramin, Carpenter, Sciscione, Tolosa, Saade and Sorokin2012; Ferraro et al., Reference Ferraro, Barrowman, Prud’homme, Walker, Wen, Rodger and Adamo2012; Kim et al., Reference Kim, Sharma, Sappenfield, Wilson and Salihu2014). In this situation, gut microbiota is associated with lower α-diversity (DiGiulio et al., Reference DiGiulio, Callahan, McMurdie, Costello, Lyell, Robaczewska, Sun, Goltsman, Wong, Shaw, Stevenson, Holmes and Relman2015), and the presence of Eisenbergiella, Lactobacillus (Crusell et al., Reference Crusell, Hansen, Nielsen, Allin, Rühlemann, Damm, Vestergaard, Rørbye, Jørgensen, Christiansen, Heinsen, Franke, Hansen, Lauenborg and Pedersen2018), Blautia, Ruminoccocus, and Feacalibacterium (Stanislawski et al., Reference Stanislawski, Dabelea, Wagner, Sontag, Lozupone and Eggesbø2017) genus and Escherichia coli (Santacruz et al., Reference Santacruz, Collado, García-Valdés, Segura, Martín-Lagos, Anjos, Martí-Romero, Lopez, Florido, Campoy and Sanz2010) species. On the other hand, Bifidobacterium and Akkermansia muciniphila (Collado et al., Reference Collado, Isolauri, Laitinen and Salminen2008; Santacruz et al., Reference Santacruz, Collado, García-Valdés, Segura, Martín-Lagos, Anjos, Martí-Romero, Lopez, Florido, Campoy and Sanz2010), along with Christensenella and Alistipes (Crusell et al., Reference Crusell, Hansen, Nielsen, Allin, Rühlemann, Damm, Vestergaard, Rørbye, Jørgensen, Christiansen, Heinsen, Franke, Hansen, Lauenborg and Pedersen2018), are associated with the opposite trend. Additionally, overweight and obese pregnant women were reported to have higher levels of Bacteroides (Collado et al., Reference Collado, Isolauri, Laitinen and Salminen2008), Staphylococcus (Collado et al., Reference Collado, Isolauri, Laitinen and Salminen2008; Santacruz et al., Reference Santacruz, Collado, García-Valdés, Segura, Martín-Lagos, Anjos, Martí-Romero, Lopez, Florido, Campoy and Sanz2010) as well as Enterobacteriaceae and E. coli (Santacruz et al., Reference Santacruz, Collado, García-Valdés, Segura, Martín-Lagos, Anjos, Martí-Romero, Lopez, Florido, Campoy and Sanz2010).
Gut microbiota modifications during GDM pregnancy
Gut microbiota is involved in human metabolism regulation but can also contribute to the pathogenesis of many diseases, including GDM (Chwalba and Otto-Buczkowska, Reference Chwalba and Otto-Buczkowska2017; Ponzo et al., Reference Ponzo, Fedele, Goitre, Leone, Lezo, Monzeglio, Finocchiaro, Ghigo and Bo2019a,Reference Ponzo, Ferrocino, Zarovska, Amenta, Leone, Monzeglio, Rosato, Pellegrini, Gambino, Cassader, Ghigo, Cocolin and Bob). During gestation, adjustments in the women’s glucose metabolism occur to ensure proper glucose levels to warrant foetal growth and development combined with appropriate maternal nutrition (Angueira et al., Reference Angueira, Ludvik, Reddy, Wicksteed, Lowe and Layden2015). In early gestation, fasting blood glucose (FBG) levels decrease, possibly due to dilution effects (caused by an increased plasma volume), increased glucose uptake by the placenta (Lain and Catalano, Reference Lain and Catalano2007; Angueira et al., Reference Angueira, Ludvik, Reddy, Wicksteed, Lowe and Layden2015), and inadequate hepatic glucose production (Lain and Catalano, Reference Lain and Catalano2007). These levels remain constant in the second trimester and increase during the last trimester (Angueira et al., Reference Angueira, Ludvik, Reddy, Wicksteed, Lowe and Layden2015). In a regular pregnancy’s last trimester, maternal insulin sensitivity declines, which is considered to be advantageous to support foetal development with increased energy requirements at this stage (Koren et al., Reference Koren, Goodrich, Cullender, Spor, Laitinen, Kling Bäckhed, Gonzalez, Werner, Angenent, Knight, Bäckhed, Isolauri, Salminen and Ley2012). In a severe GDM pregnancy, insulin decreases an additional 40% (relatively to a healthy gestation), leading to glucose intolerance (Lain and Catalano, Reference Lain and Catalano2007). To balance these alterations, and due to a decreased capacity of insulin to suppress lipolysis, an increase in free fatty acids, hepatic gluconeogenesis, and severe IR occurs (Taddei et al., Reference Taddei, Cortez, Mattar, Torloni and Daher2018).
In recent years, more information on the correlation between GDM and gut microbiota has become available, demonstrating a distinct microbiota profile associated with this pathology (Kuang et al., Reference Kuang, Lu, Li, Li, Yuan, He, Chen, Xiao, Shen, Qiu, Wu, Hu, Wu, Li, Chen, Deng, Papasian, Xia and Qiu2017; Mokkala et al., Reference Mokkala, Houttu, Vahlberg, Munukka, Rönnemaa and Laitinen2017; Crusell et al., Reference Crusell, Hansen, Nielsen, Allin, Rühlemann, Damm, Vestergaard, Rørbye, Jørgensen, Christiansen, Heinsen, Franke, Hansen, Lauenborg and Pedersen2018; Ferrocino et al., Reference Ferrocino, Ponzo, Gambino, Zarovska, Leone, Monzeglio, Goitre, Rosato, Romano, Grassi, Broglio, Cassader, Cocolin and Bo2018; Hasan et al., Reference Hasan, Aho, Pereira, Paulin, Koivusalo, Auvinen and Eriksson2018; Cortez et al., Reference Cortez, Taddei, Sparvoli, Ângelo, Padilha, Mattar and Daher2019; Liu et al., Reference Liu, Pan, Lv, Yang, Zhang, Chen, Lv and Sun2015; Ye et al., Reference Ye, Zhang, Wang, Chen, Gu, Wang, Leng, Gu and Xie2019; Ma et al., Reference Ma, You, Huang, Long, Zhang, Guo, Zhang, Wu, Xiao and Tan2020; Zheng et al., Reference Zheng, Xu, Huang, Yan, Chen, Zhang, Tian, Liu, Yuan, Liu, Luo, Guo, Song, Zhang, Liang, Qin and Li2020). At a genus level, increased abundance of Klebsiella (Kuang et al., Reference Kuang, Lu, Li, Li, Yuan, He, Chen, Xiao, Shen, Qiu, Wu, Hu, Wu, Li, Chen, Deng, Papasian, Xia and Qiu2017), Collinsella (Collado et al., Reference Collado, Isolauri, Laitinen and Salminen2008; Santacruz et al., Reference Santacruz, Collado, García-Valdés, Segura, Martín-Lagos, Anjos, Martí-Romero, Lopez, Florido, Campoy and Sanz2010; Carreno et al., Reference Carreno, Clifton, Hauth, Myatt, Roberts, Spong, Varner, Thorp, Mercer, Peaceman, Ramin, Carpenter, Sciscione, Tolosa, Saade and Sorokin2012; Koren et al., Reference Koren, Goodrich, Cullender, Spor, Laitinen, Kling Bäckhed, Gonzalez, Werner, Angenent, Knight, Bäckhed, Isolauri, Salminen and Ley2012; Jost et al., Reference Jost, Lacroix, Braegger and Chassard2014; Kim et al., Reference Kim, Sharma, Sappenfield, Wilson and Salihu2014; Priyadarshini et al., Reference Priyadarshini, Thomas, Reisetter, Scholtens, Wolever, Josefson and Layden2014; DiGiulio et al., Reference DiGiulio, Callahan, McMurdie, Costello, Lyell, Robaczewska, Sun, Goltsman, Wong, Shaw, Stevenson, Holmes and Relman2015; Gomez-Arango et al., Reference Gomez-Arango, Barrett, McIntyre, Callaway, Morrison and Dekker Nitert2016; Stanislawski et al., Reference Stanislawski, Dabelea, Wagner, Sontag, Lozupone and Eggesbø2017; Aatsinki et al., Reference Aatsinki, Uusitupa, Munukka, Pesonen, Rintala, Pietilä, Lahti, Eerola, Karlsson and Karlsson2018; Crusell et al., Reference Crusell, Hansen, Nielsen, Allin, Rühlemann, Damm, Vestergaard, Rørbye, Jørgensen, Christiansen, Heinsen, Franke, Hansen, Lauenborg and Pedersen2018; Ferrocino et al., Reference Ferrocino, Ponzo, Gambino, Zarovska, Leone, Monzeglio, Goitre, Rosato, Romano, Grassi, Broglio, Cassader, Cocolin and Bo2018; Meijnikman et al., Reference Meijnikman, Gerdes, Nieuwdorp and Herrema2018; Smid et al., Reference Smid, Ricks, Panzer, Mccoy, Azcarate-Peril, Keku and Boggess2018; Crusell et al., Reference Crusell, Hansen, Nielsen, Allin, Rühlemann, Damm, Vestergaard, Rørbye, Jørgensen, Christiansen, Heinsen, Franke, Hansen, Lauenborg and Pedersen2018), Rothia (Angueira et al., Reference Angueira, Ludvik, Reddy, Wicksteed, Lowe and Layden2015; Chwalba and Otto-Buczkowska, Reference Chwalba and Otto-Buczkowska2017; Mokkala et al., Reference Mokkala, Houttu, Vahlberg, Munukka, Rönnemaa and Laitinen2017; Crusell et al., Reference Crusell, Hansen, Nielsen, Allin, Rühlemann, Damm, Vestergaard, Rørbye, Jørgensen, Christiansen, Heinsen, Franke, Hansen, Lauenborg and Pedersen2018; Taddei et al., Reference Taddei, Cortez, Mattar, Torloni and Daher2018; Liu et al., Reference Liu, Pan, Lv, Yang, Zhang, Chen, Lv and Sun2015; Ponzo et al., Reference Ponzo, Fedele, Goitre, Leone, Lezo, Monzeglio, Finocchiaro, Ghigo and Bo2019a,Reference Ponzo, Ferrocino, Zarovska, Amenta, Leone, Monzeglio, Rosato, Pellegrini, Gambino, Cassader, Ghigo, Cocolin and Bob; Zheng et al., Reference Zheng, Xu, Huang, Yan, Chen, Zhang, Tian, Liu, Yuan, Liu, Luo, Guo, Song, Zhang, Liang, Qin and Li2020), Eubacterium (Kuang et al., Reference Kuang, Lu, Li, Li, Yuan, He, Chen, Xiao, Shen, Qiu, Wu, Hu, Wu, Li, Chen, Deng, Papasian, Xia and Qiu2017), Ruminococcus (Cortez et al., Reference Cortez, Taddei, Sparvoli, Ângelo, Padilha, Mattar and Daher2019), Blautia (Crusell et al., Reference Crusell, Hansen, Nielsen, Allin, Rühlemann, Damm, Vestergaard, Rørbye, Jørgensen, Christiansen, Heinsen, Franke, Hansen, Lauenborg and Pedersen2018; Ye et al., Reference Ye, Zhang, Wang, Chen, Gu, Wang, Leng, Gu and Xie2019; Zheng et al., Reference Zheng, Xu, Huang, Yan, Chen, Zhang, Tian, Liu, Yuan, Liu, Luo, Guo, Song, Zhang, Liang, Qin and Li2020), Prevotella (Cortez et al., Reference Cortez, Taddei, Sparvoli, Ângelo, Padilha, Mattar and Daher2019; Zheng et al., Reference Zheng, Xu, Huang, Yan, Chen, Zhang, Tian, Liu, Yuan, Liu, Luo, Guo, Song, Zhang, Liang, Qin and Li2020), Parabacteroides (Kuang et al., Reference Kuang, Lu, Li, Li, Yuan, He, Chen, Xiao, Shen, Qiu, Wu, Hu, Wu, Li, Chen, Deng, Papasian, Xia and Qiu2017; Dong et al., Reference Dong, Han, Duan, Lin, Li and Liu2020), Eisenbergiella and Tyzzerella (Ma et al., Reference Ma, You, Huang, Long, Zhang, Guo, Zhang, Wu, Xiao and Tan2020), and a reduced richness in Akkermansia (Cortez et al., Reference Cortez, Taddei, Sparvoli, Ângelo, Padilha, Mattar and Daher2019), Marvinbryantia, Acetivibrio, Anaerosporobacter (Jost et al., Reference Jost, Lacroix, Braegger and Chassard2014), and Roseburia (Kuang et al., Reference Kuang, Lu, Li, Li, Yuan, He, Chen, Xiao, Shen, Qiu, Wu, Hu, Wu, Li, Chen, Deng, Papasian, Xia and Qiu2017; Cortez et al., Reference Cortez, Taddei, Sparvoli, Ângelo, Padilha, Mattar and Daher2019) were described in patients with GDM compared with normoglycemic women (Figure 1). Mokkala et al. (Reference Mokkala, Houttu, Vahlberg, Munukka, Rönnemaa and Laitinen2017) reported changes in the gut microbiota before GDM diagnosis, indicating that higher abundance of Ruminococcaceae in the intestine, a family of bacteria important for starch digestion in the large intestine and production of SCFAs (Oriá et al., Reference Oriá, Empadinhas and Malva2020), may be associated with an increased probability to develop GDM (Mokkala et al., Reference Mokkala, Houttu, Vahlberg, Munukka, Rönnemaa and Laitinen2017).
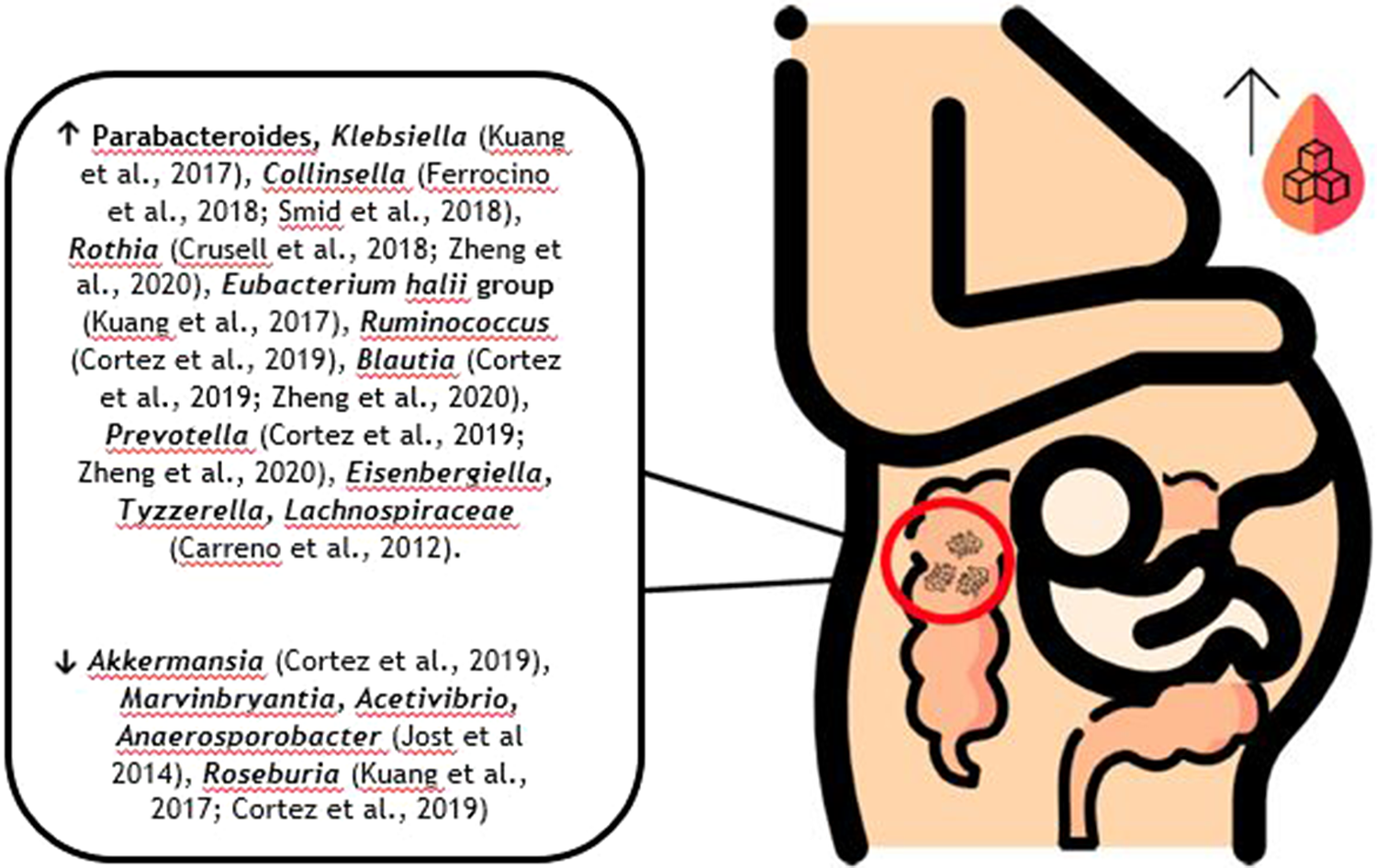
Figure 1. Possible composition of the gut microbiota in pregnant women with GDM according to the available literature.
There is evidence suggesting associations between some bacterial taxa and GDM indicators. Desulfovibrio, reported as a GDM biomarker (Crusell et al., Reference Crusell, Hansen, Nielsen, Allin, Rühlemann, Damm, Vestergaard, Rørbye, Jørgensen, Christiansen, Heinsen, Franke, Hansen, Lauenborg and Pedersen2018), is also a known lipopolysaccharide (LPS) producer, which is one of the strongest inducers of inflammation (Cani et al., Reference Cani, Osto, Geurts and Everard2012), associated with IR (Kim et al., Reference Kim, Keogh and Clifton2018) and leading to intestinal barrier damage (Sanchez-Alcoholado et al., Reference Sanchez-Alcoholado, Castellano-Castillo, Jordán-Martínez, Moreno-Indias, Cardila-Cruz, Elena, Muñoz-Garcia, Queipo-Ortuño and Jimenez-Navarro2017; Zhang et al., Reference Zhang, Han, Chen, Li, Silva-Zolezzi, Parés, Wang and Qin2018a). Collinsella has been related to higher scores in the Homeostasis Model Assessment for Insulin Resistance (HOMA-IR) scale and insulin levels (Ferrocino et al., Reference Ferrocino, Ponzo, Gambino, Zarovska, Leone, Monzeglio, Goitre, Rosato, Romano, Grassi, Broglio, Cassader, Cocolin and Bo2018). Blautia was associated with glucose intolerance (Egshatyan et al., Reference Egshatyan, Kashtanova, Popenko, Tkacheva, Tyakht, Alexeev, Karamnova, Kostryukova, Babenko, Vakhitova and Boytsov2016) and unfavourable metabolic profile in high BMI patients (Crusell et al., Reference Crusell, Hansen, Nielsen, Allin, Rühlemann, Damm, Vestergaard, Rørbye, Jørgensen, Christiansen, Heinsen, Franke, Hansen, Lauenborg and Pedersen2018; Ye et al., Reference Ye, Zhang, Wang, Chen, Gu, Wang, Leng, Gu and Xie2019). Prevotella was positively associated with increased LPS and gut inflammation mediated by pro-inflammatory cytokines (Alves et al., Reference de Brito Alves, de Oliveira, Carvalho, Cavalcante, Pereira Lira, Nascimento, Magnani, Vidal, Braga and de Souza2019).
Although such associations have been established, subjacent mechanisms concerning host and microbiota interactions remain unidentified (Angueira et al., Reference Angueira, Ludvik, Reddy, Wicksteed, Lowe and Layden2015). Also, there are some controversial results since Megasphera and Eggerthella were reported to be enriched in normoglycaemic controls in some studies (Stanislawski et al., Reference Stanislawski, Dabelea, Wagner, Sontag, Lozupone and Eggesbø2017; Wen and Duffy, Reference Wen and Duffy2017) while in others both genera were described to be enriched in the GDM group (Zheng et al., Reference Zheng, Xu, Huang, Yan, Chen, Zhang, Tian, Liu, Yuan, Liu, Luo, Guo, Song, Zhang, Liang, Qin and Li2020). Additionally, at a phylum level, Actinobacteria was found simultaneously to be increased (Crusell et al., Reference Crusell, Hansen, Nielsen, Allin, Rühlemann, Damm, Vestergaard, Rørbye, Jørgensen, Christiansen, Heinsen, Franke, Hansen, Lauenborg and Pedersen2018) and decreased in GDM cohorts (DiGiulio et al., Reference DiGiulio, Callahan, McMurdie, Costello, Lyell, Robaczewska, Sun, Goltsman, Wong, Shaw, Stevenson, Holmes and Relman2015; Cortez et al., Reference Cortez, Taddei, Sparvoli, Ângelo, Padilha, Mattar and Daher2019).
In an attempt to outline available information in a more visual format, Figure 1 presents the possible composition of a pregnant woman with GDM gut microbiota, mainly at the genus level, separating those currently described to be increased from those currently described as being decreased in these patients.
Finally, GDM mother’s offspring gut microbiota have differences in α-richness compared with neonates of mothers without GDM, with a higher abundance of Actinobacteria, associated with higher levels of fasting glucose, and reduction in Bacteroides abundance. At a genus level, an increase of opportunistic pathogens, such as Escherichia and Parabacteroides, and a decrease in probiotic Lactobacillus were observed in GDM mother’s offspring (Su et al., Reference Su, Nie, Shao, Duan, Jiang, Wang, Xing, Sun, Liu and Xu2018; Ponzo et al., Reference Ponzo, Ferrocino, Zarovska, Amenta, Leone, Monzeglio, Rosato, Pellegrini, Gambino, Cassader, Ghigo, Cocolin and Bo2019b). Therefore, the influence of the mother’s gut microbiota composition, blood glucose, BMI, and dietary intake on the gut microbiota of their offspring needs to be further investigated.
Interactions between diet and gut microbiota during pregnancy
The microbial community living in the gut highly depends on the host’s diet, one of the most significant contributors to the modulation of intestinal microbiota and human health (Hasan and Yang, Reference Hasan and Yang2019). Among nutrients, the effects of complex carbohydrates (CHO) are the best studied, having an important impact on microbiota composition (Gentile and Weir, Reference Gentile and Weir2018). It has been reported that diets rich in CHO can modify the gut microbial composition in only a few days or weeks, because in the large intestine there is an intensive fermentative activity of resistant starch and fibres, creating a set of metabolites produced by bacteria – the human metabolome – that can be detected in the host faeces, urine, and blood that can pass the intestinal barrier (Flint et al., Reference Flint, Duncan, Scott and Louis2015). Dietary fibre is the energy source for commensal SCFA-producing bacteria and its fermentation has the potential to decrease postprandial blood glucose, insulin responses and, decrease cholesterol absorption. Dietary patterns marked by low-fibre intake inhibit the growth of SCFA-producing bacteria and enable the development of other bacterial strains that use glycoproteins as energy, leading to harmful effects on the gut barrier (Gomez-Arango et al., Reference Gomez-Arango, Barrett, Wilkinson, Callaway, McIntyre, Morrison and Dekker Nitert2017). A different study also showed that, in early pregnancy, diets associated with higher ingestion of fibres, like vegetarian diets, resulted in a diminished abundance of Collinsella (lactate producing bacteria, instead of SCFA, associated with IR), as well as an increased richness of Roseburia (a butyrate producing bacteria) when compared with the gut microbiota of early pregnant women with omnivore diets (Barret et al., Reference Barrett, Gomez-Arango, Wilkinson, McIntyre, Callaway, Morrison and Dekker Nitert2018). Additionally, Prevotella was found to be enriched in maternal microbiota related to diets with higher CHO intake, whereas Ruminococcus was more enriched in the cohorts ingesting vegetal protein and fat diets (García-Mantrana et al., Reference García-Mantrana, Selma-Royo, González, Parra-Llorca, Martínez-Costa and Collado2020). Furthermore, in overweight and obese pregnancies higher fibre intake increased intestinal microbiota richness, while greater fat intake (saturated fatty acids, monounsaturated fatty acids, and n-6 polyunsaturated fatty acids) decreased its richness (Röytiö et al., Reference Röytiö, Mokkala, Vahlberg and Laitinen2017).
Current literature also reports the association between pro-inflammatory bacteria and dietary patterns. Proteobacteria were associated with a diet abundant in vitamin D, retinol, and mono-unsaturated fat in normal-weight pregnant women, whereas vitamin E was associated with the opposite trend (Mandal et al., Reference Mandal, Godfrey, McDonald, Treuren, Bjørnholt, Midtvedt, Moen, Rudi, Knight, Brantsæter, Peddada and Eggesbø2016). One study implemented routine dietary counselling according to International Federation of Gynecology and Obstetrics (FIGO) guidelines (Hod et al., Reference Hod, Kapur, Sacks, Hadar, Agarwal, di Renzo, Roura, McIntyre, Morris and Divakar2015) to a cohort of 41 overweight pregnant women with GDM, where participants completed a 3-day food record and it was found that only one-third accomplished the recommendations, with two-third consuming a diet rich in fat and low in fibre (Ferrocino et al., Reference Ferrocino, Ponzo, Gambino, Zarovska, Leone, Monzeglio, Goitre, Rosato, Romano, Grassi, Broglio, Cassader, Cocolin and Bo2018). Adherent participants, whose diet was poor in saturated and total fats, showed a decreased abundance of Bacteroides in their gut microbiota composition, which is associated with high-fat animal-based diets (David et al., Reference David, Maurice, Carmody, Gootenberg, Button, Wolfe, Ling, Devlin, Varma, Fischbach, Biddinger, Dutton and Turnbaugh2014). In conclusion, food and its constituents are crucial in regulating host health and disease since they can modulate the environment in which gut microbes live, as well as their diversity and metabolism.
Medical nutrition therapy in GDM
Most GDM women are diagnosed between 24 and 28 weeks of pregnancy, when there is already an increased maternal IR that tends to be higher each week (Farabi and Hernandez, Reference Farabi and Hernandez2019). Medical nutrition therapy (MNT), defined as an individualised nutritional plan developed between the woman and the dietitian (American Diabetes Association Professional Practice Committee, 2022), can be an effective first-line therapy to treat GDM. It should be a food plan that provides adequate calories for both mother and foetus, based on nutrition assessment and the control of the amount and distribution of CHO in the diet, in order to achieve adequate nourishment and normoglycaemia without ketosis and also to improve glycaemic control (Reader, Reference Reader2007; Moreno-Castilla et al., Reference Moreno-Castilla, Hernandez, Bergua, Alvarez, Arce, Rodriguez, Martinez-Alonso, Iglesias, Mateu, Santos, Pacheco, Blasco, Martin, Balsells, Aranda and Mauricio2013). For a successful treatment, individual and personalised dietary counselling and prescription should be assumed by a registered dietitian (or universal equivalent) for all pregnant women diagnosed with GDM (Duarte-Gardea et al., Reference Duarte-Gardea, Gonzales-Pacheco, Reader, Thomas, Wang, Gregory, Piemonte, Thompson and Moloney2018).
The major focus of MNT is to lower postprandial plasma glucose levels, either by adjusting CHO distribution or by altering the glycaemic load (GL; Hernandez et al., Reference Hernandez, Anderson, Chartier-Logan, Friedman and Barbour2013). The first steps of diet manipulation were taken during the 1950s and 60s, focusing on CHO restriction, with approximately 40% of total daily calories (Hernandez, Reference Hernandez2016), considering the principle that it could help lower postprandial glucose and prevent foetal hyperinsulinemia (Mulla, Reference Mulla2016). Currently, it is recommended by the Institute of Medicine (United States of America – USA) a minimum of 175 g CHO/day for pregnant women, equivalent to 35% of a 2000 calories diet, with an extra 45 g compared with non-pregnant women, since an average of 33g glucose/day are required to support foetal brain development and functioning (Trumbo et al., Reference Trumbo, Schlicker, Yates and Poos2002), and also 71 g of protein and 28 g of fibre. Low-CHO diets remain the conventional diet therapy for GDM in some countries. Hence, more studies are needed to construct solid evidence about CHO restriction diets (Trumbo et al., Reference Trumbo, Schlicker, Yates and Poos2002; Moreno-Castilla et al., Reference Moreno-Castilla, Mauricio and Hernandez2016; Mulla, Reference Mulla2016). Moderation seems to be key, since proportions greater than 55% CHO are associated with increased postprandial plasma glucose (Filardi et al., Reference Filardi, Panimolle, Crescioli, Lenzi and Morano2019).
Considering CHO, part is categorised as complex, since their structure is resistant to digestion or even completely undigested, resulting in a slower rise in blood glucose, low-GI (Mustad et al., Reference Mustad, Huynh, López-Pedrosa, Campoy and Rueda2020). Worldwide, 10 clinical practice guidelines (in a total of 16 analysed) on CHO considerations for GDM recommend a low-GI diet (foods under 55 on the GI scale; Tsirou et al., Reference Tsirou, Grammatikopoulou, Theodoridis, Gkiouras, Petalidou, Taousani, Savvaki, Tsapas and Goulis2019), as it can reduce maternal FBG and 2-hour postprandial glucose, compared with high-GI diet. Viana et al. (Reference Viana, Gross and Azevedo2014) systematically reviewed various dietary patterns and concluded that a low-GI diet was the only one confirmed as beneficial for GDM women. Moreover, this diet may also have beneficial effects on the offspring by significantly reducing FBG, postprandial glucose levels, insulin use, and risk of macrosomia (Zhang et al., Reference Zhang, Yu, Xiao, Hu, Xin and Yu2018b; Xu and Ye, Reference Xu and Ye2020). Different types of CHO have diverse impacts on gut microbiota composition. Mardinoglu et al. (Reference Mardinoglu, Wu, Bjornson, Zhang, Hakkarainen, Räsänen, Lee, Mancina, Bergentall, Pietiläinen, Söderlund, Matikainen, Ståhlman, Bergh, Adiels, Piening, Granér, Lundbom, Williams, Romeo, Nielsen, Snyder, Uhlén, Bergström, Perkins, Marschall, Bäckhed, Taskinen and Borén2018) conducted a short-term intervention study on 10 obese individuals with non-alcoholic fat disease, in order to understand the gut microbial composition, by applying a restricted-CHO diet with increased protein for 14 days. The results show a reduction of CHO-degrading bacteria Ruminococcus, Eubacterium, Clostridium, and Bifidobacterium, and levels of SCFA, along with an increased richness in Lactococcus, Eggerthella, and Streptococcus (Mardinoglu et al., Reference Mardinoglu, Wu, Bjornson, Zhang, Hakkarainen, Räsänen, Lee, Mancina, Bergentall, Pietiläinen, Söderlund, Matikainen, Ståhlman, Bergh, Adiels, Piening, Granér, Lundbom, Williams, Romeo, Nielsen, Snyder, Uhlén, Bergström, Perkins, Marschall, Bäckhed, Taskinen and Borén2018). Another study examined the effects of a CHO-restricted diet on the gut bacteria in mice, showing a significant increase in Clostridium (bacteria that promote inflammation) and Suterella (associated with increased LPS and gut inflammation; He et al., Reference He, Wu, Hayashi, Nakano, Nakatsukasa and Tsuduki2020).
Regarding fibre intake, a study about the effects of high fibre with low-GI on gut microbiota in type 2 diabetes patients reported a decreased richness in E. coli and Enterococcus (opportunistic pathogens) and a significant increase in Bifidobacterium and Lactobacillus (beneficial bacteria) compared with the control group (Singh et al., Reference Singh, Chang, Yan, Lee, Ucmak, Wong, Abrouk, Farahnik, Nakamura, Zhu, Bhutani and Liao2017). Bifidobacterium is solidly associated with SCFA production – produced by the fermentation of microbiota-accessible carbohydrates (MACs) – decreased gut LPS levels and improved intestinal mucosal barrier. Lactobacillus is associated with anti-inflammatory and anti-carcinogenic effects, and also SCFA production (Singh et al., Reference Singh, Chang, Yan, Lee, Ucmak, Wong, Abrouk, Farahnik, Nakamura, Zhu, Bhutani and Liao2017). Additionally, the richness of Lactobacillus and Bifidobacterium are associated with the intake of prebiotic fibres (Moszak et al., Reference Moszak, Szulińska and Bogdański2020). These are indigestible fermented fibres that promote bacterial growth in the intestine with health benefits for the host, such as fructooligosaccharides (FOS), galactooligosaccharides (GOS), and inulin (Wilson and Whelan, Reference Wilson and Whelan2017). The majority of GDM guidelines recommend an augmented quantity of fibre, with some indicating approximately 28 g/day of fibres intake (Tsirou et al., Reference Tsirou, Grammatikopoulou, Theodoridis, Gkiouras, Petalidou, Taousani, Savvaki, Tsapas and Goulis2019).
DASH diet is another dietary pattern that has been studied for patients with GDM. Characterised by low-GI, low-energy density with high quantities of fibre and decreased levels of sodium, it was originally designed for patients with hypertension, but favourable effects were reported for metabolic syndrome and type 2 diabetes (Asemi et al., Reference Asemi, Samimi, Tabassi, Sabihi and Esmaillzadeh2013a,Reference Asemi, Tabassi, Samimi, Fahiminejad and Esmaillzadehb). The American Academy of Nutrition and Dietetics (USA) also considers this dietary pattern to be effective in improving both mother and foetal outcomes in GDM, including glucose tolerance, IR, glycosylated haemoglobin, insulin requirements, lipid profile and incidence of macrosomia (Duarte-Gardea et al., Reference Duarte-Gardea, Gonzales-Pacheco, Reader, Thomas, Wang, Gregory, Piemonte, Thompson and Moloney2018), HOMA-IR results and medication needs (Yamamoto et al., Reference Yamamoto, Kellett, Balsells, García-Patterson, Hadar, Solà, Gich, van der Beek, Castañeda-Gutiérrez, Heinonen, Hod, Laitinen, Olsen, Poston, Rueda, Rust, van Lieshout, Schelkle, Murphy and Corcoy2018), systolic blood pressure and lipid profiles – these last two reported on a 4-week DASH diet in patients with GDM (Asemi et al., Reference Asemi, Samimi, Tabassi, Sabihi and Esmaillzadeh2013a,Reference Asemi, Tabassi, Samimi, Fahiminejad and Esmaillzadehb). DASH shares numerous similarities with the Mediterranean diet, except for the intake of olive oil. Fruits, vegetables, and grains are the major food sources in the DASH pattern diet, containing different fibre types (Jama et al., Reference Jama, Beale, Shihata and Marques2019), which may predict its positive role in GDM nutrition, as discussed above. Although there is a lack of studies directly targeting the influence of a DASH diet on GDM women’s gut microbiota, it is already demonstrated that high adherence to DASH promotes the increased richness of SCFA-producing bacteria, such as Roseburia, whereas no adherence leads to higher urinary trimethylamine N-oxide (TMAO) levels, a microbial metabolite possibly associated with cardiovascular and neurological disorders (Filippis et al., Reference de Filippis, Pellegrini, Vannini, Jeffery, la Storia, Laghi, Serrazanetti, di Cagno, Ferrocino, Lazzi, Turroni, Cocolin, Brigidi, Neviani, Gobbetti, O’Toole and Ercolini2016). Further studies are required to better clarify the DASH diet role in gut microbiota modulation for GDM management.
Probiotics in GDM
Even though the adherence of the probiotics to the intestinal mucosal cells is challenging, which influences its effect (O’Sullivan et al., Reference O’Sullivan, Thornton, O’Sullivan and Collins1992; Kullen et al., Reference Kullen, Amann, O’Shaughnessy, O’Sullivan, Busta and Brady1997), regular use of probiotics is reported to beneficially modulate intestinal microbiota composition metabolic activities (Taylor et al., Reference Taylor, Woodfall, Sheedy, O’Riley, Rainbow, Bramwell and Kellow2017). Thus, maternal gut microbiota modulation with probiotic intervention is emerging as a safe approach capable of improving intestinal commensal bacteria and also providing beneficial effects for both mother and foetus health (Swartwout and Luo, Reference Swartwout and Luo2018; Hasain et al., Reference Hasain, Che Roos, Rahmat, Mustapa, Raja Ali and Mokhtar2021). Currently, probiotic agents are mainly produced by gram-positive bacteria such as lactic acid bacteria, namely Bifidobacterium and Lactobacillus. Its use, particularly L. casei, L. helvetica, L. acidophilus, and L. rhamnosus are associated with an improvement of some GDM biomarkers (Pereira et al., Reference de Melo Pereira, de Oliveira Coelho, Magalhães Júnior, Thomaz-Soccol and Soccol2018). Several other promising data suggest that probiotics may positively contribute to beneficial effects on glucose metabolism or even prevent GDM, either by probiotic-fortified foods or capsules (Dolatkhah et al., Reference Dolatkhah, Hajifaraji, Abbasalizadeh, Aghamohammadzadeh, Mehrabi and Mesgari Abbasi2015; Lindsay et al., Reference Lindsay, Brennan, Kennelly, Maguire, Smith, Curran, Coffey, Foley, Hatunic, Shanahan and McAuliffe2015; Ahmadi et al., Reference Ahmadi, Jamilian, Tajabadi-Ebrahimi, Jafari and Asemi2016; Jafarnejad et al., Reference Jafarnejad, Saremi, Jafarnejad and Arab2016; Karamali et al., Reference Karamali, Dadkhah, Sadrkhanlou, Jamilian, Ahmadi, Tajabadi-Ebrahimi, Jafari and Asemi2016; Wickens et al., Reference Wickens, Barthow, Murphy, Abels, Maude, Stone, Mitchell, Stanley, Purdie, Kang, Hood, Rowden, Barnes, Fitzharris and Crane2017; Badehnoosh et al., Reference Badehnoosh, Karamali, Zarrati, Jamilian, Bahmani, Tajabadi-Ebrahimi, Jafari, Rahmani and Asemi2018; Kijmanawat et al., Reference Kijmanawat, Panburana, Reutrakul and Tangshewinsirikul2019).
Seven studies assess the effect of probiotics on metabolic health in pregnant women with GDM (Table 1). Only one randomised control trial (RCT) demonstrated no impact on the metabolic health of GDM pregnant women, with the consumption of one single strain probiotic capsule, L. salivarius, in a dose of 1x10^9 CFU/day during 6 weeks. A double-blind, placebo-controlled study performed with 60 GDM pregnant women demonstrated that probiotic intervention with 3 strains, L. acidophilus, L. casei and B. bifidum, in a dose of 6x10^9 CFU/day during 6 weeks resulted in a significant decrease of FBG, insulin, HOMA-IR and HOMA for β cell function (HOMA-β) levels in the probiotic group compared with the placebo group. There were also positive results in the probiotic group concerning lipid metabolism, with considerable reductions in serum triglycerides and very low density lipoprotein (VLDL) cholesterol. Similar results were reported when administering a symbiotic supplement (probiotics plus inulin) with the same probiotic strains and doses used in Karamali et al. (Reference Karamali, Dadkhah, Sadrkhanlou, Jamilian, Ahmadi, Tajabadi-Ebrahimi, Jafari and Asemi2016) to GDM pregnant women, supplemented with 800 mg of inulin (a prebiotic fibre) during a 6-week-treatment, resulting in decreased insulin levels, and HOMA-IR and HOMA-β results, along with similar lipid outcomes when compared with placebo (Ahmadi et al., Reference Ahmadi, Jamilian, Tajabadi-Ebrahimi, Jafari and Asemi2016). Another RCT involving 70 pregnant women diagnosed with GDM showed that daily consumption of a probiotic capsule containing eight different strains, S. thermophilus, B. breve, B. longum, B. infantis, L. acidophilus, L. plantarum, L. paracasei and L. delbrueckii, in a dose of 15 × 10^9 CFU during 8 weeks, had significant differences in insulin levels and HOMA-IR; however, no changes were observed in FBG and HbA1c in the probiotic group compared with the placebo group (Jafarnejad et al., Reference Jafarnejad, Saremi, Jafarnejad and Arab2016). Data from a double-blind, placebo-controlled, and randomised study revealed that an 8-week treatment of probiotics in a 4 × 10^9 CFU/day dose with four probiotic strains, L. acidophilus, Bifidobacterium sp., S. thermophilus, and L. bulgaricus, showed reductions in FBG, HOMA-IR and GWG (Dolatkhah et al., Reference Dolatkhah, Hajifaraji, Abbasalizadeh, Aghamohammadzadeh, Mehrabi and Mesgari Abbasi2015). Moreover, a 4-week randomised double-blind and placebo-controlled study performed with a two-strain probiotic capsule containing L. acidophilus and B. bifidus in a 2 × 10^9 CFU dose in 57 GDM pregnant women, demonstrated significant improvements in glucose metabolism in the probiotic group compared with the placebo, comprising fasting plasma insulin, FBG, and HOMA-IR (Kijmanawat et al., Reference Kijmanawat, Panburana, Reutrakul and Tangshewinsirikul2019). Two other RCTs conducted with the same number of participants (n = 60, 30 in the probiotic group, 30 in placebo group) and the same three strains of probiotics, L. acidophilus, L. casei and L. bifidum, and doses (6 × 10^9 CFU/day) but with different treatment duration and type of participants, 6 weeks with GDM pregnant women (Badehnoosh et al., Reference Badehnoosh, Karamali, Zarrati, Jamilian, Bahmani, Tajabadi-Ebrahimi, Jafari, Rahmani and Asemi2018) and 12 weeks with healthy pregnant women (Ahmadi et al., Reference Ahmadi, Jamilian, Tajabadi-Ebrahimi, Jafari and Asemi2016), have both exhibited positive results in the probiotic group. In GDM pregnant women probiotic treatment significantly reduced FBG and other inflammatory biomarkers, total glutathione, high sensitivity C-reactive protein, malondialdehyde, and nitric oxide (Badehnoosh et al., Reference Badehnoosh, Karamali, Zarrati, Jamilian, Bahmani, Tajabadi-Ebrahimi, Jafari, Rahmani and Asemi2018), whereas in healthy pregnant women substantially diminished insulin levels, HOMA-IR and HOMA-β (Ahmadi et al., Reference Ahmadi, Jamilian, Tajabadi-Ebrahimi, Jafari and Asemi2016). Hasain et al. (Reference Hasain, Che Roos, Rahmat, Mustapa, Raja Ali and Mokhtar2021) conducted a meta-analysis of studies using multispecies probiotics, including Lactobacillus and Bifidobacterium, similarly to the previous studies presented. Authors reported significant reduction in different glycemic control biomarkers as FPG, fasting serum insulin, and HOMA-IR in women with GDM when taking a probiotic supplementation in a dose between 106 to 109 CFU. However no significant impact was observed when comparing the data for total cholesterol levels, which does not concur with other studies presented in this review. Further studies can help clarify this discrepancy.
Table 1. Studies of probiotics in GDM diagnosed women during gestation.
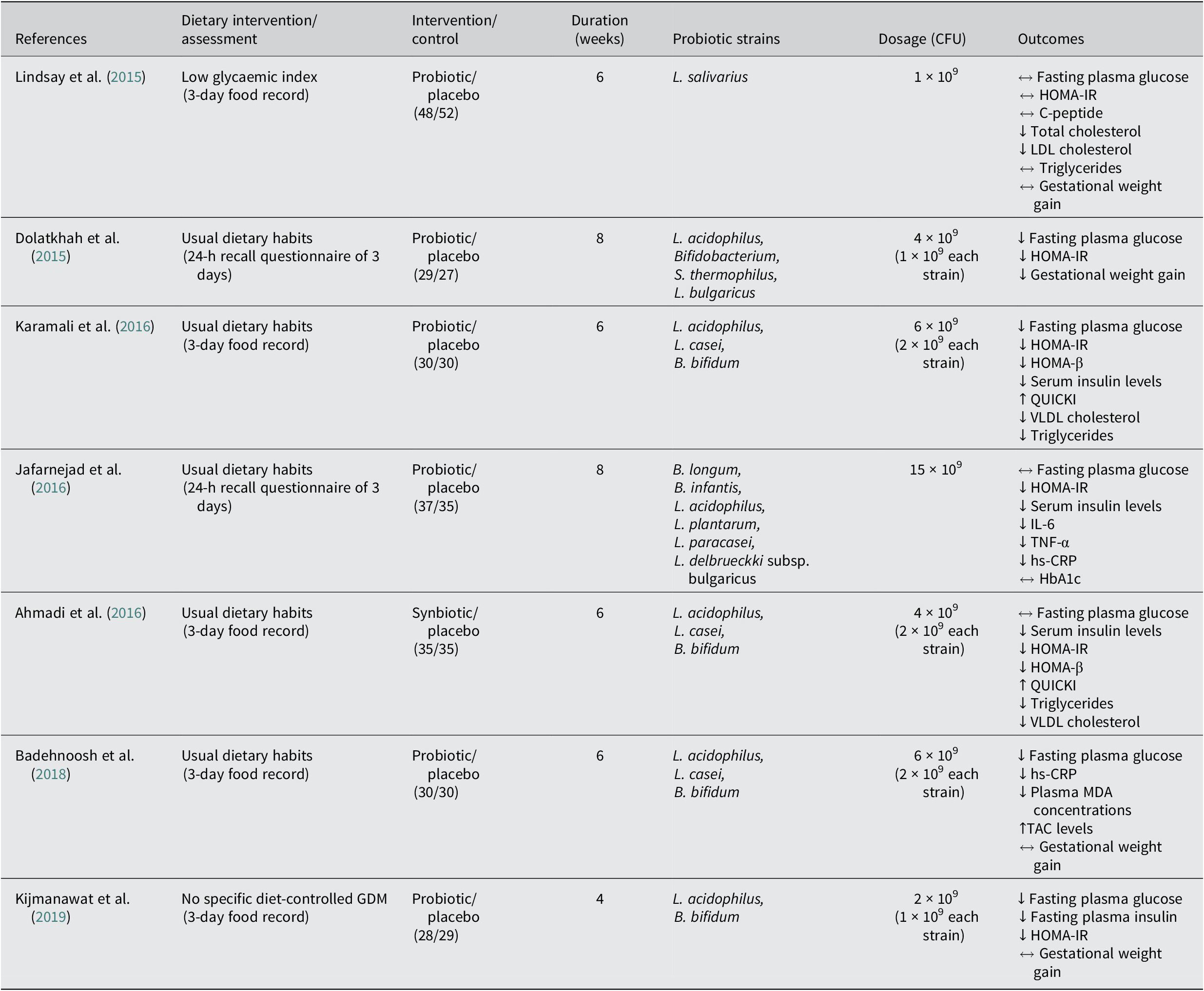
↔ No significant differences between probiotic and control groups; ↓significantly lower in the probiotic group compared with the control; ↑significantly higher in the probiotic group compared with the control.
HbA1c, glycosylated haemoglobin; HOMA-IR, homeostatic model of assessment of insulin resistance; HOMA-β, homeostatic model assessment for B-cell function; hs-CRP, high-sensitivity C-reactive protein; IL-6, interleukin 6; MDA, malondialdehyde; QUICKI, quantitative insulin sensitivity check index; TAC, total antioxidant capacity; TNF-α, tumour necrosis factor alpha; VLDL, very low density lipoprotein.
Current literature reveals that probiotic therapy may be an important non-pharmacological approach in terms of improving glycaemic control in pregnant women diagnosed with GDM. Probiotic mechanisms of action for treating diabetes mellitus are diverse and depend on different aspects (Khursheed et al., Reference Khursheed, Singh, Wadhwa, Kapoor, Gulati, Kumar, Ramanunny, Awasthi and Dua2019). Anti-diabetic effects emerge when the administration of probiotics is accomplished, homeostasis is recovered, along with diminished LPS levels, supporting the synthesis of different SCFA (butyrate, acetate, and propionate) on the intestine. This leads to an increased release of incretin hormones (like GLP-1), stimulating insulin secretion and delaying gastric emptying, which affects blood glucose levels and reduces intestinal permeability, by improving tight junction proteins, thus diminishing inflammation, oxidative stress, glucose intolerance, and IR (Sanchez-Alcoholado et al., Reference Sanchez-Alcoholado, Castellano-Castillo, Jordán-Martínez, Moreno-Indias, Cardila-Cruz, Elena, Muñoz-Garcia, Queipo-Ortuño and Jimenez-Navarro2017; Khursheed et al., Reference Khursheed, Singh, Wadhwa, Kapoor, Gulati, Kumar, Ramanunny, Awasthi and Dua2019).
However, additional studies are needed to clarify the underlying mechanisms of action through which probiotic therapy improve glycaemic control in GDM pregnant women and determine variables such as what strains, dosage and duration of probiotic treatment confer the highest benefits during gestation. Hsu et al. (Reference Hsu, Lin, Hou and Tain2018) reported the influence of maternal therapy with Lactobacillus casei probiotic and inulin prebiotic for hypertension treatment in rat’s offspring, showing a protective effect when a high fructose diet is administered during pregnancy and lactation. However, the mechanism behind the effect on the offspring is still not clear, as it may be due to a direct consequence of the probiotic passage through the milk or the placenta or the modification of the mother’s metabolism. Other studies evaluated the use of probiotics to manage offspring overweight. The meta-analysis developed by Wang et al. (Reference Wang, Tung, Chang, Lin and Chen2020) reveals the decrease of the newborn birth weight when women with GDM are treated with probiotics. On the other hand, the intake of probiotic by obese pregnant women has the opposite effect, increasing the newborn birth weight.
Discrepancies between studies are also present. Badehnoosh et al. (Reference Badehnoosh, Karamali, Zarrati, Jamilian, Bahmani, Tajabadi-Ebrahimi, Jafari, Rahmani and Asemi2018) and Karamali et al. (Reference Karamali, Nasiri, Taghavi Shavazi, Jamilian, Bahmani, Tajabadi-Ebrahimi and Asemi2018) observed a positive effect of 6 weeks probiotic therapy with L. acidophilus, L. casei, and B. bifidum on offspring birth weight from women with GDM, while Kijmanawat et al. (Reference Kijmanawat, Panburana, Reutrakul and Tangshewinsirikul2019) observed that supplementation with only L. acidophilus and B. bifidum during 4 weeks do not produce any effect on the infant weight. The success of the probiotic intake seems to be dependent on the type of probiotic strains used, being cocktails with higher diversity of microorganism more beneficial. The duration of treatment also appears to influence the effect of the treatment, with Wang et al. (Reference Wang, Tung, Chang, Lin and Chen2020) analysis indicating the need of at least 6 weeks to see effects.
Although proven safe and without adverse effects both for mother and offspring (Didari et al., Reference Didari, Solki, Mozaffari, Nikfar and Abdollahi2014), additional studies need to elucidate the modification not only in the mother’s metabolism and gut microbiome but also in the offspring. All of this should be taken in consideration when recommending probiotic treatment to pregnant women diagnosed with GDM.
Conclusion
Gut microbiota suffers alterations during healthy and pathological gestations like GDM. Modulating its composition through diet and probiotics can be a valid non-pharmacological preventive approach to reduce adverse GDM outcomes in both mother and offspring. Dietary management without probiotics, particularly with a low-GI approach, the currently most recommended diet for patients with GDM, showed benefits in reducing maternal FBG and 2-hour postprandial glucose, apart from being associated with beneficial gut bacteria, such as Bifidobacterium and Lactobacillus. As reviewed, probiotic supplements may ameliorate glycaemic control and inflammatory status of GDM pregnant women, demonstrating the ability to reduce fasting plasma glucose, IR, and improved lipid profiles. However, further high-quality studies are needed to verify the effectiveness of dietary interventions with probiotics as well as the definition of the bacterial strains, doses, and duration of treatment that have the best clinical significance for GDM pregnant women. Achieving this will securely increase the use of probiotic supplementation in GDM diets to better contribute to healthier GDM pregnancies and post-partum outcomes for both mother and offspring.
Author contribution
Conceptualisation and writing – original draft: M.C.C.; Writing – review and editing: S.A. and S.G.P.; Supervision: S.G.P.
Significance statement
With the increase of gestational diabetes mellitus (GDM) and the universal difficulties in the pharmacological management of diseases during pregnancy, the role of diet interventions with probiotics supplementation is incrementally becoming more important. Current literature contributes relevant information on the best approach to manage GDM through medical nutrition therapy, particularly regarding carbohydrates, lipids, and fibre intake, combined with probiotics supplementation. That is the theme that this manuscript reviews highlights, including future approaches to propel this field of work.
Disclosure statement
The authors declare no conflicts of interest.
Funding
This work was supported by the Portuguese Foundation for Science and Technology under the grants UIDB/05704/2020 for the research unit and CEECINST/00051/2018 for Sónia Gonçalves Pereira.