Introduction
Dietary fibre, which is derived from fruits and vegetables, legumes, whole-grain breads, and cereals, is an umbrella term comprising various types of carbohydrate polymers that cannot be digested nor absorbed by the human small intestine. Consequently, dietary fibre ends up in the colon, where it is fermented by the gut microbiota. Consumption of dietary fibre has been linked to various beneficial physiological effects, including effects on affective (Lawton et al., Reference Lawton, Walton, Hoyland, Howarth, Allan, Chesters and Dye2013) and cognitive (Sandberg et al., Reference Sandberg, Björck and Nilsson2018) processes. Notably, dietary fibre consumption can aid in prevention or treatment of symptoms of depression, anxiety, and stress (Jacka et al., Reference Jacka, O’Neil, Opie, Itsiopoulos, Cotton, Mohebbi, Castle, Dash, Mihalopoulos, Chatterton, Brazionis, Dean and Hodge2017; Silk et al., Reference Silk, Davis, Vulevic, Tzortzis and Gibson2009), which are psychological states characterised by negative mood (e.g., worry, fear, sadness, loss of interest, or emotional tension) that are often accompanied by impaired facets of cognition. Moreover, preclinical data suggests that impaired facets of cognition, such as attention, mental flexibility, and executive functioning, can be restored by dietary fibre (Chunchai et al., Reference Chunchai, Thunapong, Yasom, Wanchai, Eaimworawuthikul, Metzler, Lungkaphin, Pongchaidecha, Sirilun, Chaiyasut, Pratchayasakul, Thiennimitr, Chattipakorn and Chattipakorn2018).
Since dietary fibres vary in origin, chemical composition, and physicochemical properties (comprising solubility, fermentability, and viscosity), they can exert different effects on the body. These differences in physicochemical properties might differentially affect the communicative pathways to the brain, and consequently variably influence affective and cognitive processes. Commonly accepted mechanisms by which dietary fibres are thought to affect cognitive and affective processes are the gut microbiome and short chain fatty acids (SCFAs) (Dalile et al., Reference Dalile, Van Oudenhove, Vervliet and Verbeke2019). Here, we shed light on additional potential mechanisms that may contribute to the positive effects of dietary fibres on these processes, such as the immune system, cholesterol, the intestinal barrier, brain-derived neurotrophic factor (BDNF), and blood pressure, and would help explain additional variance that is not explained by SCFAs. Since in human studies most evidence is associative, we also included animal studies that assess the impact of dietary fibre intake on these various mechanisms. To this end, the current review assesses microbiota-dependent and microbiota-independent biological mechanisms that may underlie the effects of dietary fibre on affective processes and cognition and outlines which physicochemical properties may predict such effects.
Dietary fibres: a definition
Since dietary fibres are characteristically heterogeneous, different classifications have been used to define them, including origin, chemical composition, and physicochemical properties with additional subcategorisation based on the degree of polymerisation. The most commonly used definition of dietary fibre is according to the Codex Alimentarius, which states that dietary fibres include (1) edible carbohydrate polymers occurring in foods as consumed, (2) edible carbohydrates obtained from food raw materials by physical, enzymatic or chemical means and (3) synthetic carbohydrate polymers, which have beneficial physiological effect demonstrated by generally accepted scientific evidence (Joint FAO/WHO Food Standards Programme Secretariat of the CODEX Alimentarius Commission, 2010). In 2016, the Food and Drug Administration (FDA) defined dietary fibre as either (1) non-digestible soluble and insoluble carbohydrates (with three or more monomeric units), and lignin that are intrinsic and intact in plants, or (2) isolated or synthetic non-digestible carbohydrates (with three or more monomeric units), and induce physiological effects that are beneficial to human health (Food and Drug Administration, 2020). At that time, only seven non-digestible carbohydrates were included in the latter category (β-glucan soluble fibre, psyllium husk, cellulose, guar gum, pectin, locust bean gum, and hydroxypropylmethylcellulose). Since then, the list has been extended with ten additional types of dietary fibre [mixed plant cell wall fibres, arabinoxylan (AX), alginate, inulin, and inulin-type fructans (ITFs), high amylose starch (resistant starch 2), galactooligosaccharides (GOS), polydextrose, resistant maltodextrin/dextrin, cross linked phosphorylated RS4, and glucomannan]. Certain types of fibres, such as ITFs and GOS fulfil the criteria of a prebiotic. Prebiotics are defined as “a substrate that is selectively utilised by host microorganisms conferring a health benefit” (Gibson et al., Reference Gibson, Hutkins, Ellen Sanders, Prescott, Reimer, Salminen, Scott, Stanton, Swanson, Cani, Verbeke and Reid2017).
Properties of dietary fibres
The physicochemical characteristics of dietary fibres are subtyped by solubility, viscosity and fermentability. Table 1 provides an overview of the most frequently investigated types of fibres and their concomitant physicochemical properties.
Table 1. Overview of different types of dietary fibres with their concomitant origin, structure and degree of solubility, viscosity and fermentability.

Solubility
Dietary fibres can be water-soluble or water-insoluble, with most plant foods containing a mixture of both. Approximately 20 per cent of consumed dietary fibre is soluble, whereas 80 per cent is insoluble. Insoluble fibres consist mainly of cellulose, hemicellulose, and lignin, and are primarily present in wheat bran, most grain products and vegetables (Cho and Dreher, Reference Cho and Dreher2001). Soluble fibres consist of polysaccharides such as pectin, mucilage, and gum, and are predominantly found in some fruits (e.g., dried prunes, oranges, and grapefruit), oats, barley, dried beans, and legumes (e.g., lentils and pinto beans) (Cho and Dreher, Reference Cho and Dreher2001). Although soluble fibres are less prevalent in foods than insoluble fibres, they have an important influence on digestive and absorptive processes, such as delaying gastric emptying, decreasing glucose absorption, enhancing immune function, and lowering total and low-density lipoprotein (LDL) cholesterol levels (Cho and Dreher, Reference Cho and Dreher2001; Jenkins et al., Reference Jenkins, Kendall, Axelsen, Augustin and Vuksan2000). Insoluble fibres are important for shortening bowel transit time, increasing faecal bulk, and softening stool (Cho and Dreher, Reference Cho and Dreher2001).
Viscosity
Viscosity, the capacity to gel with water, depends on the solubility of the fibre with soluble fibres having higher viscosity. Viscous fibres reduce postprandial glucose response after carbohydrate-rich meals, lower total and LDL cholesterol levels (Jenkins et al., Reference Jenkins, Kendall, Axelsen, Augustin and Vuksan2000; McRorie and McKeown, Reference McRorie and McKeown2017), and slow gastric emptying and macronutrient absorption from the gut (McRorie and McKeown, Reference McRorie and McKeown2017).
Fermentability
The fermentability of dietary fibre varies greatly, ranging from not at all (e.g., lignin) to almost complete fermentation (e.g., pectin) (Mudgil and Barak, Reference Mudgil and Barak2013). Soluble fibres are fermented in the colon, whereas insoluble fibres are less and more slowly fermented. The fermentation of soluble fibres results in the production of short-chain fatty acids (SCFAs) which, in turn, exhibit anti-inflammatory effects in the gut, maintain intestinal barrier integrity (Lewis et al., Reference Lewis, Lutgendorff, Phan, Söderholm, Sherman and McKay2010), induce mucin secretion in the gastrointestinal tract (Monk et al., Reference Monk, Lepp, Wu, Pauls, Robinson and Power2017), and promote gastrointestinal motility (Cherbut et al., Reference Cherbut, Ferrier, Rozé, Anini, Blottière, Lecannu and Galmiche1998). In addition to solubility, chain length and particle size determine fermentability with shorter polymers and small particles being more readily fermented. In in vitro incubation experiments, neutral pectin fractions produced more SCFAs and more acetate than acidic fractions, whereas rhamnose and arabinan produced high proportions of propionate (Onumpai et al., Reference Onumpai, Kolida, Bonnin and Rastall2011). Wood derived xylan and mannan derivatives produced similar total amounts of SCFAs as fructooligosaccharides (FOS) but proportionally more propionate and butyrate, respectively (La Rosa et al., Reference La Rosa, Kachrimanidou, Buffetto, Pope, Pudlo, Martens, Rastall, Gibson and Westereng2019). In contrast, the glycosidic bond configuration has little impact on SCFA production (Harris et al., Reference Harris, Edwards and Morrison2017).
Dietary fibre studies on cognitive and affective processes
Here, we provide a brief overview of the state of the literature on the effects of dietary fibre on cognitive and affective processes for the sake of completeness. Cross-sectional and prospective cohort studies across the lifespan continue to show positive associations between higher dietary fibre intake and better cognitive and affective processes (Muth and Park, Reference Muth and Park2021; Swann et al., Reference Swann, Kilpatrick, Breslin and Oddy2020). Affective processes are measured by, for instance, better mental health quality of life and lower incidents of depression and anxiety. Moreover, dietary source of fibres appears to be differentially associated with the incidence of depression (Kim et al., Reference Kim, Byeon and Shin2020). Despite assessing effects on the short-term, human interventional studies enable controlled administration of specific fibres and exclusion of confounding effects. These studies are displayed in Table 2. Briefly, 18 studies were found, with 7 of them (Azpiroz et al., Reference Azpiroz, Dubray, Bernalier-Donadille, Cardot, Accarino, Serra, Wagner, Respondek and Dapoigny2017; Buigues et al., Reference Buigues, Fernández-Garrido, Pruimboom, Hoogland, Navarro-Martínez, Martínez-Martínez, Verdejo, Carmen Mascarós, Peris and Cauli2016; Dehhaghi et al., Reference Dehhaghi, Kazemi, Panahi and Guillemin2019; Farhangi et al., Reference Farhangi, Zare Javid, Sarmadi, Karimi and Dehghan2018; Grimaldi et al., Reference Grimaldi, Gibson, Vulevic, Giallourou, Castro-Mejía, Hansen, Leigh Gibson, Nielsen and Costabile2018; Kao et al., Reference Kao, Safarikova, Marquardt, Mullins and Lennox2019; Silk et al., Reference Silk, Davis, Vulevic, Tzortzis and Gibson2009) conducted in populations with various mental, physical, or gastrointestinal disorders, and the remaining 11 conducted in healthy populations (Best et al., Reference Best, Bryan and Burns2008, Reference Best, Kemps and Bryan2009, Reference Best, Howe, Bryan, Buckley and Scholey2015; Childs et al., Reference Childs, Röytiö, Alhoniemi, Fekete, Forssten, Hudjec, Lim, Steger, Yaqoob, Tuohy, Rastall, Ouwehand and Gibson2014; Lawton et al., Reference Lawton, Walton, Hoyland, Howarth, Allan, Chesters and Dye2013; Pasman et al., Reference Pasman, Blokdijk, Bertina, Hopman and Hendriks2003; Ramnani et al., Reference Ramnani, Costabile, Bustillo and Gibson2015; Schmidt et al., Reference Schmidt, Cowen, Harmer, Tzortzis, Errington and Burnet2015; Smith, Reference Smith2005; Smith et al., Reference Smith, Sutherland and Hewlett2015; Talbott and Talbott, Reference Talbott and Talbott2009). The duration of the intervention periods varied, with three studies carrying out single administration (Best et al., Reference Best, Bryan and Burns2008, Reference Best, Howe, Bryan, Buckley and Scholey2015; Smith et al., Reference Smith, Sutherland and Hewlett2015), one study comprising 2 days (Pasman et al., Reference Pasman, Blokdijk, Bertina, Hopman and Hendriks2003), and the others comprising 2–13 weeks (Azpiroz et al., Reference Azpiroz, Dubray, Bernalier-Donadille, Cardot, Accarino, Serra, Wagner, Respondek and Dapoigny2017; Best et al., Reference Best, Kemps and Bryan2009; Buigues et al., Reference Buigues, Fernández-Garrido, Pruimboom, Hoogland, Navarro-Martínez, Martínez-Martínez, Verdejo, Carmen Mascarós, Peris and Cauli2016; Childs et al., Reference Childs, Röytiö, Alhoniemi, Fekete, Forssten, Hudjec, Lim, Steger, Yaqoob, Tuohy, Rastall, Ouwehand and Gibson2014; Farhangi et al., Reference Farhangi, Zare Javid, Sarmadi, Karimi and Dehghan2018; Grimaldi et al., Reference Grimaldi, Gibson, Vulevic, Giallourou, Castro-Mejía, Hansen, Leigh Gibson, Nielsen and Costabile2018; Kao et al., Reference Kao, Safarikova, Marquardt, Mullins and Lennox2019; Kazemi et al., Reference Kazemi, Noorbala, Azam, Eskandari and Djafarian2019; Lawton et al., Reference Lawton, Walton, Hoyland, Howarth, Allan, Chesters and Dye2013; Ramnani et al., Reference Ramnani, Costabile, Bustillo and Gibson2015; Schmidt et al., Reference Schmidt, Cowen, Harmer, Tzortzis, Errington and Burnet2015; Silk et al., Reference Silk, Davis, Vulevic, Tzortzis and Gibson2009; Smith, Reference Smith2005; Talbott and Talbott, Reference Talbott and Talbott2009). Eleven studies utilised ITFs, with the majority of them (nine studies) showing some positive effects on affective and cognitive indices. Five studies utilised mixtures of fibre, mostly revealing positive effects of interventions, unless it was administered only once. One study administrated β-glucans and one study administered resistant starch. Not all studies are of equal quality, with some lacking proper control conditions. Notably, most human intervention studies administered dietary fibre supplements rather than whole foods. Whole foods are not only rich in dietary fibre but also contain minerals and antioxidants that may improve mood and cognition (Gomez-Pinilla, Reference Gomez-Pinilla2008). Moreover, it cannot be excluded that some placebos (e.g., maltodextrin) may have had an effect on mood and/or cognition as well, possibly via gut-independent mechanisms (Kendig et al., Reference Kendig, Lin, Beilharz, Rooney and Boakes2014). Future intervention studies should take such effects into account. Some fibres such as pectin, hemicelluloses (e.g., AX), and gums (e.g., guar, xanthan, and Arabic gum) are yet to be explored in the context of mood and cognition. As these fibres are highly viscous, they may affect mood and cognition through microbiota-independent mechanisms as discussed below. Overall, the majority of studies point to beneficial effects on mood and cognition, but none of these studies causally addresses potential mechanisms of action. In the next section, we will discuss potential mechanisms, both microbiota-dependent as well as microbiota-independent, that may be involved in dietary fibre’s beneficial effects on affective and cognitive processes.
Table 2. Human studies assessing the effect of dietary fibre interventions on mood and cognition.

Abbreviations: GOS, galactooligosaccharides; FOS, fructooligosaccharides; XOS, xylooligosaccharides.
a CAR a measure of hypothalamic–pituitary–adrenal (HPA) axis function (Clow et al., Reference Clow, Hucklebridge, Stalder, Evans and Thorn2010). CAR has been previously associated with anticipation of daily stress (Fries et al., Reference Fries, Dettenborn and Kirschbaum2009) and executive function (Butler et al., Reference Butler, Klaus, Edwards and Pennington2017).
Biological mechanisms
Dietary fibre may affect mood and cognition via various biological mechanisms, both microbiota-dependent and microbiota-independent, which are reviewed in this section. Figure 1 provides an overview of the different biological mechanisms that may underlie dietary fibres’ effect on affective and cognitive processes according to their physicochemical properties.
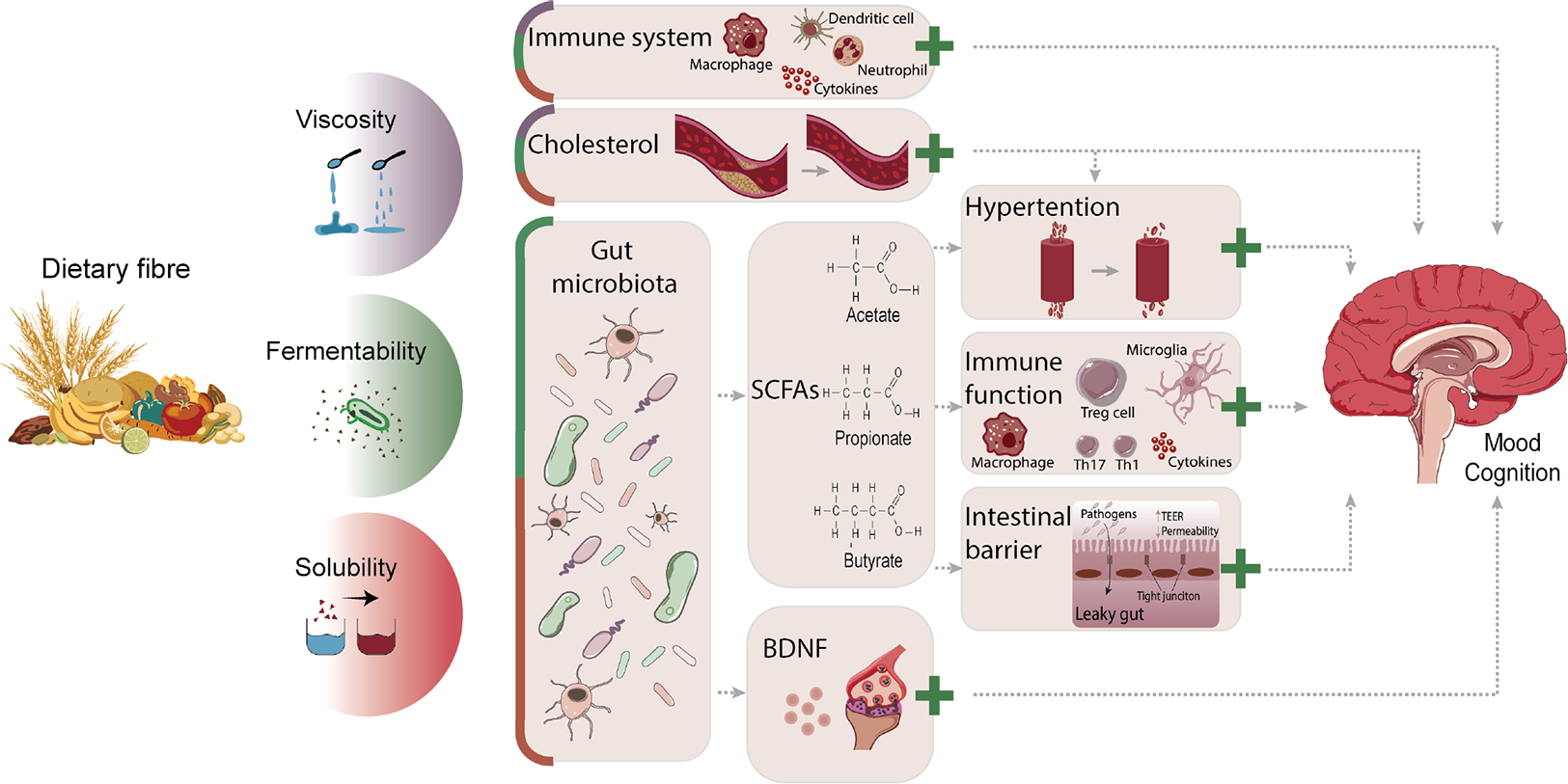
Figure 1 Overview of potential biological mechanisms underlying dietary fibres’ effects on mood and cognition according to physicochemical properties. The degree of viscosity, fermentability, and solubility of dietary fibres influences gut microbiota composition and function, the immune system, as well as cholesterol levels, through which mood and cognition can be modulated. Via the direct immune route, viscous, fermentable, and soluble fibres can reduce pro-inflammatory cytokines, lower numbers and activation of splenic macrophages and DCs, and increase neutrophils in the blood. Cholesterol levels can be lowered by viscous, fermentable, and soluble fibres and impact the brain, but can also indirectly affect it by reducing hypertension. Fermentability and solubility of dietary fibre modulate the gut microbiota, by which it can influence affective and cognitive processes via SCFA-dependent mechanisms or BDNF levels. SCFAs can lower hypertension as well as improve immune function and intestinal barrier integrity. Via the indirect immune route, SCFAs suppress pro-inflammatory reactions by reducing pro-inflammatory cytokines, increasing anti-inflammatory cytokines and Treg cells, as well as restoring microglial cell morphology and reversing microglial immaturity. All these mechanisms have been associated with modulation of mood and cognition in health and disease. The likelihood that the different biological mechanisms underlie dietary fibres’ effect on mood and cognition are displayed in proportion, with larger cubicle areas reflecting increased potential. The green plus sign indicates beneficial effects. BDNF, brain-derived neurotrophic factor; DCs, dendritic cells; Treg cells, regulatory T-cells; SCFAs, short chain fatty acids.
Gut microbiome
Dietary fibre’s ability to influence affective and cognitive processes is most likely through its interactions with the gut microbiome. The gut microbiome refers to the 1 × 1013 to 1 × 1014 microorganisms that reside in the human large intestine, which is one of the most densely and diversely colonised organs in the human body. Pre-clinical studies suggest that the gut microbiome plays an important modulating role in affective and cognitive processes. Studies in germ-free (GF) mice suggested a causal role of the gut microbiota in developing brain function and behaviour, as those mice exhibit compromised social, cognitive, and anxiety-like behaviours (Desbonnet et al., Reference Desbonnet, Clarke, Shanahan, Dinan and Cryan2014), such as reduced non-spatial memory, lower social motivation, and symptoms of anxiety compared to their conventionally raised specific pathogen-free (SPF) counterparts (Clarke et al., Reference Clarke, Grenham, Scully, Fitzgerald, Moloney, Shanahan, Dinan and Cryan2013; Desbonnet et al., Reference Desbonnet, Clarke, Shanahan, Dinan and Cryan2014). Also, faecal microbiota transplantation of “depressive microbiota” derived from patients with major depressive disorder (MDD; a disorder specifically known for its symptoms of negative mood and impaired cognition) to GF mice (Zheng et al., Reference Zheng, Zeng, Zhou, Liu, Fang, Xu, Zeng, Chen, Fan, Du, Zhang, Yang, Yang, Meng, Li, Melgiri, Licinio, Wei and Xie2016) resulted in depression-like behaviours relative to colonisation with “healthy microbiota” derived from healthy donors.
Human studies also suggest that differences in the gut microbiome composition are associated with mood and cognition. For instance, gut microbiota composition of healthy subjects differs from that of patients suffering from MDD. Specifically, the relative abundance of Firmicutes (Jiang et al., Reference Jiang, Ling, Zhang, Mao, Ma, Yin, Wang, Tang, Tan, Shi, Li and Ruan2015), Bifidobacteria and Lactobacilli (Aizawa et al., Reference Aizawa, Tsuji, Asahara, Takahashi, Teraishi, Yoshida, Ota, Koga, Hattori and Kunugi2016) appears lower in MDD patients compared to healthy controls, whereas Actinobacteria, Proteobacteria, and Bacteroidetes appear to be higher (Jiang et al., Reference Jiang, Ling, Zhang, Mao, Ma, Yin, Wang, Tang, Tan, Shi, Li and Ruan2015). Increases in the relative abundance of Bifidobacteria and Lactobacilli in particular have been associated with lower anxiety and better memory and learning in both rodents (Bravo et al., Reference Bravo, Forsythe, Chew, Escaravage, Savignac, Dinan, Bienenstock, Cryan and Designed2011) and humans (Messaoudi et al., Reference Messaoudi, Lalonde, Violle, Javelot, Desor, Nejdi, Bisson, Rougeot, Pichelin, Cazaubiel and Cazaubiel2011). Nevertheless, it seems unlikely that bacterial strains are directly responsible for these effects. Rather, it is speculated that bacterial metabolites like SCFAs and BDNF mediate the interaction between the microbiota and psychological functioning.
Short-chain fatty acids
Soluble fibres (e.g., pectin and gums) provide substrates for bacterial fermentation, which in turn leads to the production of SCFAs in the colon. Acetate, propionate, and butyrate are the most abundant SCFAs, and they are present in the colon in an approximate molar ratio of 60:20:20, respectively. Type and availability of substrate, as well as gut transit time and composition of the gut microbiota influence the levels and relative proportions of SCFAs in the colon. Following their production by microbial fermentation of dietary fibre, SCFAs are rapidly absorbed by colonic cells via monocarboxylate transporters and produce energy for the cells. Those that are not absorbed travel via the basolateral membrane into the portal circulation. In the liver, SCFAs are incorporated in glucose, cholesterol, and fatty acids, thus only small amounts actually reach systemic circulation, and subsequently the brain (Boets et al., Reference Boets, Gomand, Deroover, Preston, Vermeulen, De Preter, Hamer, Van Den Mooter, De Vuyst, Courtin, Annaert, Delcour and Verbeke2017).
SCFAs and cognitive and affective processes
While numerous animal studies have relied on intracerebroventricular (ICV), intraperitoneal, subcutaneous, and oral administrations to deliver SCFAs and investigate their impact on brain and behaviour (see Dalile et al., Reference Dalile, Van Oudenhove, Vervliet and Verbeke2019), few studies explored whether the effects of dietary fibre/prebiotics on affective and cognitive functioning are mediated by or correlated with the observed increases in SCFA levels. One of these studies showed that FOS- and GOS-induced increases in cecal SCFAs correlated with effects on depressive and anxious behaviour and stress responses in mice (Burokas et al., Reference Burokas, Arboleya, Moloney, Peterson, Murphy, Clarke, Stanton, Dinan and Cryan2017). Moreover, drinking water supplemented with a GOS mixture (B-GOS; 3 per cent) for 3 weeks increased plasma acetate levels and upregulated subunits of the N-methyl-d-aspartate receptor (NMDAR) in the brain (Gronier et al., Reference Gronier, Savignac, Di Miceli, Idriss, Tzortzis, Anthony and Burnet2018; Kao et al., Reference Kao, Spitzer, Anthony, Lennox and Burnet2018), which is implicated in synaptic plasticity and memory formation, as well as Acetyl Co-A Carboxylase mRNA, an enzyme in the brain that plays an important role in supplying fatty acids for myelination. Indeed, in one of these studies, mice fed with the GOS mixture gained greater cognitive flexibility (Gronier et al., Reference Gronier, Savignac, Di Miceli, Idriss, Tzortzis, Anthony and Burnet2018). Unfortunately, the other did not test whether the GOS mixture induced changes in cognition (Kao et al., Reference Kao, Spitzer, Anthony, Lennox and Burnet2018). Direct administration of acetate also induced similar neurochemical changes as that of GOS (Gronier et al., Reference Gronier, Savignac, Di Miceli, Idriss, Tzortzis, Anthony and Burnet2018), suggesting that acetate may play a mechanistic role in the observed effects of GOS administration.
Unfortunately, studies in humans administering prebiotics and assessing effects on affective and cognitive processes do not quantify SCFAs and do not explore the extent to which circulating SCFAs mediate the observed effects (Dalile et al., Reference Dalile, Van Oudenhove, Vervliet and Verbeke2019). However, one study found that increasing colonic propionate by consumption of 10 g of an inulin–propionate ester (thereby delivering of 2.36 g propionate to the colon, which is 2.5 times habitual daily propionate production) influenced brain anticipatory reward responses during a functional magnetic resonance imaging (fMRI) food picture evaluation task in non-obese men (Byrne et al., Reference Byrne, Chambers, Alhabeeb, Chhina, Morrison, Preston, Tedford, Fitzpatrick, Irani, Busza, Garcia-Perez, Fountana, Holmes, Goldstone and Frost2016). In parallel, the subjective appeal of high-energy food pictures decreased and energy intake during an ad libitum meal was reduced. These results support the mediating role of SCFAs in microbiota-gut-brain axis communication. Moreover, our group recently showed that daily administration of known amounts of a SCFA mixture equivalent to 20 or 10 g of AX (174.2 mmol acetate, 13.3 mmol propionate, and 52.4 mmol butyrate, or half the dose, respectively) to the colon of healthy men for 1 week significantly reduced the cortisol response to an acute psychosocial stress challenge (Dalile et al., Reference Dalile, Vervliet, Bergonzelli, Verbeke and Van Oudenhove2020). Furthermore, we found that the increase in circulating SCFAs was associated with a decrease in cortisol response to stress. This preliminary study indicates that SCFAs are clearly an important mechanism through which fermentable fibres can affect HPA axis (a major neuroendocrine system that regulates stress responses as well as affective processes) reactivity to stress.
Conceptually, SCFAs may influence affective and cognitive processes by influencing multiple pathways that have been reviewed elsewhere (Dalile et al., Reference Dalile, Van Oudenhove, Vervliet and Verbeke2019). However, the extent to which increasing the substrate of SCFAs (soluble fibres) increases SCFA concentrations and consequently influences affective and cognitive processes, remains understudied. In the following sections, we focus on potential mechanisms by which fibre consumption may alter affective and cognitive processes via interactions with SCFAs and other microbiota-gut-brain axis mediators, namely, the immune system, the intestinal barrier, and hypertension. As studies that directly investigate the effect of dietary fibre on SCFAs and these mediators and subsequent changes in affective and cognitive processes are currently lacking, we summarise the available evidence for an effect of SCFAs on these intermediate systems and for the involvement of these systems in the regulation of affective and cognitive processes.
Immune system (indirect effects)
Immune system and cognitive and affective processes
The immune system is important for both affective and cognitive processes. Challenging the immune system activates cytokines which are involved in the repair of damaged tissue and the restoration of homeostasis. The inflammatory response may also elicit deleterious effects, such as alterations in mood and cognition. This became evident from, for instance, a study vaccinating healthy volunteers with Salmonella typhi which increased IL-6, IL-1Ra, and tumour necrosis factor (TNF)-α, but also decreased mood without any signs of physical sickness (Wright et al., Reference Wright, Strike, Brydon and Steptoe2005). Other studies using acute inflammatory stimulation showed similar results (Brydon et al., Reference Brydon, Walker, Wawrzyniak, Whitehead, Okamura, Yajima, Tsuda and Steptoe2009; Strike et al., Reference Strike, Wardle and Steptoe2004). Also, endotoxin-induced inflammation showed a dose-dependent elevation in pro-inflammatory cytokines IL-6 and TNF-α, but also anti-inflammatory cytokine IL-10 (Grigoleit et al., Reference Grigoleit, Kullmann, Wolf, Hammes and Wegner2011). These elevations were accompanied by a dose-dependent increase in anxiety, negative mood, and poor long-term memory. Involvement of inflammation in negative mood and poor cognitive function is supported by numerous reviews that repeatedly showed an increased incidence of negative mood symptoms with elevated levels of pro-inflammatory markers (CRP, TNF-α, IL-1β, IL-2, and IL6) in peripheral blood and cerebrospinal fluid (CSF) in patients with MDD (Dowlati et al., Reference Dowlati, Herrmann, Swardfager, Liu, Sham, Reim and Lanctôt2010; Hannestad et al., Reference Hannestad, Dellagioia and Bloch2011; Hiles et al., Reference Hiles, Baker, De Malmanche and Attia2012). Regarding cognition, higher levels of CRP are associated with impairment in several cognitive domains such as with lower psychomotor speed and poorer executive function (Krogh et al., Reference Krogh, Benros, Jørgensen, Vesterager, Elfving and Nordentoft2014).
Reducing inflammation has been shown to improve mood (Allison and Ditor, Reference Allison and Ditor2015). Moreover, mood stabilisers such as lithium and valproate suppressed IL-6 levels in patients with bipolar disorder compared to untreated patients (Kim et al., Reference Kim, Jung, Myint, Kim and Park2007). Atypical antipsychotics, which are used as a treatment for both MDD and bipolar disorder, have also been shown to decrease levels of TNF-α and IL-6 in animal models (Bian et al., Reference Bian, Kato, Monji, Hashioka, Mizoguchi, Horikawa and Kanba2008; Kato et al., Reference Kato, Monji, Hashioka and Kanba2007). Furthermore, meta-analyses assessing various cytokine levels after anti-depressant treatment in people with depression show significant decreases in IL-6, IL-1β, and CRP (Hannestad et al., Reference Hannestad, Dellagioia and Bloch2011; Hiles et al., Reference Hiles, Baker, De Malmanche and Attia2012). It seems therefore likely that inflammation plays a significant role in mood. To this end, reducing inflammation may aid negative mood symptoms in some individuals.
SCFAs and immune system
SCFAs are able to modulate the immune system. SCFAs may reduce inflammation by modulating molecular signalling pathways, including free fatty acid receptor (FFAR) 2 and 3 activation (Vinolo et al., Reference Vinolo, Rodrigues, Nachbar and Curi2011), and histone deacetylation (HDAC) inhibition (Waldecker et al., Reference Waldecker, Kautenburger, Daumann, Busch and Schrenk2008). As SCFAs directly interact with FFAR2 on immune cells, which is suggested to regulate regulatory T-cells (Treg cells) in the colon (Smith et al., Reference Smith, Howitt, Panikov, Michaud, Gallini, Bohlooly-Y, Glickman and Garrett2013), Treg cells may then suppress immune responses, thereby reducing inflammation.
Of all SCFAs, butyrate seems the most potent SCFA in modulating the immune system. Butyrate increases the expression of anti-inflammatory molecules in dendritic cells (DCs) and macrophages, thereby supporting Treg differentiation (Singh et al., Reference Singh, Gurav, Sivaprakasam, Brady, Padia, Shi, Thangaraju, Prasad, Manicassamy, Munn, Lee, Offermanns and Ganapathy2014), and hence suppress inflammation. Furthermore, due to its ability to inhibit HDAC activity, butyrate decreases the secretion of proinflammatory cytokines interleukin (IL)-12 and IL-6 in dendritic cells and allows dendritic cells to promote anti-inflammatory cytokine IL-10-secreting T cells (Chang et al., Reference Chang, Hao, Offermanns and Medzhitov2014). Moreover, butyrate inhibits the production of pro-inflammatory cytokines interferon (IFN)-y and IL-2 (Looijer-Van Langen and Dieleman, Reference Looijer-Van Langen and Dieleman2009). Propionate may also modulate inflammation by inducing forkhead box P3 (FOXP3) expression, a protein that regulates the development and function of Tregs (Arpaia et al., Reference Arpaia, Campbell, Fan, Dikiy, Van Der Veeken, Deroos, Liu, Cross, Pfeffer, Coffer and Rudensky2013). Furthermore, both propionate and acetate have been found to increase the production of anti-inflammatory cytokine IL-10 (Cavaglieri et al., Reference Cavaglieri, Nishiyama, Claudio Fernandes, Curi, Miles and Calder2003).
Indeed, most animal studies using prebiotics indicate beneficial effects on the immune system by lowering proinflammatory cytokine expression. For instance, gene expression of pro-inflammatory cytokines IL-1β and (TNF)-α decreased in the brain of mice fed with a high fibre (5 per cent inulin) diet but not in mice fed a low fibre (1 per cent cellulose) diet (Matt et al., Reference Matt, Allen, Lawson, Mailing, Woods and Johnson2018). The decrease in IL-β and TNF-α correlated significantly with faecal butyrate levels, with higher butyrate levels corresponding with lower pro-inflammatory gene expression. Furthermore, 3-week administration of a FOS + GOS mixture (dissolved in drinking water for 0.3–0.4 g/mouse/day) reduced the elevations in proinflammatory cytokine levels (IL-6 and TNF-α) caused by chronic stress induction in C57BL/6J male mice (Burokas et al., Reference Burokas, Arboleya, Moloney, Peterson, Murphy, Clarke, Stanton, Dinan and Cryan2017). Moreover, mice fed with a high fermentable fibre (10 per cent pectin) diet became less sick and recovered faster from lipopolysaccharide (LPS)-induced sickness compared to mice fed with non-fermentable fibre (5 per cent cellulose) (Sherry et al., Reference Sherry, Kim, Dilger, Bauer, Moon, Tapping, Fahey, Tappenden and Freund2010). LPS-stimulated macrophages from the mice fed with pectin showed decreased pro-inflammatory cytokines IL-1β, TNF-α, interferon (IFN)-γ, IL-12, and nitrate, and increased anti-inflammatory cytokine IL-1RA compared to mice fed with cellulose. With regards to brain-based inflammation levels, increased levels of anti-inflammatory IL-1RA and IL-4 mRNA, and a decrease in pro-inflammatory IL-1β and TNF-α were observed in mice fed with pectin compared to mice fed with cellulose (Sherry et al., Reference Sherry, Kim, Dilger, Bauer, Moon, Tapping, Fahey, Tappenden and Freund2010). Since IL-4 expression is stimulated by histone acetylation, the authors hypothesised that the increased butyrate concentrations observed as a result from dietary fibre fermentation of pectin stimulated IL-4, and hence may aid immune response regulation (McLoughlin et al., Reference McLoughlin, Berthon, Jensen, Baines and Wood2017).
In humans, few studies have administered SCFAs and found an effect on inflammation as shown by a systematic review where only two of five studies revealed statistically significant decreases in serum inflammatory markers, namely, decreases in IL-1β following colonic infusion of SCFA mixture, and decreases in TNF levels following rectal acetate administration. Some studies using prebiotics support the hypothesis that SCFAs induce immunomodulatory effects SCFAs. For instance, in healthy elderly, consumption of a mixture of GOS (B-GOS; 5.5 g/day) for 10 weeks increased levels of anti-inflammatory cytokine IL-10, and lowered levels of pro-inflammatory cytokines IL-1, IL-6, and TNF-α (Vulevic et al., Reference Vulevic, Drakoularakou, Yaqoob, Tzortzis and Gibson2008). Furthermore, supplementation with inulin and xylooligosaccharides (XOS; 6.64 g/day) for 4 weeks lowered the expression of pro-inflammatory IL-1b, IL-8, IL-12, and TNF-α, whereas it increased anti-inflammatory IL-10 and IL-13 in the blood of healthy subjects (Lecerf et al., Reference Lecerf, Dépeint, Clerc, Dugenet, Niamba, Rhazi, Cayzeele, Abdelnour, Jaruga, Younes, Jacobs, Lambrey, Abdelnour and Pouillart2012). In a recent meta-analysis, 9 out of 13 studies reported a significant decrease in one or more systemic pro-inflammatory cytokine (primarily TNF-α, IL-6, c-reactive protein (CRP), or IFN-γ) after consumption of prebiotic oligosaccharides compared with control (McLoughlin et al., Reference McLoughlin, Berthon, Jensen, Baines and Wood2017). In contrast, in two studies that were conducted in healthy participants, CRP, TNF-α, and IL-6 increased following oligosaccharide supplementation. Another systematic review, conducted in 10 prebiotic and synbiotic (supplements combining probiotics and prebiotics) trials, of which 7 prebiotic and 3 synbiotic, representing 534 obese/overweight subjects, also found inconsistent effects of prebiotic treatment on immunomodulation. They found that only 6 out of 10 trials (two with GOS, one with inulin, and three with different synbiotics) reduced CRP levels, four out of four trials (one with oligofructose-enriched inulin, one with inulin, and two with different synbiotics) reduced levels of IL-6 and/or TNF, and four out of the five trials (one with oligofructose-enriched inulin, one with inulin, one with GOS, and one with different synbiotics) lowered endotoxin levels (Fernandes et al., Reference Fernandes, do, Mocellin, Kuntz and Trindade2017). Taken together, it might still be too early to promote the use of prebiotics for reduction of low-grade inflammation. However, the evidence seems promising and more studies are needed to conclude whether prebiotics can lower inflammation via increased SCFA production in humans, and in turn promote beneficial effects on mood and cognition.
Intestinal barrier
Intestinal barrier and cognitive and affective processes
The intestinal barrier is mainly responsible for regulating the absorption of nutrients, electrolytes, and water from the lumen, and to prevent pathogenic microorganisms and toxic luminal substances to enter the host. A dysfunctional intestinal barrier may lead to increased intestinal permeability, and hence to the translocation of luminal antigens, bacteria, and toxins through the intestinal wall into the blood stream. In turn, this may cause low-grade inflammation (Caspani and Swann, Reference Caspani and Swann2019) and hence may affect affective and cognitive processes. Indeed, some studies report associations between depressive symptoms and increased levels of indirect markers of intestinal permeability such as intestinal-type fatty acid-binding protein (I-FABP), fatty acid-binding protein 2 (FABP2), LPS, and zonulin (Ohlsson et al., Reference Ohlsson, Gustafsson, Lavant, Suneson, Brundin, Westrin, Ljunggren and Lindqvist2019; Stevens et al., Reference Stevens, Goel, Seungbum, Richards, Holbert, Pepine and Raizada2018). Moreover, a study using a direct measure of intestinal permeability (lactulose to mannitol ratio) also found a positive association with depression severity (Calarge et al., Reference Calarge, Devaraj and Shulman2019).
SCFAs and intestinal barrier
SCFAs are the preferred energy substrates for the colonic epithelium (Cushing et al., Reference Cushing, Alvarado and Ciorba2015) and can contribute to enhanced intestinal barrier function. This has been shown in in vitro and in in vivo experiments in which the application of individual and combinations of SCFAs increased transepithelial electrical resistance (Feng et al., Reference Feng, Wang, Wang, Huang and Wang2018; Suzuki et al., Reference Suzuki, Yoshida and Hara2008), decreased paracellular transport markers (Suzuki et al., Reference Suzuki, Yoshida and Hara2008), and stimulated the formation of tight-junctions (Feng et al., Reference Feng, Wang, Wang, Huang and Wang2018). Furthermore, SCFAs may protect the intestinal barrier from disruption induced by LPS through the inhibition of the NLPR3 inflammasome and autophagy (Feng et al., Reference Feng, Wang, Wang, Huang and Wang2018).
Interestingly, an experimental study showed that 1-week oral administration of a SCFA mixture (67.5 mM acetate, 25 mM propionate, and 40 mM butyrate) alleviated stress-induced increases in intestinal permeability and decreased depressive-like behaviour in mice (Van De Wouw et al., Reference Van De Wouw, Boehme, Lyte, Wiley, Strain, O’sullivan, Clarke, Stanton, Dinan, Cryan, Barrett, Grundy and Cryan2018). Moreover, animal studies show that inulin supplementation (0.5 per cent as well as 1 per cent) increased expression of intestinal barrier function-related genes after Salmonella enterica infection in SPF chickens (Song et al., Reference Song, Li, Everaert, Liu, Zheng, Zhao and Wen2020). In obese mice, oligofructose increased the relative abundance of Bifidobacterium spp., leading to increases in GLP-2 and tight-junctions, lower levels of inflammation, and a significant improvement of intestinal permeability (Cani et al., Reference Cani, Possemiers, Van De Wiele, Guiot, Everard, Rottier, Geurts, Naslain, Neyrinck, Lambert, Muccioli and Delzenne2009). As ITFs stimulate SCFA production, the effect is likely mediated by SCFAs, although this is speculative as SCFA concentrations were not measured.
Human studies that evaluated the impact of dietary fibre on intestinal permeability yielded inconsistent results. Administration of green bananas and pectin for 1 week significantly reduced intestinal permeability and stool output in children with diarrhoea (Rabbani et al., Reference Rabbani, Teka, Zaman, Majid, Khatun and Fuchs2001). Another study in healthy subjects found a significant reduction in intestinal permeability markers zonulin and GLP-2 after consumption of inulin-pasta (8 weeks) compared to control pasta (Russo et al., Reference Russo, Linsalata, Clemente, Chiloiro, Orlando, Marconi, Chimienti and Riezzo2012). In contrast, a high dose of inulin (30–35 g/day) for 1 week in patients on liquid enteral nutrition did not affect intestinal permeability (Sobotka et al., Reference Sobotka, Brátova, Šlemrová, Maňák, Vižd’a and Zadák1997) neither did supplementation with GOS (5.5 g/day) for 12 weeks in men with well-controlled type 2 diabetes (Pedersen et al., Reference Pedersen, Gallgher, Horton, Ellis, Ijaz, Wu, Jaiyeola, Diribe, Duparc, Cani, Gibson, Hinton, Wright, La Ragione and Robertson2016).
These results suggest that the beneficial effects of SCFA on intestinal permeability are not always evident from human studies. Fermentable fibres may induce beneficial effects on affective and cognitive processes via stimulating SCFA production. However, there is a need for human studies that assess whether improvements in intestinal barrier function by means of SCFA stimulation are also associated with improvements in mood and cognition, albeit by attenuating stress-induced negative effects, to confirm this speculation.
Hypertension
Hypertension and cognitive and affective processes
Hypertension, also known as high blood pressure, is a well-established risk factor for cognitive impairment and dementia (Forte et al., Reference Forte, De Pascalis, Favieri and Casagrande2020). Cognitive impairment is more frequent in patients with hypertension, and this is positively associated with the severity (stage) of hypertension (Muela et al., Reference Muela, Costa-Hong, Yassuda, Moraes, Memória, Machado, Macedo, Shu, Massaro, Nitrini, Mansur and Bortolotto2017). Moreover, the prevalence of anxiety disorders is higher in patients with essential hypertension (Vetere et al., Reference Vetere, Ripaldi, Ais, Korob, Kes and Villamil2007). However, it remains to be established to what extent a reduction in blood pressure improves affective processes and cognition. A systematic review and meta-analysis of randomised controlled trials (RCTs) indicated that pharmacological treatment of hypertension reduces cognitive decline in older adults (Gupta et al., Reference Gupta, Perdomo, Billinger, Beddhu, Burns and Gronseth2020). On the other hand, recent large-scale associative studies report mixed findings. In 14,195 hypertensive older adults from the United States and Australia, the use of beta blockers alone or combined with angiotensin-receptor blockers was associated with depressive symptoms, but this was not the case when using angiotensin-receptor blockers on its own, angiotensin-converting enzyme inhibitors, calcium channel blockers, or any other possible combination of drugs among them (Agustini et al., Reference Agustini, Mohebbi, Woods, McNeil, Nelson, Shah, Murray, Ernst, Reid, Tonkin, Lockery and Berk2020). However, another cross-sectional study comprising 1.8 million patients from Scotland reported that angiotensin antagonists and calcium channel blockers were associated with episodes of MDD in hypertensive patients with or without history of mood disorders (Shaw et al., Reference Shaw, Mackay, Pell, Padmanabhan, Bailey and Smith2020). Another nation-wide population-based study of 5.4 million people in Denmark found that no drugs belonging to angiotensin agents, calcium antagonists, β-blockers, or diuretics were associated with an increased risk of depression. In contrast, angiotensin agents, calcium antagonists, and β-blockers were associated with decreased rates of depression. Moreover, non-clinical evidence indicates that mood and blood pressure are related, with increasing intensity of negative moods (stress, anxiety, and anger) being associated with increased blood pressure, with feeling tired (low energy) being associated with the opposite pattern, while changes in intensity in positive mood (happy) showing little changes in blood pressure (Shapiro et al., Reference Shapiro, Jamner, Goldstein and Delfino2001).
SCFAs and hypertension
Meta-analyses and reviews of RCTs reveal that higher dietary fibre consumption reduces blood pressure, an effect that is most pronounced in patients with hypertension, regardless of the type of fibre (Aleixandre and Miguel, Reference Aleixandre and Miguel2016). Some studies report differences in gut microbiota composition, particularly in SCFA-producers, as well as in SCFA profiles in spontaneously hypertensive rats (SHR) and in hypertensive patients (Yang et al., Reference Yang, Santisteban, Rodriguez, Li, Ahmari, Carvajal, Zadeh, Gong, Qi, Zubcevic, Sahay, Pepine, Raizada and Mohamadzadeh2015). For example, patients with high blood pressure exhibit significantly less butyrate-producers such as Roseburia and Eubacterium but also lower plasma butyrate concentrations compared to normotensive subjects (Kim et al., Reference Kim, Goel, Kumar, Qi, Lobaton, Hosaka, Mohammed, Handberg, Richards, Pepine and Raizada2018). Furthermore, intraperitoneal or intramedullary butyrate administration (Kim et al., Reference Kim, Goel, Kumar, Qi, Lobaton, Hosaka, Mohammed, Handberg, Richards, Pepine and Raizada2018; Wang et al., Reference Wang, Zhu, Lu, Liu, Zhang, Xu, Liu, Li and Yang2017), acetate (Marcques et al., Reference Marcques, Nelson, Chu, Horlock, Fiedler, Ziemann, Tan, Kuruppu, Rajapakse, El-Osta, Mackay and Kaye2017), and propionate (Bartolomaeus et al., Reference Bartolomaeus, Balogh, Yakoub, Homann, Markó, Höges, Tsvetkov, Krannich, Wundersitz, Avery, Haase, Kräker, Hering, Maase, Kusche-Vihrog, Grandoch, Fielitz, Kempa, Gollasch, Zhumadilov, Kozhakhmetov, Kushugulova, Eckardt, Dechend, Rump, Forslund, Müller, Stegbauer and Wilck2019) administration in drinking water resulted in anti-hypertensive effects in rodents. Most recently, expression levels of butyrate‐sensing receptors FFAR3 and olfactory receptor (OLFR) 59 in the hypothalamus of SHR were found to be lower than in control rats, likely underlying the reduced effects of centrally administered butyrate on blood pressure in the SHR. Finally, functional magnetic resonance imaging revealed reduced activation of cardio regulatory brain regions of SHR compared to controls following ICV injection of butyrate to reach physiological concentrations (3–5 μM/L) in the CSF (Yang et al., Reference Yang, Magee, Colon-Perez, Larkin, Liao, Balazic, Cowart, Arocha, Redler, Febo, Vickroy, Martyniuk, Reznikov and Zubcevic2019). Only correlational evidence exists in humans, which indicate that higher SCFA-producing bacteria are associated with lower blood pressure and high faecal SCFA levels (De La Cuesta-Zuluaga et al., Reference De La Cuesta-Zuluaga, Mueller, Álvarez-Quintero, Velásquez-Mejía, Sierra, Corrales-Agudelo, Carmona, Abad and Escobar2019; Verhaar et al., Reference Verhaar, Collard, Prodan, Levels, Zwinderman, Bäckhed, Vogt, Peters, Muller, Nieuwdorp and van den Born2020). Studies that aim to reduce hypertension in humans by supplementing prebiotics or other dietary fibres would benefit from quantifying SCFAs to determine the extent to which they mediate the effect of these dietary interventions on blood pressure. These studies suggest that more research is needed to understand the role of hypertension in mood and cognition, and non-pharmacological interventions such as dietary fibre or SCFA supplementation may be ripe targets for such research.
Brain-derived neurotrophic factor
BDNF is a nerve growth factor found in the brain and in the periphery with an important function in normal neural development and in long-term memory. It is considered as a potential marker for neuronal integrity and brain functions. As central and peripheral BDNF are highly correlated in rats (r = 0.86) (Harris et al., Reference Harris, Lennen, Brydges, Jansen, Pernet, Whalley, Marshall, Baker, Basso, Day, Holmes and Hall2016), circulating levels of BDNF may be used as an appropriate measure for brain levels of BDNF. Dietary fibre may indirectly increase circulating BDNF levels via modification of the microbiota, in particular levels of Bifidobacterium and Lactobacillus. Increases in the relative abundance of these strains and consequent increases in BDNF levels were accompanied by a reduction in depressive symptoms as well as improvements in cognitive performance in both animal (Bercik et al., Reference Bercik, Verdu, Foster, Macri, Potter, Huang, Malinowski, Jackson, Blennerhassett, Neufeld, Lu, Khan, Corthesy-Theulaz, Cherbut, Bergonzelli and Collins2010, Reference Bercik, Park, Sinclair, Khoshdel, Lu, Huang, Deng, Blennerhassett, Fahnestock, Moine, Berger, Huizinga, Kunze, Mclean, Bergonzelli, Collins and Verdu2011) and human studies (Haghighat et al., Reference Haghighat, Rajabi and Mohammadshahi2019).
Dietary fibres have indeed shown to increase BDNF levels in both animals and humans, and some already found associations with improvements in cognition. For instance, in a rat study administering placebo, inulin, E. faecium, or E. faecium + inulin, BDNF levels only increased in the probiotic and synbiotic group, but not in the prebiotic group, with only the synbiotic group showing improved memory (Romo-Araiza et al., Reference Romo-Araiza, Gutiérrez-Salmeán, Galván, Hernández-Frausto, Herrera-López, Romo-Parra, García-Contreras, María Fernández-Presas, Jasso-Chávez, Borlongan, Ibarra, Kumar, Smulders and Yegla2018). Furthermore, in healthy subjects, an evening meal consisting of rye kernel bread increased plasma BDNF levels by 27 per cent at fasting on the next morning compared to white wheat bread (Sandberg et al., Reference Sandberg, Björck and Nilsson2018). Consumption of the same bread for three consecutive days was associated with a higher abundance of Prevotella compared to the white wheat bread (Prykhodko et al., Reference Prykhodko, Sandberg, Burleigh, Björck, Nilsson and Fåk Hållenius2018), and the Prevotella genus was positively associated with plasma levels of BDNF. Unfortunately, no plasma SCFAs were measured which prevented evaluating the relationship between SCFAs and BDNF. Despite evidence that butyrate increases acetylation around the promoters of BDNF, thereby increasing its transcription (Intlekofer et al., Reference Intlekofer, Berchtold, Malvaez, Carlos, Mcquown, Cunningham, Wood and Cotman2013) and the myriad pre-clinical studies showing that it increases following butyrate administration (Stilling et al., Reference Stilling, de Wouw, Clarke, Stanton, Dinan and Cryan2016), our recent study in healthy subjects showed that colonic administration of SCFAs did not alter serum BDNF levels (Dalile et al., Reference Dalile, Vervliet, Bergonzelli, Verbeke and Van Oudenhove2020). Together, the evidence thus far seems to indicate that the relationship between dietary fibre consumption and BDNF levels is mediated by changes in gut microbiota composition, rather than increases in colonic or circulating SCFAs.
Direct effects of dietary fibre on the immune system
Dietary fibre and immune system
Few studies explored immune effects of dietary fibres that are independent of the gut microbiota. A recent study demonstrated that dietary cellulose supplementation modulates the immune response. Mice were fed either a normal diet or a high-fibre cellulose diet for 2 weeks and were, subsequently, injected with endotoxin (LPS). Mice fed the high-fibre cellulose diet showed lower levels of proinflammatory cytokines (IL-1α, IL-1β, and IFN-α), lower numbers and activation of splenic macrophages and DCs, and hyporesponsiveness of T cells (Di Caro et al., Reference Di Caro, Cummings, Alcamo, Piganelli, Clark, Morowitz and Aneja2019). Splenic macrophages and DCs are innate effector cells that are crucial for the host defence to protect against bacterial infections (Di Caro et al., Reference Di Caro, Cummings, Alcamo, Piganelli, Clark, Morowitz and Aneja2019). Since cellulose is hardly fermentable, the observed effects likely arose from a direct interaction of the fibre with the immune system, rather than from SCFA production.
Also, other glucans, more specifically β-1,3/1,6-glucans, modulate the innate and acquired immune system. Those compounds bind to pattern recognition receptors including complement receptor 3, scavenger receptors, lactosylceramide, and dectin-1, which are expressed on cells of myeloid origin, including macrophages, dendritic cells, and neutrophils (Murphy et al., Reference Murphy, Davis and Carmichael2010). Although small amounts of β-glucan may be absorbed after oral administration, it is most likely that β-glucan primarily act in the gut epithelium. β-Glucan has been shown useful in the prevention of treatment of allergic disease. No studies to date have evaluated whether the impact of β-glucan on the immune system results in improvements in cognitive or affective processes.
Cholesterol
Cholesterol and cognitive and affective processes
Cholesterol is important for normal brain function and our mental well-being as well as cognition. However, its involvement is complex. Cholesterol demand by neurons in the brain is very high, since it is implicated in a variety of neuronal processes such as neurite formation and synaptic activity. Several studies found that higher levels of LDL cholesterol were associated with depressive mood in men (Kim et al., Reference Kim, Hong and Hwang2019; Tedders et al., Reference Tedders, Fokong, Mckenzie, Wesley, Yu and Zhang2011) whereas low levels of high-density lipoprotein (HDL) cholesterol were significantly associated with depression symptoms in women (Tedders et al., Reference Tedders, Fokong, Mckenzie, Wesley, Yu and Zhang2011) and men (Lehto et al., Reference Lehto, Niskanen, Tolmunen, Hintikka, Viinamäki, Heiskanen, Honkalampi, Kokkonen and Koivumaa-Honkanen2010), and is a potential risk factor for developing a mood disorder in females (Kim et al., Reference Kim, Han, Jang and Park2018). Up to 44 per cent of the subjects who were diagnosed with a mood disorder suffered from mild hypercholesterolemia (>5.2 and 6.2 mmol/L) and 21 per cent from hypercholesterolemia (>6.2 mmol/L) (Davison and Kaplan, Reference Davison and Kaplan2012). Importantly, dietary cholesterol cannot cross the blood brain barrier. Therefore, the mechanisms by which cholesterol influences learning and memory are thought to combine peripheral (atherogenic and proinflammatory) and central (accumulation of intracellular beta amyloid) effects.
There is some evidence that pharmacological reduction of plasma cholesterol with statins, the most common cholesterol-lowering drugs, may improve cognitive function in subjects without dementia (Schreurs, Reference Schreurs2010). However, the effects of statins on mood modulation remain controversial. For instance, chronic statin treatment in rats (10 mg/kg atorvastatin; 10 mg/kg simvastatin; 30 mg/kg pravastatin) reduced anxious behaviour in the open-field task (Citraro et al., Reference Citraro, Chimirri, Aiello, Gallelli, Trimboli, Britti, De Sarro and Russo2014; Wang and Gao, Reference Wang and Gao2009). Preclinical studies using the forced swimming test also support the anti-depressant effects of statin treatment (Can et al., Reference Can, Ulupınar, Özkay, Yegin and Öztürk2012). Another study demonstrated that statin treatment decreased the risk of depression (Yang et al., Reference Yang, Jick and Jick2003). In contrast, low dose statin treatment (simvastatin 1 mg/kg; atorvastatin 0.5 mg/kg) did not improve spatial memory and learning in guinea pigs, but instead increased levels of anxiety (Maggo et al., Reference Maggo, Clark and Ashton2012). Furthermore, some studies in humans found that depressive symptoms increased rather than attenuated after statin treatment (Lechleitner et al., Reference Lechleitner, Hoppichler, Konwalinka, Patsch and Braunsteiner1992; Morales et al., Reference Morales, Wittink, Datto, Difilippo, Cary, Tenhave and Katz2006). It is possible that lowering cholesterol induces positive effects on cognitive and affective processes by lowering blood pressure (see section “Hypertension”).
Dietary fibre and cholesterol
Soluble rather than insoluble fibres possess cholesterol lowering abilities in humans. The mechanism is most likely related to the viscous properties of soluble fibre. By increasing the viscosity in the small intestine, reabsorption of bile acids is reduced, in turn leading to increased de novo synthesis of bile acids from cholesterol in the liver and lower circulating cholesterol levels (Fuller et al., Reference Fuller, Beck, Salman and Tapsell2016). The role of β-glucan in lowering cholesterol has been extensively documented and led the U.S. Food and Drug Administration (FDA) to authorise the use of health claims on oat-containing products, stating that consumption of β-glucans at least 3 g per day lowers the risk of cardiovascular disease (Food and Drug Administration, 2019). These health claims have also been approved by the European Commission, as well as in various other jurisdictions (European Commision, 2011, 2012). A meta-analysis showed that also pectin, soluble fibre from psyllium, and guar gum have cholesterol-lowering abilities (Brown et al., Reference Brown, Rosner, Willett and Sacks1999). Viscous fibres should be utilised in intervention studies to test their effects of affective and cognitive processes, and if supported, investigate whether their cholesterol-lowering ability drives the effects on brain function in humans.
Conclusion
Collectively, studies suggest that dietary fibres may be promising in inducing psychological changes in cognitive and affective processes. The evidence base indicates that fermentable fibres, such as ITFs, β-glucans, pectin, and gums, are the strongest candidates to modulate these psychological functions. This is mainly due to their ability to modulate the microbiota and increase SCFA production. However, their ability to increase BDNF levels may also reinforce their effects on cognitive and affective processes. On the other hand, viscous fibres such as β-glucan and non-fermentable fibres such as cellulose may exert beneficial effects on cognitive and affective processes via microbiota-independent mechanisms such as lowering cholesterol and inflammation, respectively. Notably, a research gap remains, where the effects of different types of fibres on mood and cognition via the proposed mechanisms still needs to be investigated. In addition, while preclinical studies of dietary fibres on gastrointestinal mechanisms are ample, well-designed studies of dietary fibre effects on mood and cognition with simultaneous assessment of putative mechanisms of actions in humans are needed. The aim with this review was to outline potential additional microbiota-dependent and independent mechanism through which different fibre properties can act to affect affective and cognitive processes in order to give a more complete picture of the effects of dietary fibres and to help explain additional variance in inter-individual psychological responses to fibre consumption. The resolution of various mechanistic and functional links outlined here may better facilitate personalised nutrition for maintaining optimal psychological functioning in states of health or disease.
Acknowledgement
The authors would like to thank Francesca Zoppas for her helpful contribution to content of the review.
Data availability statement
Data sharing is not applicable to this article as no new data were created or analysed.
Notes on contributors
Danique La Torre is a PhD student in the Translational Research Center in Gastrointestinal Disorders (TARGID) at KU Leuven. Her research focuses on the interaction between stress and intestinal permeability, and dietary and physical interventions that could modulate this interaction.
Kristin Verbeke is a Pharmacist by training, and now a Full Professor and head of the Translational Research Center in Gastrointestinal Disorders (TARGID) at KU Leuven. She has published widely on the bacterial colonic metabolism in humans, including on the development of analytical methods to quantify bacterial metabolism as well as dietary intervention studies that aim to modulate the gut microbiome.
Boushra Dalile is a Junior Postdoctoral researcher at the Translational Research Center in Gastrointestinal Disorders (TARGID), KU Leuven. She conducts research within the gut-brain axis, mainly revolving around the role of the bacterial metabolites short-chain fatty acids in human stress sensitivity and anxiety.
Funding
The PhD of B.D. is financially supported by an unrestricted grant from Société des Produits Nestlé S.A.
Disclosure
The authors declare none.
Author contributions
Conceptualization, D.L.T., K.V., B.D.; Supervision, K.V., B.D.; Visualisation, B.D., D.L.T.; Writing – original draft, D.L.T., K.V., B.D.; Writing – review and editing, D.L.T., K.V., B.D. All authors have read an approved the final version of this manuscript.