1. Introduction
The Zagros fold–thrust belt (ZFTB; Koyi, Reference Koyi1988) formed owing to convergence and collision between the northeastern part of the Arabian plate and Central Iran/Eurasia, with associated SW-directed obduction of ophiolitic material derived from Neo-Tethyan oceanic crust during Late Cretaceous time (Berberian & King, Reference Berberian and King1981). North-northeastward movement of the Arabian plate relative to Central Iran during Late Cretaceous and later times resulted in the formation of the fold–thrust belt, which has since migrated southwestwards (Hessami et al. Reference Hessami, Koyi, Talbot, Tabasi and Shabanian2001). Different estimates for the onset of deformation in the ZFTB have been proposed (e.g. Falcon, Reference Falcon1967; Stöcklin, Reference Stöcklin1968; Wells, Reference Wells1969; Ricou, Reference Ricou1970; Nowroozi, Reference Nowroozi1972; Haynes & McQuillan, Reference Haynes and McQuillan1974; Alavi, Reference Alavi1980, Reference Alavi2004; Alavi & Mahdavi, Reference Alavi and Mahdavi1994; Berberian & King, Reference Berberian and King1981; Ni & Barazangi, Reference Ni and Barazangi1986).
Geological evidence has led early workers to suggest that emplacement of ophiolites and collision of Arabia and Central Iran did not occur simultaneously; they suggest that obduction, as one of the mechanisms for emplacement of ophiolites, did occur sometime in Late Cretaceous time (Berberian & King, Reference Berberian and King1981; Ziegler, Reference Ziegler2001) before the final closure of the Tethys Ocean that occurred in Oligocene–Miocene time (Koop & Stoneley, Reference Koop, Stoneley, Kent, Bott, McKenzie and Williams1982).
In general, the sedimentary record shows that the Late Cretaceous represents a period of major change in the area of the present-day Zagros foreland basin (Loutfi, Baslaib & Abu Hamd, Reference Loutfi, Baslaib and Abu Hamd1987; Burchette, Reference Burchette, Simo, Scott and Masse1993; Droste & Steenwinkel, Reference Droste, Van Steenwinkel, Eberli, Massaferro and Sarg2004; Farzadi, Reference Farzadi2005, Reference Farzadi2006a). Rapid thickness variations and facies changes in Albian and Cenomanian sedimentary rocks, which have been documented both from seismic data and in the field, have been related to the reactivation of deep-seated pre-existing N–S-trending normal and strike-slip faults (Koop & Stoneley, Reference Koop, Stoneley, Kent, Bott, McKenzie and Williams1982; Sepehr & Cosgrove, Reference Sepehr and Cosgrove2004; Sherkati & Letouzey, Reference Sherkati and Letouzey2004).
This paper documents the presence of two angular unconformities within the Zagros Imbricate Zone (i.e. the highly deformed zone immediately SW of the Zagros Suture) (Figs 1, 2) of the northwestern part of the ZFTB in the Kurdistan Region. The aim of the present study is to indicate the temporal and spatial beginning of the deformations that are associated with obduction of ophiolites upon the northern margin of Arabia.

Figure 1. (a) Simplified geological map of the Qandil Mountain area in the Kurdistan Region showing the Lower Cretaceous–Paleocene angular unconformity which is located between outcrops of the Qulqula Radiolarian Formation and Red Bed Series (modified from Sissakian, Reference Sissakian1997). Cross-section A–A′ is shown in Figure 8. (b) Names of areas and towns that are mentioned in the text, X1 – SE of Mawat town, X2 – south of Chwarta town. (c) Simple tectonic map of the studied area showing the tectonic zone of the Kurdistan Region in NE Iraq (Jassim & Goff, Reference Jassim and Goff2006).
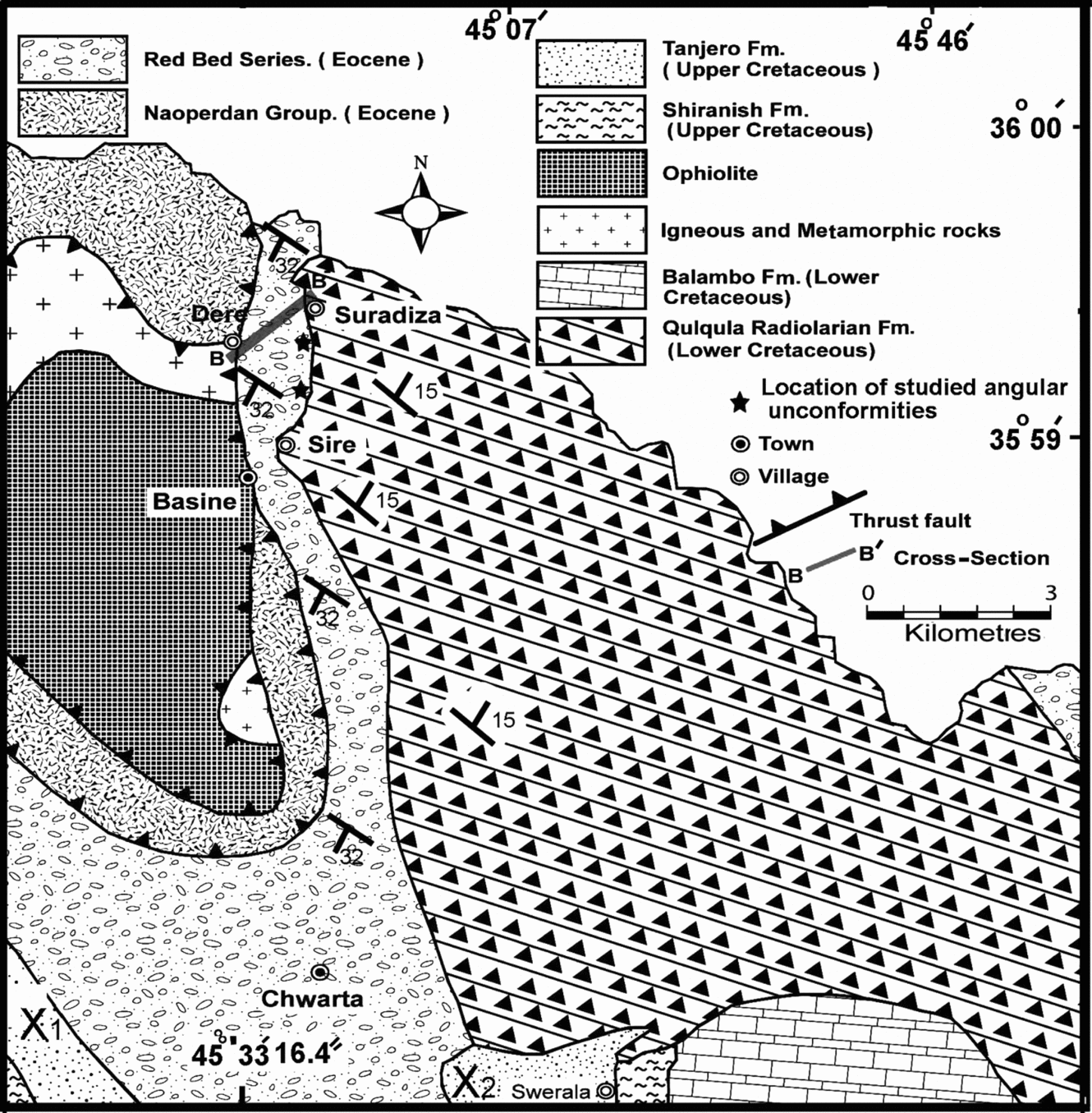
Figure 2. Geological map of the Chwarta area showing the location of the Lower Cretaceous–Paleocene angular unconformity (black stars) (Modified from Sissakian, Reference Sissakian2000). Cross-section B–B′ is shown in Figure 10. X1 and X2 are locations of outcrops of red beds.
1.a. Timing of deformation
A range of different timings has been suggested for both the obduction and collision phases of the Zagros orogeny. Some of these differences may arise from imprecise data and/or erroneous interpretation. However, some variations should perhaps be expected, given the lengthy evolution of the orogen and its complex tectonic history. Glennie (Reference Glennie, Alsharhan and Scott2000) proposed that the Neo-Tethys began to close with Early Cretaceous (Aptian?) subduction of oceanic crust beneath Central Iran. A major relative sea-level fall, attributed to a compressional event in Late Aptian time, is recognized as an unconformity throughout the Middle East (Harris et al. Reference Harris, Frost, Seiglie, Schneidermann and Schlee1984; Sharland et al. Reference Sharland, Archer, Casey, Davis, Halls, Heward, Horbury and Simmons2001). Considerable crustal-scale subsidence occurred on the NE margin of the Arabian plate between Turonian and Maastrichtian times (Sherkati & Letouzey, Reference Sherkati and Letouzey2004). Radiometric dating (Haynes & Reynolds, Reference Haynes and Reynolds1980) confirms a syn-post-Cenomanian emplacement age. Berberian & King (Reference Berberian and King1981) suggested a Santonian–Maastrichtian age for the emplacement of ophiolites, radiolarites and turbidites. Koop & Stoneley (Reference Koop, Stoneley, Kent, Bott, McKenzie and Williams1982) indicated truncation and onlap on a pre-Late Cretaceous unconformity (Middle Turonian, dated at 92 Ma). The Late Cretaceous has also been proposed as the time of obduction of the oceanic crust onto the Arabian plate by Alavi (Reference Alavi1994), Haynes & McQuillan (Reference Haynes and McQuillan1974) and Berberian & King (Reference Berberian and King1981). Sediments were deposited within a compressional foreland basin setting following onset of Mid-Turonian ophiolite obduction along the eastern margin of the plate (Ziegler, Reference Ziegler2001). Ziegler (Reference Ziegler2001) suggested that the pre-Cenozoic unconformity at 63 Ma (Sharland et al. Reference Sharland, Archer, Casey, Davis, Halls, Heward, Horbury and Simmons2001) marks the cessation of ophiolite obduction along the eastern margin of the Arabian plate. Beydoun (Reference Beydoun, MacNiocaill and Ryan1999) proposed that obduction in the form of an ophiolite belt took place as a result of a collision between the leading edge of the NE Arabian passive margin and an oceanic ridge (or possibly an island arc system) during Campanian time (c. 75 Ma).
Different timings, on the other hand, have been proposed for the continental collision between Arabia and Central Iran/Eurasia. Takin (Reference Takin1972) suggested that the earliest compressional movements of Zagros-related deformation occurred in Turonian to Campanian times. Collision has been suggested to have occurred during Eocene (Numan, Reference Numan1997, Reference Numan2001; Numan & Al-Azzawi, Reference Numan and Al-Azzawi2002; Al-Qayim, Reference Al-Qayim2000); Eocene–Oligocene (Hempton, Reference Hempton1987; Beydoun, Hughes Clarke & Stoneley, Reference Beydoun, Hughes Clarke, Stoneley, MacQueen and Leckie1992; Hooper et al. Reference Hooper, Baron, Agah, Hatcher and Al-Husseini1994); Oligocene–Miocene (Koop & Stoneley, Reference Koop, Stoneley, Kent, Bott, McKenzie and Williams1982); Late Miocene (McQuarrie et al. Reference McQuarrie, Stock, Verdel and Wernicke2003; Stoneley, Reference Stoneley1981); and Early Pliocene (Falcon, Reference Falcon, Kent, Satterthwaite and Spence1969) times. More recently, on the basis of magnetostratigraphic studies, Homke et al. (Reference Homke, Verges, Garces, Emamia and Karpuzc2004) investigated the timing and duration of the growth of the frontal folds of the Pusht-e Kuh arc in Lurestan Province, Iran, and concluded that if the deformation migrated following a foreland sequence, the deformation in the hinterland of the Simply Folded Belt must have started before 8.1–7.2 Ma and well before the generally accepted Late Pliocene maximum folding phase (Haynes & McQuillan, Reference Haynes and McQuillan1974; Stöcklin, Reference Stöcklin1968; Hamzepour, Paul & Wiesner, Reference Hamzepour, Paul and Wiesner1999). However, Alavi (Reference Alavi2007) published a tectonic map of the Late Maastrichtian–Early Paleocene period in which it is shown that during this time continental collision of some parts of the Iranian and Arabian plates occurred and the Neo-Tethys closed.
Alavi (Reference Alavi2004) summarized the tectonic evolution of the Zagros orogen in terms of three sequential phases: (1) subduction of Neo-Tethyan oceanic crust beneath the Iranian plate during Early to Late Cretaceous time; (2) obduction of slivers of Neo-Tethyan oceanic crust onto the Afro-Arabian massive continental margin in Late Cretaceous time (Turonian to Campanian) (Takin, Reference Takin1972; Stöcklin, Reference Stöcklin1977); and (3) convergence and collision of the Afro-Arabian and Iranian plates in Late Maastrichtian time (Berberian & King, Reference Berberian and King1981).
2. Materials and methods
This work is mainly based on the geological mapping and stratigraphic relationships of the Cretaceous–Tertiary outcrops in the Kurdistan Region, where most probably the first uplift of the Zagros orogenic belt occurred. During the fieldwork four outcrop sections were analysed, which are (from NW to SE) the Suwais, Qulqula Gorge, Do Awan and Basine sections. Stratigraphic units are recognized palaeontologically and lithologically through preparation of 100 thin-sections. A new geological map is drawn by introducing major modifications to that of Sissakian (Reference Sissakian2000) (Figs 1a, 2); two cross-sections and four stratigraphic columns are drawn across the angular unconformities (Fig. 3). On these figures the dip, strike and temporal relationships of the units are shown. Two conglomerate successions are studied, which are related to the unconformities in the Imbricate Zone, and the attitude of their imbricated pebbles are measured and plotted on stereonets (Fig. 4) to indicate palaeocurrent direction (palaeo-sediment transport direction). Our observations are compared to the recent sequence stratigraphic and palaeontological studies by Karim (Reference Karim2006a), M. Baziany (unpub. M.Sc. thesis, Univ. Sulaimani, Iraq, 2006) and Ghafor & Baziany (Reference Ghafor and Baziany2009). In the distal areas (basin and slope of the Zagros foreland basin), the equivalents of these conglomerates (in time and according to lateral facies changes) consist of sandstone and calcareous shales. These conglomerates (as the main materials of this study) and their equivalents are mapped in the field and in thin-sections.
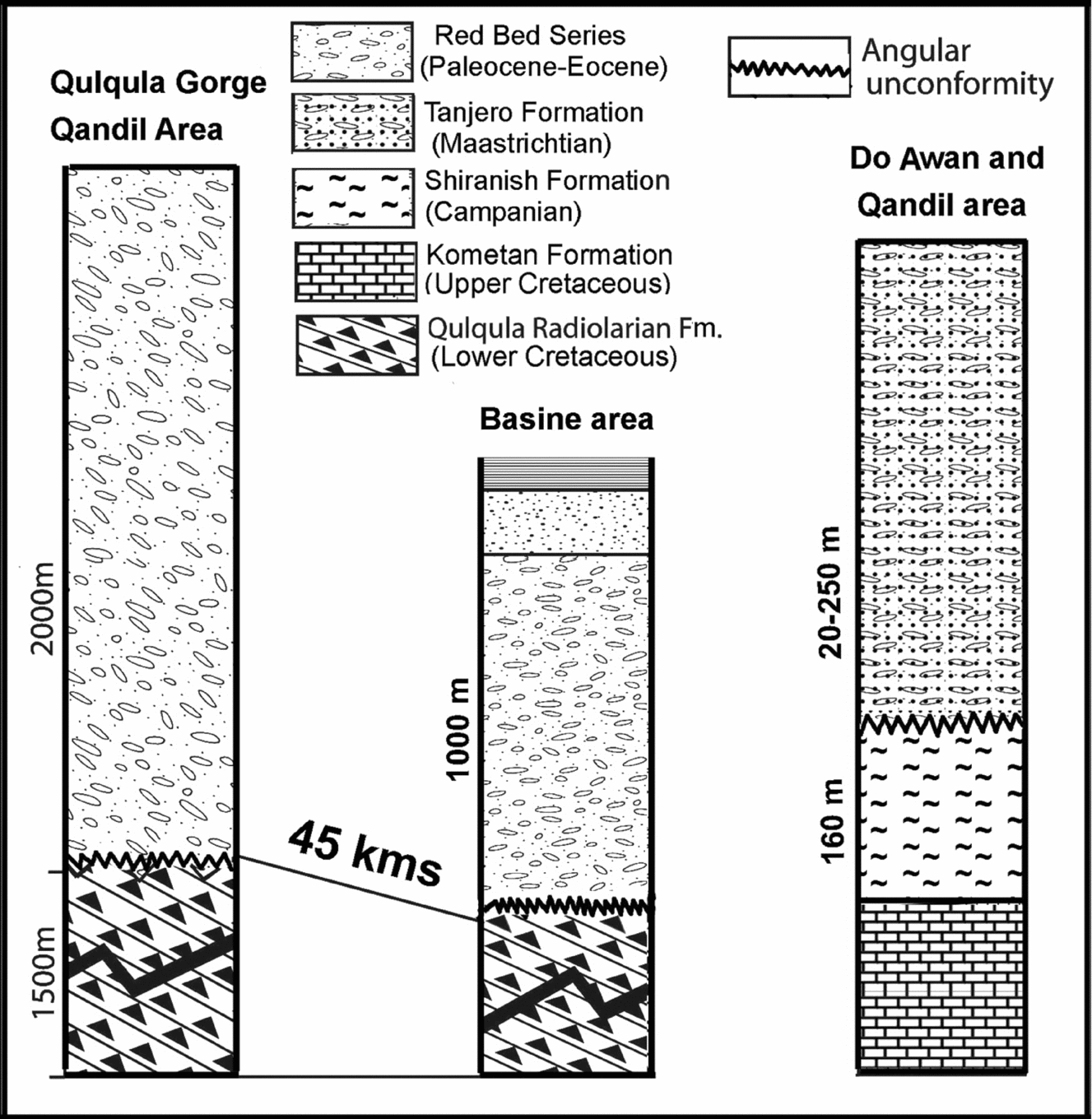
Figure 3. Lithological column of the studied areas showing the stratigraphic positions of the angular unconformities.

Figure 4. Imbricated pebbles of Red Bed Series. (a) Qulqula Gorge section. (b) Same structure at the Basine section. (c) Imbricated pebbles of Tanjero Formation east of Chwarta town. The black lines are showing bedding planes to which the imbricated pebbles make acute angles against palaeocurrent direction. (d) Stereonet of the poles of the imbricated pebbles of the Red Bed Series showing SW palaeocurrent direction.
3. The nature of the unconformities
Two main conglomerates are recognized in this study. The first conglomerate belongs to the Lower Maastrichtian Tanjero Formation and is about 500 m thick. This unit is related to an unconformity referred to here as the Campanian–Maastrichtian unconformity (CMU) and is located between the Campanian Shiranish Formation and the Maastrichtian Tanjero Formation (Fig. 3). These units crop out in the Kurdistan Region (Iraq), in a narrow strip close to the Iran–Iraq border within the Imbricate Zone (Buday, Reference Buday1980; Buday & Jassim, Reference Buday and Jassim1987; Sissakian, Reference Sissakian1997) (Fig. 1b, c). The rocks of this zone are thrust southwestward over the Arabian plate and subdivided into several minor zones such as the Shalair, Penjween–Walash and Qulqula–Khuwakurk zones (Jassim & Goff, Reference Jassim and Goff2006). The second conglomerate belongs to the Paleocene–Eocene Red Bed Series and is about 1000 m thick, and is related to a first unconformity, referred to as the Cretaceous–Tertiary unconformity (CTU). This unconformity is located between the Lower Cretaceous Qulqula Radiolarian Formation and the Paleocene–Eocene Red Bed Series (Fig. 5). In the next Sections, we will describe these unconformities in detail.

Figure 5. Close-up field photograph and line drawing showing an angular unconformity between the Qulqula Radiolarian Formation and Red Bed Series of Basine (north of Chwarta town) (For location, see Fig. 2).
3.a. Cretaceous–Tertiary unconformity (CTU)
The Qulqula Radiolarian Formation consists of more than 2000 m of alternating intervals of bedded cherts, calcareous shales (Fig. 6) and limestones (mostly siliceous). The thin-section of the chert shows only recrystallized circular radiolarians (Fig. 6a), while the limestone consists of pelletal-bioclastic limestone (Fig. 6b). According to Buday (Reference Buday1980) and Karim (Reference Karim2003), the precise age and stratigraphic position of the Qulqula Radiolarian Formation is unclear because of its complex structures. Waddington (Reference Waddington1955) proposed that the formation is older than Jurassic. However, Karim (Reference Karim1975) inferred an age of Albian to Cenomanian from foraminiferal data, while Buday (Reference Buday1980) and Jassim & Goff (Reference Jassim and Goff2006) proposed that the formation is Lower Cretaceous.

Figure 6. (a) Siliceous limestone of the Qulqula Radiolarian Formation at the Qulqula Gorge section showing content of radiolarian fossils (circular white spots). (b) Bioclast-peloidal limestone of same formation and the same section. (c) Alveolina ovulum (U. Paleocene), equatorial section, Qulqula Gorge section. (d) Nummulites perforatus (L. Eocene), axial section, Qulqula Gorge section.
The Paleocene–Eocene Red Bed Series of the second unconformity consists of more than 1500 m of red claystones, sandstones and conglomerates. This conglomerate contains about 10% limestone clasts (pebbles), which contain Late Paleocene and Eocene foraminifera. The depositional trend and direction of the sediment transport is tested by palaeocurrent analysis of imbricated pebbles, which indicate a SW sediment transport direction (Fig. 4). The Red Bed Series is interpreted to have been deposited in a foreland basin (K. H. Karim, unpub. Ph.D. thesis, Univ. Sulaimani, Iraq, 2004; S. T. M. Al-Barzinjy, unpub. Ph.D. thesis, Univ. Sulaimani, Iraq, 2005). Al-Barzinjy (unpub. Ph.D. thesis, Univ. Sulaimani, Iraq, 2005) considered that both the Red Bed Series and the Paleocene Kolosh Formation were deposited in a single large-scale foreland basin, which covered most of Iraq during Tertiary time. In the Chwarta, Mawat and Qandil areas (Kurdistan Region) (Figs 1, 2), the Red Bed Series is underlain by the Qulqula Radiolarian Formation (Lower Cretaceous) (Figs 3, 5); while to the SW, it is underlain by the Tanjero Formation (Maastrichtian). The contact between the Red Bed Series and the Tanjero Formation is gradational (conformable) (Lawa, Al Karadakhi & Ismail, Reference Lawa, Al Karadakhi and Ismail1998). However, Karim (unpub. Ph.D. thesis, Univ. Sulaimani, Iraq, 2004) described this contact to be gradational in some places and sharp (unconformable) in others. Thus, the contact is gradational at an outcrop SE of Mawat (35°45′47.5″N, 45°29′50.5″E), whereas the contact is unconformable 3 km south of Chwarta (Fig. 1b).
Field observations, in the present study, showed that an angular unconformity between the formations is present at two localities. The first (36°20′35″N, 45°10′11″E) is at Qulqula Gorge near Qandil Mountain, about 20km NE of Raniya (Figs 7, 8). The second location (35°55′10″N, 45°34′36″E) is 15 km NE of Chwarta and 3 km north of Basine (Figs 5, 9, 10, 11). The difference in bedding dip between the Qulqula Radiolarian Formation and Red Bed Series ranges between 17° and 21° at the two localities (Figs 5, 7, 8). At both localities, the Upper Cretaceous Kometan, Shiranish and Tanjero formations are entirely absent.

Figure 7. Outcrop photographs showing the angular unconformity between the limestones (a) and cherts (b) of the Qulqula Radiolarian Formation and the overlying conglomerates of the Red Bed Series. Location: left side of Qulqula Gorge, Kurdistan Region, Iraq.

Figure 8. Schematic cross-section of the Qulqula Gorge along the left side of Marado valley, showing the angular unconformity between the Lower Cretaceous Qulqula Formation and the overlying Eocene Red Beds Series. See location of A–A′ in Figure 1.

Figure 9. Field photograph of the angular unconformity between the Qulqula Radiolarian Formation and the overlying Red Bed Series in the Basine area (location in Fig. 2).

Figure 10. Geological cross-section of the Basine area, passing through Suradiza and Dere villages showing the angular unconformity.

Figure 11. General geological map of northeastern Iraq showing outcrops of the Campanian–Maastrichtian units (Shiranish and Tanjero formations) (modified from Sissakian, Reference Sissakian2000).
In most places, the unconformity surface is covered, but where observable, it is sharp and more or less undulating (Fig. 5). Efforts to observe or photograph a clear surface were not successful owing to the relative softness or friability of the stratigraphic units that bound the surface as mentioned above. The Qulqula Radiolarian Formation contains some siliceous limestone, which is resistant to karstification; therefore, signs of this process are not observed.
3.b. The Campanian–Maastrichtian unconformity (CMU)
The Campanian Shiranish Formation consists of about 200 m of bluish-white marls and marly limestones. It consists of deep marine facies (hemipelagite), which contain abundant Late Cretaceous planktonic foraminifera (see Bellen et al. Reference Bellen, Dunnington, Wetzel and Morton1959). It does not contain any sedimentary structures except for bedding; therefore, the depositional trend cannot be known directly. But it can be estimated by palaeocurrent direction from the Tanjero Formation (see below). This is because the contact between the two formations is conformable in all sections (see Buday, Reference Buday1980; Jassim & Goff Reference Jassim and Goff2006). However, recently Karim & Surdashy (Reference Karim and Surdashy2005b) and Karim (Reference Karim2006a) concluded that the contact is conformable except for in a few sections in coastal areas of the Late Cretaceous Zagros foreland basin, which are unconformable. Two of these sections are the Do Awan and Suwais village sections where the CMU is located and studied.
The Maastrichtian Tanjero Formation consists of about 400 m of sandstones in distal areas to the south of the Sulaimani and Dokan areas (Bellen et al. Reference Bellen, Dunnington, Wetzel and Morton1959; Buday, Reference Buday1980; Jassim & Goff, Reference Jassim and Goff2006), while it consists of about 500 m of indurate conglomerates in more proximal areas around the Chwarta, Mawat and Qandil areas (Fig. 1b) (Karim, Reference Karim2006a, unpub. Ph.D. thesis, Univ. Sulaimani, Iraq, 2004). In these areas, the conglomerate was tested by palaeocurrent analysis of the imbricated pebbles, and the palaeocurrent direction was found to be towards the SW.
Field observations show that this unconformity is more subtle than the Lower Cretaceous–Tertiary unconformity, and there is an angular difference of only 3–6° in bedding attitude of the over- and underlying formations. The unconformity surface is sharp and undulating (Fig. 12) on which there are no signs of tectonic polishing, brecciation, striation and mineralization. Based on these observations, the angular relationship is interpreted to be most probably a sedimentary rather than tectonic (i.e. thrusting) feature.

Figure 12. Field photographs of the angular unconformity between the conglomerates of the Maastrichtian Tanjero Formation and the hemipelagites of the Campanian Shiranish Formation at (a) Qandil area (36°20′31.4″N, 45°07′17.6″E) (directly to the east of Suwais village); (b) at Do Awan (35°59′4.68″N, 45°24′36″E), with line interpretations.
The angular unconformity was observed at two localities close to the Lower Cretaceous–Tertiary unconformity. The first locality was near Do Awan, about 10 km NW of Mawat (35°59′4.68″N, 45°24′36″E). The second was a few kilometres north of Suwais (36°20′31.4″N, 45°07′17.6″E) (Fig. 1b) and about 30 km NE of Ranyia. Across this angular unconformity, about 50 m of the Shiranish Formation is missing at the unconformable contact. The estimation of this removed thickness is based on the fact that the thickness of the Shiranish Formation is about 200 m, while its thickness, in the mentioned locality, is about 150 m.
4. Tectonic significance of the angular unconformities
4.a. Tectonic significance of the CTU
The stratigraphic position of the CTU is interpreted to indicate a major tectonic phase in the Imbricate Zone. First, the absence of Upper Cretaceous rock units (Kometan, Shiranish and Tanjero formations) across the CTU is interpreted to indicate uplift and erosion during ophiolite–radiolarite obduction. This may be interpreted in two ways: if deformation had taken place in post-Cenomanian–Turonian time, then the Upper Cretaceous rock units, i.e. Kometan, Shiranish and Tanjero formations, had no chance to form. In other words, their absence is not attributed to erosion, but to their non-deposition. However, if deformation had occurred after the Upper Cretaceous rocks were deposited, i.e. Kometan, Shiranish and Tanjero formations, then the absence of these units is owing to their erosion earliest in Late Cretaceous time if not later. This erosion continued until Paleocene time when the area subsided to form a shallow basin where the conglomerates of the Paleocene–Eocene Red Bed Series were deposited. It can be seen that at this time the orogenic wedge (Qulqula Radiolarian Formation) is thrust over the Kometan Formation.
Facies distribution maps (Buday, Reference Buday1980) indicate that the palaeogeographic slope direction of the basin (in the study area) was towards the NE during Early Cretaceous to Middle Turonian times. This means that during these times the sediment transport was towards the NE. Later, during Coniacian and Santonian times, the palaeoslope direction was reversed (i.e. towards the SW) in the study area, which is also indicated by sedimentary structures such as flute casts and cross-bedding (Karim & Surdashy, Reference Karim and Surdashy2005a). This reversal corresponds approximately to the period of ophiolite obduction in Oman (Coleman, Reference Coleman1981), while Karim & Surdashy (Reference Karim and Surdashy2005b) interpreted this reversal to indicate the continental collision of the Iranian and Arabian plates. According to Karim (Reference Karim2003), prior to collision, the Qulqula Radiolarian Formation, as a thick sequence of bedded cherts and calcareous shales, transformed into an accretionary prism in the trench that was associated with the convergence of the two plates. During later collision, these rocks were intensely deformed and uplifted and thrust (as an orogenic wedge) onto the NE margin of the Arabian plate. In front of this wedge, a large-scale foreland basin developed on the Arabian plate margin where the Shiranish, Tanjero and Kolosh formations (in addition to the Red Bed Series) were deposited (Karim & Surdashy, Reference Karim and Surdashy2005a, Reference Karim and Surdashyb, Reference Karim and Surdashy2006).
Such an unconformity is reported from the Iranian Zagros as well. Farzadi (Reference Farzadi2006c) recognized channel-like features in the uppermost part of the Sarvak Formation in western Iran. Farzadi (Reference Farzadi2006b) suggested that this subtle channel-like feature near the top of the Sarvak Formation on the NE flank of the Parsi anticline probably indicates the Turonian unconformity. These channels are located close to the Kuh-e Bangestan anticline where the Turonian unconformity is well developed. Homke et al. (Reference Homke, Vergés, Serra-Kiel, Bernaola, Sharp, Garcés, Montero-Verdú, Karpuz and Goodarzi2009) showed that at the base of the Paleocene (within the Gurpi Formation) there lacks NP1 and NP2 zones, implying a hiatus of ~2 Ma at c. 65.5 Ma. They inferred that this hiatus corresponds to an early folding phase near the Cretaceous–Paleocene boundary.
The difference in bedding dip of the Qulqula Radiolarian Formation relative to the Red Bed Series, and the absence of Upper Cretaceous rocks at the CTU, indicate a significant phase of orogenic deformation. Uplift and erosion of Lower Cretaceous (and/or younger) units may have occurred at any time between their deposition and the deposition of the Red Bed Series in Paleocene–Eocene time. If it had occurred in Mid to Late Cretaceous time, the unconformity may be attributed to the obduction phase of the orogeny. However, if it had occurred during Late Cretaceous–Early Tertiary time, it is likely to be owing to the early stages of continental collision rather than obduction since the latter could not have occurred in the northwestern segment of the Zagros later than that in the southeastern extension of the belt (i.e. Oman ophiolites).
Sometime between Mid Cretaceous and Paleocene time, owing either to obduction in Mid–Late Cretaceous time or to collision (Oligo-Miocene), deformation was transferred southwestward, resulting in a series of structures interpreted as fault-related folds upon which the Red Bed Series was unconformably deposited. The fact that the Red Bed Series is nearly flat-lying in these two areas indicates that little post-depositional deformation took place in this part of the Zagros. This in turn means that the deformation front had propagated further to the SW, and new structures were forming towards the distal area of the foreland after the deposition of the Red Bed Series in the study area.
These observations are not inconsistent with previous estimates of the timing of obduction of the oceanic crust on the Arabian continental crust (e.g. Buday, Reference Buday1980; Buday & Jassim, Reference Buday and Jassim1987; Numan, Reference Numan1997; Jassim & Goff, Reference Jassim and Goff2006). However, they suggest that orogenic deformation in the Imbricate Zone consisted of separate phases which began in Santonian time (obduction of ophiolite) with southwestward advance of the orogenic front in Paleocene–Eocene time, and continued with other phases in Oligo-Miocene time (continental collision).
The presence of these unconformities has significant tectonic implications. In addition to the presence of many ophiolite bodies in the east and west of the study area, clasts of ophiolite (gabbro and peridotite) are present in the conglomerates and sandstones of the Upper Cretaceous Tanjero Formation in many places (Karim, Reference Karim2006b, unpub. Ph.D. thesis, Univ. Sulaimani, Iraq, 2004). It is therefore assumed that the CTU formed after ophiolite obduction.
4.b. Tectonic significance of the CMU
As indicated by the studies of Al-Barzinjy (unpub. Ph.D. thesis, Univ. Sulaimani, Iraq, 2005), Karim (Reference Karim2006a) and Karim & Surdashy (Reference Karim and Surdashy2005a, Reference Karim and Surdashyb, Reference Karim and Surdashy2006), the areas where the unconformities now occur were coastal areas of the Late Cretaceous (?) and Paleocene foreland basins. The deposition of 500 m of boulder and gravel conglomerates of the Tanjero Formation indicates a high-relief source area (a mountain belt), which underwent intense erosion and transportation. It is possible that the areas where the CMU is located were the first areas that were bulged up and eroded as deformation reached the coastal area of the foreland basin during the ophiolite obduction.
Erosion of part of the Shiranish Formation across the CMU is interpreted to reflect the advance of the deformation front southwestward, beyond the location of the CTU. This is because the former unconformity (CMU) is located a few kilometres to the south of the latter one (CTU). Now, this distance is short, but it is possible that originally the distance was more during Late Cretaceous time and shortened by folding and thrusting.
The unconformity between the Shiranish and Tanjero formations is characterized by the presence, in some places, of up to 500 m of conglomerate resting on the lower part of the Shiranish Formation (Fig. 8). It seems that the Shiranish Formation, in the coastal area of the Campanian foreland basin, was tilted gently and partially eroded before being unconformably overlain by the conglomerates and sandstones of the Tanjero Formation during Maastrichtian time. The CTU occurs along the strike of (and on) closely spaced anticlines (Fig. 13) of the Qulqula Radiolarian Formation, which may have formed in an accretionary prism during Early–Mid Cretaceous time.

Figure 13. Bedded cherts and siliceous shale of the Qulqula Radiolarian Formation showing medium-scale folds which are exposed along the side road-cut at 4 km to the east of Chwarta town inside the Imbricate Zone.
The presence of these two unconformities at different stratigraphic levels and times could be used to argue that deformation along the c. 2000 km long ZFTB was variable both in style and timing. It is argued here that it is likely that ophiolite obduction, propagation of the deformation front, nature, temporal and spatial location and distribution of the foreland basins and collision were variable along the belt. However, it is worth underlining here that if obduction (or continental colliding) had started simultaneously along the entire length of the suture, the only way to fit a scissoring-style (protractor-style) closure for the Neo-Tethys would be to assume that the obducted slab in the NW was shorter, and that collision in the NW occurred earlier than in the SE where obduction lasted longer since the obducted slab was longer. This can partly be supported by the far-reaching obduction of the Semail ophiolite in the Oman Mountains, which was emplaced onto the Arabian continental margin during Late Cretaceous time (Searle & Cox, Reference Searle and Cox1999). However, the observations in this paper do not provide any evidence about the size of the ophiolite bodies in the NW Zagros, nor how far they have been emplaced.
An anticlockwise rotation of Arabia (and Africa) relative to Eurasia has been suggested by many workers (Kahle & Muller, Reference Kahle and Mueller1998; Sella, Dixon & Mao, Reference Sella, Dixon and Mao2002; Vernant et al. Reference Vernant, Nilforoushan, Hatzfeld, Abbassi, Vigny, Masson, Nankali, Martinod, Ashtiani, Bayer, Tavakoli and Chéry2004). And as stated above, based on this rotation, a scissoring-style closure of the Neo-Tethys Ocean has been proposed with the collision starting in the NW and propagating southeastward (Dercourt et al. Reference Dercourt, Zonenshain, Ricou, Kazmin, Le Pichon, Knipper, Grandjacquet, Sbortshikov, Geyssant, Lepvrier, Pechersky, Boulin, Sibuet, Savostin, Sorokhtin, Westphal, Bazhenov, Lauerm and Biju-Duval1986). However, Peynircioglu et al. (Reference Peynircioglu, Langereis, Kaymakci and Van Hinsbergen2010) used palaeomagnetic data to argue that observed rotation in the western part and northeastern edges of the Arabian plate is owing to local fault block rotations and does not represent the rotation of the entire plate. They argued further that large regions in the western part and northeastern edges of the Arabian plate (e.g. Urfa, Kocak and Mardin regions) show no rotations since Eocene time, and were likely part of the rigid Arabian plate. Whether Arabia was rotating until Eocene time or not is beyond the scope of this article. However, our field data indicate that deformation due to ophiolite emplacement has been variable in the northwestern segment of the ZFTB. This variation in propagation of the deformation front is likely to have occurred along this c. 2000 km long belt. Any rotation of Arabia relative to Eurasia would have likely added to this variation in deformation along the belt.
5. Conclusions
This paper reports the presence of two angular unconformities for the first time in the NW segment of the ZFTB. One of the unconformities is located between the Qulqula Radiolarian Formation (Lower Cretaceous) and Red Bed Series (Paleocene–Eocene); and the second is located between the Shiranish (Campanian) and the Tanjero (Maastrichtian) formations. These two unconformities, which are attributed to the ophiolite emplacement in the northwestern Zagros, indicate variable modes of obduction that have resulted in a variable style of deformation and propagation of the deformational front in the northwestern segment of the ZFTB in the Kurdistan Region. We argue that similar variation is expected along the c. 2000 km long ZFTB.
Acknowledgements
We would like to thank two anonymous reviewers and the special issue editor for their thorough but very constructive comments and suggestions. This work was funded by a grant from the Swedish Research Council VR and SIDA.