INTRODUCTION
Cryptosporidium is associated with disease in at least 79 mammalian species including humans in addition to reptiles, amphibians, avian species and fish [Reference O'Donoghue1]. Cryptosporidiosis was recognized as a worldwide health problem in 2004 when the WHO included it as neglected disease. In 2012, the National Institute of Allergy and Infectious Diseases (NIAD) listed Cryptosporidium parvum as a ‘priority pathogen’ – an organism/biological agent able to exert emerging pathogen threats in the United States. Five species are mostly associated with human cryptosporidiosis cases, including C. hominis, C. parvum, C. meleagridis, C. felis, and C. canis [Reference Xiao2, Reference Xiao and Fayer3]. In industrialized nations, C. hominis and C. parvum are the most common species responsible for human infections. In cattle, significant disease first appeared when C. parvum, once considered to cause an opportunistic infection, occurred in association with neonatal diarrhoea in calves [Reference Pohlenz4]. Cryptosporidiosis now is considered an important aetiological component of the calf diarrhoea complex. Initial human cryptosporidiosis cases have been well documented in immunosuppressed individuals; the defining recognition of Cryptosporidium as a public health problem [Reference Thompson, Palmer and O'Handley5] came in 1993 with the world's largest recorded waterborne disease outbreak in Wisconsin, USA, involving more than 4 000 000 people [Reference MacKenzie6]. In developing countries, cryptosporidiosis is increasingly considered an important diarrhoeal disease in children that may lead to nutritional deficiencies and impairment of growth [Reference Ahs7, Reference Dillingham, Lima and Guerrant8].
The principal zoonotic reservoirs of cryptosporidiosis are humans, cattle, and other domestic animals. Cryptosporidium is transmitted by oocysts via the faecal–oral route, animal-to-person or from person-to-person, as well as by ingestion of contaminated water and food. The relative importance of transmission routes is not entirely clear, mainly due to the inability of traditional diagnostic tools to differentiate parasite species and genotypes [Reference Ramirez, Ward and Sreevatsan9]. An environment which comprises large numbers of infectious oocysts and large numbers of susceptible animals and humans predisposes the spreading of cryptosporidiosis cases [Reference Mosier and Oberst10]. Cryptosporidium oocysts remain viable in either soil or water for various periods under favourable environmental conditions of humidity and temperature [Reference Davies11] and can withstand chlorine disinfection and filtration of water [Reference Carpenter12]. In animal and human populations, an effective management of cryptosporidiosis requires better epidemiological information on the sources of infection, methods of spread and relative risk for different populations. Such knowledge on Cryptosporidium in developing countries is limited and mostly incoherent.
This study was conducted in Ismailia province, Egypt, 135 km east of Cairo. The province is located mostly in the middle part of the Suez Canal in the northeastern part of the Nile Delta. It consists of seven districts, which cover 25 villages and 712 sub-villages with about 1·05 million inhabitants and about 35 000 cattle and 26 000 buffaloes [13]. Fifty percent of the human population is rural.
Water is the limiting factor, only 0·0002% of the province's areas are feasible for agriculture. Human and large livestock populations are concentrated along the small, planted, canal-irrigated banks of the Suez Canal in Ismailia province and along 36 smaller sub-water canals, particularly the Ismailia canal system, running in an east–west direction from the Suez Canal [Reference El-Nahrawy14].
The majority of the animal breeders are mixed smallholders. Most (98%) of them have <0·4 ha with an average herd size of <10 animals. Most cattle are low-producing native crossbreeds, often held in mixed herds with buffaloes for milk production, with male animals and non-productive females fattened for meat [Reference El-Nahrawy14]. Livestock production is integrated, using some fodder crops and agricultural residues. Egyptian clover (berseem) is a key forage crop providing feed for the animals. Otherwise, feed sources are limited during summer and the farmers graze their animals on the banks of the irrigation channels, using canal water for watering the animals. Animals in the smallholder system are kept in close proximity to the home, either in corrals near the farmer's house or tied up in a barn constructed from locally available materials and employing husbandry methods in which hygienic quality is very poor.
Diarrhoea in children in Egypt is frequent, but seeking healthcare in hospitals, if at all, is impeded by long transportation, and particularly by the exorbitant cost of treatment, which represents almost 30% of a family's monthly income. Only 43% of healthcare seekers have health insurance. Hospital care for a child is often postponed for more than 3 days after onset of diarrhoeal symptoms [Reference Barakat and Halawa15].
In a recently published study we described the sampling and laboratory methodology and results of prevalence and genetic diversity of Cryptosporidium in livestock animals and children from an investigation of Ismailia province [Reference Helmy16].
In the current study, we used risk difference statistics and spatial scan statistics on spot maps to better understand the infection dynamics of Cryptosporidium in and among the livestock animals and children. The results illustrate the potential of a population-approximated study in geographical areas and may assist the planning of targeted control programmes against Cryptosporidium in Ismailia province.
MATERIALS AND METHODS
Study design and sample collection
Animal sample collection was based on an estimated number of 6700 holdings in Ismailia province (Cooperative counts). A representative sample scheme [Reference Helmy16] was obtained by weighing the calculated number of 191 sample herds proportional to the distribution of holdings in the seven districts of the province. Fayed district is located at the southern Suez Canal, Ismailia at central Suez Canal, expanding into the west at Ismailia canal, followed westwards along the canal by Kassasin and Abo swair districts, with El-Tal El Kabier located below them. West Kantara and East Kantara cover areas of the west and east banks of the northern part of the Suez Canal, respectively. Predominantly smallholder holdings were investigated and within herds the samples were allocated to certain animals within the herds. Accordingly, herds were sampled from April to June 2011 with an emphasis on calves as the risk group and some older diarrhoeic animals.
The sample size for humans was calculated using Win Episcope v. 2.0 program (www.clive.ed.ac.uk/winepiscope/), assuming a 50% prevalence. Stool samples were collected from 165 children (⩽10 years) with diarrhoea, hospitalized in one of the province's nine district hospitals or, in single cases, coming from farming households directly. All samples were collected from children after the consent of their parents during April–June 2011 (Table 1). Admission of diarrhoeic children to the hospitals depends on many circumstances, but stool samples are not routinely tested for Cryptosporidium. Therefore, sampling was not random.
Table 1. Numbers of collected samples according to location, species and age groups of livestock animals and children

Epidemiological information on the key demographics for each farm/herd and each child were collected via accompanying questionnaires. Attributes of animals (type of ground floor on which animals are kept, the source of water, clinical manifestations, and consistency of the faeces of each sampled animal) and humans (location of patient's residence, source of drinking water, consistency of the faeces, and contact with animals) were recorded using a standardized questionnaire that was completed by the senior investigator who asked all animal owners and the patients (or their parents) for the information. For identification of risk factors, the age and sex of animals and humans were also considered.
Diagnostics
Screening of Cryptosporidium was previously published in detail by Helmy et al. [Reference Helmy16] using the copro-antigen immunochromatographic dipstick test RIDA® QUICK Cryptosporidium/Giardia Combi (N1122) (R-Biopharm AG, Germany) and PCR for partial amplification of the 18S small subunit ribosomal DNA (18S rDNA) gene, followed by nested PCR and conventional PCR–RFLP assays for amplification and differentiation of Cryptosporidium spp. [Reference Helmy16–Reference Helmy19].
Statistical analysis
Statistical analysis was performed using mid-P exact probability tests to analyse the data; differences were considered significant for P values <0·05 (http://www.openepi.com). Prevalences in animal samples as identified by PCR were calculated by positivity by the RIDA® QUICK test and extrapolation of the PCR results from testing 10% samples negative by the RIDA® QUICK test; the 10% randomly drawn samples were assumed to be representative of the total of the RIDA® QUICK test-negative samples [Reference Lindley and Scott20]. Estimation of prevalences from cases/non-cases with 95% confidence intervals (two-sided) was performed according to Armitage & Berry [Reference Armitage and Berry21]. Odds ratios (ORs) were calculated with OpenEpi software (http://www.openepi.com). Locations of herds and of children (home address) were georeferenced. Cases/non-cases for locations were plotted as circles (humans) or triangles (animals) on an ArcGIS landuse shape file (http://www.geofabrik.de) of Ismailia province. The spatial scan statistic of SaTScan™ v. 9.11 software [Reference Kulldorff, Huang and Konty22] was used to investigate spatial clusters of animal or human cases and specifically the excess of their specific Cryptosporidium species in a given region. As the underlying animal and human populations at risk were unknown, the Bernoulli and the Poisson model options, respectively, of the scan statistic were used, with 0/1 event data for animal and human cases. SaTScan adjusts for the underlying spatial inhomogeneity of a background population. The spatial scan method is implemented by moving a circular window of variable radius systematically across the map, starting at the centroid of each location in the dataset. The radius of the window starts at 0 and expands to include the nearest region centroids; its maximum size does not exceed the specified maximum value of 50% of the total population. The cluster assessment is performed by comparing the number of cases within the window with the number expected if cases are randomly distributed in space. The test of significance of an identified cluster is based on a maximum likelihood ratio test. The Bernoulli and Poisson processes are used to calculate maximum likelihood ratios and compare them to the distribution of ratio values of random datasets generated by Monte Carlo replicas under the null hypothesis of no clustering. Maximum likelihood constitutes the most likely cluster, being the cluster that is less likely to have occurred by chance. The null hypothesis of no clusters was rejected when the simulated P value was ⩽0·05. The calculated relative risk indicates how many more cases are likely to occur within than outside the cluster.
RESULTS
The 191 animal herds were from 57 locations, the 165 diarrhoeal children from 45 locations of Ismailia province. By the RIDA® QUICK screening test, 46·6% (89/191) of the herds were identified as Cryptosporidium positive, with 19·5% (157/804) individual animal samples reacting positively. In cattle, the prevalence was 20·4% (114/593) and in buffaloes it was 19·2% (43/211). By contrast, only 6·7% (11/165) of human samples were positive for Cryptosporidium [Reference Helmy16].
Using PCR results, Helmy et al. [Reference Helmy16] calculated a total extrapolated crude herd prevalence of Cryptosporidium of 73·3% (140/191) and a respective total individual animal prevalence of 32·3%. The 31·2% positive cattle and the 35·5% positive buffalo prevalences (Table 2) were not different (P < 0·05). The age-specific joint calf prevalence was 43·0% in the 1 day to 3 months age group, which was significantly higher (P < 0·05) than that of 30·1% in calves aged >3 months to 1 year, 25·9% in animals aged >1 year to 2 years, and 15·8% in cattle and buffaloes aged >2 years. The odds of positive PCR results for Cryptosporidium was 2·4 times higher for the youngest calf age group than for older calves. Between sexes, the 35·9% (118/329) prevalence of males was statistically not different from the 29·9% (142/475) prevalence of females. The prevalences of Cryptosporidium in diarrhoeic animals and in animals with clinical symptoms of fever and dehydration were 38·1% (OR 2·4) and 46·7% (OR 1·9), respectively, both rates being significantly higher than for animals with normal faeces and no acute clinical signs despite emaciation (20·4% and 30·6%, respectively). Between districts, prevalence was the same for 6/7 districts, with differences only occurring between single districts Abo swair (40·4%) and East Kantara (46·3%) vs. El-Tal El Kabier (25·3%). The odds of high prevalence in East Kantara was 2·4 times that of the other districts. For husbandry risk factors, Cryptosporidium prevalence in animals kept on muddy rather than sandy dry ground was the same but significantly increased in animals that were watered with canal or underground water (49·8%, OR 1·6) rather than tap water (34·2%) (Table 2). In our companion paper [Reference Helmy16] we reported the total Cryptosporidium prevalence in children as 6·7% (11/165) by the RIDA® QUICK screening test and 49·1% by PCR. Using PCR results, prevalence in children was significantly higher than in the animal samples (P < 0·001). A prevalence of 57·5% in 1½- to 6-year-old children (Table 3) was significantly higher (OR 3·6) than in younger (1 month–1·5 years) and in older (>6 years) age groups. Moreover, the prevalence of Cryptosporidium differed between locations, with El-Tal El Kabier (75·0%, OR 5·5) and Kassasin (76·5%, OR 5·9) showing significantly higher prevalences than other districts. PCR results also revealed that prevalence was significantly higher in children with watery and pasty stools (52·7%, OR 5·2, P = 0·01) than in those with normal stools (17·6%) [Reference Helmy16]. Concerning risk factors, children who consumed underground water had a significantly higher prevalence of 66·7% (OR 3·0) than children who consumed tap water (39·8%). Likewise, prevalence was higher (60·9%, OR 2·3) in children living in villages than in city children (37·3%). Contact with animals had a significant effect on prevalence; prevalences of 75·8% in children that had a history of contact with ruminants and about 42·3% in children with previous contact with chickens were significantly higher (ORs 4·3 and 5·2, respectively) than in children for which no history of contact with animals was recorded (Table 3).
Table 2. Cryptosporidium PCR-based herd and individual animal prevalences with 95% confidence intervals and odds ratios
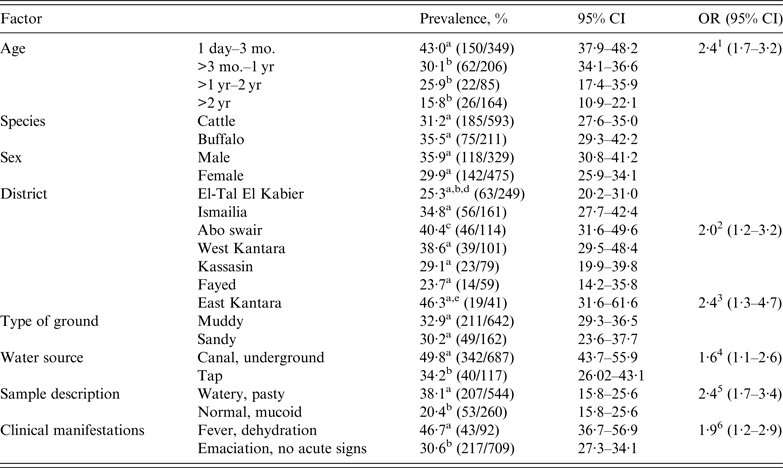
OR, Odds ratio; CI, confidence interval.
Different superscripts (a,b,c,d) within a factor category indicate significant prevalence differences at P< 0·05.
1 The prevalence of Cryptosporidium on average in the 1 day–3 mo. age group was 2·4 times greater than in the other age groups.
2 The prevalence of Cryptosporidium on average in Abo swair was 2·0 times greater than in the other districts.
3 The prevalence of Cryptosporidium on average in East Kantara was 2·4 times greater than in the other districts.
4 The prevalence of Cryptosporidium on average was 1·6 times greater in animals watered with canal/underground water than in those receiving tap water.
5 The prevalence of Cryptosporidium on average was 2·4 times greater in animals with watery, pasty faeces than in those with normal, mucoid faeces.
6 The prevalence of Cryptosporidium on average was 1·9 times greater in animals with fever, dehydration than in those with emaciation but no acute signs.
Table 3. Cryptosporidium PCR-based prevalences of paedriatic children with 95% confidence intervals and odds ratios

Different superscripts (a, b, c) within a factor category indicate significant prevalence differences at P< 0·05.
1 The prevalence of Cryptosporidium on average in the 1½–6 yr age group was 3·6 times greater than in the other age groups.
2 The prevalence of Cryptosporidium on average in El-Tal El Kabier district was 5·5 times greater than in the other districts.
3 The prevalence of Cryptosporidium on average in Kassasin district was 5·9 times greater than in the other districts.
4 The prevalence of Cryptosporidium on average was 3·0 times greater in children consuming underground water rather than tap water.
5 The prevalence of Cryptosporidium on average was 2·3 times greater in children living in villages than in cities.
6 The prevalence of Cryptosporidium on average was 4·3 times greater in children having had contact with ruminants than in those having no contact.
7 The prevalence of Cryptosporidium on average was 5·2 times greater in children having had contact with chickens than in those having no contact.
8 The prevalence of Cryptosporidium on average was 5·2 times greater in children with watery, pasty stools than in those with normal, mucoid stools.
Most infections in animals were caused by C. parvum (65·7%), followed by single infections with C. ryanae and C. bovis and three double infections of C. parvum with C. ryanae, C. bovis and C. andersoni each [Reference Helmy16]. No differences in the distributions of Cryptosporidium spp. were detected between cattle and buffaloes. C. parvum as major species was significantly more prevalent in the 1 day–3 month age group than in older animals, in males compared to females, and in animals that consumed canal or underground rather than tap water.
While C. parvum prevailed in livestock animals, the majority of species in the human samples were C. hominis (60·5%) and C. parvum (38·3%). C. parvum had a significantly higher prevalence (83·9%) in the mid-age (>1½–6 years) than in the younger and older children's age groups, slightly more frequent in Ismailia than in the other six districts and significantly (41·7%, Helmy et al. [Reference Helmy16]) more frequent in children having had contact with animals than those not having had contact (14·3%). Whether children had consumed tap or underground water did not statistically affect the prevalence of C. hominis (63·3% vs. 36·7%).
Formal cluster analysis did not identify animal clusters, neither for Cryptosporidium cases nor for C. parvum. In contrast, two such clusters were identified for human cryptosporidiosis cases and for C. hominis (circles in Fig. 1). Both clusters are located with almost the same centre in the medium-western part of Ismailia canal but the clusters are of different size. The human Cryptosporidium cases cluster (log likelihood ratio 8·3) has a radius of 17 km and incorporates 33 of the total 81 Cryptosporidium cases (20% of total population), resulting in a relative risk of 1·89 (P = 0·01). The C. hominis cluster (log likelihood ratio 7·7) has a radius of 26·2 km and covers 45 C. hominis identifications of the total 81 cases of this species (27% of total population) in the entire study area. The resulting relative risk was 1·70 (P = 0·02).

Fig. 1. Locations of space clusters of human cryptosporidiosis (—) and of C. hominis (-----) cases in Ismailia province, Egypt.
DISCUSSION
For Egypt, the present work provides the first geographical area-related results of population structures and dynamics of Cryptosporidium in and between livestock animals and children as a particular risk group of the average human population. Prevalence rates for Cryptosporidium may differ between countries and within the same country according to Ghenghesh et al. [Reference Ghenghesh23], who analysed data from Arabic countries where prevalence of cattle varied between 13·9% and 86·7% (mean 20·8%) and prevalences between 0% and 82·4% (mean 37%) for various, often very small, specimen numbers of immunocompromised human patient groups. The differences are mainly due to the sampling design, type of populations studied, husbandry and management system, location, season of study, sanitary conditions inside and around farms and diagnostic methods used.
The only recent study on Cryptosporidium prevalence in cattle [Reference Amer24] in another region of Egypt focused on some of the rare modern large farms rather than on the prevailing traditional smallholder farms and showed a prevalence of 30·2% in dairy calves aged <6 weeks which was similar to the 32·2% individual animal prevalence of the present study. Both studies underline that the Cryptosporidium problem in farm ruminants is not trivial and, so far, obviously has not received adequate attention.
The prevalence of Cryptosporidium spp. in calves did follow the known pattern of negative correlation with increasing age [Reference Singh25, Reference Nasir26]; age being a major risk factor led to a significant 2·7-fold increase in prevalence, from 15·9% in growing or adult cattle to 42·9% in calves. Predisposition to infection in calves could be due to several influences. Cryptosporidium sporozoites possess surface lectins that may mediate attachment of the organism to specific carbohydrates in the intestinal mucosa. The carbohydrate composition of the mucosa can be altered by age, intestinal microflora and diet. Immunological immaturity may also influence the age-related susceptibility to infection [Reference Mosier and Oberst10]. Watery or pasty faeces and clinical symptoms of fever and dehydration may serve as clinical diagnostic aids scince Cryptosporidium prevalence in both categories markedly increased compared to animals with normal faeces or without acute clinical signs.
The source of water was a major risk factor for animals. Animals consuming underground or canal water not only had a four times higher infection rate (80·2%) than animals supplied with tap water (19·8%), but also differed in Cryptosporidium species composition. C. parvum was 7·6 times more frequently detected in animals watered with underground/canal water than from tap water [Reference Helmy16]. Rayan et al. [Reference Rayan27] also confirm high concentrations of Cryptosporidium oocysts in Ismailia canal water, particularly those of C. parvum, and stress the importance of this water for zoonotic transmission. They observed that farm animals and humans were the major sources of Cryptosporidium oocyst contamination in surface water. Sewage is subjected to minimal treatment and effluent is discharged into canals, lakes and seas. Contamination of underground water or shallow wells often occurs at high quantity [Reference Khalifa, Yacout and Sadek28] by runoff surface water. Tap water from water-treatment plants which use rapid sand filters and sequentially add chlorine-based disinfectants is apparently not entirely free from Cryptosporidium, although it is of better hygienic quality, particularly in regards to C. parvum [Reference Abou-Eisha29]. In contrast to Youssef et al. [Reference Youssef, Khalifa and el Azzouni30] who detected no protozoa in Alexandria tap water using modified Ziehl–Nielsen staining, recent investigations [Reference Rayan27] using PCR confirmed 3% oocyst prevalence in Ismailia tap water.
Compared to the 32·2% prevalence of farm animals, the prevalence of 49·1% in the children cohort was significantly higher and child infections were clustered. One reason for the high prevalence might be the fact that the whole human sample originated only from diarrhoeic children while only 60·5% of samples originated from diarrhoeic animals [Reference Helmy16]. In a review in Egypt on inpatient and outpatient clinics, Youssef et al. [Reference Youssef, Khalifa and el Azzouni30] estimated a high Cryptosporidium prevalence of up to 47% for people with diarrhoea.
In the present study, Cryptosporidium infections of children were a result of a combination of their age and their place of residence. The principal age pattern observed (mostly children aged <5 years) was similar to other developing countries [Reference Bhattacharya31–Reference Gatei34]. In their review for the Arab world, Ghenghesh et al. [Reference Ghenghesh23] summarized that Cryptosporidium-associated diarrhoea occurs mainly in younger children and inversely correlates with greater prevalence in children aged ⩽1 year, particularly in rural and suburban regions. Abd El Kader et al. [Reference Abd El Kader35] identified exclusively for urban patients of the Egyptian capital Cairo that 61·9% of cryptosporidiosis cases occur in children aged <4 years, without clear differences between children aged 2 and 5 years. Abdel-Messih et al. [Reference Abdel-Messih36] reported on hospitalized children with diarrhoea in the rural Nile Delta and found that younger age (<12 months) was a risk factor for cryptosporidiosis.The majority of children in this study also were from rural areas. Surprisingly, however, is that from these diarrhoeal child patients from rural areas those aged <1½ years were found to be infected less often than children aged between 1½ and 6 years (when they enter primary school). One probable explanation is that breastfeeding children in the rural areas makes them less exposed to Cryptosporidium [Reference Bhattacharya31, Reference Abdel-Messih36].
As for animals, water was identified a risk factor for infection of children. Children consuming underground water had a significantly higher prevalence (66·7%, OR 3·0) than children who consumed tap water (39·8%). Likewise, contact with animals did increase the risk of infection of children about fourfold. Associations of human infections with exposure to infected animals, particularly calves, have been reported previously [Reference Ungar37, Reference Casemore38]. Shoukry et al. [Reference Shoukry, Dawoud and Haridy39] specified in their investigation of Ismailia province that 88% of C. parvum-infected children had been in contact with animals.
Cryptosporidium populations and factors associated with infections did differ between livestock animals and children. Single infections with C. parvum in about 2/3 of cases did prevail in animals, while concurrent human infections in about 60% of cases were due to C. hominis and close to 40% due to C. parvum. C. parvum was the most common species responsible for the majority of animal infections (65·7%). In animals, C. parvum infection points to environmental sources, particularly contaminated water and animal-to-animal transmission. C. parvum in humans is probably via animal-to-human (zoonotic) transmission and C. hominis via person-to-person (anthroponotic) transmission. In contrast to Rayan et al. [Reference Rayan27] we found a predominance of C. hominis over C. parvum in human infections as did other recent studies using molecular diagnostics [Reference Abd El Kader35]. El-Helalya et al. [Reference El-Helalya, Alyb and Attiab40] identified a predominant anthroponotic transmission due to C. hominis, particularly in urban Egypt. Such predominance of anthroponotic species as the leading cause of human cryptosporidiosis is recorded for industrialized nations such as Australia, Canada, Japan, USA and some developing countries like Peru, Thailand, South Africa. In some European countries, e.g. France, Belgium and England the relationship of C. parvum/C. hominis is more balanced and C. parvum dominates in The Netherlands or Italy. Wherever the anthroponotic transmission is not clear, subgenotyping of C. parvum isolates is necessary to characterize zoonotic or anthroponotic genotypes.
SatScan analysis addressed the question whether or not animal and human cases and their predominant Cryptosporidium species were clustered over and above the level that would be expected under natural environmental heterogeneity. If no clustering is detected, spatial randomness is suggested. SatScan scanned for clusters of geographical size between 0% and 50% of the population at risk as recommended [Reference Kulldorff, Huang and Konty22]. The program avoids arbitrary spatial aggregations through its continuously moving window. By this, pre-selection bias of identifying suspected locations can be avoided. The program rather adjusts for heterogeneous population densities in different study areas, being one result of non-true random samples, as was the case in this work. The ‘populations at risk’ consequently used by the program were the totals of cases and Cryptosporidium key species identified in animals and humans, respectively.
The spatial pattern analysis permits an improved understanding of disease dynamics and transmission when there is a strong correlation between the spatial distribution of disease, of hosts or of transmission risk factors. Such a situation is not suggested for Cryptosporidium total cases and those of the predominant C. parvum species for livestock of Ismailia province. Cryptosporidium cases and C. parvum being distributed randomly over the province would suggest no particular effect of animal husbandry, hygiene and herd factors like stocking rate.
By contrast, in humans two significant clusters of Cryptosporidium cases of children and those particularly caused by C. hominis were identified. Both clusters tended to occur not directly along the Suez Canal, with its close to 500 000 human population urban centre Ismailia, but along its main sub-canal, the Ismailia canal. Spatial analysis, in principle, agrees with the statistical analyses which also determined that El-Tal El Kabier and Kassasin districts, located in both circles, had significantly high numbers of human Cryptosporidiosis cases. It should be hypothesized that particular person-to-person hygiene risk factors in the handling of young children in the areas of these entirely rural districts are of particular importance compared to, for example, urban Ismailia. Abdel-Messih et al. [Reference Abdel-Messih36] point to contaminated food and bottles and El-Helalya et al. [Reference El-Helalya, Alyb and Attiab40] to mothers not washing their hands before feeding their children.
CONCLUSION
Our study estimates the importance of Cryptosporidium infections in animals and humans in Ismailia province. Animal cryptosporidiosis and C. parvum are randomly distributed over the province, while in humans cryptosporidiosis and C. hominis hotspots were identified at Ismailia canal, where higher, particularly person-to-person, transmission risks than in other areas of the province are likely.
Three infection modes exist, infection of young calves particularly with C. parvum from contaminated water and later on continuous animal-to-animal transmission of this particular species. Two distinct pathways of transmission of Cryptosporidium exist concerning human infection, zoonotic (bovine to human) and anthroponotic (human to human). Zoonotic infection is mainly due to C. parvum via contact with water and animals. Anthroponotic transmission is dominated by C. hominis. The need exists for further epidemiological studies on the impact of cryptosporidiosis for animal and human health. Furthermore, there is a need to implement appropriate control measures to interrupt the zoonotic pathways as well as anthroponotic infection chains in regard to animal and human cryptosporidiosis.
ACKNOWLEDGEMENTS
We thank Dr Hendrik Wilking for generating the map, Dr Juergen Kruechen for his guidance during species identification. We also thank Dr Ahmed Abdel-Maksoud and Dr Ahmed Sobieh for their help during samples collection. The German Academic Exchange Service (DAAD) and the Ministry of Higher Education in Egypt provided funds for the study.
DECLARATION OF INTEREST
None.