1. Introduction
Emerging infectious diseases are a threat to world health, according to the World Health Organization (WHO). Over the years, infectious diseases have plagued humanity (Ebola, plague, Asian cholera, yellow fever, Spanish flu). However, the contribution of medical science is enormous, given that severe and infectious diseases like smallpox and polio have been eliminated or reduced, while at the same time there are positive developments for diseases such as malaria, tuberculosis, and HIV/AIDS (Gresham et al., Reference Gresham, Smolinski, Suphanchaimat, Kimball and Wibulpolprasert2013; Hays, Reference Hays2005). These achievements should not reassure the international community though, as new diseases emerge and deadly diseases of the past are re-emerging, causing epidemics and pandemics. But how do human activities relate to the emergence and spread of communicable zoonotic diseases? The present paper aims to highlight this existing relationship.
Humans with their activities invade the wild and disrupt the natural ecosystems, thus fueling the emergence of zoonotic diseases, as they come into direct contact with wild animals infected with viruses. These diseases rapidly spread to the human population through travel, trade, urbanization, migration, and human behavior, causing epidemics or pandemics that in turn result in human losses and huge social and economic implications. In the present study, the bidirectional relationship between human activities and epidemics/pandemics is highlighted through the multi-layered review of the COVID-19 pandemic (origin of SARS-CoV-2, clinical picture of COVID-19, modes of transmission, relationship of weather conditions and air pollution in the spread of disease, effects, and therapeutic developments).
2. Zoonoses: definitions, classification, and typology of zoonotic dynamics
2.1 Zoonosis
Disease or infection transmitted to humans by vertebrate animals (mammals, birds, reptiles, amphibians, and fish) that carry germs such as viruses, bacteria, fungi, and parasites (CDC, 2021).
2.2 Transmission of zoonoses
Transmission occurs through various ways (direct contact, indirect contact, carrier, consumption of contaminated food and water) and in multiple environments (urban centers, rural areas, trips, zoos, and open-air markets for the sale and consumption of wild animals) (Kock & Caceres-Escobar, Reference Kock and Caceres-Escobar2022; Wegner et al., Reference Wegner, Murray, Springmann, Muller, Sokolow, Saylors and Morens2022) (Table 1).
Table 1. Means of transmission of zoonoses

A dominant role in the transmission of zoonoses is played by ‘natural’ hosts that act as reservoirs of pathogens, such as various species of bats, rodents, and birds that are natural reservoirs of viruses (chanterelles, arenaviruses, arboviruses, and coronaviruses). A key link in the transmission chain is the ‘intermediate’ hosts (wild, domestic animals, and arthropods) through which pathogens can evolve and pass from the ‘natural’ hosts to humans. Zoonotic diseases are also transmitted to animals and humans through the bite of arthropod vectors (mosquitoes, ticks, fleas) (Wegner et al., Reference Wegner, Murray, Springmann, Muller, Sokolow, Saylors and Morens2022) (Tables 2 and 3; Figure 1).
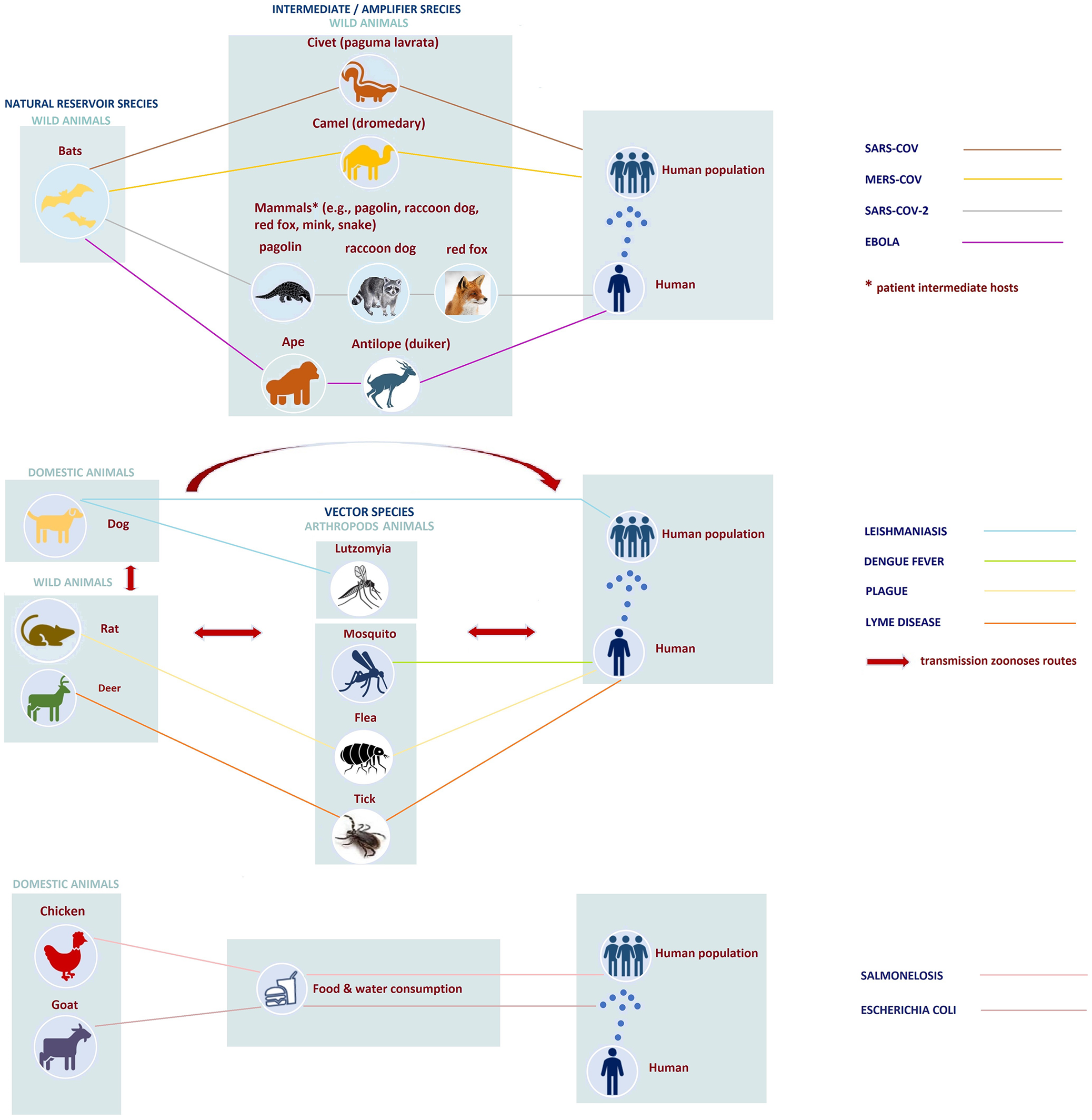
Figure 1. Schematic representation of transmission of zoonoses to humans.
Table 2. Transmission of zoonoses through direct and indirect contact with wild or domestic animals and consumption of contaminated water or food

a Potential intermediate hosts.
Table 3. Transmission of zoonoses through vector

2.3 Classification of zoonoses
Zoonoses are classified based on the causative agents (pathogens) responsible for their occurrence, the transmission cycle (orthozoonoses, cyclozoonoses, metazoonoses, saprozoonoses), and reservoir hosts anthropozoonoses, zooanthroponoses, amphixenoses, human diseases) (Kock & Caceres-Escobar, Reference Kock and Caceres-Escobar2022; Leal Filho et al., Reference Leal Filho, Ternova, Parasnis, Kovaleva and Nagy2022) (Table 4).
Table 4. Classification of zoonoses

2.4 Dynamics of zoonosis typology
The course of a zoonosis depends on the dynamics of the pathogen. Wolfe et al. (Reference Wolfe, Dunavan and Diamond2007) proposed a five-stage classification by which a pathogen that initially infects only animals (stage I) goes on to develop into a pathogen that infects only human populations (stage V).
In intermediate stages (II–IV) pathogens based on their behavior are divided into (a) pathogens transmitted from animals to humans causing ‘primary’ infections, but do not show secondary transmission from human to humans (stage II), such as West Nile virus or Brucella abortus, (b) animal-borne pathogens that spread to human populations, causing limited transmission cycles until extinction (stage III), such as Ebola, Marburg and monkeypox viruses, and (c) pathogens retained in animal tanks and can cause self-sustaining transmission chains in human populations (stage IV), such as Yersinia pestis (plague) and pandemic influenza.
Over the years pathogens shaped the ability to change behavior and while in the past some of them may have caused limited cycles of transmission in human populations until they disappeared (stage III), they have now re-emerged causing major epidemic outbreaks. An example is the Ebola virus that seems to have evolved into a pathogen that mainly infects human populations (stage V). Ebola has caused many epidemic outbreaks since 1976 when it first appeared, with the largest one in West Africa (2014–2016) and recently with the outbreak in Uganda affecting the country for the first time since 2012 (WHO, 2022c) (Figure 2).

Figure 2. Five stages through which pathogens cause disease in humans. Adapted from Wolfe et al. (Reference Wolfe, Dunavan and Diamond2007).
Similar behavior is shown by other viruses, such as that of monkeypox which was discovered in 1970 and has caused many epidemic episodes in the past. The virus strongly re-emerged in 2022, as it spread to many countries (mainly in Europe and America), outside the endemic areas of Africa, recording from the beginning of the year to October (26-10-2022) more than 76,500 cases and 36 deaths in 109 countries worldwide (WHO, 2022d).
3. Human activities and epidemics of zoonoses: a two-way relationship
The occurrence and transmission of zoonoses in the human population is related to the existence of several factors, of which human activities seem to play a decisive role. Deforestation for the purpose of residential and industrial activity and the development of agricultural crops, the trade and consumption of wild animals are some activities with which humans directly interfere with the natural environment, disrupting biodiversity and natural ecosystems.
A similar disturbance is caused by climate change (a result of human activities) and extreme weather events (heatwaves, floods, etc.), affecting the life cycles of pathogens and their vectors (insects, rodents, mammals), as well as their geographical allocation. Human either directly or indirectly with his activities disrupts the balance of wildlife and fuels the emergence of zoonotic diseases (Loh et al., Reference Loh, Zambrana-Torrelio, Olival, Bogich, Johnson, Mazet, Karesh and Daszak2015; UNEP, 2020) (Figure 3).

Figure 3. Impact of direct and indirect human activities on biodiversity and natural ecosystems.
These activities lead many animals that are potential carriers of pathogens to explore new natural habitats, thus forcing them closer to inhabited areas. Such is the case with some species of bats that manage to continue their life cycle and grow in new acceptable habitats such as humanized environments (Afelt et al., Reference Afelt, Lacroix, Zawadzka-Pawlewska, Pokojski, Buchy and Frutos2018; Plowright et al., Reference Plowright, Eby, Hudson, Smith, Westcott, Bryden, Middleton, Reid, Mc Farlane, Martin, Tabor, Skerratt, Anderson, Grameri, Quammen, Jordan, Freeman, Wang, Epstein and McCallum2015; Reuter et al., Reference Reuter, Wills, Lee, Cordes and Sewall2016). This increases the risk of infectious diseases as humans and pets come closer to wild animals carrying pathogens (Halliday & Rohr, Reference Halliday and Rohr2019; Karesh et al., Reference Karesh, Dobson, Lloyd-Smith, Lubroth, Dixon, Bennett, Aldrich, Harrington, Formenty, Loh, Machalaba, Thomas and Heymann2012; Keesing et al., Reference Keesing, Belden, Daszak, Dobson, Harvell, Holt, Hudson, Jolles, Jones, Mitcell, Myers, Bogich and Ostfeld2010).
In addition to human activities, microbial resistance to antibiotics, inadequate health systems, the lack of specialized health personnel, the poor quality of public health, the low level of health services provided, and the absence of preventive measures and infectious disease surveillance systems contribute to the increase in communicable diseases.
These emerging diseases due to travel, trade, urbanization, population migration, and human behavior rapidly spread across the planet, causing epidemics and pandemics (CDC, 1994), which sequentially affect people and their activities with great impact on public health, health systems, the economy, tourism, education, and society.
The relationship between human activities and epidemics/pandemics could be characterized as a two-way relationship. It is a chain process whose beginning and the end are human and his activities (Figure 4).

Figure 4. Two-way relationship between human activities and epidemics–pandemics.
4. Categorization of factors favoring the emergence and transmission of zoonotic diseases in humans
These factors could be distinguished into those that favor the emergence of zoonotic diseases (direct and indirect human activities, antimicrobial resistance to antibiotics) and those that contribute to their transmission to the human population (travel, trade, urbanization, human behavior) (Figures 5 and 6).

Figure 5. Factors of occurrence of zoonoses.

Figure 6. Factors of the global spread of zoonoses.
4.1 Factors that favor the appearance of zoonotic diseases
4.1.1 Human activities (direct)
A series of activities, such as deforestation, reforestation, changing land use for agriculture, urban and industrial development, exploitation-trade, and consumption of wild animals, directly affect the natural environment by contributing to the degradation of biodiversity and by disturbing the balance of wildlife.
Extensive deforestation in the Amazon, southeast Asia, Central and West Africa has been linked to the spread of animal-borne diseases. Human intervention within the rainforests of Central and West Africa is reportedly linked to Ebola outbreaks. Olivero et al. (Reference Olivero, Fa, Real, Márquez, Farfán, Vargas, Gaveu, Mohammad, Park, Suter, King, Leendertz, Sheil and Nasi2017) observed such outbreak of the disease in populated areas created by extensive deforestation between 2001 and 2014.
Reforestation, another form of ecosystem disruption, is responsible for the emergence of borreliosis in the United States and Europe. This reverse process has led to an increase in deer population. The Ixodes ricinus sea bream, which is responsible for transmitting the borreliosis disease, exists in deer's skin. Human activity in these areas has resulted in the direct contact of a large part of the population with the disease carrier (Fineberg & Wilson, Reference Fineberg and Wilson2010; Spielman, Reference Spielman1994).
The re-emergence of leishmaniasis in parts of Brazil is also related to the change of the use of the forest areas, where the natural trees are replaced by others suitable for the paper industry. This change in the natural ecosystem has favored the growth of foxes, through which the disease was transmitted to the human population (Patz et al., Reference Patz, Graczyk, Geller and Vittor2000).
Furthermore, the exploitation marketing and consumption of wild animal meat is associated with the occurrence of zoonotic diseases in humans. In China, for example, research has shown that both SARS-CoV (2002–2003) and SARS-CoV-2 coronaviruses, related to bat viruses, may have been transmitted to humans through wild animals (vectors: Paguma larvata Footnote 1 for SARS-CoV and probably pangolin,Footnote 2 raccoon dog, red fox, mink, snake, etc. for SARS-CoV-2) sold for consumption in popular markets in China (Mackenzie & Smith, Reference Mackenzie and Smith2020; Song et al., Reference Song, Tu, Zhang, Wang, Zheng, Lei, Chen, Gao, Zhou, Yiang, Zheng, Wang, Cheng, Pan, Xuan, Chen, Luo, Zhou, Liu and Zhao2005; Wong et al., Reference Wong, Javornik Cregeen, Ajami and Petrosino2020).
4.1.2 Human activities (indirect)
Human activities (burning fossil fuels, cutting down trees, forest fires) are a key factor in climate change, due to the increase in greenhouse gas emissions (CO2, N2O, CH4, and chlorofluorocarbons) into the atmosphere. The result of climate change is the rise of global warming and extreme weather events (floods, heatwaves, droughts).
Climate change contributes to the reduction of biodiversity, affects ecosystems, the life cycles of pathogens and vectors (insects, rodents, mammals), their geographical distribution, and enhances the occurrence of diseases.
On the one hand, temperature rise has been found to extend the geographical distribution of pathogen habitats (mosquitoes, ticks), causing shifts in infectious diseases such as malaria, dengue fever, yellow fever, and Lyme disease, from areas of lower latitude to higher altitudes. On the other, heavy rains and floods favor malaria and leptospirosis as environmental conditions (humidity, stagnant water) influence the female mosquito's reproduction, which is the primary vector of these diseases (Harvell et al., Reference Harvell, Mitchell, Ward, Altizer, Dobson, Ostfeld and Samuel2002).
In parts of Asia and South America, periodic floods due to El NinoFootnote 3 (ENSO) have been associated with malaria epidemics (Kovats et al., Reference Kovats, Bouma, Hajat, Worrall and Haines2003) like in the coastal region of northern Peru (Bayer et al., Reference Bayer, Danysh, Garvich, Gonzálvez, Checkley, Álvarez and Gilman2014) and in Brazil, where the malaria epidemic of 2000 may be due to high temperatures and heavy rainfall (Vasconcelos et al., Reference Vasconcelos, Costa, Travassos da Rosa, Luna, Rodrigues, Barros, Dias, Monteiro, Oliva, Vasconcelos, Oliveira, Sousa, Da Silva, Cruz, Martins and Travassos Da Rosa2001). Likewise, Karande (Reference Karande2003) believed that leptospirosis occurred in Mumbai, India after the 2000 floods.
Sunshine and warm temperatures combined favor the multiplication of pathogens as is the case with the bacterium Vibrio cholerae, the causative agent responsible for cholera (Islam et al., Reference Islam, Sharker, Rheman, Hossain, Mahmud, Islam, Uddin, Yunus, Osman, Ernst, Rector, Larson, Luby, Endtz and Cravioto2009).
Heatwaves, as a result of global warming, combined with prolonged periods of drought due to the El Nino phenomenon, make forests extremely flammable and vulnerable to catastrophic fires, which accelerate deforestation (Lenton et al., Reference Lenton, Held, Kriegler, Hall, Lucht, Rahmstorf and Schellnhuber2008; Nobre et al., Reference Nobre, Sampaio, Borma, Castilla-Rubio, Silva and Cardoso2016). Anthropogenic deforestation processes and fires work collaboratively to reduce biodiversity and disturbance of natural ecosystems.
4.1.3 Antimicrobial resistance to antibiotics and public health systems
Antimicrobial resistance to antibiotics combined with poor hygiene conditions in health facilities contribute to the appearance of infections. O'Neill (Reference O'Neill2014) has reached the conclusion that by 2050, approximately 10,000,000 people will die from antimicrobial resistance, while the cost to health systems will exceed 100 trillion dollars.
Similar estimates for public health and health systems are given in a recent report by 13 international organizations, which revealed the economic damage would be as catastrophic as the global financial crisis of 2008–2009, and by 2030, antimicrobial resistance could force up to 24 million people in extreme poverty (IAGG, 2019).
In addition, the risk of developing infectious diseases and causing epidemics increases especially in poor countries with limited health resources, such as sub-Saharan African countries. For example, the 2014 Ebola outbreak in West Africa quickly escalated into an epidemic due to inadequate public health infrastructure and logistical problems, lack of effective disease surveillance systems, hospital infection control, and contact tracing, isolation, and patient care (Buseh et al., Reference Buseh, Stevens, Bromberg and Kelber2015).
4.2 Factors contributing to the spread of zoonotic diseases
4.2.1 International travels and trade
International travels and trade have in the past contributed to the spread of infectious diseases in humans. Yellow fever was transmitted from Africa to America in the 16th–17th centuries during the transportation of black slaves (Hays, Reference Hays2005). The Asian cholera started in 1817 from the Ganges (Calcutta) plain and spread with merchant ships and British Navy ships, to the Middle East, Africa, and Europe. Additionally, the Spanish flu of 1918–1919, which appeared toward the end of World War I was transmitted worldwide by warships carrying troops (Vinet Freddy, 2020). In the recent past, the SARS-CoV epidemic (2002–2003) appeared to have spread to 31 countries through travel and trade; factors that are also responsible for the spread of SARS-CoV-2 coronavirus from China to all countries worldwide, causing the COVID-19 pandemic.
4.2.2 Intense urbanization
Intense urbanization, poor sanitation, and shortages of clean drinking water contribute to the growth of pathogens and the spread of infectious diseases. Dengue fever occurs in cities with tropical and subtropical climate, where the Aedes aegypti mosquito, a carrier of the virus, accumulates in piles of waste, in pots, and in open water storage containers, and infects humans (Fineberg & Wilson, Reference Fineberg and Wilson2010). Forecasts of increasing urbanization in the future are ominous and raise concerns about the development of new infectious diseases. It is estimated that by 2050, 66% of the world's population will live in cities and the total area of urban areas worldwide will increase by more than 1.5 million square kilometers by 2030 (Seto et al., Reference Seto, Güneralp and Hutyra2012).
4.2.3 Migration of populations
The migration of populations from countries with a high epidemiological burden is a means of invading infectious diseases in host countries where these diseases are not endemic. The recurrence of brucellosis in Germany in recent years is associated with increased migration flows from Turkey where the disease is endemic (Al Dahouk et al., Reference Al Dahouk, Neubauer, Hensel, Schöneberg, Nöckler, Alpers, Merzenich, Stark and Jansen2007). Also, Chagas disease, a parasitic disease endemic to Latin American countries, now appears in non-endemic areas such as Europe. It is estimated that 68,000–120,000 people carriers of the disease live in European countries and that 30% may develop gastrointestinal disorders (Requena-Méndez et al., Reference Requena-Méndez, Aldasoro, de Lazzari, Sicuri, Brown, Moore, Gascon and Muñoz2015; Troy et al., Reference Troy, Rickman and Davis2005).
4.2.4 Human behavior
Human behavior (sexual activity, intravenous drug use, etc.) contributes to the development of sexually transmitted diseases such as HIV/AIDS, hepatitis, and tuberculosis (Lederberg et al., Reference Lederberg, Shope and Oaks1992).
A typical example is a COVID-19 pandemic, where human behavior is directly related to the spread of the disease. When protective measures are applied (mask use, social distancing) then the disease recedes, while when these are circumvented, the virus spreads faster in the human population.
5. Two-way relationship between human activities and zoonotic diseases in the case of the COVID-19 pandemic
The COVID-19 pandemic caused by the coronavirus SARS-CoV-2 that is currently affecting the planet is a much typical example of the two-way relationship between human activities and zoonotic epidemics/pandemics, as shown in Figure 7.
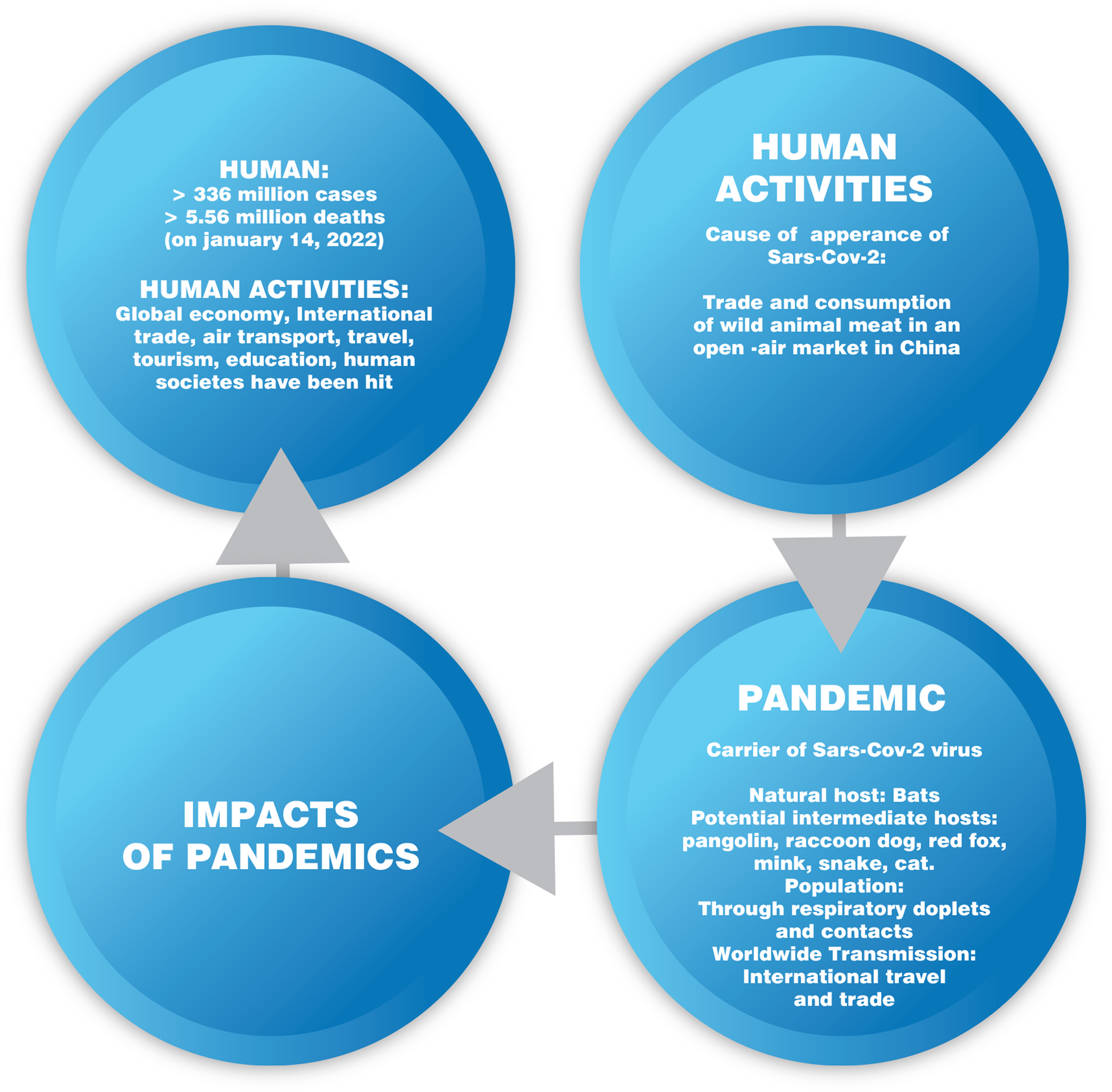
Figure 7. Two-way relationship between human activities and COVID-19 pandemic.
5.1 Occurrence of the SARS-CoV-2 in 2019 in China and the COVID-19 pandemic
5.1.1 Natural and intermediate host
The SARS-CoV-2 coronavirus, appeared in December 2019 in China, belongs to the same family (Coronaviridae-b) of the SARS-CoV and MERS-CoV coronaviruses that caused epidemics in 2002 and 2012, respectively (Docea et al., Reference Docea, Tsatsakis, Albulescu, Cristea, Zlatian, Vinceti, Moschos, Tsoukalas, Goumenou, Drakoulis, Dumanov, Tutelyan, Onischenko, Aschner, Spandidos and Calina2020; Mousavizadeh & Ghasemi, Reference Mousavizadeh and Ghasemi2021). It has a positive polarity RNA genome, encodes a non-structural replicase polyprotein and four structural proteins (spike S, envelope E, membrane M, and nucleocapsid N), and it uses the S protein, which is located on its surface to enter the host cells (Carfì et al., Reference Carfì, Bernabei and Landi2020; Datta et al., Reference Datta, Talwar and Lee2020; del Rio et al., Reference del Rio, Collins and Malani2020).
The high sequence identity of the two coronavirus genes (96.2% for Rhinolophus affinis – RaTG13 and 93.3% for Rhinolophus malayanus – RmYN02) of bats suggests that bats may be the natural reservoir of SARS-CoV-2 (Chan et al., Reference Chan, To, Tse, Jin and Yuen2013; Nguyen et al., Reference Nguyen, Abdelrazek, Nguyen, Aryal, Nguyen, Reddy, Nguyen, Khatami, Nguyen, Hsu and Yang2022; Ren et al., Reference Ren, Wu, Guo, Yao, Wang, Xiao, Pisco, Wu, Lei, Liu, Shi, Han, Zang, Xiao, Zhong, Wu, Li, Quake, Huang and Wang2020; Zhou et al., Reference Zhou, Chen, Hu, Li, Song, Liu, Wang, Liu, Yang, Holmes, Hughes, Bi and Shi2020a, Reference Zhou, Yang, Wang, Hu, Zhang, Zhang, Shi, Zhu, Li, Huang, Chen, Chen, Luo, Gao, Jiang, Liu, Chen, Shen, Wang and Shi2020b). Although direct infection of humans with bat viruses has not yet been documented, it could, however, occur in mixed unsanitary environments such as bazaars, where people mix with domestic or wild animals that carry viruses and exchange them with each other, making them more contagious, and easier to be transmitted to humans (Menachery et al., Reference Menachery, Yount, Debbink, Agnihothram, Gralinski, Plante, Graham, Scobey, Ge, Donaldson, Randell, Lanzavecchia, Marasco, Shi and Baric2015).
Human interaction with bats or any other wild animal (intermediate host) may be the cause of transmission of SARS-CoV-2 to humans, as the first confirmed cases of SARS-CoV-2 coronavirus were associated with patients, who developed severe pneumonia after visiting the Wuhan seafood and wildlife flea market. The intermediate host of SARS-CoV-2 may be related to some species of pangolins from Malaysia or Bangladesh. Researchers have identified coronavirus (CoV pangolin) of the same family (Coronaviridae-b) as SARS-CoV-2 and found a close genetic relationshipFootnote 4 (Xiao et al., Reference Xiao, Zhai, Feng, Zhou, Zhang, Zou, Li, Guo, Li, Shen, Zhang, Shu, Huang, Li, Zhang, Shu, Huang, Li, Zhang and Shen2020) and a high similarity of the viral sequenceFootnote 5 between the two coronaviruses (Lam et al., Reference Lam, Jia, Zhang, Shum, Jiang, Zhu, Tong, Shi, Ni, Liao, Li, Jiang, Wei, Yuan, Zheng, Gui, Li, Pei, Qiang and Cao2020; Liu et al., Reference Liu, Jiang, Wan, Hua, Li, Zhou, Wang, Hou, Chen and Chen2020; Zhang et al., Reference Zhang, Wu and Zhang2020b).
In addition to the pangolin, other animals in the Wuhan flea market may have been infected with SARS-CoV-2 and transmitted the virus to humans, such as raccoon dogs and red foxes (Worobey et al., Reference Worobey, Levy, Malpica Serrano, Crits-Christoph, Pekar, Goldstein, Rasmussen, Kraemer, Newman, Koopmans, Suchard, Wertheim, Lemey, Robertson, Garry, Holmes, Rambaut and Andersen2022).
According to Shi et al. (Reference Shi, Wen, Zhong, Yang, Wang, Huang, Liu, He, Shyai, Sun, Zhao, Liu, Liang, Cui, Wang, Zhang, Guan, Tan, Wu, Chen and Bu2020) and Kim et al. (Reference Kim, Kim, Kim, Kim, Park, Yu, Chang, Kim, Lee, Casel, Um, Song, Jeong, Lai, Kim, Chin, Park, Chung and Choi2020) minks are highly sensitive to SARS-CoV-2 and prone to infection.
An example is the appearance of SARS-CoV-2 on mink farms in DenmarkFootnote 6 (Enserink, Reference Enserink2020; Oreshkova et al., Reference Oreshkova, Molenaar, Vreman, Harders, Oude Munnink, Hakze-van der Honing, Gerhards, Tolsma, Bouwstra, Sikkema, Tacken, de Rooij, Weesendorp, Engelsma, Bruschke, Smit, Koopmans, van der Poel and Stegeman2020; Oude Munnink et al., Reference Oude Munnink, Sikkema, Nieuwenhuijse, Molenaar, Munger, Molenkamp, VanDer Spek, Tolsma, Rietveld, Brouwer, Bouwmeester-Vincken, Harders, Hakze-VanDer Honing, Wegdam-Blans, Bouwstra, Geurtsvankessel, VanDer Eijk, Velkers, Smit and Koopmans2021), in Spain, Sweden, Lithuania, Greece,Footnote 7 Italy, and the United States (WHO, 2020b). Other research has shown that the snakes might also be potential vectors, where SARS-CoV-2 could recombine and acquire the ability to infect humans (Ji et al., Reference Ji, Wang, Zhao, Zai and Li2020). Similarly, the potential susceptibility of domestic pets was also investigated (cats, dogs, pigs, chickens, ducks) in SARS-CoV-2 infection due to their close contact with humans. According to Shi et al. (Reference Shi, Wen, Zhong, Yang, Wang, Huang, Liu, He, Shyai, Sun, Zhao, Liu, Liang, Cui, Wang, Zhang, Guan, Tan, Wu, Chen and Bu2020), cats are very susceptible to SARS-CoV-2; dogs have low susceptibility, while pigs, chickens, and ducks are not susceptible to the virus.
5.1.2 COVID-19 disease caused by SARS-CoV-2 and transmission among the human population
Symptoms and clinical picture of COVID-19 disease. COVID-19 is a disease of the respiratory system with mild-to-very severe symptoms (cough, myalgia, fever, fatigue, and shortness of breath). The clinical picture of the disease ranges from asymptomatic or mild respiratory infection to uncontrolled pneumonia with acute respiratory distress syndrome, multiorgan failure, and death (Guan et al., Reference Guan, Ni, Hu, Liang, Ou, He, Liu, Shan, Lei, Hui, Du, Li, Zeng, Yuen, Chen, Tang, Wang, Chen, Yiang and Zhong2020; Huang et al., Reference Huang, Wang, Li, Ren, Zhao, Hu, Zhang, Fan, Yu, Gu, Cheng, Yu, Xia, Wei, Wu, Xie, Yin, Li, Liu and Cao2020; Wang et al., Reference Wang, Hu, Hu, Zhu, Liu, Zhang, Wang, Xiang, Cheng, Xiong, Zhao, Li., Wang and Peng2020). Complications following the acute phase of the infection, such as rare multisystem inflammatory disease in children and adults, 2–5 weeks after initial infection (Datta et al., Reference Datta, Talwar and Lee2020) are also identified, mainly from the cardiovascular and gastrointestinal systems. The manifestations of those complications were dermatological and mucosal, resembling the Kawasaki disease in children.
Modes of transmission of SARS-CoV-2 and the cause of the pandemic. The SARS-CoV-2 virus is mainly transmitted mainly by respiratory droplets produced by persons infected with the virus (Dhand & Li, Reference Dhand and Li2020) through human contact, or by touching infected surfaces (van Doremalen et al., Reference van Doremalen, Bushmaker, Morris, Holbrook, Gamble, Williamson, Tamin, Harcourt, Thornburg, Gerber, Lloyd-Smith, de Wit and Munster2020). Asymptomatic carriers also contribute to the transmission of the disease (Zhao et al., Reference Zhao, Lu, Deng, Tang and Lu2020), while fecal transmission is rare (Zuo et al., Reference Zuo, Uspal and Wei2020). It however, needs further investigation, since the virus has been detected in fecal samples of symptomatic and asymptomatic patients (Gao et al., Reference Gao, Chen and Fang2020; Zhang et al., Reference Zhang, Li, Zhang, Wang and Molina2020a). Also, in some patients who were free of respiratory symptoms, the virus was still present in their feces (Wu et al., Reference Wu, Guo, Tang, Hong, Zhou, Dong, Yin, Xiao, Tang, Qu, Kyang, Fang, Mishra, Lu, Shan, Jiang and Huang2020a).
Close monitoring of wastewater has been shown to contribute to the early detection of SARS-CoV-2, the identification of the true magnitude of the pandemic, and the design of appropriate measures to prevent the transmission of the disease (Mallapaty, Reference Mallapaty2020; Medema et al., Reference Medema, Heijnen, Elsinga, Italiaander and Brouwer2020). The disease is highly contagiousFootnote 8 as it has been proven since the beginning of the pandemicFootnote 9 until today.
Effect of weather and air pollution on the spread and mortality of COVID-19 disease. The effect of weather on the spread and mortality of COVID-19 disease has been the subject of many studies. Some have shown that the rate of spread of the disease decreased with increasing temperature and humidity (Chatziprodromidou et al., Reference Chatziprodromidou, Apostolou and Vantarakis2020; Wang & Di, Reference Wang and Di2020).
Research in 166 countries (Wu et al., Reference Wu, Jing, Liu, Ma, Yuan, Wang, Wang, Du and Liu2020b) showed that the increase in temperature (+1°C) and relative humidity (+1%) was associated with a decrease in daily epidemiological data.Footnote 10 Similarly, according to Qi et al. (Reference Qi, Xiao, Shi, Ward, Chen, Tu, Su, Wang, Wang and Zhang2020) daily incidenceFootnote 11 in China decreased significantly when the average daily temperature and relative humidity increased. The critical temperature value leading to a decrease in the rate of exponential transmission of the disease was also studied and it was found that at an ambient temperature of 30°C, the basal rate of reproduction (R 0) was about 1, while it increased significantly (R 0 ≅ 2.5) when the temperature reached 0°C (Livadiotis, Reference Livadiotis2020).
However, according to Paraskevis et al. (Reference Paraskevis, Kostaki, Alygizakis, Thomaidis, Cartalis, Tsiodras and Dimopoulos2021), climatic conditions alone cannot lead to a reduction in cases and prevent the emergence of new outbreaks of COVID-19, in the absence of public health protection measures. Other studies also reached this conclusion when they incorporated public health protection measures into their research models (Jüni et al., Reference Jüni, Rothenbühler, Bobos, Thorpe, da Costa, Fisman, Slutsky and Gesink2020; Oliveiros et al., Reference Oliveiros, Caramelo, Ferreira and Caramelo2020).
Many researchers have focused on the possible relationship between air pollution and COVID- 19 mortality. According to Conticini et al. (Reference Conticini, Frediani and Caro2020), high mortality from COVID-19 (>12%) in northern Italy (Lombardy, Emilia-Romagna, Piedmont) was associated with high levels of air pollution and increased rates of population aging. Ogen's (Reference Ogen2020) study found that very high mortality rates (83%) in northern Italy and Madrid, Spain were associated with high concentrations of carbon dioxide (>100 μmol/m2).
A similar conclusion was reached by studies (Travaglio et al., Reference Travaglio, Yu, Popovic, Selley, Leal and Martins2021) for the urban areas in England (London, Midlands) and for the United States (Wu et al., Reference Wu, Jing, Liu, Ma, Yuan, Wang, Wang, Du and Liu2020b), which highlighted the relationship between long-term exposure of the human population to harmful particulate matterFootnote 12 (PM2.5) and mortality risk from COVID-19.
The pandemic from its outbreak until today has had a huge impact on health and all areas of human activities. The impact was spectacular within the first year of the pandemic, as the virus was unknown, and there were no treatments to prevent and suppress COVID-19. Two years later, the pandemic continues to plague the world's population as the virus mutates and becomes more contagious. But societies are gradually opening, and human activities are returning to more normal levels, as they now have more knowledge about the virus, there are vaccines, medicines, early detection methods, and tried and tested crisis management policies.
5.2 Public health and health systems
5.2.1 Infections and deaths from COVID-19, mortality rates, and excess mortality
Public health has been hit hard by the COVID-19 pandemic, with millions of cases and deaths worldwide (553.77 million confirmed cases, 6.35 million confirmed deaths, as of July 7, 2022). The regions most affected are Europe and the Americas, followed by Asia, Africa, and Oceania (Our World in Data, 2022).
However, this image is not representative of the real situation. An estimate of the pandemic's health footprint could be derived from the case fatality rate, which translates as the ratio between confirmed deaths and cases, but this approach also appears to be precarious. The actual impact of the pandemic may differ greatly from the picture that emerges from the reported data (infections and deaths). The reasons for this difference may be due to limited controls, the way cases and deaths are recorded by each country, and the problems of assigning the cause of death. The countries of the African continent, for example, are characterized by inadequate health systems and incomplete infectious surveillance structures. According to the WHO, the measurement of excess mortality is the parameter that can essentially contribute to understanding the impact of the pandemic and to the comparison of mortality estimates between countries, even when data are incomplete or unavailable. The first estimate of excess mortality emerged under an innovative methodology developed by the WHO and the United Nations and showed that it was approximately 14.9 million between January 1, 2020 and December 31, 2021 (WHO, 2022b).
5.2.2 SARS-CoV-2 variants and the waves of the pandemic
Two and a half years since the start of the pandemic, the SARS-CoV-2 virus, in its attempt to survive, is constantly mutating and appearing with new variants which, according to the WHO, are distinguished into variants of interest (VOI) and variants of concern (VOCs). Some of them caused strong waves of infections, with the main characteristics being high transmissibility, increasing virulence, and reducing the effectiveness of therapeutic methods and vaccines (Table 5).
Table 5. Variants of SARS-CoV-2

The prevailing variant of concern today is Omicron (B.1.1.529) which appeared in November 2021 and between June 10 and July 10, 2022 (WHO, 2022a) accounting for 84% of viral sequences. In the past, four other VOCs (Alpha, Beta, Gamma, and Delta) have emerged, causing less or equally significant impact on global public health. The Alpha variant (B.1.1.7) mainly affected Europe, the United Kingdom where it appeared in September 2020, North America, Asia, and to a lesser extent Oceania and South America. The Beta (B.1.351) that appeared in South Africa in May 2020 mainly affected Africa, as did the Gamma (P.1) that appeared in Brazil in 2020 and affected South America. Particularly aggravating was the contribution of the Delta variant (B.1.617.2) to public health (GISAID, 2022). The variant first appeared in India in October 2020 and affected all continents equally, causing a strong wave of infections and deaths. By October 2021 it was the dominant variant accounting for 90% of viral sequences (GISAID, 2022; WHO, 2022a) (Table 6) .
Table 6. Spread of VOCs by continent

Alpha affected all regions of the world differently.
Beta significantly affected only Africa.
Gamma significantly affected only South America.
Delta significantly affected all regions of the world.
Omicron dominates in all regions of the world.
5.2.3 Health systems
In the first months of the pandemic, the resilience of health systems was shaken. Many countries, underestimating the risk of the disease (e.g. United States) or adopting the theory of ‘herd immunity’ (e.g. United Kingdom, Netherlands, Belgium, Luxembourg, Sweden), did not take immediate precautionary and restrictive measures (Doumas et al., Reference Doumas, Imprialos, Patoulias, Katsimardou and Stavropoulos2020).
In contrast, countries that adopted timely measures such as lockdown and large-scale case detection tests have succeeded in reducing the spread of the disease. According to Morris and Schizas (Reference Morris and Schizas2020), the time between the onset of the first confirmed case per million population and the imposition of the quarantine measure proved to be a critical factor.
Greece, a country with the lowest funded health system (8.45% of GDP) in Europe, imposed an immediate (within 6 days) general lockdown and in 1 month had only 63 deaths and 1613 confirmed cases. On the other hand, the delay in imposing the lockdown (13–18 days), contributed to the rapid spread of the disease, in countries (Italy, Spain) with gross public health costs, like Greece. In the first 30 days, Italy recorded 63,927 cases and 6077 deaths, while Spain recorded 87,956 cases and 7716 deaths.
The countries of East Asia with the immediate imposition of a general lockdown significantly reduced the spread of the disease in Europe and North America. China, for example, imposed a lockdown 1 day after the first confirmed case per million population, resulting in 1-month mortality at just 1.86 per million population. In the United States, on the other hand, unprecedented chaos occurred and the world's most prepared country for infectious disease management, according to the Global Health Insurance Index (Nalabandian et al., Reference Nalabandian, O'Brien, League, Ravi, Meyer, Snyder, Mullen and Warmbrod2019), was hit hard.
According to WHO (2020a), significant disruptions were caused to health services provided which were reduced, suspended, or discontinued (emergency: 22%, emergency procedures: 19%, blood transfusions: 23%, suspension of dental care, outpatient follow-up: 76%, routine examinations: 70%, diagnostic treatments: cancer 55%, mental health 61%, non-communicable diseases 69%, malaria 46%, tuberculosis 42%, antiretroviral therapy 32%, family planning and contraception 8%).
5.3 Global and European economy
The COVID-19 pandemic has led the world economy into a recession which, according to the World Bank, is unique as it is the first to be caused exclusively by a pandemic in the last 150 years, but also deeper than that of World War II.
According to the Organization for Economic Co-operation and Development (OECD), world GDP shrank by 4.2% in 2020, while the global economy is expected to recover in the next 2 years (OECD, 2020b). Government debt has risen to almost 100% of GDP due to the huge costFootnote 13 (IMF, 2020) of policies to rescue national economies and reduce tax revenues (OECD, 2020a).
In Europe, the pandemic negatively affected all indicators of the European economy (GDP, unemployment, public debt). According to the European Commission (2020), the economies of the EU and the euro area countries shrank by 7.4 and 7.8%, respectively, in 2020 and will gradually recover over the next 2 years (2021: +4.1% and +4.2% respectively, 2022: +3%).
Unemployment has risen dramatically, and it is estimated that 88–115 million people will be plunged into extreme poverty, mainly in South Asia and sub-Saharan Africa (Blake & Wadhwa, Reference Blake and Wadhwa2020).
In Europe, the figures in 2020 and 2021 are overwhelming (EU: 7.7% in 2020, 8.6% in 2021, euro area: 8.3% in 2020 and 9.4% in 2021) while the expected improvement in 2022 will lag pre-pandemic levels (EU: 8.0 vs. 6.7% in 2019, euro area: 8.9 vs. 7.5% in 2019). Deficits and public debt will also increase due to increased expenditures and reduced tax revenues (euro area deficit: 0.6% in 2019, 8.8% in 2020, 6.4% in 2021 and 4.7% in 2022, debt to GDP ratio: 85.9% in 2019, 101.7% in 2020, 102.3% in 2021 and 102.6% in 2022) (European Commission, 2020).
5.4 Global air transport
The impact of the pandemic on air transport is enormous, according to the International Civil Aviation Organization (ICAO). An impact unprecedented in historical chronicles, compared to past crises (oil crisis 1973, Iran–Iraq war 1980–1988, Gulf War 1990–1991, the financial crisis in Asia in 1997, terrorist attack on September 11, 2001, SARS epidemic 2002–2003, financial crisis 2007–2008).
In 2020, air passenger seats offered for international and domestic travel fell by 50% compared to 2019, which corresponds to 2.699 million fewer passengers, while airlines recorded losses of $371 billion in gross revenue. For the first quarter of 2021, the estimates translate into a reduction of the offered passenger seats from 26 to 45% which corresponds to a total reduction of 393–635 million passengers, and the loss in gross revenue will amount to 57–90 billion dollars (ICAO, 2021).
5.5 Global tourism industry
In 2020, travel restrictions stopped tourism around the world. In the first 8 months, international tourist arrivals fell by 70% and revenue losses skyrocketed to $730 billion, eight times higher than that in the global financial crisis of 2009.
The picture of international arrivals in the northern hemisphere gradually improved in July (−81%) and August (−79%), compared to the first half (decrease >90%) of the year (UNWTO, 2020). The viability of mainly small- and medium-sized enterprises and jobs was greatly affected (OECD, 2020a). According to the World Travel and Tourism Council (WTTC, 2020), 142.6 million jobs were lost worldwide. Cruise lines lost $50 billion in revenue and 334,000 jobs from mid-March to the end of September (CLIA, 2020), while the impact on travel agents was also significant. In the first half of 2020, the travel group TUI reported revenue losses of 75 million euros (TUI GROUP, 2020), and Booking Holdings recorded an 87% decrease in revenue from overnight stays, compared to the corresponding period of 2019 (Booking Holdings, 2020).
The tourism industry will recover from the third quarter of 2021 as predicted by the World Tourism Organization, while the OECD estimates that this will happen by 2022 and return to pre-epidemic levels, not before 2023 (OECD, 2020c).
5.6 World trade
In 2020, trade in goods and services was adversely affected by border closures and cross-border restrictions. In the first half, trade in products decreased by 14.3% compared to the same period in 2019, while the decline in trade in services reached 23%, well above the 9% recorded during the financial crisis of 2009. Impacts were higher in Europe (exports: −24.5%, imports: −19.3%) and North America (exports: −21.8%, imports: 14.5%), compared to Asia (exports: −6.1%, imports: −7.1%). However, not all categories of tradable products and services were equally affected. The sectors of the automotive industry (−70%), fuel and mining products (−38%), industrial (−19%), and agricultural (−5%) products showed large reductions. In contrast, by September 2020, trade in telecommunications equipment products increased by 9% compared to the corresponding period of 2019, due to teleworking and tele-education, while the increase in Personal Protective Equipment (PPE) trade was spectacular (122% in May and 92% in the second quarter of 2020) (WTO, 2020). In September 2020, travel services (−68%), construction (−16%), and arts and entertainment (−14%) recorded a large decline, compared to the corresponding period of 2019, while they increased legal, administrative, accounting services, financial (+2%), and advertising services (+1%) (WTO, 2021).
5.7 Food security and nutrition
Severe disruptions occurred in food supply chains due to business closures, high production, and low demand, inadequate storage facilities, restrictions on basic foodstuffs (rice, wheat) from exporting countries, and high levels of contamination among workers. These disturbances affected food availability, prices, and product quality (Barrett, Reference Barrett2020). In Thailand, Vietnam, and the United States the price of rice increased by 32, 25, and 10%, respectively, between February and April 2020 (Katsoras, Reference Katsoras Angelo2020).
Imports of agricultural products (seeds, fertilizers) were also affected, and these products became rarer and more expensive in countries such as China and West Africa (Arouna et al., Reference Arouna, Soullier, Mendez del Villar and Demont2020; Pu & Zhong, Reference Pu and Zhong2020).
In many poor countries, social protection programs (school mealsFootnote 14) stopped with the closure of schools. About 370 million children lost access to these programs, worsening the financial situation of poor households (Moseley & Battersby, Reference Moseley and Battersby2020). Malnutrition is projected to increase, as an additional 83–132 million people will face food insecurity as a direct result of the pandemic, of which 38–80 million will come from poor countries dependent on food imports (Torero, Reference Torero2020).
5.8 Education
The closure of schools and universities during the first wave of the pandemic affected 94% of the global student population (1.6 billion children and young people) in more than 190 countries worldwide. To ensure continuity in the educational process, distance home education was implemented. Pre-existing problems in education worsened and inequalities widened. According to UNICEF (2020) at least 463 million students worldwide did not have access to distance education without the necessary technological equipment, a suitable home learning environment, and the required parental support.
Similarly, higher education shifted to online teaching, with many foreign students returning to their homeland, thus losing many of the benefits of international mobility (OECD, 2020a). University institutions in Australia, Canada, the United Kingdom, and the United States that mostly rely on international students were severely affected, as tuition funds account for a large share of their revenue and is a major source of funding for academic research (OECD, 2020d).
5.9 Society and psychology of citizens
The measures of social isolation, and closure of businesses and activities, affected people's social relationships and daily life, endangering their mental and emotional health. According to Fountoulakis et al. (Reference Fountoulakis, Apostolidou, Atsiova, Filippidou, Florou, Gousiou, Katsara, Mantzari, Padouva-Markoulaki, Papatriantafyllou, Sacharidi, Tonia, Tsagalidou, Zymara, Prezerakos, Koupidis, Fountoulakis and Chrousos2021), during the first lockdown in Greece in the period from April 11 to May 1, 2020, daily life was greatly affected, such as physical activity, exercise, appetite, sleep, increased need to strengthen relationships within the family (increased need for communication >40%, emotional support 24.16%, and improvement in the quality of relationships 24.6%), while the conflicts mainly concerned families with children more vulnerable in confinement and with difficult management behavior.
Separation from loved ones, loss of freedom, uncertainty about the course of the disease (Cao et al., Reference Cao, Fang, Hou, Han, Xu, Dong and Zheng2020; Li & Wang, Reference Li and Wang2020), restriction of movement, loss of social contacts, employment, income, and fear of disease transmission, resulted in the appearance of psychological disorders (stress, depression, suicidal behaviors) (Kawohl & Nordt, Reference Kawohl and Nordt2020). Also, loneliness due to social isolation adversely affected the mental and physical health mainly of the elderly and people with disadvantaged socioeconomic status and physical and mental illness (Druss, Reference Druss2020).
Increased psychological disorders were found in children, students, and healthcare staff. According to Orgilés et al. (Reference Orgilés, Morales, Delvecchio, Mazzeschi and Espada2020) children aged 3–18 years from Italy and Spain had 76.6% difficulty concentrating, 52% boredom, 39% irritability, 38.8% anxiety and nervousness, and 31.3% feeling of loneliness. Anxiety symptoms developed in students in China due to the slowdown in their academic activities (Alvarez et al., Reference Alvarez, Argente and Lippi2020), their living in urban areas, the financial situation of their families (Cao et al., Reference Cao, Fang, Hou, Han, Xu, Dong and Zheng2020), and the infection of their relatives or friends.
Health personnel showed symptoms of depression (50%), anxiety (44.6%), insomnia (34%), and risk (71.5%) (Lai et al., Reference Lai, Ma, Wang, Cai, Hu, Wei, Wu, Du, Chen, Li, Tan, Kang, Yao, Huang, Wang, Wang, Liu and Hu2020). Their interpersonal relationships were severed for fear of transmission to their family and friends (Brooks et al., Reference Brooks, Webster, Smith, Woodland, Wessely, Greenberg and Rubin2020) and many developed secondary traumatic stress disorder (Zaffina et al., Reference Zaffina, Camisa, Monducci, Vinci, Vicari and Bergamaschi2014) when asked to choose which patients to be admitted to the intensive care unit (Rana et al., Reference Rana, Mukhtar and Mukhtar2020; Roden-Foreman et al., Reference Roden-Foreman, Solis, Jones, Bennett, Roden-Foreman, Rainey, Foreman and Warren2017).
6. Therapeutic developments in the treatment of the pandemic
From the beginning of the pandemic, the scientific community used all the drugs in its quiver to treat patients with COVID-19 as they did not have effective vaccines to treat the disease. The US Food and Drug Administration (FDA) and the European Medicines Agency (EMA) have approved a variety of drugs (COVID19 Vaccine Tracker, 2022a; EMA, 2022) that have been tested to treat SARS-CoV-2 infection, pneumonia, multiples organ dysfunction, and thrombolysis, such as antiviral, anti-inflammatory drugs used to treat other diseases (rheumatoid arthritis), anticoagulants, and monoclonal antibodies (Table 7).
Table 7. Medicines that have received emergency approval

Research for new treatments resulted in the development of specialized vaccines less than a year after the pandemic broke out, some of which were approved by international and national health organizations. On January 14, 2022, there are 174 candidate vaccines, 557 ongoing vaccines in trials, 33 approved vaccines, and vaccination campaigns in many countries around the world (COVID19 Vaccine Tracker, 2022a).
Depending on the mechanism they use to activate the human immune system, vaccines are divided into two categories: (A) complex virus vaccines and (B) whole virus vaccines (Table 8).
Table 8. Categories of vaccines and mechanism of human immunization

Of the 33 approved vaccines, 13 belong to the protein subunit vaccine class, 10 to an inactivated virus, 6 to the non-replicating virus vector, 3 to mRNA, and 1 to DNA. Three vaccines have been approved and administered in the United States, while there are five approved vaccines in the European Union. Pfizer-BioNTech (BNT162b2), Moderna (mRNA-1273), and Johnson & Johnson (Ad26.COV2.S) approved vaccines from the US FDA are available in the United States.
In the countries of the European Union, in addition to the above three vaccines, the vaccines from AstraZeneca (AZD1222) and Novovax (Nuvaxovid, Covovax, NVX-CoV23) are also administered, which are also approved by the EMA (EMA, 2022; FDA, 2022).
In addition to Europe and America, other countries have developed vaccines against COVID-19 and have launched systematic vaccination campaigns (COVID19 Vaccine Tracker, 2022a), such as China, Japan, Taiwan, India, Iran, Russia, Turkey, Kazakhstan, Cuba, and Australia. These vaccines are also given in other countries such as Arab, Asian, South American, and some European countries.
Developed and some developing countries have started systematic vaccinations and have vaccinated hundreds of thousands or even millions of citizens since the end of December 2020.
In the Americas, Europe, Asia, and Oceania by July 7, 2022, rates of fully vaccinated citizens exceed 60% (South America: 76%, Asia: 70.78%, Europe: 65.87%, North America: 63.98%, Oceania: 62.43%) and of those who have received at least one dose are over 65% (South America: 84.7%, Asia: 75.98%, Europe: 68.82%, North America: 73.05%, Oceania: 65.16%) (Our World in Data, 2022) (Figure 8).

Figure 8. Vaccination rates by continent on July 7, 2022.
In the African continent, the picture is disappointing, as the percentage of fully vaccinated is only 19.53% and those who have received one dose at 25.08% (Our World in Data, 2022). Nonetheless, even this very low vaccination coverage does not represent all countries on the continent. There are countries with rates of fully vaccinated citizens below 10% (Congo: 2.4%, Madagascar: 4.2%, Cameroon: 4.5%, Mali: 6.3%, Burkina Faso: 7%, Somalia: 9.9%, Sudan: 9.9%) and countries with rates exceeding 50% (Morocco: 63.2%, Rwanda: 64.9%, Botswana: 58.4%, Tunisia: 52%) (COVID19 Vaccine Tracker, 2022b).
The picture is bleak as the People's Vaccine Alliance report of October 2021 shows that rich countries are accumulating large stocks of doses and failing to meet their commitments to poor countries, while pharmaceutical companies are exploiting their monopolies to gain enormous wealth (Malpani & Maitland, Reference Malpani and Maitland2021).
On the one hand, the report shows that the G7Footnote 15 and the Group of Europe (EU plus Iceland and Norway) have delivered 261 million of the promised 1.8 billion tranches to low- and middle-income countries, while more than 10 million have been disbursed to other high-income countries, such as Canada and the United Kingdom, which received approximately 1.5 million doses of vaccine from COVAXFootnote 16 in 2021.
On the other, these two countries have broken their promises, as the United Kingdom has delivered only 9.6% and Canada 8% of the promised installments. Similarly, other countries are failing to meet their commitments, such as Germany delivering only 12% of the promised 100 million installments, France only 9% of the 120 million installments, and the United States 16% of the 1.1 billion installments (WTO, 2021). Pharmaceutical companies also promote vaccines in rich countries and do not meet their commitments to COVAX and the vaccine acquisition trust (VAT).Footnote 17
In 2021, four major pharmaceutical companies (AstraZeneca, Pfizer/BioNTech, Moderna, and Johnson & Johnson) provided a total of 47 times more installments in high-income countries than in low-income countries. Rich countries representing only 16% of the world's population bought about 49% of these companies vaccines, while in poor countries through COVAX and AVAT mechanisms not even 50% of the agreed doses were delivered (Table 9).
Table 9. Agreed supply and deliveries of doses of vaccines by Johnson & Johnson, AstraZeneca, Pfizer/BioNTech, and Moderna, to COVAX and AVAT (for 2021)

Vaccination of the world population is two-speed. Rich countries already promote third- or fourth-dose vaccines (Israel) in their populations and vaccinations in adolescents and children, whereas in poor countries, due to lack of vaccines, high-risk population groups (e.g. elderly and public health workers) have not been vaccinated (WHO, 2021). In the Americas, Asia, Europe, and Oceania the percentage of the population that has received a booster dose of vaccines exceeds 28% (Asia: 28.98%, North America: 37.26%, Oceania 38.16%, Europe 41.30%, South America: 48.48%), while in Africa it reaches only 2.26% (Our World in Data, 2022)
As the pandemic spreads to every corner of the globe, equitable access to vaccines and treatments for all of humanity is the only answer to the global health crisis. The more vaccinated the world's population is, the less likely the virus is to spread, mutate, and make existing vaccines less effective (Williams & Burgers, Reference Williams and Burgers2021).
7. Discussion and conclusions
Humans' relationship with pandemics follows a two-way path. Humans, through their activities, disturb the environment and natural ecosystems and come into direct contact with wild animals that carry pathogens, resulting in their infection with zoonoses and the occurrence of epidemics–pandemics. The effects of pandemics have as the final recipient the human and his activities.
‘We are invading the tropical forests, the wild nature where so many species of plants and animals live and with them so many viruses unknown to us. We cut down the trees, kill the animals or capture them and send them to the markets. We disrupt ecosystems and drive viruses out of their natural hosts, making them search for new ones. Well, we usually become their new home’, characteristically states (Quammen, Reference Quammen2012), in his book ‘Spillover: Animal Infections and the Next Pandemic’.
The COVID-19 pandemic is a prime example of this two-way relationship. Human activities (trade–sale and consumption of wild animals) in the open market of the city of Wuhan in China, probably contributed to the creation of a favorable environment for the transmission of the SARS-CoV-2 from bats (natural host) to humans, probably through a wild animal-intermediate host (pangolin, raccoon dog, red fox, mink, snake, etc.). The virus was easily transmitted to the human population through respiratory droplets and human contact and spread across the globe through aviation, travel, and trade, causing the COVID-19 pandemic. The pandemic since its inception has caused enormous impacts on public health, health systems, the global economy, transportation, trade, tourism, education, and human societies.
The global medical and scientific community since the beginning of the pandemic used all the therapeutic means at its disposal to relieve symptoms and treat patients, developing safe and effective vaccines and continuing research to produce new vaccines and drugs for the control and mitigation of the pandemic.
The ideal scenario would be the eradication of the pandemic from every corner of the planet as happened in the past with the eradication of smallpox and poliomyelitis. However, this scenario is not certain to be repeated in the case of the pandemic, as shown by the behavior of the virus to date (mutations, the emergence of new variants, causing successive waves of infections). Humanity will have to learn to coexist with a controlled virus as it does with other viruses (flu virus). By increasing the vaccination coverage of the global population, the chances of mitigating the dynamics of the virus and its ability to mutate into something more powerful, spread, and affect the effectiveness of vaccines are enhanced. The stake is high and will only be won if country leaders, pharmaceutical companies, and international and national health organizations work together to ensure fair and equal access to vaccines and treatments for all people.
In addition to this bet, the pandemic brought to the surface other important issues that humanity needs to face, such as the protection of the natural environment, the elimination of illegal wildlife trade, and the prevention of zoonotic diseases. The answer to these questions can only be given if bold decisions are taken in the context of global cooperation by adopting policies to preserve and restore natural ecosystems, impose strict control regulations on the movement, trade, and consumption of wild animals, and develop modern zoonotic surveillance systems.
The benefit of these decisions would be very large, comparing the estimated cost of prevention policies ($22–31.2 billion) with the economic impact of the pandemic ($16 trillion by the end of 2021) if vaccines will prove effective in pandemic control. The costs of prevention strategies could be reduced even further ($17.7–26.9 billion) if the benefits of reducing deforestation are accounted for (IPBES, 2020).
Today many financial possibilities exist, although the question is whether the resources will be distributed in interventions not only for the recovery and resilience of national economies but also in prevention policies, which would significantly reduce the risk of a new pandemic.
Supplementary material
The supplementary material for this article can be found at https://doi.org/10.1017/sus.2022.18.
Author contributions
D.D.T., P.T.N., and C.T. wrote the original draft.
Financial support
This research received no specific grant from any funding agency, commercial, or not-for-profit sectors.
Conflict of interest
The authors declare no conflict of interest.