INTRODUCTION
In 2011, salmonellosis was the second most common reported foodborne zoonotic disease in humans in the European Union with 95 548 cases reported to ECDC [1]. These figures underestimates the real incidence of infections as the number of reported cases depends on healthcare-seeking behaviour as well as the organization of the healthcare systems including laboratory services and practices in the different countries. Salmonella infections in humans are most often diagnosed by bacterial culture of faecal samples which requires the shedding of viable bacteria which may decrease depending on the time since onset of infection. By contrast specific antibodies against a pathogen will be present in serum for at least some months, depending on the antibody isotype. We have previously shown that IgM and IgA antibodies against Salmonella persist for 2–4 months, whereas IgG antibodies may persist for up to 12 months [Reference Strid2]. On this basis it has been suggested that seroepidemiological methods can be applied as an active surveillance tool to measure the force of Salmonella transmission at the population level [Reference Kuhn3].
A mathematical model was previously developed to estimate Salmonella seroincidence in humans. In principle, the time since last infection recognized by the immune system (and the associated variability) can be calculated based on a set of IgG, IgM and IgA values measured in a single serum sample [Reference Simonsen4]. Using this model, the individual estimates of time since infection in each serum sample in a cross-sectional collection is converted to an annual seroincidence estimate in the population [Reference Simonsen5, Reference Falkenhorst6]. This method can be applied to compare the force of infection independent of healthcare systems and laboratory surveillance in different countries.
There are several challenges that must be dealt with in order to make this principle feasible. Our previous work was to a large extent based on the analysis of collections of serum samples that were representative of general healthy populations [Reference Simonsen5, Reference Falkenhorst6]. However, in many countries, such collections are not available or are reserved for other scientific purposes. Hence, in order to determine whether seroepidemiology is a feasible and practical tool, we investigated whether the analysis of sera from convenience samples (e.g. routinely collected sera from blood donors and pregnant women) would yield similar estimates of Salmonella seroincidence compared with community-based samples from healthy individuals since community-based samples are not widely available in most countries.
MATERIAL AND METHODS
Community cohort samples
In 2006, a random sample of 7770 Danish adults (with Danish citizenship and born in Denmark) aged 18–69 years living in one of the 11 municipalities of the former Copenhagen County were invited to participate in a general health examination. From June 2006 to July 2007, 3471 individuals agreed to participate. More details about the procedures including non-response have been described previously [Reference Thuesen7]. All participants completed a questionnaire and had a blood sample taken. For 1780 randomly selected individuals, a serum sample was analysed for Salmonella antibodies.
Convenience samples
For the present study, two convenience samples were obtained from the Department of Clinical Immunology at Copenhagen University Hospital. The department tests sera from blood donors and pregnant women [Reference Cowan8, 9] from about 35% of the Danish population, including the Capital region and Western Zealand. In October 2012, residual sera from 500 anonymous blood donors were collected and sent to Statens Serum Institut (SSI).
From August to December 2012, residual sera from pregnant women were forwarded to SSI aiming at the following age distribution: 80 sera per age group was the goal for all 5-year age groups from 20–24 up to 40–44 years while the aim was 50 sera for females aged <20 or >44 years.
ELISA method
All serum samples were tested for IgG, IgM and IgA Salmonella antibodies at the serological laboratory at SSI using an in-house Salmonella ELISA method, as described in Falkenhorst et al. [Reference Falkenhorst10].
Statistical analyses
The distribution of IgG, IgM and IgA, optical density (OD) values in blood donor sera, pregnancy sera and sera from the community cohort, were compared by the non-parametric Kruskal–Wallis test.
The IgG, IgM and IgA antibody responses were measured during a follow-up study of 303 patients with stool culture confirmed S. Typhimurium or S. Enteritidis infections [Reference Strid2, Reference Falkenhorst10]. Based on these antibody measurements a mathematical model was developed to describe the longitudinal behaviour of the antibody response to a Salmonella infection. Using this model the measured antibody values from the cross-sectional samples were back-calculated to time since last infection and these values were converted to an incidence estimate. Details of this model can be found in Simonsen et al. [Reference Simonsen4].
Seroincidences were compared pairwise by calculating means and percentiles of the posterior distributions of incidence rate ratios [Reference Simonsen5].
WinBUGS was used to estimate the longitudinal behaviour of antibodies after infection [Reference Lunn11], while the statistical program SAS was used for the descriptive analyses and the estimation of incidence rates and ratios [12].
RESULTS
Descriptive statistics
Table 1 shows the age distributions of participants in the community cohort (all subjects as well as subjects stratified by gender), and of the serum collection of pregnant women. Sera from the community cohort originated from individuals with a median age of 50 years (range 18–71 years). The age distributions for males and females were similar. The proportion of females was 55·1%. The majority of females in the community cohort were aged >40 years while the majority of pregnant women were aged between 20 and 44 years (median age 31 years) (Table 1). The age and sex distribution was not available for the blood donors; however, our 500 sera came from a blood donor population with an age range from 17 to 67 years and a median age of 40 years. The sex distribution was 52·8% male and 47·2% female.
Table 1. Comparison of age distributions in the community cohort and in pregnant women
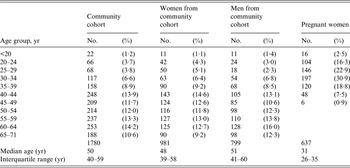
The measured IgG, IgM and IgA OD values were compared visually by box-and-whiskers plots (Fig. 1). For all three antibody classes, the lowest OD values were observed in the samples from the community cohort whereas the highest values were observed in the samples from pregnant women. Testing for similar distributions using the Kruskal–Wallis test confirmed the observed IgG, IgM and IgA differences in pregnant women, blood donors and community cohort data. For each of the three antibodies IgG, IgM and IgA we found statistically different distributions between the three cohorts (P values <0·001).
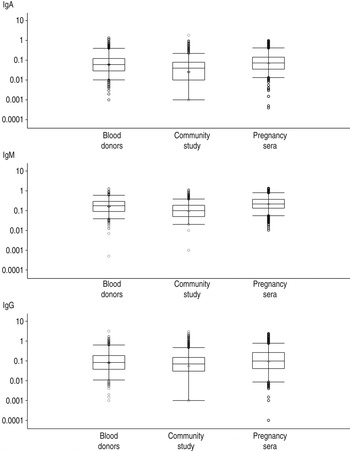
Fig. 1. The distribution of measured IgG, IgM and IgA optical density values in the three-sample community study, blood donors and pregnant women in a box-and-whiskers plot. The box represents the 25th–75th percentile, the line across the box is the median while the diamond is the mean, the lower and upper lines represent the 2·5th–92·5th percentiles and the circles represent the measurements below and above the lower and upper limits.
Of the 637 pregnant women, 17 were aged <20 years and eight were aged ⩾44 years. After omitting these from the analysis in order to obtain an age distribution similar to the community cohort, we compared the measured Salmonella IgG, IgM and IgA antibodies in 612 sera from pregnant women aged between 20 and 44 years with Salmonella antibodies in 371 sera from females aged between 20 and 44 years from the community cohort (Table 2). The antibody levels in pregnant women were still higher compared to women of the same age from the community cohort (Table 2).
Table 2. Antibodies against non-typhoid Salmonella measured in mixed lipopolysaccharide ELISA as optical density (OD) values

Median and interquartile range values are shown for the community study, blood donors, pregnant women and two subsamples including women aged between 20 and 44 years. The samples were collected in Denmark in 2006–2007 and 2012.
Calculation of seroincidence
The estimated seroincidence in pregnant women was 319 infections/1000 population per year, corresponding to a mean annual risk of 27%. This mean seroincidence was 1·8 times higher than estimated from blood donors (182 seroconversions/1000, mean annual risk 17%). In the community cohort, the mean seroincidence was 77/1000, or a mean annual risk of 7%, which was 4·1 times lower than for pregnant women and 2·4 times lower than for blood donors (Table 3). The pairwise comparisons showed significantly different seroincidences between the community cohort and the pregnant women; however, the estimated seroincidences between the community cohort and the blood donors as well as between the pregnant women and the blood donors did not differ significantly at the 10% level (Table 4). For the community cohort, seroincidences were estimated for the following subgroups: males and females, participants aged <44 years and ⩾44 years, females aged <44 years and ⩾44 years, and males aged <44 years and ⩾44 years (Table 3). Pairwise comparisons between these subgroups showed no significant differences in seroincidences (Table 4); however, there is a tendency that younger individuals have a higher seroincidence compared to older individuals, particularly younger women (Table 3). We also estimated the seroincidence in women aged between 20 and 44 years participating in the community cohort and obtained an estimate of 128 seroconversions/1000; this was still significantly different compared to pregnant women of the same age but the difference was not as marked as between all pregnant women and all women from the community (Table 4).
Table 3. Estimated Salmonella seroincidence per year in Denmark, in a community cohort (years 2006–2007), in blood donors (year 2012) and in pregnant women (year 2012)
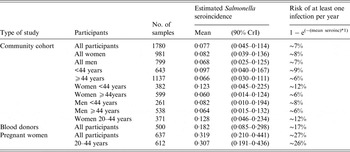
CrI, credibility interval.
Table 4. Pairwise comparisons of estimated Salmonella seroincidences using rate ratios

CrI, credibility interval.
DISCUSSION
In many countries, programmes for Salmonella control have successfully applied serological methods to follow the occurrence of Salmonella in food animals [Reference Wegener13–Reference Stockmarr, Bødker and Nielsen16]. There is currently no human counterpart to the systematic monitoring of food animals by serological methods. The end point for a reduction in human illness is monitored by passive public health surveillance of culture-confirmed cases. It is well known that these data are subject to a number of limitations, including patterns of healthcare-seeking behaviour, practices at the healthcare level for obtaining faecal specimens for culture as well as laboratory and reporting practices [Reference Falkenhorst6, Reference Hardnett17].
In European studies, we have demonstrated a close correlation between country-specific seroincidence and the occurrence of Salmonella in food animals as well as with a less biased assessment of the relative occurrence of Salmonella in humans (the ‘Swedish travellers approach’) [Reference Falkenhorst6, Reference Mølbak18]. Most of these studies have taken advantage of collections of human samples that were carefully selected to achieve a high degree of representativeness of the general population. Such collections are difficult and costly to obtain, and it is important to be able to use more easily available serum collections to enable monitoring of the serological status in the human population as a feasible tool. In the present study we showed that Salmonella seroincidence in Denmark was more than four times higher when estimated from pregnant women compared to the community cohort. Although the difference between the seroincidence in pregnant women and individuals from the community cohort was reduced when the different age distributions in the two populations were taken into account we still found a significantly higher seroincidence in pregnant women compared to the community cohort. The samples were taken early in pregnancy and the outcome is likely to reflect a history of infections prior to conception, i.e. before pregnancy-related lifestyle changes may have modified the risk of infection. The individuals who participated in the community cohort had a higher educational level and a higher personal income than non-responders and non-responders were more often living alone than the responders [Reference Thuesen7]. It cannot be excluded that some participants who contributed to the community study did so because they have an interest in a healthy lifestyle, and therefore community-based samples may be more selected than desired by researchers.
The mean Salmonella seroincidence was 1·7 times higher when estimated from pregnant women than from blood donors; however, this comparison did not account for the fact that blood donor sera were drawn from a population with a median age of 40 years while pregnant women had a median age of 31 years. It is likely that a higher age distribution in blood donors compared to pregnant women has overestimated the difference in the mean seroincidence between pregnant women and blood donors and similarly between blood donors and the community cohort.
The finding of a higher seroincidence in pregnant women compared to the general population is in contrast with data from The Netherlands in 2006, where 530 leftover sera from the screening programme for pregnant women were analysed for Salmonella antibodies. The Salmonella seroincidence was 86/1000 (90% credibility interval 28–175) persons which tended to be lower than the population-based estimate of 149/1000 persons per year based on representative sera from 2006 to 2007 [19]. The reason for the observed difference in seroincidence in sera from pregnant women and population-based sera in The Netherlands and Denmark is not known. Both the Dutch and the Danish sera were analysed using the same in-house Salmonella ELISA from SSI and performed at SSI, and the same seroincidence back-calculation model was used for the seroincidence calculations. In addition, several technical reasons like handling and storage of sera have also been explored but no technical explanation can be identified.
The main limitation of the present study is the fact that the community-based samples were taken in 2006 and 2007 whereas our convenience samples were obtained 6 years later. As the number of notified human Salmonella cases in Denmark decreased from 1665 cases in 2006 to 1199 cases in 2012, a lower seroincidence in 2012 compared to 2006 might be expected. However, notified cases represent individuals so ill that they seek medical care. The estimated seroincidence represents all Salmonella infections including asymptomatic and mild symptomatic infections. Although fewer individuals become clinically ill from Salmonella this does not imply that a similar decrease in subclinical infections will occur.
The study is subject to other limitations. We know that the incidence of culture-confirmed Salmonella infections exhibits a marked seasonality, with more infections diagnosed in the late summer. The samples from the community cohort were collected during the entire year, whereas the convenience samples were collected in a short time period in the autumn of 2012. We have previously found that seasonality is a minor issue with regard to serology compared to the variation in stool cultures [Reference Simonsen20]. However, blood samples sent to SSI for diagnostic purposes during 2008–2013 were analysed for Salmonella antibodies and the first preliminary results indicated seasonality with the highest antibody levels in late summer. Therefore we cannot exclude that the season of convenience sampling introduced a bias towards a higher estimate compared to an all-season sampling scheme. Furthermore, children were not included in any of the studies.
Finally, we wish to emphasize that none of the estimated measures of seroincidence reflect the burden of clinical illnesses. This method does not distinguish between clinical infections and exposures to Salmonella that result in (re)activation of the immune system without apparent clinical illness.
In conclusion, we found that estimates of Salmonella seroincidence depend on the population from which the serum samples are drawn. Consequently, it is important to define a certain population under surveillance in order to apply serology in humans as a tool to monitor the progress of Salmonella control programmes in Europe. Anonymized residual blood sera where the age and sex distributions are known could potentially be used for such a monitoring programme. The impact of different age and sex distributions over time can be adjusted statistically. Although the results are likely to be valid when comparing within a population over time this needs to be further investigated before setting up a serological surveillance programme. Based on the mathematical model used in this study a seroincidence calculator tool was developed in the statistical program R by Peter Teunis from the Centre for Infectious Disease Control, National Institute of Public Health and the Environment (RIVM) in The Netherlands. From March 2015 this tool will be available from the ECDC homepage [21].
ACKNOWLEDGEMENTS
We acknowledge the efficient laboratory work from Bjørn Kantsø, Daniel Silla Jatta and Tina Hilal Ceper from the Division of Microbiological Diagnostics and Infection Control, Statens Serum Institut, Copenhagen, Denmark. We also acknowledge Johanna Takkinen from the European Centre for Disease Prevention and Control, Solna, Sweden for excellent collaboration during the project
The project has been co-funded by the European Commission within the 6th Framework Programme (contract no. 506122), and by the European Centre for Disease Control and Prevention, project OJ/2009/06/03-PROC/2009/021.
DECLARATION OF INTEREST
None.