INTRODUCTION
The European conference on methicillin-resistant Staphylococcus aureus (MRSA), organized in 2008 by the Federation of Veterinarians of Europe, concluded that it was time to tackle MRSA [1]. Ten years previously, a conference on MRSA organized by veterinarians would have seemed rather peculiar. However, the ever emerging recognition of a new type of MRSA, believed to be of animal origin, and the expanding number of reports on MRSA transfer between animals and humans, has led to the growing awareness that MRSA is now a problem in both human and veterinary medicine.
When studying the epidemiological aspects of animal MRSA, food production animals (cattle, pigs and poultry, hereafter referred to as livestock) are of particular concern. Not only are they recorded as the primary source of the newly emerging MRSA type, studies also suggest that they are involved in transfer of MRSA strains between animals and humans (and vice versa). Indeed, as livestock they are in close contact with humans (farmers, farm co-workers, veterinarians, etc.) and once they have entered the food chain, they might serve as convenient vehicles for bacterial transfer, possibly threatening food handlers and consumers.
In this review, the current knowledge on the prevalence, epidemiology, evolution and medical importance of MRSA in both livestock and derived food products is summarized. As an introduction, the most relevant facts on S. aureus and methicillin resistance are listed, followed by a short description of the most frequently used typing methods for both methicillin-susceptible S. aureus (MSSA) and MRSA.
MRSA: methicillin-resistant S. aureus
S. aureus is the best characterized species among the staphylococci, a genus of Gram-positive, A-T rich cocci comprising over 50 species and subspecies according to the NCBI Taxonomy browser [2]. It forms part of the normal staphylococcal flora of humans and various animal species [Reference Williams3, Reference Devriese4]. S. aureus is also the most important human pathogenic Staphylococcus species, with clinical conditions ranging from common minor skin infections to severe, often life-threatening infections [Reference Lowy5].
In animals, S. aureus is one of the three major pathogenic Staphylococcus species, together with S. (pseud)intermedius and S. hyicus [Reference Hermans, Devriese, Haesebrouck, Gyles, Prescott, Songer and Thoen6]. The scale of infections it may be involved in is as broad as the number of animal species suffering from it, ranging from pneumonia, joint infections, osteomyelitis and septicaemia in poultry [Reference McNamee and Smyth7–Reference Alfonso and Barnes9], subcutaneous abscesses, mastitis and pododermatitis in rabbits [Reference Okerman10, Reference Hermans, Devriese and Haesebrouck11], dermatitis and cellulitis in horses [Reference Middleton12, Reference Fjordbakk, Arroyo and Hewson13] to septicaemia in pigs [Reference Devriese4]. However, S. aureus plays its most significant animal pathogenic role as cause of intramammary infections in cattle and small ruminants [Reference Hermans, Devriese, Haesebrouck, Gyles, Prescott, Songer and Thoen6, Reference Waage14–Reference Piepers16], leading to considerable economic losses in cattle farming [Reference Hermans, Devriese, Haesebrouck, Gyles, Prescott, Songer and Thoen6, Reference Bar17, Reference Huijps, Lam and Hogeveen18].
S. aureus owes its strong pathogenic capacities to the presence of a large number of various virulence factors [Reference Lowy5, Reference Johnson, Metzger and Spero19–Reference Fournier and Lindsay24]. In addition, an important impediment in the control of S. aureus infections is its tendency to gain resistance to almost all classes of antimicrobial agents to which it is subjected [Reference Lowy25]. Of particular concern is the acquired resistance to the β-lactamase stable β-lactam antibiotics, historically known as methicillin resistance. Indeed, methicillin resistance is caused by the expression of an alternative penicillin-binding protein, called PBP2a or PBP2' [Reference Georgopapadakou26, Reference Hartman and Tomasz27]. Since PBP2a shows a very low affinity for almost all β-lactam antibiotics [Reference Hartman and Tomasz27], MRSA is resistant to almost all antibiotics of this very comprehensive group, of which many members are still widely used in both human and veterinary medicine.
PBP2a is encoded by the mecA gene [Reference Matsuhashi28, Reference Ubukata29]. This gene is localized in a mobile genetic element, named the staphylococcal cassette chromosome mec (SCCmec). According to their structural composition, SCCmec elements are categorized into different types. Each type is marked with a number. While initially it seemed that there were only a few different SCCmec types, it has now become clear that more exist, and the nomenclature is rapidly evolving. Currently, eight different types are recognized (Table 1). These types are all based on SCCmec elements found in human MRSA strains.
Table 1. Summary of SCCmec types currently described in methicillin-resistant S. aureus
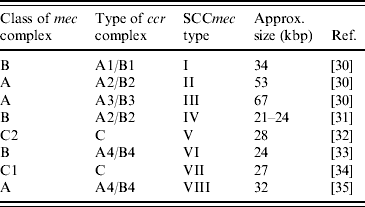
SCCmec, Staphylococcal cassette chromosome mec.
Molecular typing of (MR)SA
PFGE
Pulsed-field gel electrophoresis, assigning isolates into pulsotypes, is presently considered as the gold standard method for typing of S. aureus, of both human and animal origin. As a standard for S. aureus, the enzyme SmaI is used for the macro restriction [Reference Tenover36–Reference Murchan38].
Multilocus sequence typing (MLST)
In MLST of S. aureus, internal fragments of seven housekeeping genes are amplified and sequenced [Reference Maiden39, Reference Enright40]. A sequence type (ST) is assigned [Reference Enright40] using the S. aureus MLST database [41]. Strains that differ in only one or two loci are called single locus variants (SLVs) and double locus variants (DLVs), respectively. STs, SLVs and DLVs are grouped into clonal complexes (CCs) using ‘based upon related sequence types’ (BURST) analysis. In a CC, the ST that has the highest number of different SLVs and DLVs is called the ancestral ST, and the CC is numbered after its ancestral ST [Reference Enright42].
spa-typing
The polymorphic X-region of the staphylococcal protein A (spa) gene contains a variable number of different repeats of mostly 24 bp [Reference Frénay43]. In spa-typing, this repeat region is amplified and sequenced. The total number of repeats and the sequence of each repeat determine a repeat profile, the spa type [Reference Shopsin44, Reference Harmsen45].
Recently, the Panel on Biological Hazards of the European Food Safety Authority (EFSA) has recommended spa-typing for discrimination between MRSA strains from livestock [46]. It should be noted that most of these strains are not typable with PFGE following SmaI digestion and that they generally belong to the ST398 sequence type (see below).
SCCmec-typing
An SCCmec element is composed of two essential gene complexes: the mec complex, containing mecA and its direct regulatory genes, and the ccr complex, responsible for the mobility of SCCmec [Reference Ito30, Reference Hiramatsu47–Reference Katayama, Ito and Hiramatsu50]. Variants of these complexes are distinguished according to their structural composition [Reference Hiramatsu51]. The assignment of SCCmec types is essentially based on which variant of each complex is present (Table 1). This is generally investigated using PCR techniques. Both simplex PCRs [Reference Ito, Katayama and Hiramatsu49, Reference Okuma52] and multiplex PCRs [Reference Oliveira and de Lencastre53–Reference Milheiriço, Oliveira and de Lencastre56] have been developed.
While the mec and ccr complexes show some limited variation, the other parts of SCCmec, called J regions, can vary greatly within and between SCCmec types [Reference Hiramatsu51]. Subtypes of SCCmec are distinguished based upon differences in the J regions (e.g. [Reference Oliveira, Tomasz and de Lencastre57–Reference Shore59]), and these can also be detected by PCR [Reference Oliveira and de Lencastre53–Reference Kondo55, Reference Milheiriço, Oliveira and de Lencastre60].
An important impediment of the above-mentioned methods is that they are all based on SCCmec sequences found in MRSA strains of human origin. Recent studies have shown that these methods fail to identify some SCCmec elements found in MRSA strains of livestock origin [Reference Nemati61, Reference Vanderhaeghen62] and many SCCmec elements found in methicillin-resistant non-S. aureus staphylococci (MRNaS) [Reference Ibrahem63, Reference Ruppé64]. As it is expected that additional SCCmec types will be identified in the near future, the present methods will require continuous updating. For example, the recently identified SCCmec types VII and VIII [Reference Berglund34, Reference Zhang35], are not included in the current methods.
History of MRSA
The history of MRSA is mainly situated in human medicine and started in 1961, when MRSA was first isolated in a UK hospital [Reference Barber65, Reference Jevons66]. From then onwards, MRSA began to spread in hospitals throughout the world, but it was at the end of the 1980s and during the 1990s that its prevalence became fully manifest in many countries [Reference Panlilio67–Reference Styers70]. At the beginning of the present century, it was shown that most of the then-known international epidemic hospital strains, i.e. hospital-acquired MRSA (HA-MRSA), belonged to only five CCs: CC5, CC8, CC22, CC30 and CC45 [Reference Enright42], and that they generally possessed one of the larger SCCmec types I–III [Reference Robinson and Enright71], partly explaining their resistance to most clinically used antimicrobial agents [Reference Lowy5, Reference Speller72, Reference Tenover, Biddle and Lancaster73]. As it is assumed that in humans, the use of large quantities of antimicrobial agents can lead to selection and emergence of organisms resistant to these agents [Reference Tenover and McGowan74], prolonged antimicrobial therapy has been designated a risk factor for the acquisition of HA-MRSA [Reference Monnet and Frimodt-Møller75, Reference Monnet76], as have prolonged hospitalization, care in an intensive-care unit, surgical procedures, and close proximity to a hospital patient who is infected or colonized with MRSA [Reference Thompson, Cabezudo and Wenzel77, Reference Boyce78].
While the problems with HA-MRSA were not yet at their height, a second phase in the history of MRSA appeared halfway through the 1990s, when MRSA infections involving strains different from HA-MRSA were increasingly documented in non-hospitalized patients [Reference Udo, Pearman and Grubb79–Reference Chambers83]. Such cases, called community-associated or community-acquired MRSA (CA-MRSA), have since been reported worldwide. Although there has been some discussion about the origin of several (early) cases [Reference Salgado, Farr and Calfee84], analysis of the genetic background of CA-MRSA strains has shown a clear distinction from typical HA-MRSA, as they predominantly belong to ST1, ST8, ST30, ST59, ST80 and ST93 [Reference Vandenesch85]. In addition, CA-MRSA mostly possess the smaller SCCmec types IV and V [Reference Ito32, Reference Okuma52, Reference Tristan86], which is assumed to be, at least in part, an explanation for the generally more antimicrobial-susceptible phenotype of CA-MRSA. The carriage of the genes encoding Panton–Valentine leukocidin (PVL), a cytotoxin believed by many authors to be responsible for severe infections of the skin and soft tissues [Reference Lina87, Reference Badiou88], and highly lethal necrotizing pneumonia [Reference Gillet89–Reference Gillet91], is considered to be typical for certain CA-MRSA strains [Reference Vandenesch85, Reference Dufour92]. The pathogenic role of PVL is, however, still under discussion [Reference Voyich93, Reference Bubeck Wardenburg94]. Compared to HA-MRSA, CA-MRSA also seems to possess different risk factors for acquisition, as it has been most often reported in populations of intravenous drug users, men who have sex with men, prison inmates, contact sport teams, military recruits and children [Reference Herold80, Reference Saravolatz, Pohlod and Arking95–Reference David101].
Although MRSA was first isolated in animals in 1972, from Belgian cows with mastitis [Reference Devriese, Van Damme and Fameree102], animals do not appear to play a significant role during most of the history of MRSA. Based on the results of biotyping methods available at the time, it was concluded that those first isolates were of human origin [Reference Devriese and Hommez103]. Further, in occasional later reports on MRSA isolated from animals (mainly companion animals) the strains were mostly human genotypes [Reference Cefai, Ashurst and Owens104–Reference Hanselman, Kruth and Weese108]. This appeared unsurprising, given the increasing prevalence of MRSA in non-hospitalized community members at the time. The problem of (human) MRSA in companion animals has since grown, and entirely new concerns were raised in 2005, when MRSA was found to be associated with pig farming in The Netherlands [Reference Voss109].
This third phase in the history of MRSA was initiated ‘accidentally’, by the unexpected isolation of MRSA from a family of pig farmers and one of their pigs [Reference Voss109]. Results of subsequent investigations showed that pig farmers from the same geographical region were carrying MRSA in a >760-fold higher carriage rate than the general Dutch population.
Markedly, PFGE analysis of the MRSA strains showed that they were all resistant to digestion with restriction endonuclease SmaI, but spa-typing and randomly amplified polymorphic DNA (RAPD) analysis proved that all strains were closely related to each other [Reference Voss109]. When a few months later a pig farmer from another region, an unrelated veterinarian working mostly with pigs, that veterinarian's son and a nurse treating the boy were all found to be colonized with a related MRSA strain, it was concluded that pig farming might pose a significant risk for MRSA carriage in humans [Reference Voss109].
At that time, the importance of this specific type of MRSA was not clear; moreover, the extent of the problem concerning farming of pigs as well as other animals could only be speculated upon. However, in the following months and years, a multitude of reports, from The Netherlands as well as many other countries, showed that this type of MRSA had not only spread among pigs, other animal species (veal calves, chickens, horses, dogs, rats) and humans in relation to farming [Reference Nemati61, Reference Wulf110–Reference van de Giessen126], but was also capable of causing disease in animals [Reference Vanderhaeghen62, Reference Sergio115, Reference Witte119, Reference van Duijkeren127–Reference Schwarz, Kadlec and Strommenger129] as well as humans [Reference van Loo116, Reference Witte119, Reference Ekkelenkamp130–Reference Krziwanek, Metz-Gercek and Mittermayer134]. It is now understood that this MRSA is disseminating clonally, possessing some typical features:
• Non-typability with standard PFGE with SmaI digestion; this was shown to be due to the presence of a new restriction/methylation system leading to protection from SmaI digestion [Reference Bens, Voss and Klaassen135]. It is not clear how this novel system was achieved. The specific system was thus far unknown for S. aureus and even for the genus Staphylococcus [Reference Bens, Voss and Klaassen135].
• Being mainly MLST ST398. Very rarely have SLVs or DLVs, such as ST752 and ST753, been reported [Reference van Loo116]. All strains thus belong to the same CC, CC398 [41, Reference van Loo116, Reference Ekkelenkamp130].
• Various spa types; we have knowledge of 25 different yet related spa types that have been reported to belong to CC398 (Tables 2 and 3). Most of these types are combinations of repeat sequences r02, r08, r16, r24, r25 and r34 (Table 2). Some other repeats are present less frequently but are closely related to some of the common repeats (Table 3). As new spa types have continuously been reported so far, it seems likely that more types will be described in the future.
Table 2. Repeats of 25 spa types reported to belong to CC398 MRSA strains
spa, Gene encoding Staphylococcus protein A; MRSA, methicillin-resistant Staphylococcus aureus.
Table 3. Sequence of ancestral repeats (*) and possibly derived repeats
• Carriage of SCCmec types IVa or V; some studies also found SCCmec type III [Reference Nemati61, Reference van Loo116, Reference Wulf122]. However, the method they used, a multiplex PCR described by Zhang et al. [Reference Zhang54], is under debate concerning its reliability for typing SCCmec type III [Reference Jansen, Box and Fluit139]. Moreover, several studies have stated that SCCmec type IV was present [Reference van Loo116, Reference Denis123, Reference van Duijkeren127, Reference van Duijkeren136, Reference Yu140]. No subtyping was performed by these authors, so it is not clear whether their strains belonged to IVa. Currently, no other subtype than IVa has been found in this MRSA clone. Markedly, the SCCmec elements often appear to be non-typable with the common SCCmec-typing techniques [Reference Nemati61, Reference Vanderhaeghen62, Reference van Loo116].
• General absence of PVL; differentiating these strains from typical CA-MRSA strains. Further, many other virulence(-associated) factors, known to be present in typical HA- or CA-MRSA strains, have been shown to be absent in MRSA of ST398 [Reference Monecke141, Reference Walther142]. Despite this large absence of virulence(-associated) factors, MRSA ST398 strains have been found to cause disease, in both animals [Reference Vanderhaeghen62, Reference Sergio115, Reference Witte119, Reference van Duijkeren127–Reference Schwarz, Kadlec and Strommenger129] and humans [Reference van Loo116, Reference Witte119, Reference Ekkelenkamp130–Reference Wulf133]. It is as yet unclear which factors are involved. More investigations are urgently required to elucidate this.
• Resistance against tetracycline, and frequent resistance against macrolides, lincosamides, aminoglycosides and trimethoprim. Fluoroquinolone resistance has also been reported [Reference Nemati61, Reference Vanderhaeghen62, Reference Guardabassi, Stegger and Skov112, Reference Witte119, Reference Denis123, Reference van Duijkeren127, Reference Lewis132].
Some different descriptions have been proposed for referring to these MRSA strains. Because of their typical resistance to SmaI digestion, they are sometimes called non-typable MRSA (NT-MRSA). There are, however, arguments against the use of this description. First, it is obvious that these strains are not non-typable. Many other techniques have proven to be useful for typing the ‘so-called’ NT-MRSA [Reference Voss109, Reference de Neeling111, Reference van Belkum143, Reference Rasschaert144]. Moreover, when using other restriction enzymes, positive results can be obtained with PFGE [Reference Fanoy138, Reference Rasschaert144]. Second, from bacteraemia blood samples from a Hong Kong hospital collected in 2000–2001, two MRSA isolates were obtained with a clonal background formerly unknown for Hong Kong, ST398 [Reference Ip145]. However, they proved to be digestible by SmaI, and were assigned to pulsotype I.
It seems reasonable that the clonal background should resolve the name. ST398 is a type that was virtually absent from the human population before initial reports in the early 2000s, and typing data appears to support the view that its recent presence in humans is a direct result of its emergence in livestock, and more specifically, pigs [Reference van Loo116, Reference Aubrey-Damon146, Reference Armand-Lefevre, Ruimy and Andremont147]. This supports a livestock origin of these MRSA strains. Consequently, livestock-associated MRSA (LA-MRSA) appears to be the most appropriate description, and this designation will be used hereafter.
MRSA in livestock
Although LA-MRSA is the most important MRSA clone residing in livestock, it is not the only MRSA type that has been reported in livestock. Below a general overview is given of MRSA in livestock.
Pigs
LA-MRSA seems to be the predominant MRSA strain in pigs, as in numerous recent studies only two mention the detection of non-LA-MRSA in pigs. In Singapore, one ST22-MRSA-IV was isolated from pigs [Reference Sergio115]. ST22-MRSA-IV had been found previously to be increasingly important in the hospital population of Singapore and is also known as UK-EMRSA-15, one of two major hospital clones in the UK [Reference Lewis132], indicating human contamination of the pigs. In Canada, 14% MRSA isolates from pigs appeared to belong to the human epidemic CMRSA-2 clone, while 74·4% of the isolates were LA-MRSA [Reference Khanna121]. The remaining strains belonged to rare clones, not related to LA-MRSA or CMRSA-2 [Reference Khanna121].
Most reports on LA-MRSA in pigs come from The Netherlands [Reference de Neeling111, Reference van Duijkeren127, Reference Huijsdens131, Reference van Duijkeren136]. In Europe, LA-MRSA has further been found in pigs in Germany [Reference Witte119, Reference Schwarz, Kadlec and Strommenger129], Denmark [Reference Guardabassi, Stegger and Skov112] and Belgium [Reference Willems117, Reference Dewaele120], while outside Europe, in Canada [Reference Khanna121, Reference Weese148], Singapore [Reference Sergio115] and USA [Reference Smith149].
Several studies have described colonization of healthy pigs. At pig level, the reported carriage rates vary considerably between countries, from 1% in Denmark [Reference Guardabassi, Stegger and Skov112], 18·6% in Canada [Reference Khanna121], to ~40% in Belgium and The Netherlands [Reference de Neeling111, Reference Willems117]. Farm-level rates are generally higher: 66% in Denmark [Reference Guardabassi, Stegger and Skov112], 68% in Belgium [Reference Willems117] and 45% in Canada [Reference Khanna121]. However, these figures have to be interpreted carefully, as the number of farms included in the studies varied considerably, from 3 in Denmark [Reference Guardabassi, Stegger and Skov112], 20 in Canada [Reference Khanna121] to 50 in Belgium [Reference Willems117]. In The Netherlands two studies found very different farm-level prevalences, 81% positive farms (44/54 farms) [Reference Huijsdens131] compared to 23% positive farms (7/31 farms) [Reference van Duijkeren136]. However, both studies used different culture techniques. In addition, the result of the former study could have been increased by cross-contamination in the slaughterhouse, where the sampling was done [Reference Huijsdens131]. In the latter study, on the other hand, the majority of farms belonged to the ambulatory clinic of the Veterinary Faculty of Utrecht, and in these farms antimicrobial use is generally more restricted than in other farms, implying a possible underestimation of farm-level prevalence [Reference van Duijkeren136].
Limited other investigations have been performed to elucidate factors possibly influencing LA-MRSA prevalences in pigs. In one study, different farm-management systems showed significant differences in LA-MRSA prevalences, with LA-MRSA being detected in 94% of open farms (fattening farms) compared to 56% in closed farms (farrow-to-finish farms) [Reference Willems117]. In another study, however, LA-MRSA prevalence appeared to differ greatly when comparing between two closed farm systems; one production system was highly MRSA positive and the other system appeared MRSA negative [Reference Smith149]. As a cause for this, differences in other aspects, such as breed and herd size, were suggested by the authors [Reference Smith149]. A third factor suggested by these authors was the origin of the sows. The sow herds of both production systems had been repopulated shortly before the date of sampling. It was established that some of the sows of the MRSA-positive system had been imported from Canada, where pigs have been found to be affected by LA-MRSA [Reference Khanna121]. The sows from the other system came from Michigan, USA [Reference Smith149]. Although the authors could not give epidemiological evidence, LA-MRSA was thus possibly brought into the positive farm via import of affected live swine or pork products. However, this should not mean Canada is the origin of LA-MRSA in the USA, as at that time the presence of LA-MRSA in other regions of the USA, such as Michigan, had possibly not been investigated.
Although more studies are required to reliably assess the influence of farm management and related aspects on LA-MRSA prevalence, these studies suggest an important role for national and international pig trading in the dissemination of LA-MRSA in pig farming. This was also suggested by a Dutch study, in which indications were found that finishing and farrowing farms may get colonized by LA-MRSA through the purchase of colonized pigs from their supplier farms [Reference van Duijkeren136]. A recent study also showed that piglets from a LA-MRSA-positive sow were 1·4 times more likely to be colonized with LA-MRSA than piglets from a negative sow [Reference Weese148]. Purchase of LA-MRSA-positive sows will thus facilitate the dissemination of LA-MRSA in a farm.
Another factor that might be implicated in LA-MRSA prevalence in pigs is age. In a Belgian study, the probability of being MRSA positive was significantly higher for piglets than for both sows and fattening pigs [Reference Willems117]. The influence of age was also investigated by a recent Canadian study, in which the dynamics of MRSA colonization in piglets was investigated over time [Reference Weese148]. All MRSA appeared to be LA-MRSA and the colonization rates were found to be low initially (<10%) but increased over time, to 35% positive piglets prior to weaning and a peak of 64% positive piglets at age 42 days. At the last day of sampling (age 70 days) 41% of piglets carried MRSA. Conversely, an earlier Canadian study found no significant difference in MRSA prevalence between three different age groups of pigs, i.e. suckling pigs, weanlings, and grower/finisher hogs [Reference Khanna121]. More investigations are thus required to elucidate any possible influence of age.
LA-MRSA has also been isolated, albeit rarely, from infections of pigs. These involved skin infections such as exudative epidermidis [Reference van Duijkeren127] or others [Reference Sergio115, Reference Schwarz, Kadlec and Strommenger129] and also infections of the urogenital tract [Reference Schwarz, Kadlec and Strommenger129] and the uterus and mammary gland [Reference Schwarz, Kadlec and Strommenger129]. Currently it is unclear which virulence factors are involved. However, considering the far higher number of unaffected carriers, LA-MRSA appears to be a colonizing strain of pigs.
Cattle
In cows, S. aureus plays a significant role as a major cause of mastitis [Reference Hermans, Devriese, Haesebrouck, Gyles, Prescott, Songer and Thoen6] and most studies on MRSA in cattle concern isolation of MRSA from mastitis. Unlike its development in humans, the first detection of MRSA in mastitis [Reference Devriese, Van Damme and Fameree102] was not the beginning of a steady increase in MRSA prevalence. Although more MRSA was detected in some originally positive farms on several occasions in the 2 years after the first isolation [Reference Devriese and Hommez103], and while MRSA was still detected in Belgian routine antibiotic susceptibility tests in subsequent years [Reference Devriese150], the prevalence fell and in 1982–1983 MRSA was no longer detected in Belgium, an absence which lasted for almost 25 years [Reference Vanderhaeghen62, Reference Devriese150]. Moreover, in other countries no MRSA was reported in mastitis for a considerable time. Reports became more frequent from the early 2000s onwards.
From these reports, it is difficult to estimate an overall prevalence of MRSA in mastitis. First, there are often inconsistencies in laboratory methods in different studies, making it difficult to make viable comparisons. The most important example of this is the lack of control on the presence of the mecA gene in many studies, which can result in unreliable data [Reference De Oliveira151], for example because of the heteroresistance of S. aureus to methicillin [Reference MacKenzie152]. Several studies reporting MRSA in mastitis failed to verify or only partially verified the presence of the mecA gene [Reference Cuteri153–Reference Turutoglu, Ercelik and Ozturk156], and should thus be treated with caution when estimating the prevalence of MRSA in mastitis. Further, the isolation procedures tend to differ considerably between different studies.
Second, when considering studies in which presence of mecA was confirmed (=true MRSA), other essential data can be missing. In France, Alves et al. recently detected true MRSA in S. aureus strains collected from mastitic milk and from the nares of cows. However, they did not mention from which body site the MRSA strains originated [Reference Alves157].
Third, prevalence of true MRSA in mastitis can be assessed at different levels, i.e. quarter-, cow- and farm-level. Some studies did not provide sufficient data to show on which level their data should be interpreted. For example, a Hungarian study found true MRSA in milk samples from subclinical mastitis originating from only one farm, but it was not elucidated whether all samples originated from different cows or from different quarters. Moreover, it was a longitudinal study, with samples being taken over a 2-year period [Reference Juhász-Kaszanyitzky158]. In South Korea true MRSA was found in bovine milk specimens. However, the total number of milk specimens was not given and not all samples originated from mastitis [Reference Lee159]. In another report the same author found true MRSA in a specific number of milk samples from different cows, although in this case the number of milk samples originating from mastitis cases was not specified [Reference Lee160].
Only two studies, from South Korea, give adequate information, and they show that the quarter-level prevalence of MRSA in mastitis is very low, ranging from 0·18% [Reference Kwon58] to 0·4% [Reference Moon161].
A factor that not so much influences a correct estimation of the MRSA prevalence in mastitis but is important from an epidemiological point of view is the origin of the MRSA strains. Using biotyping methods the first detection of MRSA in mastitis was presumed to be of human origin [Reference Devriese and Hommez103]. Yet, from the other above-mentioned studies, many did not include information or hypotheses on origin of the strains [Reference Erskine154–Reference Turutoglu, Ercelik and Ozturk156, Reference Moon161]. Most of those that did found a probable human origin [Reference Kwon58, Reference Juhász-Kaszanyitzky158–Reference Lee160], although the presence of bovine-specific MRSA strains was also suggested [Reference Cuteri153, Reference Alves157]. Recently, however, LA-MRSA has been found to be present in Belgian cases of clinical and subclinical mastitis [Reference Vanderhaeghen62]. It was shown that nearly 10% of Belgian farms suffering from S. aureus mastitis were affected by LA-MRSA and that farm-level prevalence of LA-MRSA in positive farms varied between 3·9% and 7·4% [Reference Vanderhaeghen62]. In Germany LA-MRSA has also been found in mastitis [Reference Monecke141].
As the colonization capacity of LA-MRSA in dairy cattle has not yet been investigated and the current data on LA-MRSA are still sparse, the exact burden of LA-MRSA for dairy cattle farming is unclear. However, it can be reasonably suspected that the infection of dairy cattle with LA-MRSA will grow in the future. The many species in which it has been found shows that LA-MRSA is relatively unspecific in its host colonization and might thus very well find a host in cows. In addition, although this has not been substantiated by evidence, the small timescale in which LA-MRSA has attained these different species and has spread to different countries suggests that LA-MRSA can spread easily. As β-lactam antibiotics, β-lactamase-sensitive as well as -stable, are among the most frequently used antibiotics for treatment of mastitis and tetracyclines, macrolides and aminoglycosides are also often included in the treatment or prevention schedule [Reference Sawant, Sordillo and Jayarao162–164], the typical resistances LA-MRSA exhibits against these antibiotics might cause serious problems for treatment of mastitis. Because risk factors (repeated or enduring contact with a contaminated source) and transmission routes (the milking machine and hands of the farmer) for the spread of normal mastitis-causing S. aureus are likely to be the same for spread of LA-MRSA, future research might want to focus on these topics.
While we have found no real evidence that non-LA-MRSA has ever been isolated from another body part of living cattle other than the udder, LA-MRSA has been found in the nose of beef calves. In The Netherlands, in one farm 50% of the beef calves appeared to be carrying LA-MRSA in their nose [Reference Mooij114]. As for dairy cattle, more research is required to elucidate whether LA-MRSA has a true reservoir in beef calves.
Poultry
The first report on MRSA in poultry came from South Korea, where MRSA was isolated from chicken arthritis cases. With RAPD typing the MRSA strains appeared highly similar to each other, and they were suggested to share a common ancestor with MRSA strains isolated from humans and bovine milk [Reference Lee160]. Three years later three MRSA isolates were reported, again in South Korea [Reference Lee159]. On the basis of a comparison of the sequence of the mecI gene with human strains, one strain was suspected of being human. The other had a mecI sequence that was previously undetected in humans suggesting these strains were animal specific.
More recent reports concern the detection of LA-MRSA in or associated with poultry. First, a LA-MRSA strain was isolated from chicken droppings in The Netherlands [Reference Leenders113]. LA-MRSA was also detected in Belgian poultry, where LA-MRSA was found in a collection of recent S. aureus isolates from nares and cloaca of industrial broilers in Belgium [Reference Nemati61]. In another Belgian study LA-MRSA was found in broiler chickens but not in laying hens [Reference Persoons124]; the reasons for this are unclear. However, as antibiotics are rarely used in layers, differences in antibiotic use may account for it.
The amount of data is currently too sparse to draw consistent conclusions on LA-MRSA in poultry. It is unclear whether LA-MRSA has an impact on animal and poultry farmer health. More investigations are needed to further elucidate the epidemiology.
MRSA on livestock-derived food products
As well as its importance as a hospital and community pathogen, S. aureus is also a well-known cause of food intoxication [Reference Mead165, Reference Le Loir, Baron and Gautier166]. S. aureus food poisoning is the result of the production of staphylococcal enterotoxins, of which many types have been found in strains of S. aureus [Reference Le Loir, Baron and Gautier166, Reference Ono167]. Although these enterotoxins function as superantigens, i.e. they cause immunosuppression and trigger non-specific proliferation of T cells, leading to high fever, the clinical outcomes of S. aureus food poisoning are mostly relatively mild [Reference Mead165]. Therefore, it is estimated that the actual number of foodborne illnesses caused by S. aureus is much higher than those reported [Reference Mead165].
In contrast, MRSA food poisoning is very rare. The only report on MRSA food poisoning comes from the USA, where three adults became mildly ill after they had eaten coleslaw contaminated with an MRSA producing enterotoxin C [Reference Jones168]. This strain probably came from a food handler in the market place where the coleslaw was bought and was possibly of hospital origin [Reference Jones168].
MRSA of human origin can also be found on meat. In The Netherlands, an MRSA strain isolated from raw pork appeared upon genotyping to be identical to a well-known human clone, USA300 (ST8-MRSA-IV) [Reference van Loo169]. Moreover, also in the USA, MRSA clone USA300 was found on raw pork [Reference Pu, Han and Ge170]. In that study another widespread human clone, USA100 (ST5-MRSA-II), was found on raw pork and on a sample of raw beef. Further, in South Korea MRSA of likely human origin was found twice on chicken meat [Reference Lee160, Reference Kwon171]. While in Jordan MRSA of suspected human origin was found on chicken meat [Reference Quddoumi, Bdour and Mahasneh172]. All these studies failed to report whether the detected strains were capable of producing enterotoxins. In Japan, however, a MRSA strain of human origin isolated from raw chicken samples appeared capable of producing enterotoxin C [Reference Kitai173].
The aforementioned studies did not elucidate the source of contamination of the meat. The investigated meat was selected from retail shops or meat markets. However, no research was done on the presence of MRSA in the people working in these places. There was one study from Taiwan in which 18 meat market workers were shown to carry MRSA in their nose [Reference Hsieh174], although in this study no meat was examined.
As humans are capable of contaminating meat, it seems reasonable that human MRSA on meat can also contaminate persons handling or eating contaminated meat. This could be an important route for transmission of MRSA in the community or the hospital. The risk this could pose was illustrated by a Dutch hospital outbreak of MRSA in two hospital units. The outbreak was probably initiated by transmission of MRSA via food contaminated by an MRSA-positive healthcare worker involved in food preparation [Reference Kluytmans175]. In total, 14 healthcare workers and 27 patients were involved and of 22 patients that subsequently developed clinical disease, four died. Unfortunately, the initial food specimen involved could not be revealed [Reference Kluytmans175].
Indeed, in this regard, it should be borne in mind that proving a food specimen to be a source of MRSA contamination is likely to be difficult in studies investigating this. By definition, food is sold or bought to eat. Consequently, unless (clinical) results follow immediately upon eating or handling contaminated food specimens, contamination is likely to pass unnoticed initially and by the time it is noticed, if it ever is, the contaminated specimen will often be sold, eaten or thrown away. Hence, contaminated food could hitherto have been much more involved in cases of MRSA colonization or infection than has been reported. Although none of the above-mentioned studies on human MRSA strains on meat reported contamination events due to contact with the contaminated meat, it is perhaps premature to suggest that meat contaminated with human MRSA poses low or no risk for consumers. More investigations, for instance under experimental settings, are needed to gain further insight into the actual risks. Conversely, it also seems unnecessary to make too much of the risks of contaminated food. Performing normal hygienic practices when handling foods should suffice until thorough research proves otherwise.
Of special interest in these matters is the emergence and wide dissemination of LA-MRSA in livestock, which raises the question of whether these strains are also present on derived meat and via this way could find an entry point for wider dissemination in the human population. A Dutch study proved relatively early that LA-MRSA could be present on pork [Reference van Loo169]. A very recent and much larger Dutch study confirmed this, and showed in addition a wide dissemination of LA-MRSA on many different meat products. In that study, 2217 raw meat products were investigated and MRSA strains were isolated from no less than 264 samples (11·9%). An overwhelming 85% of the strains were LA-MRSA [Reference de Boer137]. The highest isolation percentages were found in turkey, chicken and veal meat. Not only is this clear proof that LA-MRSA has found its way into the food chain, it is also significant because LA-MRSA was present in turkey, lamb and sheep meat while currently no living carriage of LA-MRSA has been reported in these animals. Despite this relatively high number of meat products contaminated with LA-MRSA, so far there are no signs that this has contributed significantly to the dissemination of LA-MRSA to humans. This may be at least partly due to the very low numbers in which LA-MRSA was found present on the meat [Reference de Boer137]. However, the comments concerning the difficulties of proving this, as in the case of human MRSA on meat, are also applicable for LA-MRSA.
In addition to meat, another livestock-derived food product that could lead to MRSA food intoxication or serve as vehicle for MRSA transmission is raw milk, i.e. when contaminated raw milk is used in the production of cheese. This was reported in Italy, where two MRSA strains of unknown origin were found in dairy cheese products [Reference Normanno176]. As these strains were found to harbour genes for expression of common staphylococcal enterotoxins, they had the potential to cause food poisoning [Reference Normanno176].
Origin and molecular evolution of LA-MRSA
LA-MRSA seems to be primarily associated with pigs. However, since little is known regarding healthy carriage of S. aureus in pigs and the staphylococcal species dominating in infections of pigs is S. hyicus [Reference Devriese4, Reference Hermans, Devriese, Haesebrouck, Gyles, Prescott, Songer and Thoen6], the emergence of LA-MRSA in pigs was not expected, and raises questions on origin and evolution of MRSA ST398.
From MSSA ST398 to MRSA ST398
As both MRSA ST398 and MSSA ST398 have been described, it can be assumed that MRSA ST398 evolved from MSSA ST398 by acquisition of SCCmec. Two issues relate to this event: the origin of MSSA ST398 and the circumstances concerning the acquisition of SCCmec by MSSA ST398. Unfortunately, very little data are available to elucidate either of these issues.
Currently, MSSA ST398 has only been described in humans [41, Reference Armand-Lefevre, Ruimy and Andremont147, Reference Bhat177] and in pigs [Reference Guardabassi, Stegger and Skov112, Reference Armand-Lefevre, Ruimy and Andremont147], suggesting that one of these species is the original host of MSSA ST398. Although it has been described more often and previously (in 1997) [41] in humans than in pigs (first occasion 2005) [Reference Armand-Lefevre, Ruimy and Andremont147], the results of an early French study support the view that MSSA ST398 is primarily pig-associated. In that study ST398 was found in certain MSSA clones that were prevalent in healthy pig farmers but not in healthy non-farmer controls. As ST398 MSSA strains were also present in infections from pigs, animal to human transmission of this clone was suggested [Reference Armand-Lefevre, Ruimy and Andremont147]. Furthermore, the low number of detections and the relatively late date of the first description in pigs could easily be due to the fact that, following from the low pathogenic relevance of S. aureus in pigs, there has been little reason for MSSA (ST398) isolates to be detected in pigs. Nonetheless, it seems that more data are necessary to reliably conclude that MSSA ST398 is essentially pig-specific. Unfortunately, conversely to humans, very few databases of S. aureus strains isolated from pigs are available, making it difficult to assess the prevalence of MSSA ST398 in pigs before recent reports.
The issue of SCCmec acquisition by MSSA ST398, evolving into MRSA ST398, is equally difficult to address. In general, little is currently known on the origin of SCCmec and mecA and on the epidemiology and mechanisms of SCCmec acquisition. Apart from the very origin of mecA in S. aureus, which is proposed to have involved a mecA homologue present in the coagulase-negative species S. sciuri [Reference Couto178–Reference Antignac and Tomasz181], it is generally assumed that the presence of SCCmec in a certain MRSA clone has been preceded, somewhere in the evolution of that clone, by a horizontal transfer of SCCmec from another source [Reference Robinson and Enright71]. This source might be another MRSA strain carrying the specific SCCmec element or, which is often suggested, a methicillin-resistant non-S. aureus Staphylococcus (MRNaS), in which various SCCmec elements are known to be present [Reference Suzuki, Hiramatsu and Yokota182–Reference Hanssen and Ericson Sollid184] and which thus could function as a reservoir for SCCmec. However, very little is known on such transmission events. Although the cassette chromosome recombinase(s) contained in the ccr complex of SCCmec are known to be responsible for integration and excision of the entire SCCmec [Reference Ma31, Reference Ito32], actual transmission events of whole SCCmec elements are very difficult to prove, and the few reports on such events largely depend on interpretation of indicative epidemiological and typing data. For example, using such data, a Swedish study recently suggested horizontal transfer of an SCCmec type V between clinical isolates of methicillin-resistant S. haemolyticus and MSSA ST45 [Reference Berglund and Söderquist185]. Yet, without further evidence, the role of MRNaS in the horizontal transfer of SCCmec to MSSA and the frequency of SCCmec transmission events between staphylococcal strains remains mere speculation.
The same applies for explaining how LA-MRSA evolved from MSSA ST398 strains. With the assumption that MSSA ST398 is originally pig-associated, it can be proposed that SCCmec transmission occurred in pigs, with MRNaS or MRSA as donor species. However, sparse literature is available on presence of MRNaS in pigs, leaving any such donor species open to speculation. Moreover, presently, MRSA strains other than LA-MRSA have rarely been reported in pigs (see above). Nonetheless, as LA-MRSA mostly harbours SCCmec types IVa and V and the smaller SCCmec types IV and V are considered to be typically carried by CA-MRSA, human CA-MRSA strains could very well have been the donor species. Indeed, human MRSA strains could be present more frequently in pigs, because, similarly to MSSA in pigs, the availability of few reports could be due to a lack of studies investigating MRSA in pigs. However, an interesting idea is that the acquisition of SCCmec by MSSA ST398 did not occur in pigs but in humans. This would be in accord with the earlier detection of MRSA ST398 in humans than in pigs. Indeed, before the first report on LA-MRSA in pigs [Reference Voss109], MRSA ST398 was detected in a Dutch woman [41, Reference Huijsdens131] and in a French pig farmer [Reference Armand-Lefevre, Ruimy and Andremont147]. A possible course of events could thus have been that after transfer to one or more humans, probably farmers, an MSSA ST398 strain from pigs acquired SCCmec from a CA-MRSA strain, and after recolonization of one or more pigs, such MRSA ST398 strain started to spread among other pigs. The fact that MRSA ST398 was first detected in The Netherlands and France does not necessarily imply that these events originated in one of those countries. The Netherlands especially seems an unlikely country, as MRSA prevalence in both the hospital and community is one of the lowest of the world.
Regardless of the exact facts of these matters, the fact that multiple SCCmec types have been identified in LA-MRSA indicates that LA-MRSA must have arisen on multiple occasions from MSSA ST398 strains. This raises other questions. For example, it is not clear whether the two most frequently detected types, IVa and V, were already present from the very beginning, or whether one element was responsible for the first cases and the other was acquired later on. The very first reports of ST398 MRSA did not include information on the SCCmec type present [41, Reference Voss109, Reference Huijsdens131, Reference Armand-Lefevre, Ruimy and Andremont147]. Moreover, several untypable SCCmec elements have been found in LA-MRSA but it is unknown exactly how many different elements LA-MRSA carries. In addition, since LA-MRSA has been detected in a large geographical area and in many different species, the acquisition of SCCmec could have occurred in different species and different countries. However, until now MSSA ST398 has only been reported from the Cape Verde islands [41], France [Reference Armand-Lefevre, Ruimy and Andremont147], the USA and the Dominican Republic [Reference Bhat177] and was found only in humans [41, Reference Armand-Lefevre, Ruimy and Andremont147, Reference Bhat177] and pigs [Reference Armand-Lefevre, Ruimy and Andremont147].
In conclusion, the origin and evolution of LA-MRSA following acquisition of different SCCmec elements by different MSSA ST398 strains involves many unanswered questions, which for the moment can only be speculated upon. The lack of long-term data on the presence of S. aureus in pigs – MSSA as well as MRSA and human as well as non-human strains – makes it plausible to fear that many of these questions will remain unanswered.
Acquisition of PVL
In addition to the SCCmec issues, another event that warrants consideration when attempting to unravel the molecular evolution of LA-MRSA is the acquisition by certain strains of the genes encoding PVL. Strains of MRSA CC398 possessing PVL genes have been found to cause human infections in China [Reference Yu140] and Sweden [Reference Welinder-Olsson186]. However, most of MRSA CC398 strains that have been investigated appeared not to possess these genes, even when the strains originated from infections (e.g. [Reference van Loo116, Reference Witte119, Reference Ekkelenkamp130, Reference Krziwanek, Metz-Gercek and Mittermayer134, Reference Smith149, Reference Declercq187]).
It is not clear how the aforementioned LA-MRSA strains acquired PVL. The genes encoding PVL are carried on mobile genetic elements (MGEs) [Reference Kaneko and Kamio188]. As both cases reported no link between patient and animal contact but instead found medical histories of patients typical for HA-MRSA [Reference Yu140] and CA-MRSA [Reference Yu140, Reference Welinder-Olsson186], it perhaps concerns that LA-MRSA strains might have already been present over a longer period in the hospital or community environment and might have acquired PVL from human MRSA strains.
Despite the fact that the importance of PVL as virulence factor remains controversial [Reference Badiou88, Reference Gillet91, Reference Voyich93, Reference Bubeck Wardenburg94], these cases illustrate the potential of LA-MRSA to take up virulence factors on MGEs. A further spread of such MGEs in LA-MRSA may impose a serious risk for both human and animal health, seen the wide dissemination of LA-MRSA in some animals and its potential to colonize and infect humans.
LA-MRSA in humans
Since the medical significance of LA-MRSA for veterinary medicine is currently rather low, perhaps the most worrying aspect of LA-MRSA is its apparent capacity to transfer between its animal carriers and humans in close contact with them. This has been extensively shown in pig farming. Several studies, from many different countries, found that living or working on a farm with colonized pigs were risk factors for LA-MRSA carriage [Reference Voss109, Reference van Loo116, Reference Khanna121, Reference Denis123, Reference Lewis132, Reference Wulf133, Reference Smith149]. In The Netherlands, an increased risk of LA-MRSA carriage has also been shown in veal calf farming [Reference Mooij114, Reference van Loo116, Reference Van Rijen, Van Keulen and Kluytmans189, Reference Graveland, Wagenaar and Heederik190], even though actual carriage of LA-MRSA by veal calves has been reported only once [Reference Mooij114]. An increased risk has not yet been shown for dairy or meat cattle farmers. To a lesser extent, poultry farmers have also been found colonized as a result of LA-MRSA carriage by their animals [Reference Leenders113]. In addition, veterinarians working with pigs and cattle were found to be at higher risk for carriage of LA-MRSA [Reference Voss109, Reference Wulf110, Reference van Loo116, Reference Wulf122, Reference Denis123].
Despite the fact that the transferring capacity of LA-MRSA was very clear from first reports [Reference Voss109, Reference Huijsdens131], at present many features concerning it remain unclear. For example, while such an extensive transfer between humans and animals has not been reported for any other clone of MRSA, it can be assumed that LA-MRSA possesses special mechanisms or characteristics. However, this assumption has not yet been verified, and consequently, possible mechanisms have not yet been elucidated. Furthermore, only sparse information is available on actual risk factors and associated transmission routes. Similarly to other HA-MRSA and CA-MRSA, the most obvious risk factor and route of transmission of LA-MRSA is direct contact with colonized patients, i.e. animals. This has been proven in pig and veal calf farming [Reference van Loo116, Reference Graveland, Wagenaar and Heederik190]. Although this can be assumed to be important in other animal farming activities, this has not been substantiated with evidence thus far. Further, the duration of animal contact and the percentage of MRSA-positive animals were shown to be risk factors in veal calf farming. In addition, contact with a contaminated environment has also been suggested [Reference Hsieh174]. Such environment could also include the air, as studies have shown that air in pig and cow stables may contain considerable amounts of (antimicrobial resistant) bacteria [Reference Matos191, Reference Gibbs192]. This has, however, not been investigated for LA-MRSA.
In general, more research is urgently needed to gain further information on risk factors and transmission routes of LA-MRSA. This is an essential requirement for efficient control measures to be implemented, and is of particular importance, since, in addition to its capacity to colonize humans, LA-MRSA has also been found capable of causing infections in humans. LA-MRSA has been isolated from (severe) infections of people in close contact with pigs [Reference van Loo116, Reference Lewis132, Reference Krziwanek, Metz-Gercek and Mittermayer134, Reference Declercq187, Reference Schneeberger and van Gend193, Reference Pan194] and poultry [Reference Ekkelenkamp130] (Table 4). There appears to be no association of LA-MRSA with certain clinical conditions, as was found in both invasive and skin-related infections (Table 4). In addition, it is not clear whether a decreased human health condition predisposes to development of LA-MRSA infections. Further, as noted for animal infections with LA-MRSA, it is currently unknown which virulence factors are of importance in human LA-MRSA infections. However, for the present, it seems that the virulence capacity and associated medical importance of LA-MRSA is much lower compared to traditional human HA- and CA-MRSA strains. Nonetheless, future research should urgently bring more insight to these matters.
Table 4. Human infections caused by LA-MRSA
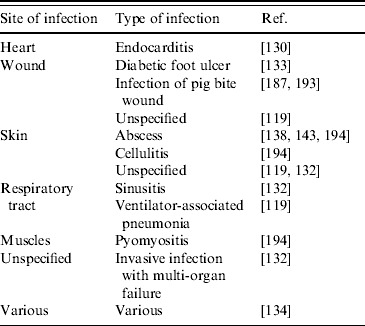
LA-MRSA, Livestock-associated methicillin-resistant Staphylococcus aureus.
Another important aspect of LA-MRSA in humans is that, although infrequently reported and not (yet) substantiated by epidemiological data, LA-MRSA appears to be capable of transfer between humans. In The Netherlands, a 6-month-old daughter of pig farmers, who presumably had not had direct contact with pigs, appeared colonized [Reference Voss109]. In that same study, the son of a veterinarian and the nurse treating the son in hospital also appeared to be carrying LA-MRSA [Reference Voss109]. This could create very dangerous situations, especially when personnel in medical settings carry LA-MRSA. In a Dutch hospital, a case was reported in which five healthcare workers and three patients appeared to carry LA-MRSA and two other patients were infected by LA-MRSA [Reference Wulf133]. Recently, also in a residential care facility for visually and intellectually disabled people a resident was diagnosed with dermal abscesses caused by LA-MRSA. Subsequent research revealed two other residents and three personnel members to be carrying LA-MRSA [Reference Fanoy138].
Contrary to what these cases suggest, LA-MRSA appears to be unable to disseminate widely in the community or hospital setting. LA-MRSA has been established in hospitals for several years but still no large dissemination has been reported. Nonetheless, it is clear that LA-MRSA could complicate the MRSA control measures for hospital settings, particularly in countries that perform strict control measures, such as The Netherlands [Reference Wertheim195]. Not only will the group of at-risk patients grow considerably, implying a higher number of screenings necessary at hospital admission, which is likely to lead to more people needing to be kept in isolation, thereby implying a serious burden for hospital accommodation and healthcare means. The relevance of this problem was shown by a recent Dutch study in which it was found that the inclusion of the new at-risk group of animal farmers had led to a threefold increase in annual MRSA incidence [Reference Van Rijen, Van Keulen and Kluytmans189].
Conclusions
MRSA in livestock should be regarded from two sides: MRSA CC398 and MRSA non-CC398. The few reports of MRSA non-CC398 in pigs and poultry concerned MRSA of human origin. There are no indications that these animals play a role as reservoir for re-infection of humans or that this will change in the near future. The situation in cattle is more complex. MRSA non-CC398 is detected more frequently than in pigs and poultry, almost solely from mastitis, indicating a problem for animal health. However, the size of the problem is difficult to assess. Quarter-level prevalence appears to be very low but cow-level and farm-level prevalence cannot be estimated due to a lack of consistent data.
Meat and milk are occasionally found to be contaminated with (human) MRSA non-CC398 strains. Although these food products have not yet been found to contribute to the dissemination of such strains in the community, care must be taken when drawing conclusions, due to a lack of thorough research. Until more studies have been performed normal hygiene measures and adequate preparation of the foods should suffice to contain this situation. This also applies in regard of the recent detection of MRSA CC398 on various meat products.
MRSA CC398 or LA-MRSA seems primarily associated with pigs but its origin is still largely unclear. Strains of this clone have spread around the world. In livestock, besides pigs also cows and poultry are affected. However, the origin and relevance of LA-MRSA in the latter animals currently remains unknown. The implication of LA-MRSA in animal infections may pose a burden on veterinary medicine but to what extent remains unclear, as do the factors responsible for the pathogenic potential of LA-MRSA.
An essential aspect of LA-MRSA is its remarkable degree of host unspecificity, also transferring between animals and humans. Possible mechanisms explaining this host unspecificity remain unknown. Compared to the extent of other types of MRSA, the impact on human healthcare is still small. However, the demonstration of the capacity of LA-MRSA to take-up toxin genes should urge the medical profession to take measures to control transmission and spread as quickly as possible. This will require thorough research to be performed to elucidate transmission routes and risk factors.
ACKNOWLEDGEMENTS
This work was funded in part by the Belgian Federal Public Service of Public Health, Food Chain Safety and Environment project number RF-6189 MRSA and by the European Project PILGRIM, FP7-Health-2007-2.3.1-4, contract no. 223050.
DECLARATION OF INTEREST
None.